DOI:
10.1039/D2RA01294D
(Review Article)
RSC Adv., 2022,
12, 12672-12701
Recent advances in the application of magnetic bio-polymers as catalysts in multicomponent reactions
Received
25th February 2022
, Accepted 7th April 2022
First published on 26th April 2022
Abstract
Magnetic nanoparticles have attracted significant attention due to their high surface area and superparamagnetic properties. Bio-polymers composed of polysaccharides including alginate, cellulose, glucose, dextrin, chitosan, and starch can be immobilized on magnetic nanoparticles. Bio-polymers can be obtained from natural sources, such as plants, tunicates, algae, and bacteria. Bio-polymers obtained from natural sources have attracted attention due to their various properties including efficient functional groups, non-toxicity, low cost, availability, and biocompatibility. According to the targets of “green chemistry”, the application of bio-polymers is effective in reducing pollution. Furthermore, they are excellent agents for the functionalization of magnetic nanoparticles to yield nanomagnetic bio-polymers, which can be applied as recoverable and eco-friendly catalysts in multicomponent reactions.
1. Introduction
Among the various magnetic nanoparticles, nano magnetic iron oxide (Fe3O4) is very important because of its low cost, easy synthesis, and high magnetic ability. Recently, magnetic nanoparticles have been extensively applied in various fields including drug delivery, sensing, water treatment, removal of heavy metals, and catalysis. However, they are unstable in alkaline and acidic media due to the easy oxidation of their surface area.1–8 These drawbacks can be alleviated via the modification of Fe3O4 nanoparticles with materials such as silanes,9,10 activated carbon,11 and biocompatible polymers.12,13 Another class of magnetic nanoparticles is ferrite magnetic nanomaterials and hexaferrite (M-type). Ferrite magnetic nanomaterials and hexaferrite have various applications including high chemical strength materials, home appliances, supercapacitors, loudspeakers, electromagnetic wave absorption, and permanent magnets.14–18 Overall, due to the properties of ferrite magnetic nanomaterials and hexaferrite (M-type), they can be used for the adsorption of various metals ions, cationic and anionic dyes from wastewater.19–23
The design and synthesis of biocompatible magnetic nanoparticles are important subjects in green chemistry.24 Bio-polymers including alginate, cellulose, glucose, dextrin, chitosan, and starch are known as polysaccharides, which are present in the carbohydrates in plants, animals, microbes, and algae (Fig. 1).24 These bio-polymers have different properties such as biodegradable nature, biocompatibility, non-toxicity, availability, low cost, and heat resistantance.25 They are excellent agents for the functionalization of magnetic nanoparticles to yield nanomagnetic bio-polymers, promoting their longevity, hardness, and strength. These composites have many applications such as drug delivery,26–28 chemotherapy,29,30 magnetic resonance imaging (MRI) agents,31,32 solar cells,33 chemical sensors,34,35 catalysts,36 water treatment,37,38 and biomedical sensors.39
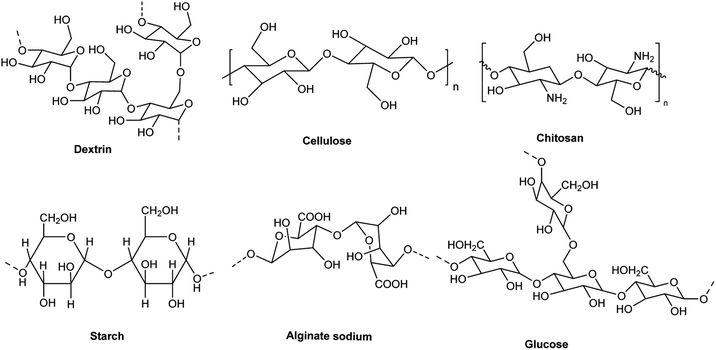 |
| Fig. 1 Various bio-polymers. | |
In comparison to conventional catalysts, heterogeneous magnetic bio-polymers have various advantages such as non-toxicity, easy separation, and eco-friendly nature. According to the functional groups on the magnetic nanoparticles, the catalysts can be grouped in various categories including, Lewis and Brønsted acids and bases.40,41 In continuation of our research,42–44 in this review, the importance of nanomagnetic bio-polymers is studied in multicomponent reactions. Multi-component reactions (MCRs) are essential tools in medicinal and organic chemistry, which have various advantages including simplicity, easy workup, availability, and reduced generation of waste. Hence, the design and application of MCRs for the synthesis of organic compounds are highly important.45–50
2. Synthesis of various magnetic bio polymers
2.1. Magnetic bio-polymers based on cellulose
2.1.1. Synthesis and application of Fe3O4/cellulose/Co-MOF nanocomposite. Initially, Fe3O4 nanoparticles 4 were modified with cellulose 5 using urea 6/NaOH 7 to provide Fe3O4/cellulose 8, which was reacted with Co(NO3)2·6H2O 9, terephthalic acid 10, and imidazole (IM) 11 in DMF to form Fe3O4/cellulose/Co-MOF nanocomposite 12 (Scheme 1).51
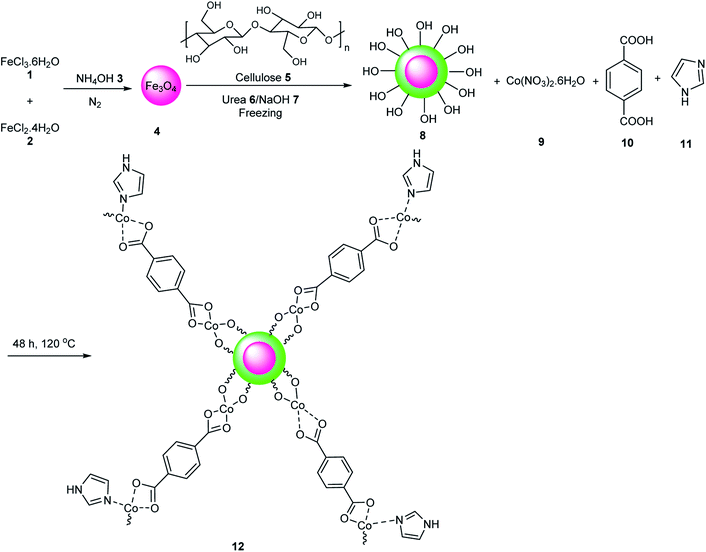 |
| Scheme 1 Synthesis of Fe3O4/cellulose/Co-MOF nanocomposite 12. | |
Fe3O4/cellulose/Co-MOF 12 has two sites including Lewis acidic sites (Co2+) and basic sites (IM), which were used in the Knoevenagel condensation reaction of aromatic aldehydes 13 with malononitrile 14 under solvent-free conditions (Scheme 2). This catalyst was reused five times without a decrease its catalytic activity.51
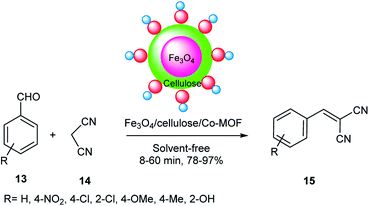 |
| Scheme 2 Knoevenagel condensation in the presence of Fe3O4/cellulose/Co-MOF 12. | |
2.1.2. Synthesis and application of Fe3O4@NCs/Sb(V). Initially, raw cotton was converted to nano-cellulose (NCs) 5 in the presence of NaOH 7, NaClO 16, and H2SO4 17 at 80 °C. Then, Fe3O4 was functionalized with nano-cellulose (NCs) 5, providing Fe3O4@NCs 8. In the next step, SbCl5 was mixed with the reaction mixture in chloroform to provide Fe3O4@NCs/Sb(V) 18 (Scheme 3).52
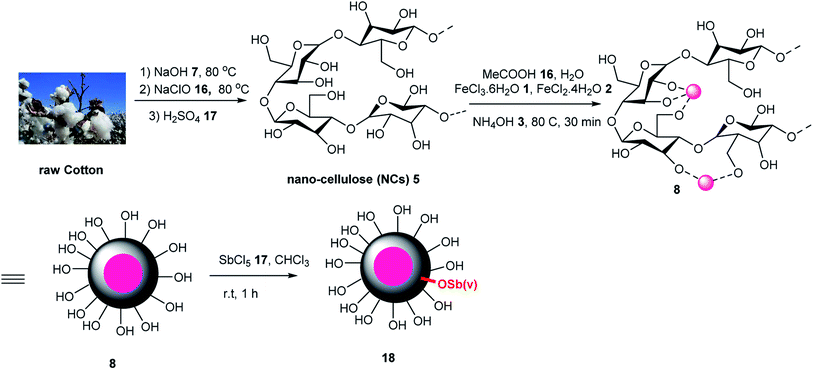 |
| Scheme 3 Synthesis of Fe3O4@NCs/Sb(V). | |
Fe3O4@NCs/Sb(V) was used as a Lewis acid for the activation of carbonyl groups, which was investigated in the synthesis of 4H-pyrimido[2,1-b]benzothiazoles 22 via the three-component reaction of aldehydes 19, 2-aminobenzothiazole 20, and ethylacetoacetate 21 under solvent-free conditions at 90 °C (Scheme 4). This catalyst was used five times without loss in its catalytic activity, which was compared with Fe3O4 with 39% yield in 3 h.52
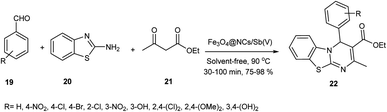 |
| Scheme 4 Synthesis of 4H-pyrimido[2,1-b]benzothiazole derivatives 22. | |
2.1.3. Synthesis and application of Fe3O4@NFC@Co(II). Fe3O4 MNPs were synthesized via the reaction of FeCl3·6H2O and FeSO4·7H2O in the presence of NH4OH solution at 80 °C under an N2 atmosphere. The Fe3O4 nanoparticles were dispersed in H2O, and then nanofiber cellulose (NFC) 24 was added to the reaction mixture at room temperature to obtain Fe3O4@NFC 25 precipitate, which was reacted with an ethanolic solution of cobalt(II) acetate to form Fe3O4@NFC@Co(II) 26 (Scheme 5).53
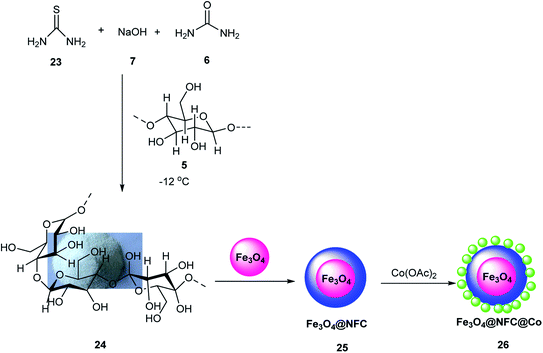 |
| Scheme 5 Synthesis of Fe3O4@NFC@Co(II) 26. | |
Its catalytic activity was useful for the synthesis of 4H-pyrans via the multicomponent reaction of aldehydes, ethylacetoacetate, and malononitrile in H2O (Scheme 6).53
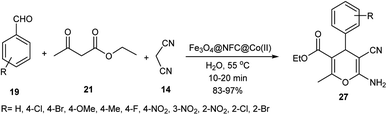 |
| Scheme 6 Synthesis of 4H-pyrans 27 in the presence of Fe3O4@NFC@Co(II). | |
Also, the catalytic activity of 26 was tested for the synthesis of pyranopyrazole derivatives 29 via the four-component reaction of hydrazine hydrate 28, ethyl acetoacetate 21, benzaldehyde 19, and malononitrile 14 in H2O (Scheme 7). The metal ions on the catalyst surface act as Lewis acids, which were activated by the malononitrile and carbonyl groups. Also, the catalyst was used five times in the model reaction without a reduction in activity.53
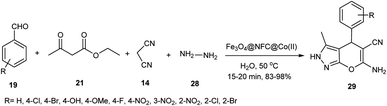 |
| Scheme 7 Synthesis of pyranopyrazole derivatives 29 using Fe3O4@NFC@Co(II). | |
2.1.4. Synthesis and application of cellulose@pumice. Initially, the microcrystalline cellulose was mixed with a solution of NaOH 7 and urea 6 in H2O, and then was cooled in an ice bath at 8 °C to form a gel solution, which was mixed with pumice powder 32 and stirred for 24 h to obtain cellulose@pumice 33 (Scheme 8).54
 |
| Scheme 8 Synthesis of cellulose@pumice 33. | |
Cellulose@pumice as an acidic catalyst activated carbonyl groups in the synthesis of 2,4,5-triarylimidazoles 36 through the reaction of benzaldehyde 19 and ammonium acetate 35 in EtOH under ultrasonic irradiation (Scheme 9). In the reusability test, this catalyst was used ten times without a reduction in activity.54
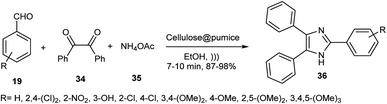 |
| Scheme 9 Synthesis of 2,4,5-triarylimidazoles derivatives 36. | |
2.1.5. The synthesis and application of (Fe3O4@NFC@NSalophCu)CO2H. Nanofiber cellulose 37 was functionalized with Fe3O4 via the sol–gel method to give Fe3O4@NFC nanoparticles 39, which were functionalized with (3-aminopropyl)triethoxysilane (APTES) 40 to obtain Fe3O4@NFC@APTES 41. The reaction of salicylaldehyde 42, paraformaldehyde 43, and HCl solution under reflux conditions provided 5-chloromethyl salicylaldehyde 44, which was reacted with 3,5-diaminobenzoic acid 45 in CH2Cl2 at room temperature to give the Schiff base 3,5-bis(((E)-5-(chloromethyl)-2-hydroxybenzylidene)amino) benzoic acid ((5-Cl-Saloph)CO2H) 46, followed by reaction with copper acetate in EtOH at room temperature to obtain complex [(5-Cl-Saloph)Cu(II)]CO2H 47. Finally, the reaction of [(5-Cl-Saloph)Cu(II)]CO2H complex 47 and Fe3O4@NFC@APTES 41 at 70 °C for 24 h gave (Fe3O4@NFC@NSalophCu)CO2H 48 (Scheme 10).55
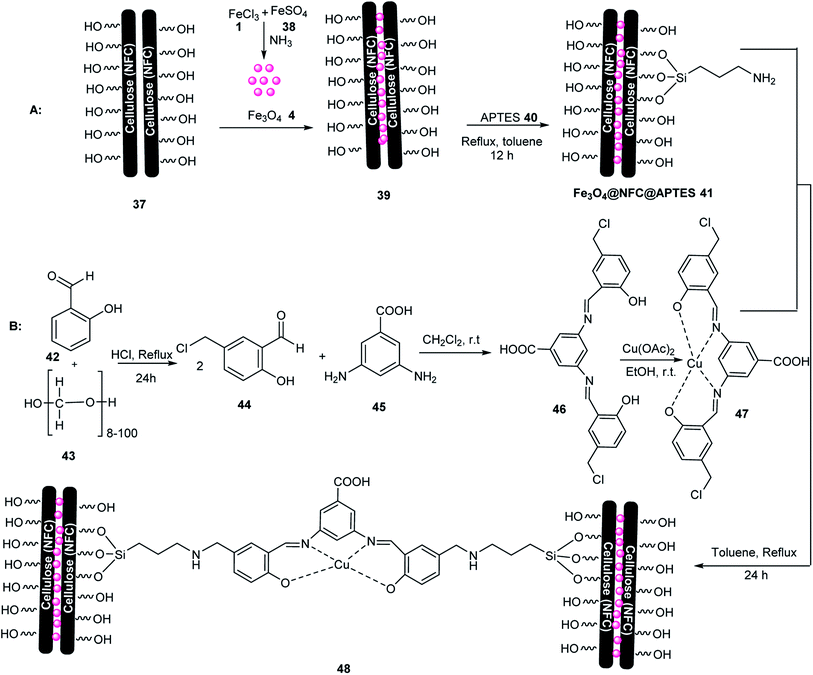 |
| Scheme 10 Synthesis of (Fe3O4@NFC@NSalophCu)CO2H. | |
(Fe3O4@NFC@NSalophCu)CO2H 48 was tested in the synthesis of 5-substituted-1H-tetrazole 51 via the multicomponent reaction of various aldehydes 19, hydroxylamine 49, and sodium azide 50 (Scheme 11), and also in the synthesis of 1-substituted-1H-tetrazoles 54 via the multicomponent reaction of aniline 52, triethyl orthoformate 53, and sodium azide 50 (Scheme 12). This catalyst was used in the click reaction four times without a decrease in its activity. In this catalyst, copper metal plays a vital role in the click reactions.55
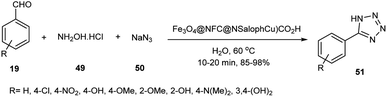 |
| Scheme 11 Synthesis of 5-substituted-1H-tetrazole 47 using (Fe3O4@NFC@NSalophCu)CO2H. | |
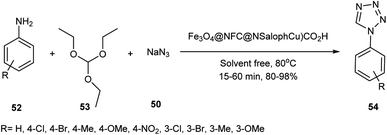 |
| Scheme 12 Synthesis of 1-substituted-1H-tetrazoles using (Fe3O4@NFC@NSalophCu)CO2H. | |
2.1.6. Synthesis and application of Fe3O4@NFC@NNSM-Mn(III). Fe3O4 nanoparticles were functionalized with NFC nanospheres to prepare Fe3O4@NFC 39, which was dispersed in toluene, and then 3-chloropropyl-trimethoxy silane 55 was added to the reaction mixture under N2 gas and reflux conditions to obtain Fe3O4@NFC–Cl 56, followed by reaction with o-phenylenediamine 54 in CH2Cl2 under reflux conditions to give Fe3O4@NFC@NN 57. Then, 5-(chloromethyl)-2-hydroxy benzaldehyde 58 was reacted with Fe3O4@NFC@NN 57 to form Fe3O4@NFC@NNS 59, which was complexed with Mn(OAc)3·2H2O 60 in EtOH to prepare Fe3O4@NFC@NNS-Mn 61, followed by reaction with melamine 62 in the presence of triethylamine in MeOH to generate Fe3O4@NFC@NNSM-Mn(III) 63 (Scheme 13).56
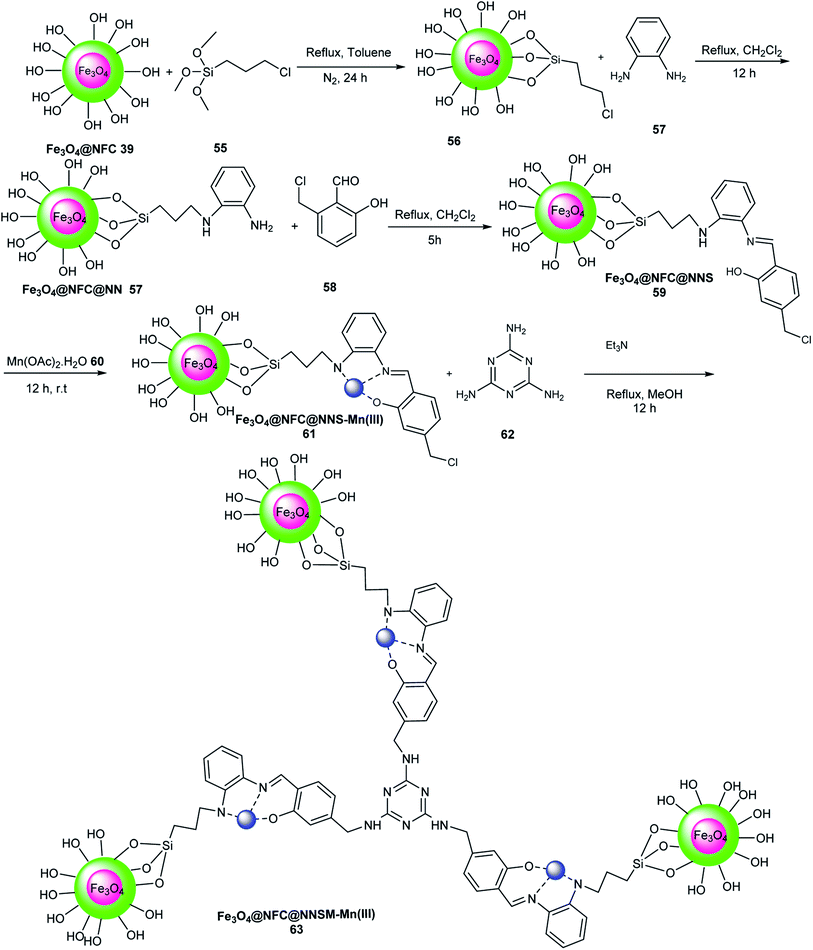 |
| Scheme 13 Synthesis of Fe3O4@NFC@NNSM-Mn(III). | |
The activity of Fe3O4@NFC@NNSM-Mn(III) 63 was tested for the synthesis of xanthenes 65 via the pseudo-three-component reaction of aldehydes 19 and dimedone 64 in EtOH at 45 °C (Scheme 14). The manganese metal on the surface of Fe3O4@NFC@NNSM-Mn(III) acts as a Lewis acid to activate the carbonyl groups. This catalyst was used five times without a decrease in its activity.56
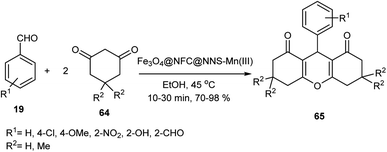 |
| Scheme 14 Synthesis of xanthene derivatives 65 in the presence of Fe3O4@NFC@NNSM-Mn(III). | |
2.1.7. Synthesis and application of Fe3O4@NFC@ONSM-Ni(II). Initially, Fe3O4@NFC 39 was functionalized with 3-chloropropyl-trimethoxy silane 55 to obtain Fe3O4@NFC@CPTMS 56. Subsequently, 2-hydroxy 4-chloromethyl benzaldehyde 44 was prepared via the reaction of salicylaldehyde 42, paraformaldehyde 43, and HCl 37% in the presence of H2SO4 as a catalyst at 70 °C for 20 h. In the next step, the Schiff base ligand was synthesized via the reaction of 2-aminophenol 66 and 5-chloromethylsalicyaldehyde 67 in dichloromethane at 40 °C for 3 h. Then, Fe3O4@NFC@ONS 68 was obtained through the reaction of 4-(chloromethyl)-2-(((2-hydroxyphenyl)imino) methyl) phenol 67 and Fe3O4@NFC@CPTMS 68 in the presence of triethylamine in acetonitrile under reflux conditions. In the next step, Fe3O4@NFC@ONS 68 was reacted with Ni(OAc)2 in EtOH at room temperature for 12 h to obtain Fe3O4@-NFC@ONS-Ni(II) 69. Finally, Fe3O4@NFC@ONSM-Ni(II) 70 was synthesized as a nanocatalyst via the reaction of Fe3O4@NFC@ONS-Ni(II) 69 and melamine 62 in the presence of triethylamine in the MeOH under reflux conditions and N2 gas for 12 h (Scheme 15).57
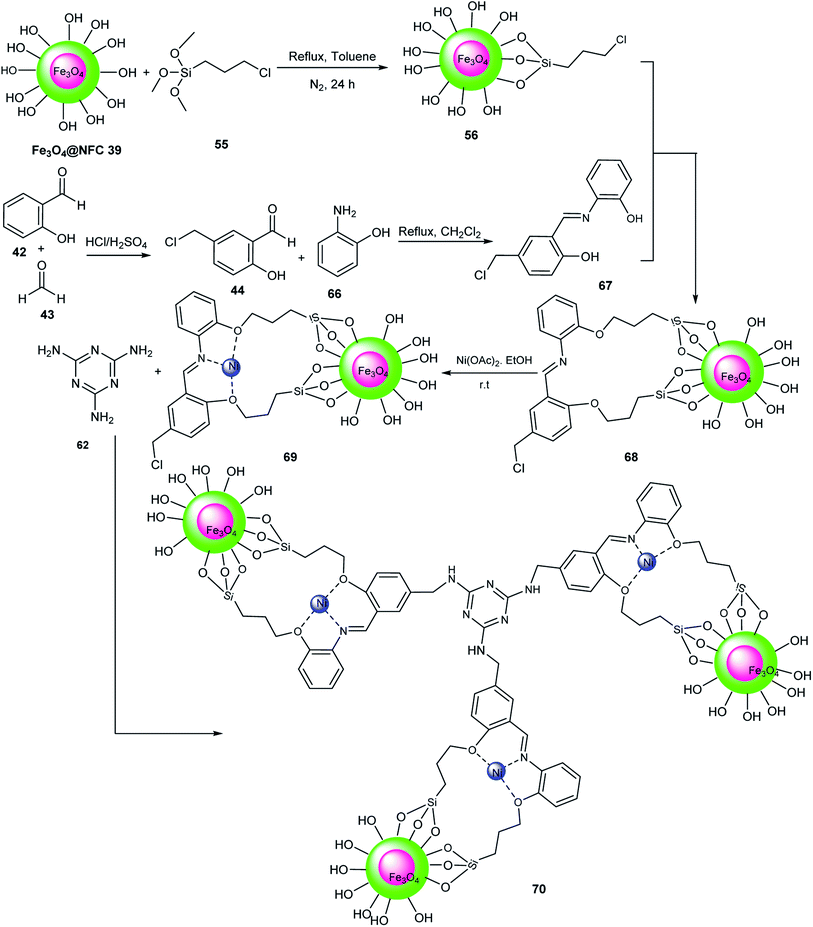 |
| Scheme 15 Synthesis of Fe3O4@NFC@ONSM-Ni(II). | |
This catalyst was used in the synthesis of polyhydroquinolines 72 through the Hantzsch reaction among benzaldehydes 19, dimedone 64, ethyl acetoacetate 21, and ammonium acetate 71 (Scheme 16).57
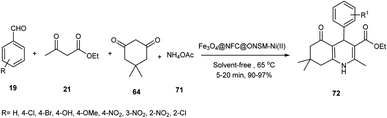 |
| Scheme 16 Synthesis of polyhydroquinolines 72 in the presence of Fe3O4@NFC@ONSM(II). | |
Also, Bagherzade et al. applied this catalyst in the synthesis of 1,4-dihydropyridine 72 via the multicomponent reaction of aldehydes 19, ethyl acetoacetate 21, and ammonium acetate 71 (Scheme 17). The carbonyl groups in the multi-component reaction were activated in the presence of nickel metal on the surface of Fe3O4@NFC@ONSM-Ni(II) as a Lewis acid. Also, this catalyst was tested 6 times without loss in its activity.57
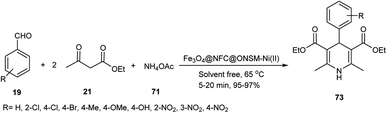 |
| Scheme 17 Synthesis of 1,4-dihydropyridine 73 in the presence of Fe3O4@NFC@ONSM-Ni(II). | |
2.1.8. Synthesis and application of cell-LA-TEA+/Fe3O4. Initially, cellulose 5 was reacted with tosyl chloride 74 in the presence of Et3N to form cell-tosyl 75, which was reacted with lactic acid 76 to produce cell-LA 77, followed by reaction with triethanolamine 78 to prepare cell-LA-TEA+ 79. Finally, cell-LA-TEA+ 79 was magnetized via the reaction of FeCl3·6H2O, FeCl2·4H2O, and ammonium solution in aqueous media to form cell-LA-TEA+/Fe3O4 80 (Scheme 18).58
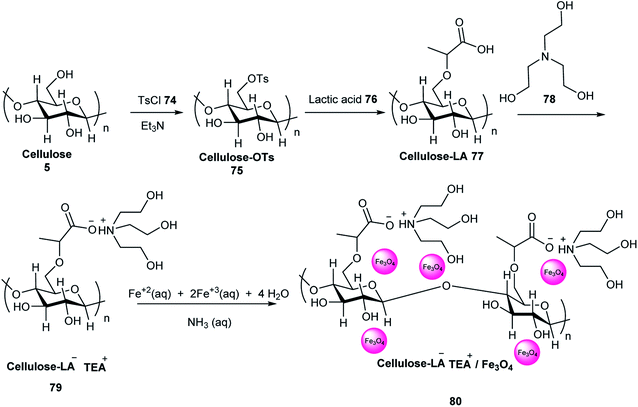 |
| Scheme 18 Synthesis of Cell-LA-TEA+/Fe3O4. | |
Cell-LA-TEA+/Fe3O4 was used as a catalyst for the regioselective synthesis of pyrazolo quinolones 82 via the three-component reaction of dimedone 64, 5-amino pyrazolone 81, and aromatic aldehydes 19 in EtOH/H2O under ultrasonic irradiation (Scheme 19). The catalyst was used with high stability in 7 cycles without loss in its activity.58
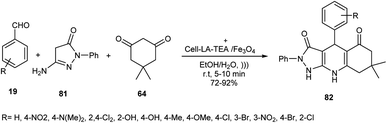 |
| Scheme 19 Synthesis of pyrazolo quinolones 82 in the presence of Cell-LA-TEA+/Fe3O4. | |
2.1.9. Synthesis and application of Fe3O4@nano-cellulose-OPO3H. Initially, nano-cellulose 5 was prepared from cotton. Then, Fe3O4@nano-cellulose (Fe3O4@NCs) 8 was obtained through the reaction of nano-cellulose solution in acetic acid with FeCl3·6HO2, FeCl2·4H2O, and ammonium hydroxide at 80 °C. Finally, Fe3O4@NCs were immobilized with P4O10 at room temperature under grinding conditions to produce Fe3O4@NCS-PA 83 (Scheme 20).59
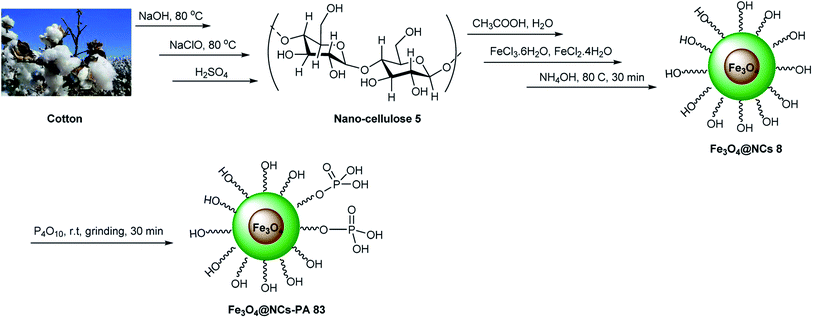 |
| Scheme 20 Synthesis of Fe3O4@NCS-PA. | |
2,3-Dihydroquinazolin-4(1H)-ones 85 were prepared via the condensation reaction of 2-aminobenzamide 84 and aldehydes 19 in the presence of Fe3O4@NCS-PA 83 as a Brønsted acid in H2O: EtOH under reflux conditions (Scheme 21). The main advantages of this method are its excellent yields, simple workup, and eco-friendly catalyst. This reaction was accomplished in H2O: EtOH under reflux conditions in the presence of Fe3O4 with 60% yield; however, Fe3O4@NCS-PA performed better than Fe3O4 in this reaction.59
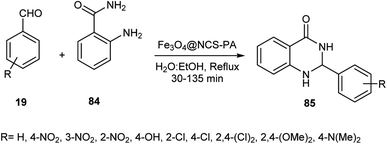 |
| Scheme 21 Synthesis of 2,3-dihydroquinazolin-4(1H)-ones. | |
2.1.10. Synthesis and application of Fe3O4@NCs/Cu(II). Fe3O4 was functionalized with nano-cellulose 5 to obtain Fe3O4@NCs 8, which was added to NaOH solution to give Fe3O4@NCs 8, followed by reaction with CuCl2 to provide Fe3O4@NCs/Cu(II) 86 (Scheme 22).60
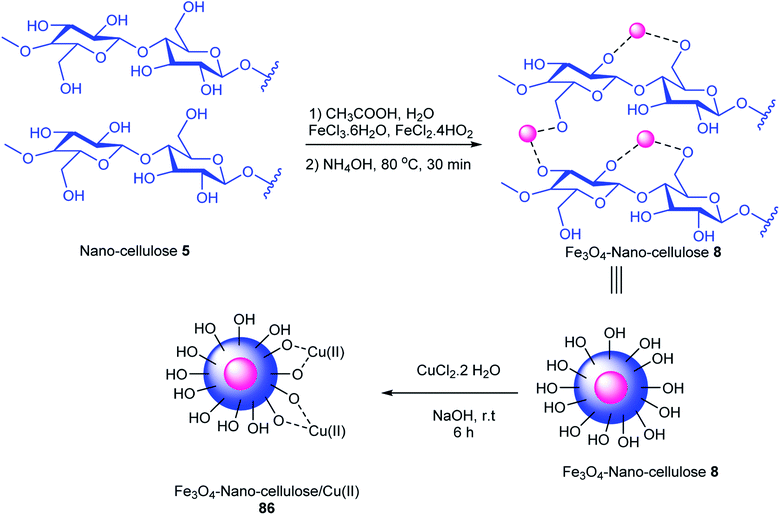 |
| Scheme 22 Synthesis of Fe3O4@NCs/Cu(II) 86. | |
Fe3O4@NCs/Cu(II) as a Lewis acid activated the carbonyl groups of the starting materials by copper metal in the synthesis of indenopyrido[2,3-d]pyrimidines 89 through the three-component reaction of 6-amino-2-(methylthio)pyrimidin-4(3H)-one 88, 1,3-indanedione 87 and aldehydes 19 in EtOH (Scheme 23). The activity of the catalyst was preserved after four runs.60
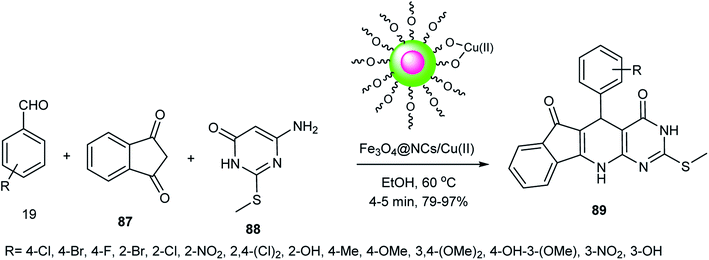 |
| Scheme 23 Synthesis of indenopyrido [2,3-d] pyrimidines in the presence of Fe3O4@NCs/Cu(II). | |
2.1.11. Synthesis and application of Fe3O4@nano-cellulose/Cu(II). The reaction of nano-cellulose 5, FeCl3·6H2O, FeCl2·4H2O, and NH4OH at 80 °C gave Fe3O4@nano-cellulose 8, which was reacted with CuCl2 using sodium hydroxide at room temperature to obtain Fe3O4@nano-cellulose/Cu(II) 90 (Scheme 24).61
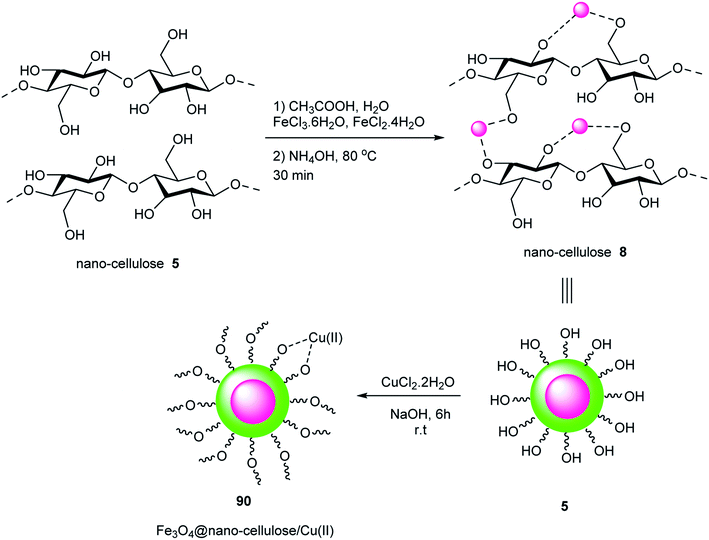 |
| Scheme 24 Synthesis of Fe3O4@nano-cellulose/Cu(II). | |
Cu(II) in Fe3O4@nanocellulose/Cu(II), as a Lewis acid, activated the carbonyl groups in the three-component reaction of aromatic aldehydes 19, 2-aminobenzothiazole 20, and ethyl acetoacetate 21 for the synthesis of 4H-pyrimido[2,1-b]benzothiazoles 22 (Scheme 25). The catalyst activity of Fe3O4@nano-cellulose/Cu(II) was preserved after four runs. The yield of this reaction in the presence of Fe3O4 as a catalyst after 3 h was reported to be about 37%. Therefore, Fe3O4@nano-cellulose/Cu(II) is more active than Fe3O4.61
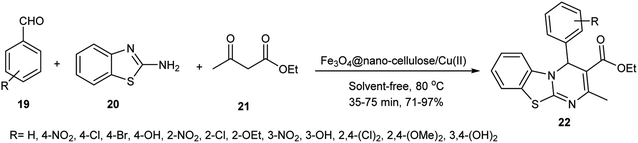 |
| Scheme 25 Synthesis of 4H-pyrimido[2,1-b]benzothiazole derivatives 22 using Fe3O4@nano-cellulose/Cu(II). | |
2.1.12. Synthesis and application of Fe3O4@NFC-ImSalophCu(II). Initially, imidazole 11 was treated with 3-chloropropyl-trimethoxy silane 55 in toluene to produce imidazole-propyl-trimethoxy silane 91, which was immobilized on Fe3O4@NFC 39 using Et3N as a catalyst in dry toluene to give Fe3O4@NFC-Im 92. The reaction of salicylaldehyde 42 and paraformaldehyde 43 in the presence of HCl/H2SO4 gave 5-(chloromethyl)-2-hydroxybenz aldehyde 44, which was reacted with 1,2-phenylenediamine 93 in dichloromethane under reflux conditions to produce N,N-bis(5-chloromethylsalicylidene)-1,2-phenylenediamine 94, followed by reaction with Cu(OAc)2 in EtOH at room temperature to prepare 5-Cl-Salophen-Cu(II) 95. Finally, Fe3O4@NFC-ImSalophCu(II) 96 was synthesized via the reaction of Fe3O4@NFC-Im 92 and 5-Cl-Salophen-Cu(II) 95 in toluene under reflux conditions (Scheme 26).62
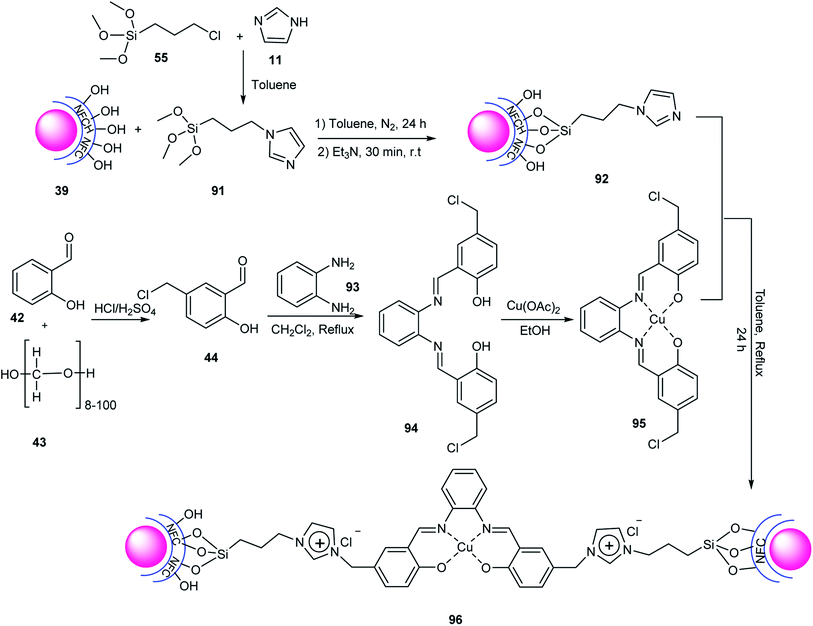 |
| Scheme 26 Synthesis of 4H-pyrimido[2,1-b]benzothiazole derivatives using Fe3O4@NFC-ImSalophCu(II) 96. | |
The copper of Fe3O4@NFC-ImSalophCu 96 was applied in the click reaction of phenacyl bromides 97, sodium azide 90, and alkynes 98 in H2O to synthesize 1,2,3-triazoles 99 (Scheme 27). The reusability of this catalyst was tested in the click reaction four times without loss in its activity.62
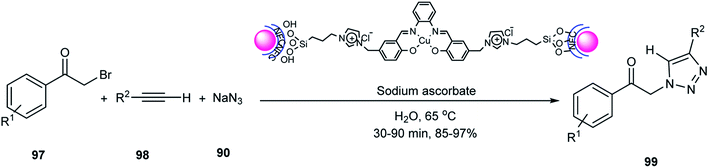 |
| Scheme 27 Synthesis of 1,2,3-triazoles 99 using Fe3O4@NFC-ImSalophCu. | |
2.1.13. Synthesis and application of Fe3O4@walnut shell/Cu(II). Nanomagnetic Fe3O4@walnut shell/Cu(II) was prepared by Mirjalili's research group. Nanomagnetic Fe3O4@walnut shell/Cu(II) 100 was prepared via the reaction of dried powdered walnut shell with NaOH, hypochlorite solution, and sulfuric acid aqueous to afford a nano walnut shell, followed by reaction with CH3COOH, FeCl3·6H2O 1, FeCl2·4H2O2, and NH4OH to generate nanomagnetic Fe3O4@walnut shell 8, which was modified by CuCl2·2H2O/NaOH to produce nanomagnetic Fe3O4@walnut shell/Cu(II) 100 (Scheme 28).63
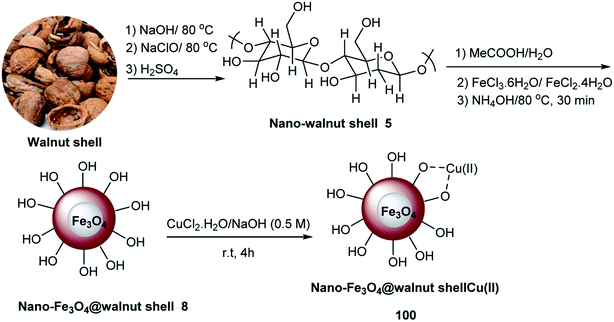 |
| Scheme 28 Synthesis of nano-Fe3O4@walnut shell/Cu. | |
Its catalytic activity was explored using 2-aryl/alkyl-2,3-dihydro-1H-naphtho[1,2-e][1,3]oxazines 102 or 104 or 106 via the pseudo-three-component reaction of β-naphthol 101 or α-naphthol 103 or phenol derivatives 105, formaldehyde 52, and various amines 43 (Scheme 29). The catalytic activity of nano-Fe3O4@walnut shell/Cu did not decrease after five-times use. The copper metal on the nano-Fe3O4@walnut shell/Cu(II) increased the reaction rate via interaction with the carbonyl group of the starting materials.63
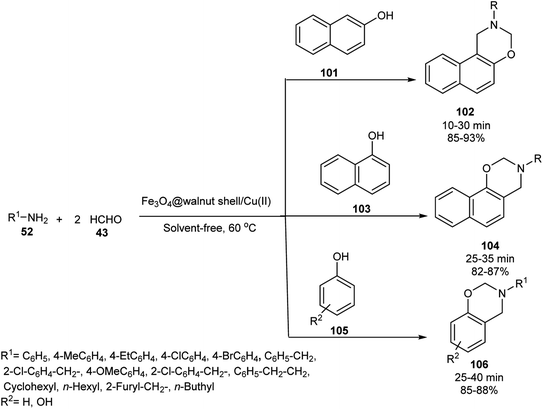 |
| Scheme 29 Synthesis of 2-aryl/alkyl-2,3-dihydro-1H-naphtho[1,2-e][1,3]oxazines 102 or 104 or 106 using nano-Fe3O4@walnut shell/Cu(II). | |
2.2. Magnetic bio-polymers based on dextrin
2.2.1. Synthesis and application of magnetic dextrin nanocomposite. Dextrin 107 is a water-soluble polysaccharide obtained via the hydrolysis of starch and glycogen. Magnetic dextrin nanocomposite 108 was prepared via the addition of dextrin 107 to a solution of FeCl3·6H2O, FeCl2·4H2O, and ammonium hydroxide at 90 °C under an N2 atmosphere via the co-precipitation method (Scheme 30).64
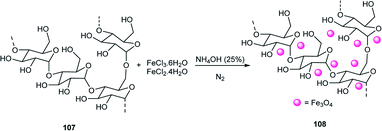 |
| Scheme 30 Synthesis of magnetic dextrin. | |
The magnetic dextrin was tested as a catalyst in the synthesis of polyhydroquinolines via four-component reactions of aromatic aldehydes 19, ethyl acetoacetate 21, dimedone 61, ammonium acetate 71 in EtOH under reflux conditions (Scheme 31). The yield of the products did not decrease after five runs. This reaction was performed in the presence of dextrin in ethanol in 28% yield, and thus magnetic dextrin is better than dextrin to catalyze this reaction.64
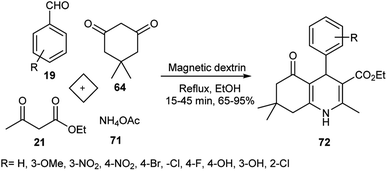 |
| Scheme 31 Synthesis of polyhydroquinolines 72 in the presence of magnetic dextrin. | |
2.2.2. Synthesis and application of magnetized dextrin. Magnetized dextrin 72 was obtained via the reaction of FeCl3·6H2O, FeCl2·4H2O, dextrin 71, and NH4OH in H2O at 90 °C under a nitrogen atmosphere (Scheme 32).65
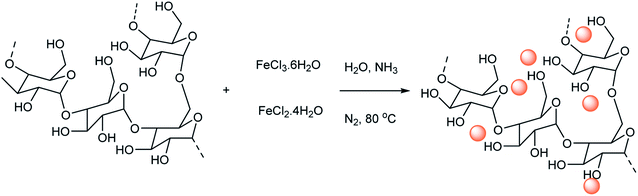 |
| Scheme 32 Synthesis of magnetized dextrin. | |
Maleki et al. studied the catalytic activity of magnetized dextrin 29 for the synthesis of dihydropyrano[2,3-c]pyrazoles 29 through the reaction of hydrazine hydrate 28, ethyl acetoacetate 21, aromatic aldehydes 19, and malononitrile 14 under reflux conditions in EtOH (Scheme 33). This catalyst was used for five runs without a decrease in its catalytic activity.65
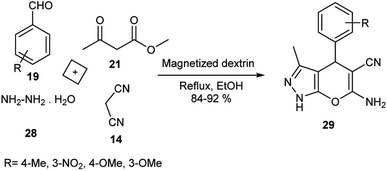 |
| Scheme 33 Synthesis of dihydropyrano[2,3-c]pyrazoles 29 using magnetized dextrin. | |
2.2.3. Synthesis and application of FND-Ti(IV). Fe3O4@nano-dextrin 108 was prepared through the reaction of nano-dextrin 107, FeCl3·6H2O, FeCl2·4H2O, and ammonia solution in H2O at 80 °C. Fe3O4@nano-dextrin 108 was coated with TiCl4 in CH2Cl2 to prepare FND-Ti(IV) 109 (Scheme 34).66
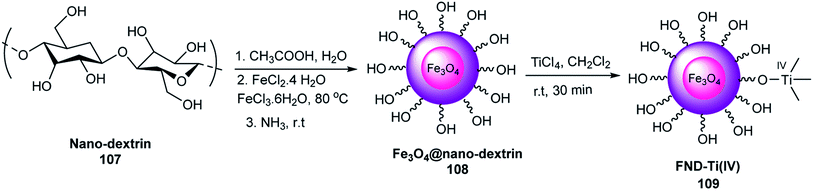 |
| Scheme 34 Synthesis of FND-Ti(IV) 109. | |
Its catalytic activity as a Lewis acid was investigated in the synthesis of 2,3-dihydroquinazolin-4(1H)-ones 85 via the condensation reaction of 2-aminobenzamide 84 and aldehydes 19 under mild conditions (Scheme 35). This catalyst was used five times without loss in its catalytic activity.66
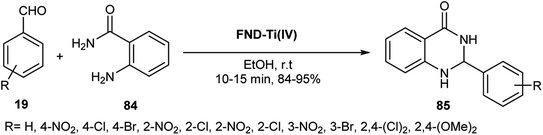 |
| Scheme 35 Synthesis of 2,3-dihydroquinazolin-4(1H)-ones 85 using Fe3O4@nano-dextrin/Ti(IV). | |
2.3. Magnetic bio-polymers based on starch
2.3.1. Synthesis and application of magnetic Ag/Fe3O4@starch nanocatalyst. To aqueous starch solution 110, citric acid 111 and sodium hypophosphite 112 were added and refluxed to give the precipitate crude product, which was crushed and dried to give cross-linked starch 113, followed by dissolving in H2O to produce gelatinized starch 114. The obtained gelatinized starch was reacted with FeCl3·6H2O, and FeSO4·7H2O in H2O at room temperature, and then mixed with NH3 dropwise to give the Fe3O4@starch nanocatalyst, which was treated with AgNO3 (aq) to generate the magnetic Ag/Fe3O4@starch 115 nanocatalyst (Scheme 36).67
 |
| Scheme 36 Synthesis of Ag/Fe3O4@starch 115. | |
The catalytic activity of Ag/Fe3O4@starch as Lewis acid 115 was evaluated in the one-pot reaction of benzaldehydes 19, malononitrile 14, and dimedone 64 in EtOH for the synthesis of 4H-pyran 116 (Scheme 37). Also, the magnetic nanocatalyst was reused five times with no loss in its activities.67
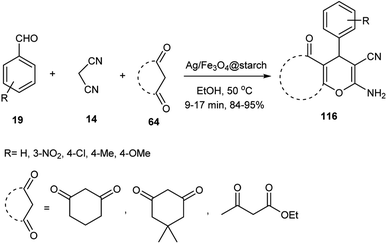 |
| Scheme 37 Synthesis of 4H-pyran 116 in the presence of Ag/Fe3O4@starch. | |
2.3.2. Synthesis and application of magnetic CuFe2O4@starch. To obtain CuFe2O4@starch 122, Cu(NO3)2 117 was reacted with Fe(NO3)3 118 using sodium hydroxide in H2O to give CuFe2O4 nanoparticles 121, followed by reaction with starch to obtain CuFe2O4@starch 122. Its catalytic activity was tested in the synthesis of 4H-chromene 125 or 126 via the three-component reaction of various aldehydes 19, malononitrile 14, 2-hydroxy-1,4-naphthoquinone 123, or 4-hydroxycoumarin 124 (Scheme 38).68
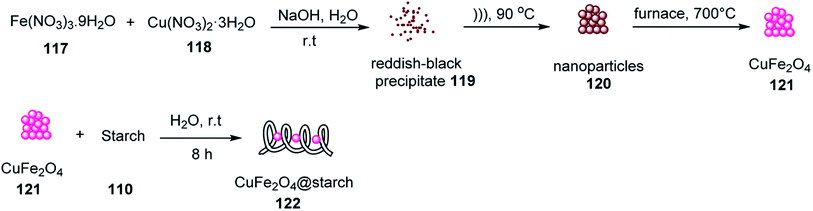 |
| Scheme 38 Synthesis of CuFe2O4@starch. | |
In another attempt, 2-amino-5-oxo-5,6,7,8-tetrahydro-4H-benzo[b]pyrans 27 or 116 were synthesized via the reaction of aldehydes 19, malononitrile 14, and enolizable C–H-activated acidic compounds, including dimedone 64 and ethyl acetoacetate 21, in the presence of CuFe2O4@starch 122 as a Lewis acid in EtOH. The reaction rate for aldehydes with electron-withdrawing groups was faster than that with electron-donating groups (Scheme 39 and 40), respectively. This catalyst was used in six runs without a decrease in its activity.68
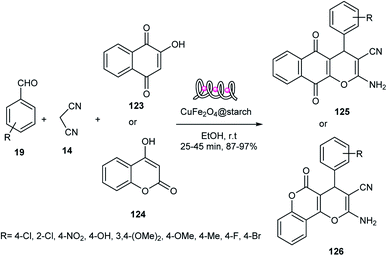 |
| Scheme 39 Synthesis of 4H-chromenes using CuFe2O4@starch. | |
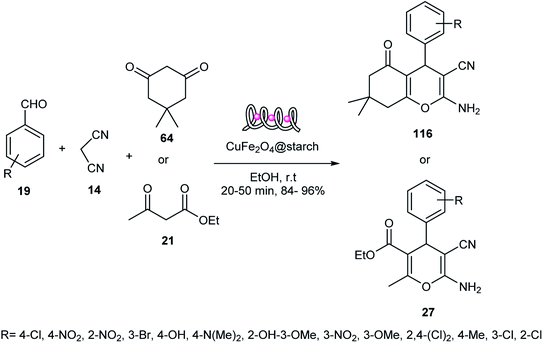 |
| Scheme 40 Synthesis of 2-amino-5-oxo-5,6,7,8-tetrahydro-4H-benzo[b]pyrans 116 or 27. | |
2.3.3. Synthesis and application of magnetic starch/SPION@SO3H. Superparamagnetic iron oxide nanoparticles (SPION) 127 were synthesized via the reaction of Fe2+, Fe3+, and ammonia in aqueous media. Then, SPION 127 was coated with tetraethyl orthosilicate (TEOS) to give SPION@SiO2 128, which was reacted with starch 110 to give magnetic starch, followed by reaction with allyltrimethoxysilane 130 to prepare allyl-functionalized magnetic starch 131. Finally, starch/SPION@SO3H 133 was synthesized via the polymerization of allyl-functionalized magnetic starch 131 and 4-styrenesulfonic acid 132 (Scheme 41).69
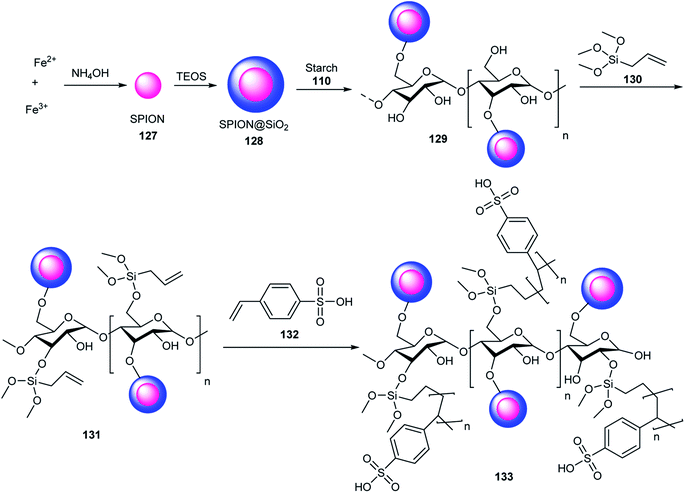 |
| Scheme 41 Synthesis of starch/SPION@SO3H. | |
Starch/SPION@SO3H 133 as a Brønsted acid (SO3H) activated the carbonyl groups in the multicomponent reaction of 4-hydroxycoumarin 124, benzaldehydes 19, dimedone 64, and ammonium acetate 71 in the synthesis of chromeno[4,3-b]quinoline-6,8(9H)-dione derivatives 134 (Scheme 42). This catalyst was applied ten times without any loss in its activity.69
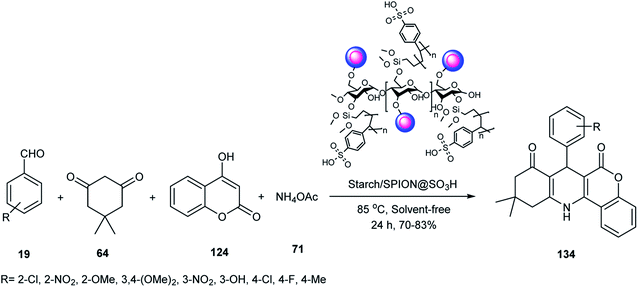 |
| Scheme 42 Synthesis of chromeno[4,3-b]quinoline-6,8(9H)-diones in the presence of Starch/SPION@SO3H. | |
2.3.4. Synthesis and application of magnetic Fe3O4@GOTfOH/Ag/St-PEG-AcA. Initially, through the Hummers' method, graphite 135 was treated with H2SO4, KMnO4, and H2O to give GO 136, which was treated with FeCl3, FeCl2, and ammonium solution at 80 °C to achieve Fe3O4@GO 137, followed by reaction with trifluoro methanesulfonic acid in CH2CH2 to give Fe3O4@GOTfOH 138. In the next step, starch 139 was dissolved in water at 80 °C. Subsequently, N,N-methylene acrylene acrylamide (MBA) 140 was dissolved in water. Then, the above-mentioned two mixtures were mixed to obtain a homogeneous viscous mixture. Then, a certain amount of acrylic acid 141 and PEG-poly 142 was added to the reaction mixture. Then, ammonium persulfate (APS) 143 solution was added to the reaction mixture until a hydrogel was obtained. The above-mentioned homogeneous solution, AgNP 144 colloidal solution and Fe3O4@GOTfOH 138 were mixed to obtain Fe3O4@GOTfOH/Ag/St-PEG-AcA 146 (Scheme 43).70
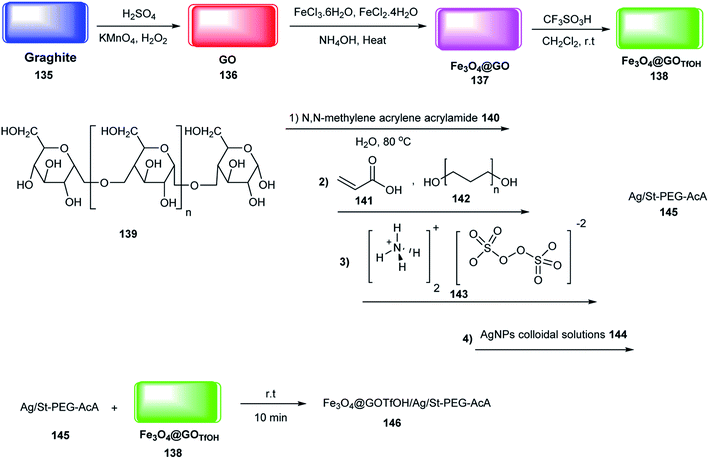 |
| Scheme 43 Synthesis of Fe3O4@GOTfOH/Ag/St-PEG-AcA 146. | |
The catalytic activity of Fe3O4@GOTfOH/Ag/St-PEG-AcA 146 was examined in the synthesis of 2,4,6-triarylpyridines 137 through the pseudo-three-component reaction of aryl aldehydes 19, acetophenone 147 and ammonium acetate 71 (Scheme 44). According to the catalyst structure, the carbonyl groups were activated via interaction with the Brønsted acid site and Lewis acid of Fe3+. Also, the catalytic activity of 146 did not decrease after 10 runs.70
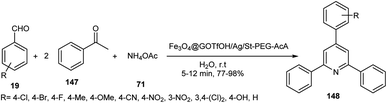 |
| Scheme 44 Synthesis of 2,4,6-triarylpyridine derivatives 148 using Fe3O4@GOTfOH/Ag/St-PEG-AcA. | |
2.3.5. Synthesis and application of magnetic γ-Fe2O3@starch-n-butyl SO3H. Fe3O4 nanoparticles 4 were synthesized via the reaction of FeCl2·4H2O 2 and FeCl3·6H2O 1 and NH4OH solution in H2O an under argon atmosphere at room temperature. Then, γ-Fe2O3 was synthesized using Fe3O4 at 250 °C. In the next step, γ-Fe2O3 was coated with starch at room temperature to obtain γ-Fe2O3@starch 150, followed by reaction with 1,4-butane sultone 151 in dry toluene under reflux conditions to produce γ-Fe2O3@starch-n-butylSO3H 152 nanoparticles (Scheme 45).71
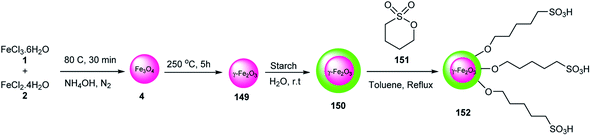 |
| Scheme 45 Synthesis of γ-Fe2O3@starch-n-butyl SO3H. | |
γ-Fe2O3@starch-n-butylSO3H 152 as Brønsted acid activated the carbonyl groups in the multicomponent reactions of aldehydes 19, dimedone 64, and 2-aminobenzimidazole 153 or phthalhydrazide 154 to synthesize tetrahydrobenzimidazo[2,1-b]quinazolin-1(2H)-ones 155 or 2H-indazolo[2,1-b]phthalazine-trione 156 (Scheme 46). This catalyst was used seven times without loss in any of its activities.71
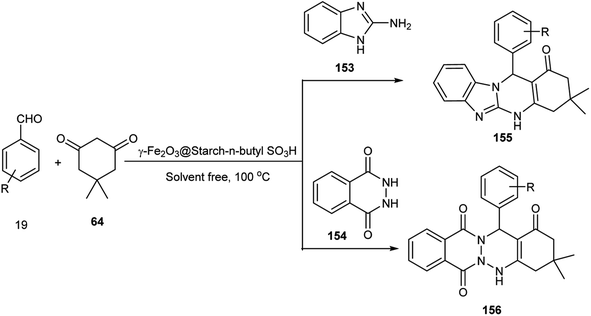 |
| Scheme 46 Synthesis of tetrahydrobenzimidazo[2,1-b]quinazolin-1(2H)-ones 155 or 2H-indazolo[2,1-b]phthalazine-triones 156 using γ-Fe2O3@starch-n-butyl SO3H. | |
2.4. Magnetic bio-polymers based on alginate
2.4.1. Synthesis and application of Fe3O4@Alg@CPTMS@Arg. Alginate 157 was immobilized on Fe3O4 4 via the co-precipitation method. Initially, FeCl3·6H2O 1, FeCl2·4H2O 2, and sodium alginate 157 were dissolved in H2O under a nitrogen atmosphere. Then, an ammonia solution was added to this mixture to give Fe3O4@Alg nanoparticles 158, which were functionalized with 3-chloropropyltrimethoxysilane 55 to prepare Fe3O4@Alg@CPTMS 159, followed by reaction with L-arginin 160 using trimethylamine in EtOH for 48 h to give Fe3O4@Alg@CPTMS@Arg 161 (Scheme 47).72
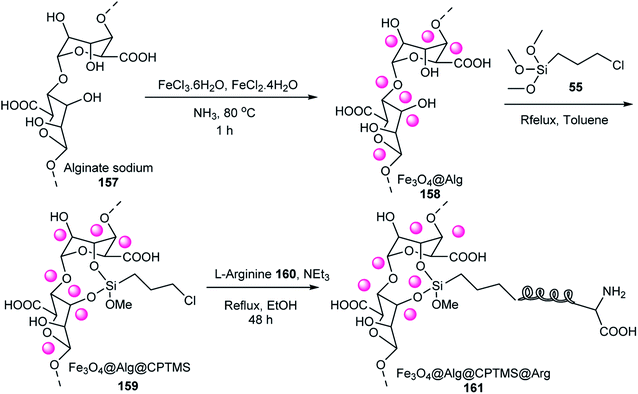 |
| Scheme 47 Synthesis of Fe3O4@Alg@CPTMS@Arg 161. | |
Subsequently, Fe3O4@Alg@CPTMS@Arg, which has two acidic and basic functional sites, activated the carbonyl groups in the synthesis of 2,4,5-triarylimidazoles through the reaction of ammonium acetate, aldehydes, and benzil in EtOH under reflux conditions (Scheme 48). The recyclability of the catalyst was tested 7 times using the model reaction without loss in any of its activities. When Fe3O4 was used as the catalyst, the yield of this reaction was about 65%.72
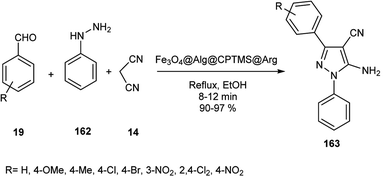 |
| Scheme 48 Synthesis of 2,4,5-triarylimidazoles 163 using Fe3O4@Alg@CPTMS@Arg. | |
2.4.2. Synthesis and application of Fe3O4@Alg@CPTMS@Arg. The reaction of Fe3O4@Alg 165 and 3-chloropropyltrimethoxysilane (CPTMS) 55 in toluene under reflux conditions and nitrogen atmosphere gave Fe3O4@Alg@CPTMS 166, which was reacted with arginine 167 in the presence of trimethylamine in dry toluene to provide Fe3O4@Alg@CPTMS@Arg nanocomposites 168 (Scheme 49).73
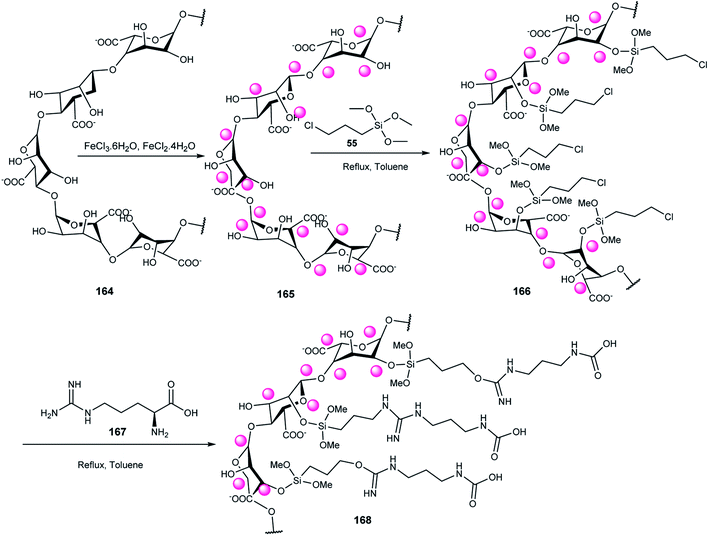 |
| Scheme 49 Synthesis of Fe3O4@Alg@CPTMS@Arg. | |
The activity of Fe3O4@Alg@CPTMS@Arg was tested as a catalyst in the synthesis of 2,4,5-triarylimidazoles 36 via the reaction of ammonium acetate 71, aldehyde derivatives 19, and benzil 34 in EtOH (Scheme 50). Its catalytic activity did not decrease after seven uses. It has two functional groups including a Lewis base (NH2) and Brønsted acidic (COOH), which catalyzed the synthesis of 2,4,5-triarylimidazoles.73
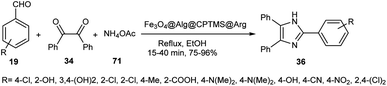 |
| Scheme 50 Synthesis of 2,4,5-triarylimidazoles derivatives in the presence of Fe3O4@Alg@CPTMS. | |
2.4.3. Synthesis and application of Fe3O4@FU. Fe3O4@FU 170 was prepared via the reaction of fucoidan powder 169, FeCl2·4H2O 2, and FeCl3·6H2O 1 in aqueous ammonia solution (25%) in distilled water under a nitrogen atmosphere at 80 °C (Scheme 51).74
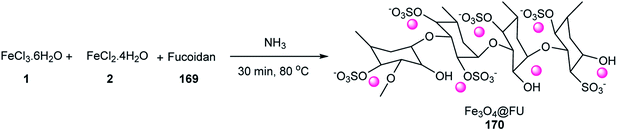 |
| Scheme 51 Synthesis of magnetic Fe3O4@FU 170. | |
The activity of Fe3O4@FU was evaluated as a catalyst in the synthesis of tri- and tetra-substituted imidazoles 36 or 171 via three- and four-component reactions of benzil 34, aldehydes 19, NH4OAc 71, and amine 52 under reflux conditions in EtOH (Scheme 52), respectively. The catalyst was used six times in the model reaction without loss in any of its activities. This reaction was accomplished in ethanol under reflux conditions in the presence of Fe3O4 as a catalyst after 40 min with 55% yield. The carbonyl groups were activated via hydrogen bonding with Fe3O4@FU as a catalyst.74
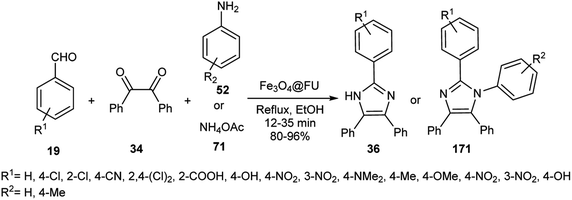 |
| Scheme 52 Synthesis of imidazole derivatives. | |
2.5. Magnetic bio-polymers based on glucose
2.5.1. Synthesis and application of Fe3O4@C@ONa. Initially, FeCl3–6H2O solution, CO(NH2)2, and glucose were added to ethylene glycol 172 to produce a black powder of carbon-coated magnetic nanoparticles (CCMNPs: Fe3O4@C) 173, followed by reaction with NaOH solution to obtain basic carbon-coated magnetic nanoparticles (BCCMNPs: Fe3O4@C@ONa) 174 (Scheme 53).75
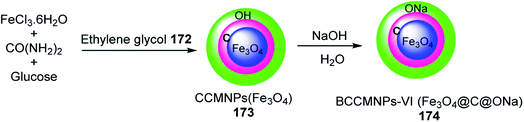 |
| Scheme 53 Synthesis of Fe3O4@C@ONa. | |
Subsequently, the catalytic activity of Fe3O4@C@ONa was tested for the synthesis of 4H-chromene derivatives 174 via the reaction of salicylaldehyde 42, dimedone 64, and β-naphthol 101 in water at 60 °C (Scheme 54). Also, the catalyst was used five times in the model reaction without loss in any of its activities. This catalyst has two functional groups, including Fe3+ as a Lewis acid and oxygen group as a Lewis base, which increased the reaction rate.75
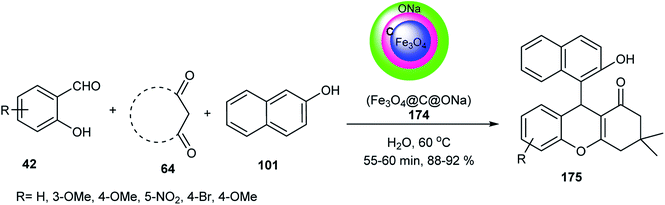 |
| Scheme 54 Synthesis of 4H-chromene derivatives using Fe3O4@C@ONa 174. | |
2.6. Magnetic bio-polymers based on chitosan
2.6.1. Synthesis and application of CSSNH@Arg. Chitosan-silica sulfate nanohybrid (CSSNH@Arg) 178 was synthesized via the reaction of chitosan 176 and silica sulfuric acid (SSA) 177 under ultrasonic irradiation (Scheme 55).76
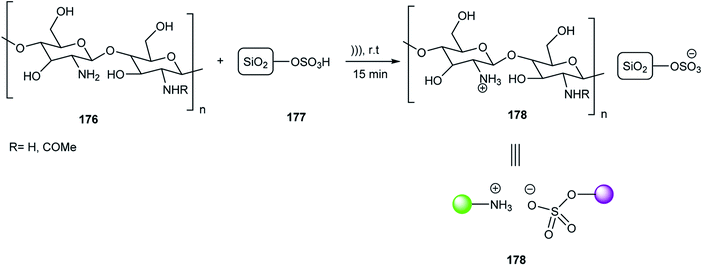 |
| Scheme 55 Synthesis of CSSNH@Arg 178. | |
A green method for the synthesis of 3,4-dihydropyrimidine-2(1H)-one/thione derivatives 179 was described by Behrouz and co-workers via the Biginelli reaction of (thio)urea 23 or urea 6, methyl acetoacetate 21, and aldehydes 19 using CSSNH@Arg 178 under solvent-free conditions (Scheme 56). According to the reusability test, this catalyst was used five times without a decrease in any of its activities. The various functional groups including hydroxyl and amine on the CSSNH@Arg can activate the carbonyl groups via hydrogen bonding.76
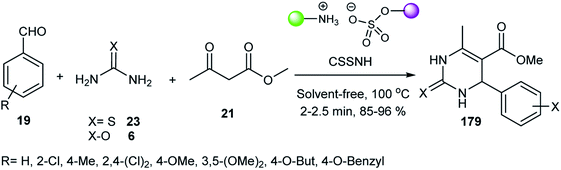 |
| Scheme 56 Synthesis of 3,4-dihydropyrimidine-2(1H)-(thio)ones using of CSSNH@Arg 178. | |
2.6.2. Synthesis and application of Fe3O4@C–SO3H. Initially, starch was mixed with sulfuric acid, and then the reaction mixture was transferred to an autoclave for 24 h at 180 °C, resulting in the formation of a solid black product (C–SO3H). The magnetic Fe3O4 nanoparticles, carbon-based solid acid (C–SO3H) 180, and H2O were placed in an oil bath at 100 °C to remove H2O, and then the reaction mixture was poured into a Teflon-sealed autoclave and heated at 180 °C for 6 h to achieve Fe3O4@C–SO3H 181 (Scheme 57).77
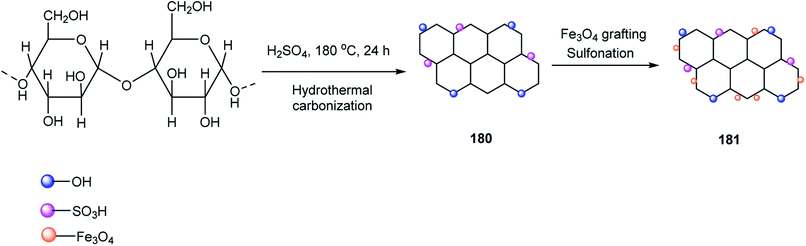 |
| Scheme 57 Synthesis of 3,4-dihydropyrimidine-2(1H)-(thio)ones in the presence of CSSNH 178. | |
CSSNH was applied as a Brønsted acid catalyst in the synthesis of 2-amino-3-cyano-4H-pyrans 126 and 2-amino-4H-chromenes 116 or 182 via the three-component reactions of malononitrile 14, benzaldehyde 19, and β-naphthol 101 or dimedone 64 or 4-hydroxycoumarin 124, respectively (Scheme 58). This catalyst was used four times without any loss in its activity.77
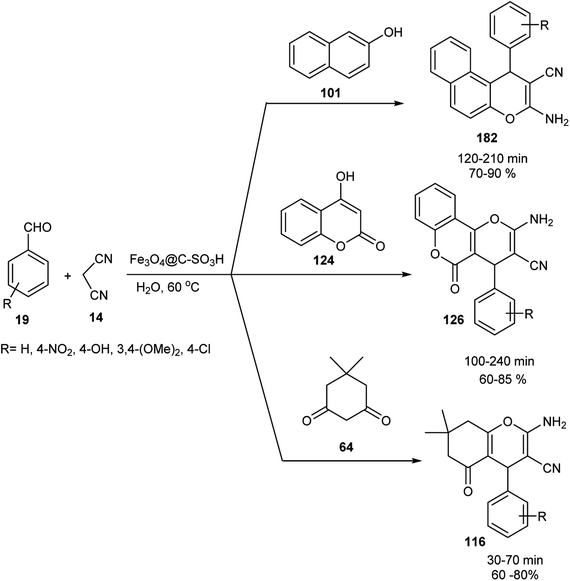 |
| Scheme 58 Synthesis of 2-amino-4H-chromenes 116 and 2-amino-3-cyano-4H-pyrans 126 in the presence of Fe3O4@C–SO3H. | |
2.6.3. Synthesis and application of magnetic chitosan-terephthaloyl-creatine bio-nanocomposite. The reaction of terephthaloyl chloride 183 and creatine powders 184 in CH2Cl2 under reflux conditions provided creatine-terephthaloyl chloride ligand 185, which was added to chitosan 176 powder in a hydrochloric acid solution, and then refluxed to give chitosan-terephthaloyl-creatine bio-nanocomposite 186, followed by immobilization on Fe3O4 to provide magnetic chitosan-terephthaloyl-creatine bio-nanocomposite 187 (Scheme 59).78
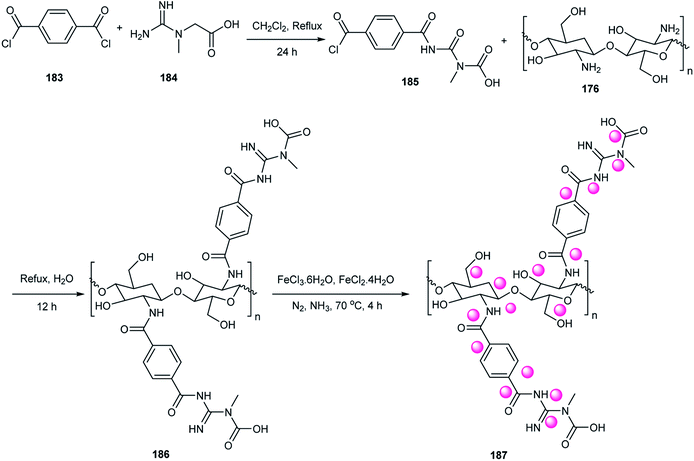 |
| Scheme 59 Synthesis of magnetic chitosan-terephthaloyl-creatine bio-nanocomposite. | |
Its catalytic activity was tested in the synthesis of polyhydroquinolines 72 via the reaction of aldehydes 19, dimedone 67, ammonium acetate 71, and ethyl acetoacetate or methyl acetoacetate 21 in EtOH (Scheme 60). In another attempt, it was used in the synthesis of 1,4-dihydropyridines 73 via the pseudo-three-component reaction of aldehydes 19, methyl acetoacetate 21, and ammonium acetate 71 (Scheme 61). The acidic site of the catalyst activated the carbonyl groups of the primary compounds. The activity of this catalyst did not decrease after use eight times.78
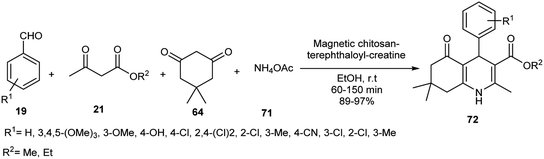 |
| Scheme 60 Synthesis of polyhydroquinoline derivatives 72. | |
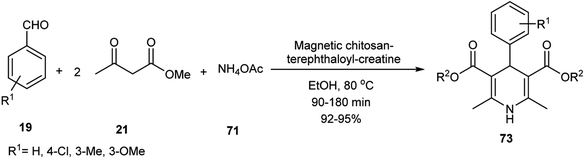 |
| Scheme 61 Synthesis of 1,4-dihydropyridine derivatives 73 in the presence of terephthaloyl-creatine bio-nanocomposite. | |
2.6.4. Synthesis and application of magnetic cyanoguanidine-modified chitosan (MCGC). Firstly, chitosan 176 was obtained via the deacetylation of chitin 74, and then it was modified by treatment with cyanoguanidine 188 in HCl solution to produce cyanoguanidine-modified chitosan (CGC) 189, which was immobilized on Fe3O4 nanoparticles to afford magnetic cyanoguanidine-modified chitosan (MCGC) 190 (Scheme 62). Its catalytic activity was investigated in the synthesis of benzimidazoloquinazolines 155 via the reaction of aldehydes 19, 2-aminobenzimidazole 153, and dimedone 64 in EtOH under reflux conditions. The sonochemical method afforded better yields in shorter reaction times than the conventional method (Scheme 63).79
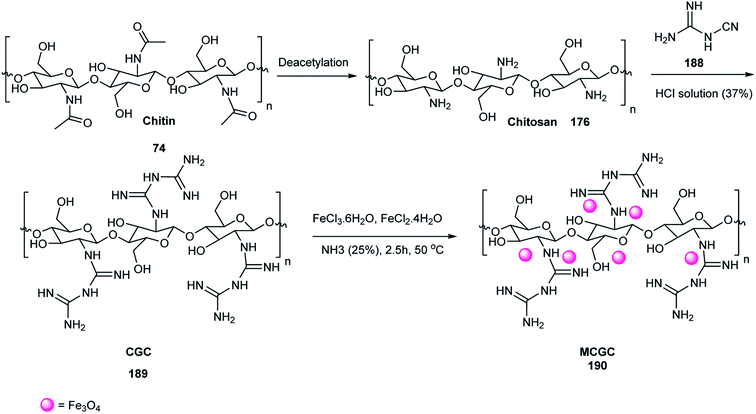 |
| Scheme 62 Synthesis of magnetic cyanoguanidine-modified chitosan (MCGC). | |
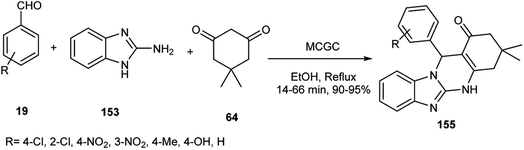 |
| Scheme 63 Synthesis of benzimidazoloquinazoline derivatives. | |
1,4-Dihydropyridines 73 were synthesized via the Hantzsch reaction of ethyl acetoacetate 35, aromatic aldehydes 19, and ammonium acetate 71 under ultrasonic irradiation in the presence of MCGC 190 as a catalyst, which activated the carbonyl groups via hydrogen bonding. Also, the yield of the reaction did not decrease after eight times usage of the catalyst (Scheme 64).79
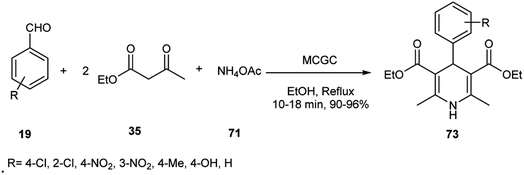 |
| Scheme 64 Synthesis of 1,4-dihydropyridines. | |
2.6.5. Synthesis and application of magnetic MnFe2O4-CS-Bu-SO3H. Manganese ferrite nanoparticles were synthesized via the co-precipitation of Fe(III) 1 and Mn(II) 189 in the presence of NaOH solution at 97 °C to give MnFe2O4 191, which was immobilized on chitosan 176 and 4-butane sultone 151 to prepare MnFe2O4-CS-Bu-SO3H 192 (Scheme 65).80
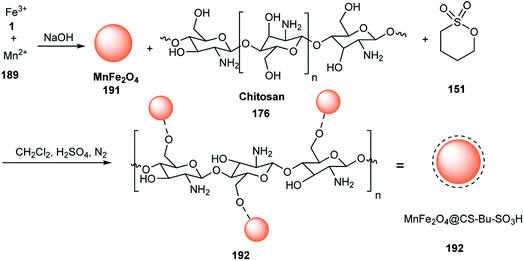 |
| Scheme 65 Synthesis of MnFe2O4-CS-Bu-SO3H 192. | |
The catalyst activity of MnFe2O4-CS-Bu-SO3H was investigated in the synthesis of spiro[acenaphthylene-1,9′-acridine]triones 194 via the multicomponent reaction of dimedone 64, aldehydes 19, and acenaphthoquinone 193 under ultrasonic irradiation in H2O (Scheme 66). Also, MnFe2O4-CS-Bu-SO3H as a Brønsted acid increased the reaction rate via hydrogen bonding with the carbonyl group. According to the reusability test, this catalyst was used five times without any loss in its activity.80
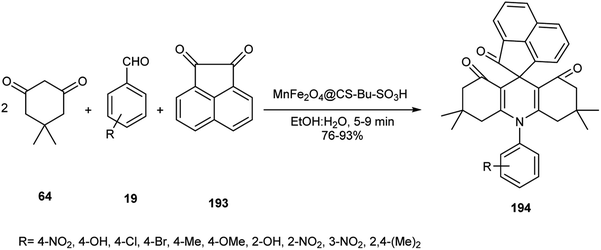 |
| Scheme 66 Synthesis of spiro[acenaphthylene-1,9′-acridine] triones 194 in the presence of MnFe2O4@CS-Bu-SO3H NPs. | |
2.6.6. Synthesis and application of magnetic Cu-MCS. Fe3O4 NPs 4 were reacted with carboxymethylated chitosan to obtain magnetic chitosan MCS 195, which was reacted with CuCl2·2H2O in H2O to generate Cu NPs@Fe3O4-chitosan (Cu-MCS) 196 (Scheme 67).
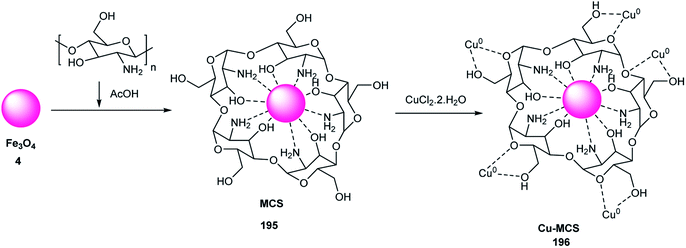 |
| Scheme 67 Synthesis of Cu-MCS. | |
The catalytic activity of Cu-MCS was verified in the synthesis of various tetrazoles 198 or 199 via the reaction of cyanamides 197 and NaN3 50 in H2O under reflux conditions (Scheme 68). This catalyst could be used five times without loss in its activity.81
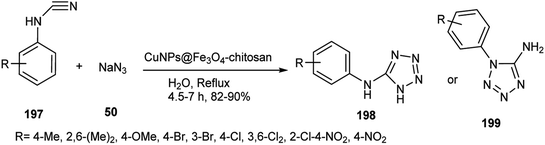 |
| Scheme 68 Synthesis of various tetrazoles 198 or 199 using Cu-MCS. | |
2.6.7. Synthesis and application of magnetic Ch-Fe3O4 NCs. Chitosan was dissolved in an acetic acid solution, and then FeCl3·6H2O 1 and FeCl2·4H2O 2 were added to the reaction mixture for 6 h at 80 °C under an N2 atmosphere. Then, NH4OH was added to the reaction mixture to obtain Ch-Fe3O4 NCs 200. Finally, the chitosan magnetic nanocomposite of Ch-rhomboclase NCs 201 was synthesized via the reaction of Ch-Fe3O4 NCs 200 with chlorosulfonic acid at room temperature under N2 gas (Scheme 69).82
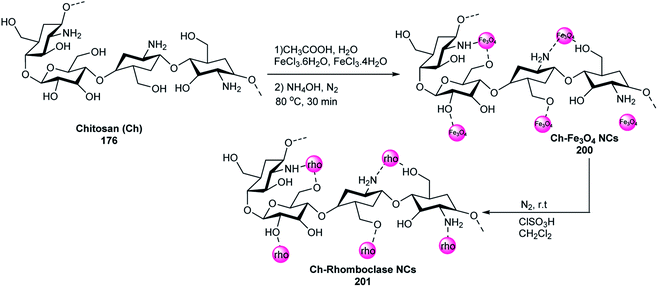 |
| Scheme 69 Synthesis of Ch-rhomboclase NCs 201. | |
The condensation reaction of benzaldehyde 19, ethyl acetoacetate 35 or dimedone 64, and ammonium acetate 71 gave 1,4-dihydropyridine derivatives 73 or 202 in the presence of Ch-rhomboclase NCs 201 under solvent-free conditions at 80 °C (Scheme 70). In the Hantzsch reaction, this acidic catalyst activated carbonyl groups via hydrogen bonding. Also, according to the reusability test, the catalyst was used seven times without any decrease in its activity.82
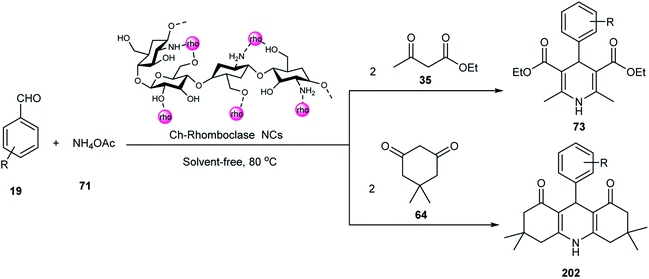 |
| Scheme 70 Synthesis of 1,4-dihydropyridine derivatives 73 or 202. | |
2.6.8. Synthesis and application of magnetic Fe3O4/CS/COF/Cu. After the preparation of an FeCl3·6H2O solution in ethylene glycol at room temperature, chitosan, sodium acetate, and ethylenediamine were added to it. Then, this mixture was placed in a Teflon-lined autoclave and heated at 200 °C for 8 h to obtain Fe3O4/CS 205, which was dispersed in DMSO under ultrasound irradiation to give CS-coated Fe3O4, followed by mixing with melamine 63 and terephthaldehyde 203. The obtained mixture was transferred in a Teflon-lined autoclave at 180 °C for 12 h to obtain Fe3O4/CS/COF 206, which was reacted with Cu(NO3)2·3H2O in EtOH under reflux conditions and argon atmosphere for 24 h to generate Fe3O4/CS/COF/Cu 207 (Scheme 71).83
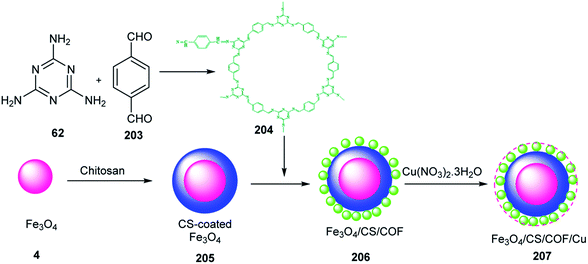 |
| Scheme 71 Synthesis of the Fe3O4/CS/COF/Cu. | |
The catalytic activity of Fe3O4/CS/COF/Cu 207 was tested in the synthesis of polyhydroquinolines 72 via the Hantzsch reaction of aldehydes 19, dimedone 64, ammonium acetate 71, and ethyl acetoacetate 35 (Scheme 72). Fe3O4/CS/COF/Cu with two sites including a Lewis acid (Cu2+) and Lewis base (imine) catalyzed the Hantzsch reaction, which was used five times without a decrease in its activity.83
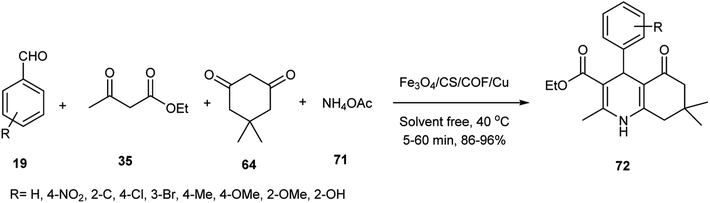 |
| Scheme 72 Synthesis of polyhydroquinoline derivatives via Hantzsch reaction. | |
2.7. Synthesis and application of magnetic ZnS/CuFe2O4/agar
FeCl3·6H2O 1, CuCl2·2H2O 208, Zn(OAc)2.2H2O 209, and thioacetamide were dissolved in distilled H2O. Then, agar 211 and ammonia solution were added to the reaction mixture to obtain ZnS/CuFe2O4/agar 212 (Scheme 73).84
 |
| Scheme 73 Synthesis of ZnS/CuFe2O4/agar 212. | |
The catalytic activity of ZnS/CuFe2O4/agar 212 was examined in the reaction of dimedone 64, malononitrile 14, and aldehydes 19 to synthesize 2-amino-tetrahydro-4H-chromene-3-carbonitriles 116 (Scheme 74). The carbonyl groups were activated in the presence of ZnS/CuFe2O4 via interaction with the hydroxyl groups of agar and Zn metal as a Lewis acid. The catalyst was reused five times with no reduction in its activity.84
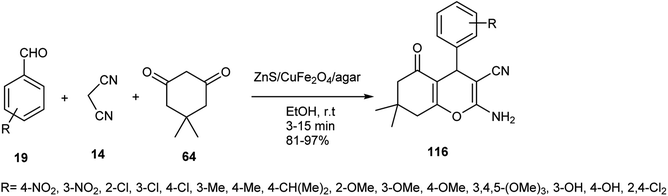 |
| Scheme 74 Synthesis of 2-amino-tetrahydro-4H-chromene-3-carbonitrile derivatives. | |
2.8. Synthesis and application of magnetic Fe3O4@xanthan gum
NH4OH solution was added to a mixture of the FeCl2·4H2O, FeCl3·6H2O (1
:
2), and an aqueous suspension of xanthan gum 213 to give Fe3O4@xanthan gum 214 (Scheme 75).85
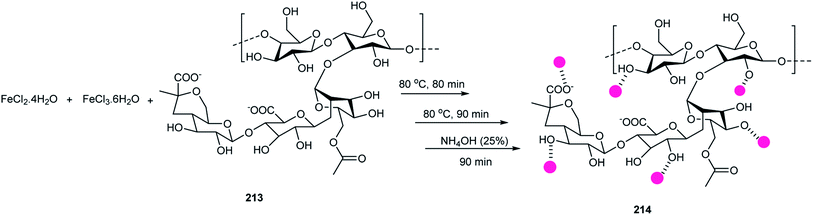 |
| Scheme 75 Synthesis of Fe3O4@xanthan gum 214. | |
Fe3O4@xanthan gum was applied in the synthesis of 2-amino-3-cyano-4H-pyran derivatives 116 via the reaction of aldehydes 19, dimedone 64, and malononitrile 14 in EtOH (Scheme 76). The model reaction was performed about nine times and the yields did not decrease. This reaction was accomplished in the presence of Fe3O4 after 45 min in 30% yield. It was shown that Fe3O4@xanthan gum activated the carbonyl groups via hydrogen bonding, which was more effective than Fe3O4.85
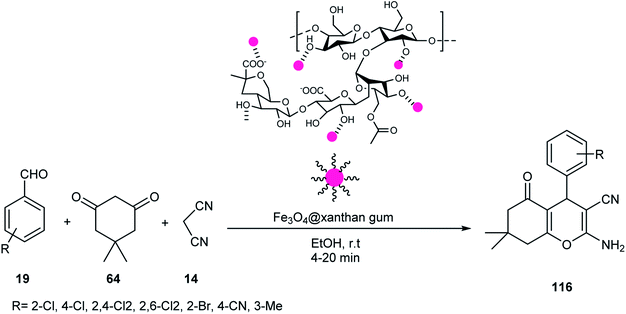 |
| Scheme 76 Synthesis of 2-amino-3-cyano-4H-pyran derivatives 116 using Fe3O4@xanthan gum. | |
3. Conclusion
Many bio-polymers can be obtained from natural sources. According to the importance of green chemistry in organic reactions, in this review, the application of bio-polymers as a catalyst in multicomponent reactions was grouped and summarized. Herein, we highlighted the immobilization of magnetic nanoparticles with bio-polymers. Due to the excellent properties of magnetic nano-catalysts, including their non-toxic nature, high surface area, simple preparation, easy surface modification, and simple separation, these systems have been applied as catalysts in multicomponent reactions. Various organic compounds such as bio-polymers were used for the modification of magnetic nanoparticles. Bio-polymers have various advantages such as biodegradable, biocompatible, and heat-resistant nature. Therefore, herein, their synthesis and catalytic activities in multicomponent reactions were studied. We believe that this article will guide researchers in the design and synthesis of various compounds according to green chemistry. Magnetic nanocomposites have many applications in various fields including, drug delivery, solar cells, chemical sensors, water treatment, biomedical sensors, and catalysts. The importance of these topics could be discussed as a review article in the future.
Conflicts of interest
There are no conflicts to declare.
Acknowledgements
We are grateful for the Research Council's support of Alzahra University.
References
- A. Ali, H. Zafar, M. Zia, U. HAQ, A. Phull, J. Ali and A. Hussain, Nanotechnol., Sci. Appl., 2016, 9, 49–67 CrossRef CAS PubMed.
- F. Erogbogbo, K.-T. Yong, R. Hu, W.-C. Law, H. Ding, C.-W. Chang, P. N. Prasad and M. T. Swihart, ACS Nano, 2010, 4, 5131–5138 CrossRef CAS PubMed.
- M. Esmaeilpour, J. Javidi, F. N. Dodeji and H. Hassannezhad, J. Iran. Chem. Soc., 2014, 11, 1703–1715 CrossRef CAS.
- L. Lai, Q. Xie, L. Chi, W. Gu and D. Wu, J. Colloid Interface Sci., 2016, 465, 76–82 CrossRef CAS PubMed.
- A. Abou-Hassan, O. Sandre, S. Neveu and V. Cabuil, Angew. Chem., 2009, 121, 2378–2381 CrossRef.
- N. O. Mahmoodi, F. Ghanbari Pirbasti and Z. Jalalifard, J. Chin. Chem. Soc., 2018, 65, 383–394 CrossRef CAS.
- M. Esmaeilpour, J. Javidi and M. Zandi, New J. Chem., 2015, 39, 3388–3398 RSC.
- X. Chen, Y. Zhou, H. Han, X. Wang, L. Zhou, Z. Yi and L. Zeng, Mater. Today Chem., 2021, 22, 100556 CrossRef CAS.
- G. U. I. Sheng, S. H. E. N. Xiaodong and L. I. N. Benlan, Rare Met., 2006, 25, 426–430 CrossRef.
- S. S. Khasraghi, M. Shojaei, M. Janmaleki and U. Sundararaj, Eur. Polym. J., 2021, 159, 110735 CrossRef.
- X. Liu, J. Tian, Y. Li, N. Sun, S. Mi, Y. Xie and Z. Chen, J. Hazard. Mater., 2019, 373, 397–407 CrossRef CAS PubMed.
- M. Muthiah, I. K. Park and C. S. Cho, Biotechnol. Adv., 2013, 3, 1224–1236 CrossRef PubMed.
- R. Mohammadi, A. Saboury, S. Javanbakht, R. Foroutan and A. Shaabani, Eur. Polym. J., 2021, 153, 110500 CrossRef CAS.
- M. A. Almessiere, Y. Slimani, A. Demir Korkmaz, A. Baykal, H. Albetran, T. A. Saleh, M. Sertkol and I. Ercan, Ultrason. Sonochem., 2020, 62, 104847 CrossRef CAS PubMed.
- H. Javadian, M. Ruiz, T. A. Saleh and A. M. Sastre, J. Mol. Liq., 2020, 306, 112760 CrossRef CAS.
- A. A. Basaleh, M. H. Al-Malack and T. A. Saleh, J. Environ. Chem. Eng., 2021, 9, 105126 CrossRef CAS.
- A. A. Basaleh, M. H. Al-Malack and T. A. Saleh, Sustainable Chem. Pharm., 2021, 23, 100503 CrossRef.
- N. Dhenadhayalan, K. C. Lin and T. A. Saleh, Small, 2020, 6, 1905767 CrossRef PubMed.
- A. M. Alansi, M. Al-Qunaibit, I. O. Alade, T. F. Qahtan and T. A. Saleh, J. Mol. Liq., 2018, 253, 297–304 CrossRef CAS.
- A. M. Alansi, T. F. Qahtan and T. A. Saleh, Adv. Mater. Interfaces, 2021, 8, 2001463 CrossRef CAS.
- A. M. Alansi, T. F. Qahtan, N. Al Abass, J. M. AlGhamdi, M. Al-Qunaibit and T. A. Saleh, Adv. Sustainable Syst., 2021, 2100267 Search PubMed.
- T. A. Saleh, Environ. Technol. Innovation, 2021, 24, 101821 CrossRef CAS.
- J. Wang, Z. Shi, J. Fan, Y. Ge, J. Yin and G. Hu, J. Mater. Chem., 2012, 22, 22459 RSC.
- T. A. Debele, S. L. Mekuria and H. C. Tsai, Mater. Sci. Eng., C, 2016, 68, 964–981 CrossRef CAS PubMed.
- A. Maleki and M. Kamalzare, Catal. Commun., 2014, 53, 67–71 CrossRef CAS.
- V. Omid, F. M. Kievit, C. Fang, N. Mu, S. Jana, M. C. Leung, H. Mok, R. G. Ellenbogen, J. O. Park and M. Zhang, Biomaterials, 2010, 31, 8032–8042 CrossRef PubMed.
- Z. M. Avval, L. Malekpour, F. Raeisi, A. Babapoor, S. M. Mousavi, S. A. Hashemi and M. Salari, Drug Metab. Rev., 2020, 52, 157–184 CrossRef PubMed.
- S. Mirza, M. S. Ahmad, M. I. A. Shah and M. Ateeq, In Metal nanoparticles for drug delivery and diagnostic applications, Elsevier, 2020, pp. 189–213 Search PubMed.
- A. Gholami, S. M. Mousavi, S. A. Hashemi, Y. Ghasemi, W.-H. Chiang and N. Parvin, Drug Metab. Rev., 2020, 52, 205–224 CAS.
- X. Sun, G. Zhang, R. Du, R. Xu, D. Zhu, J. Qian, G. Bai, C. Yang, Z. Zhang, X. Zhang and D. Zou, Biomaterials, 2019, 194, 151–160 CrossRef CAS PubMed.
- Z. Zhou, L. Yang, J. Gao and X. Chen, Adv. Mater., 2019, 31, 1804567 CrossRef PubMed.
- D. D. Stueber, J. Villanova, I. Aponte, Z. Xiao and V. L. Colvin, Pharmaceutics, 2021, 13, 943 CrossRef CAS PubMed.
- N. M. El-Shafai, M. M. Abdelfatah, M. E. El-Khouly, I. M. El-Mehasseb, A. El-Shaer, M. S. Ramadan, M. S. Masoud and M. A. El-Kemary, Appl. Surf. Sci., 2020, 506, 144896 CrossRef CAS.
- L. Gloag, M. Mehdipour, D. Chen, R. D. Tilley and J. J. Gooding, Adv. Mater., 2019, 31, 1904385 CrossRef CAS PubMed.
- S. Khizar, H. Ben Halima, N. M. Ahmad, N. Zine, A. Errachid and A. Elaissari, Electrophoresis, 2020, 41, 1206–1224 CrossRef CAS PubMed.
- M. P. Conte, J. K. Sahoo, Y. M. Abul-Haija, K. A. Lau and R. V. Ulijn, ACS Appl. Mater. Interfaces, 2018, 10, 3069–3075 CrossRef CAS PubMed.
- S. Mallakpour and M. Hatami, Appl. Clay Sci., 2019, 174, 127–137 CrossRef CAS.
- N. Shabani, A. Javadi, H. Jafarizadeh-Malmiri, H. Mirzaei and J. Sadeghi, Food Hyg., 2021, 10, 61–71 Search PubMed.
- R. Banerjee, Y. Katsenovich, L. Lagos, M. McIintosh, X. Zhang and C.-Z. Li, Curr. Med. Chem., 2010, 17, 3120–3141 CrossRef CAS PubMed.
- S. Mallakpour, M. Tukhani and C. M. Hussain, Int. J. Biol. Macromol., 2021, 179, 429 CrossRef CAS PubMed.
- S. Kamel and T. A. Khattab, Cellulose, 2021, 28, 4545 CrossRef CAS.
- G. Mohammadi Ziarani, Z. Kheilkordi, F. Mohajer, A. Badiei and R. Luque, RSC Adv., 2021, 11, 17456–17477 RSC.
- G. Mohammadi Ziarani, S. Rohani, A. Ziarati and A. Badiei, RSC Adv., 2018, 8, 41048–41100 RSC.
- F. Mohajer, G. Mohammadi Ziarani and A. Badiei, RSC Adv., 2021, 11, 6517–6525 RSC.
- Z. Kheilkordi, G. Mohammadi Ziarani and F. Mohajer, Curr. Org. Chem., 2022, 26, 287–298 CrossRef.
- G. Mohammadi Ziarani, R. Moradi, T. Ahmadi and N. Lashgari, RSC Adv., 2018, 8, 12069–12103 RSC.
- G. Mohammadi Ziarani, F. Aleali and N. Lashgari, RSC Adv., 2016, 6, 50895–50922 RSC.
- G. Mohammadi Ziarani, N. H. Nasab and N. Lashgari, RSC Adv., 2016, 6, 38827–38848 RSC.
- L. A. Thompson, Curr. Opin. Chem. Biol., 2000, 4, 324–337 CrossRef CAS PubMed.
- A. Dömling, Curr. Opin. Chem. Biol., 2002, 6, 306–313 CrossRef.
- E. Zare and Z. Rafiee, Appl. Organomet. Chem., 2020, 34, e5516 CrossRef CAS.
- S. S. Hosseinikhah and B. B. F. Mirjalili, RSC Adv., 2020, 10, 40508–40513 RSC.
- P. G. Kargar, G. Bagherzade and H. Eshghi, RSC Adv., 2020, 10, 37086–37097 RSC.
- A. Maleki, S. Gharibi, K. Valadi and R. Taheri-Ledari, J. Phys. Chem. Solids, 2020, 142, 109443 CrossRef CAS.
- G. Bagherzade, RSC Adv., 2021, 11, 19203–19220 RSC.
- G. Bagherzade and H. Eshghi, RSC Adv., 2021, 11, 4339–4355 RSC.
- P. G. Kargar, M. Noorian, E. Chamani, G. Bagherzade and Z. Kiani, RSC Adv., 2021, 11, 17413–17430 RSC.
- M. Zahedifar, B. Pouramiri and R. Razavi, Res. Chem. Intermed., 2020, 46, 2749–2765 CrossRef CAS.
- B. B. F. Mirjalili, Z. Zaghaghi and A. Monfared, J. Chin. Chem. Soc., 2020, 67, 197–201 CrossRef CAS.
- N. Safajoo, B. B. F. Mirjalili and A. Bamoniri, Polycyclic Aromat. Compd., 2021, 41, 1241–1248 CrossRef CAS.
- N. Safajoo, B. B. F. Mirjalili and A. Bamoniri, RSC Adv., 2019, 9, 1278–1283 RSC.
- G. Bagherzade and H. Eshghi, RSC Adv., 2020, 10, 32927–32937 RSC.
- A. D. Tafti and B. B. F. Mirjalili, RSC Adv., 2020, 10, 31874–31880 RSC.
- A. Maleki, F. Hassanzadeh-Afruzi, Z. Varzi and M. S. Esmaeili, Mater. Sci. Eng., C, 2020, 109, 110502 CrossRef CAS PubMed.
- Z. Amiri-Khamakani, F. Hassanzadeh-Afruzi and A. Maleki, Chem. Process., 2020, 3, 101 Search PubMed.
- E. Babaei and B. B. F. Mirjalili, Iran. J. Catal., 2020, 10, 219–226 CAS.
- A. Mohammadzadeh, A. P. Marjani and A. Zamani, S. Afr. J. Chem., 2020, 73, 55–63 CrossRef CAS.
- M. Kamalzare, M. Bayat and A. Maleki, R. Soc. Open Sci., 2020, 7, 200385 CrossRef CAS PubMed.
- M. H. Sayahi, F. Shamkhani, M. Mahdavi and S. Bahadorikhalili, Starch-Stärke, 2021, 73, 2000257 CrossRef CAS.
- S. Forouzandehdel, M. Meskini and M. R. Rami, J. Mol. Struct., 2020, 1214, 128142 CrossRef CAS.
- M. Shamsi-Sani, F. Shirini and M. Mohammadi-Zeydi, J. Nanosci. Nanotechnol., 2019, 19, 4503–4511 CrossRef CAS PubMed.
- S. Amirnejat, A. Nosrati and S. Javanshir, Appl. Organomet. Chem., 2020, 34, e5888 CAS.
- S. Amirnejat, A. Nosrati, S. Javanshir and M. R. Naimi-Jamal, Int. J. Biol. Macromol., 2020, 152, 834–845 CrossRef CAS PubMed.
- M. Banazadeh, S. Amirnejat and S. Javanshir, Front. Chem., 2020, 8, 1023 Search PubMed.
- H. Ghavidel, B. Mirza and S. Soleimani-Amiri, Polycycl. Aromat. Compd., 2021, 41, 604–625 CrossRef CAS.
- S. Behrouz, M. N. Abi and M. A. Piltan, Acta Chim. Slov., 2021, 68, 374–386 CrossRef CAS.
- S. Behrouz, M. N. Abi and M. A. Piltan, J. Appl. Chem., 2019, 14, 109–124 Search PubMed.
- S. Asgharnasl, R. Eivazzadeh-Keihan, F. Radinekiyan and A. Maleki, Int. J. Biol. Macromol., 2020, 144, 29–46 CrossRef CAS PubMed.
- K. Javanmiri and R. Karimian, Monatsh. Chem., 2020, 151, 199–212 CrossRef CAS.
- H. Naeimi and S. Lahouti, J. Org. Chem. Res., 2020, 6, 54–68 Search PubMed.
- N. Motahharifar, M. Nasrollahzadeh, A. Taheri-Kafrani, R. S. Varma and M. Shokouhimehr, Carbohydr. Polym., 2020, 232, 115819 CrossRef CAS PubMed.
- P. Kamalzare, B. Mirza and S. Soleimani-Amiri, J. Nanostructure Chem., 2021, 11, 229–243 CrossRef CAS.
- E. Zare and Z. Rafiee, J. Taiwan Inst. Chem. Eng., 2020, 116, 205–214 CrossRef CAS.
- S. Bahrami, F. Hassanzadeh-Afruzi and A. Maleki, Appl. Organomet. Chem., 2020, 34, e5949 CrossRef CAS.
- M. S. Esmaeili, M. R. Khodabakhshi, A. Maleki and Z. Varzi, Polycyclic Aromat. Compd., 2021, 41, 1953–1971 CrossRef CAS.
|
This journal is © The Royal Society of Chemistry 2022 |
Click here to see how this site uses Cookies. View our privacy policy here.