DOI:
10.1039/D2RA01616H
(Paper)
RSC Adv., 2022,
12, 20983-20990
GO/iron series systems enhancing the pH shock resistance of anaerobic systems for sulfate-containing organic wastewater treatment
Received
14th March 2022
, Accepted 29th June 2022
First published on 21st July 2022
Abstract
In this study, the effect of pH shock during the treatment of sulfate-containing organic wastewater was investigated using an anaerobic fermentation system reinforced with graphene oxide (GO)/iron series systems. The results show that the anaerobic system with the GO/iron series systems exhibited enhanced resistance to pH shock. Among them, the GO/Fe0 system had the strongest resistance to pH shock, the systems of GO/Fe3O4 and GO/Fe2O3 followed close behind, while the blank system performed the worst. After pH shock, the CODCr removal rate, SO42− removal rate, and gas production of the GO/Fe0 group were significantly improved compared with those of the control group by 51.0%, 65.3%, and 34.6%, respectively, while the accumulation of propionic acid was the lowest. Further, detailed microbial characterization revealed that the introduction of the GO/iron series systems was beneficial to the formation of more stable anaerobic co-metabolic flora in the system, and the relative abundance of Geobacter, Clostridium, Desulfobulbus and Desulfovibrio increased after acidic and alkaline shock.
Introduction
Anaerobic digestion is a cost-effective technology for treating high-strength industrial wastewater,1,2 with the recovery of bioenergy in the form of biogas.3 However, microbial flora is sensitive to environmental changes under anaerobic conditions and the hydrogen sulfide produced by sulfate-reducing bacteria is highly toxic to the whole system,4 which ultimately leads to unsatisfactory treatment results. According to previous research,5–10 it is a good choice to enhance the effect of anaerobic systems by using graphene oxide (GO)/iron series systems to treat sulfate-containing wastewater, reduce the toxic effect of hydrogen sulfide and provide good environmental conditions for the growth and metabolism of organisms.
In anaerobic biogas digestion systems, the final wastewater treatment effect is influenced by various factors. As one of the important influencing factors, pH changes will affect microbial activity and growth metabolism, which in turn affects the treatment effect of wastewater, thus affecting effluent quality. Liu et al. found that pH had a significant effect on the microbial community structure in the system: microbial abundance under alkaline and acidic conditions was lower than that under neutral conditions, and the characteristics of bacteria and archaea showed great differences under different pH conditions.11 It has been reported that waste-activated sludge hydrolysis and volatile fatty acid (VFA) accumulation can be improved by inhibiting the activity of methanogenic bacteria through alkaline modulation.12 Li et al. found that the maximum hydrogen production rate could be obtained at pH 6.0 with a glucose concentration of 7.5 g L−1.13 As can be seen, pH is very important in enabling the system to achieve the desired treatment capacity and a suitable pH environment must therefore be provided during actual operation.
However, in the actual treatment of sulfate-containing wastewater, pH fluctuations occur not only in the influent water but also during operation in the anaerobic system. Elhenawy et al. found that the pH of the anaerobic digestion system could drop from 8.1 to 4.8.14 Therefore, it is an urgent need to further investigate methods to improve the treatment efficiency of sulfate-containing wastewater under unfavorable pH conditions. Sun et al. proposed that the introduction of nano zero-valent iron (NZVI) could buffer the pH value of the system and provide a favorable environment for the growth of acid-producing bacteria.15 It was also reported that anaerobic systems could exhibit stability after a short-term pH shock due to iron corrosion to maintain pH.16 Fe3+ has been confirmed to avoid the excessive accumulation of VFAs (especially acetic acid) and regulate the pH of the system.17 Moreover, sulfate-containing wastewater is mostly acidic, which is different from general wastewater.18 Therefore, many researchers have also worked on improving the efficiency of anaerobic systems for treating sulfate-containing wastewater by adding iron in recent years. It was found that the addition of Fe0 could provide a favorable pH for methane production and reduce the toxicity of sulfides, thus improving competition between methanogenic and sulfate-reducing bacteria.19,20 Liu et al. proposed that sulfate-reducing bacteria (SRB) can still use sulfate as a terminal electron acceptor to degrade organic compounds and convert SO42− to H2S after the addition of waste iron filings at low pH.21 However, these studies tend to avoid the effects of fluctuations in process parameters (e.g., acid strength changes, pH shocks, seeded sludge) and have not yet provided a detailed assessment of the effects of long-term pH shocks on sulfate reduction bioprocesses, while the response of microbial populations in sulfide reactors to pH shocks is not well understood. Therefore, a feasibility study on the pH shock resistance of anaerobic systems reinforced with GO/iron series systems for sulfate-containing organic wastewater treatment was considered.
In this paper, the pH shock resistance mechanism of GO/iron series systems has been revealed from the perspectives of a comprehensive treatment effect, the composition and content of terminal fermentation products, and microbial co-metabolic stability. These observations can provide a theoretical reference to speed up the application of new enhanced wastewater treatment technologies in practical engineering, which is very important in the fields of environment and bioenergy utilization.
Materials and methods
Wastewater and inoculated sludge
Simulated organic wastewater containing sulfate was used in the experiment. The chemical oxygen demand (COD) concentration was 5000 mg L−1. Ammonium sulfate and potassium dihydrogen phosphate were added as nitrogen and phosphorus sources, respectively, with a CODCr
:
N
:
P ratio of 500
:
5
:
1. The inoculation sludge was obtained from the up-flow anaerobic sludge bed (UASB) reactor of a brewery in Qingdao, China.
Experimental set-up
Four anaerobic sequencing batch reactors (ASBRs) with a working volume of 500 mL were used for the experiments. The reactors were made of transparent plexiglass for easier process monitoring and sealed lids isolated the system from air. Two air ducts were set in the lid and connected to the drainage device to measure the gas production. Nitrogen gas was used to purge the head-space oxygen from the reactors before the experiment. The reactors were placed on a shaker with a speed of 120 rpm.
Experimental methods
200 mL anaerobic activated sludge was added to four anaerobic reactors with a total volume of 500 mL each. Then, GO/Fe0, GO/Fe3O4 and GO/Fe2O3 (1.2, 1.58 and 1.62 g L−1, respectively) were added to the reactors and the system without complex was the control. The influent pH value was adjusted to 6 and tests were carried out in a water-bath shaker for 12 h (40 °C, 120 rpm). At the end of each cycle, the CODCr removal rate, SO42− removal rate, and gas production of the four reactors were tested. After 7 consecutive impact cycles, the VFA contents were determined. Then, the influent pH value was restored to about 7 and the recovery experiment was carried out by repeating the above steps until the anaerobic system returned to a normal level. The experiments of the shock of pH 8 were the same as the above procedure.
Analysis methods
pH was measured using a PHS-3C pH meter (Shanghai Remagnet). A DRB200 rapid digestion instrument (American HACH) was used for digestion, and a DR1010 COD analyzer (American HACH) was used to determine the concentration of COD. The content of sulfate in the system was determined by the gravimetric method. The gas production was determined by the drainage method. VFAs were determined by a GC2014C gas chromatograph (Shimadzu, Japan). In addition, the bacterial population and abundance in the activated sludge were determined via high-throughput sequencing.
Results and discussion
Treatment effect of anaerobic systems
The removal rate of CODCr and SO42− are two important parameters that judge the anaerobic treatment efficiency of sulfate-containing wastewater. The removal ratio of CODCr and SO42− in the GO/Fe0 system was the highest after pH shock (Fig. 1), with an about 51.0% and 65.3% increase, respectively, compared with the control, with the GO/Fe2O3 and GO/Fe3O4 systems following close behind. The variation of gas production was similar to the removal ratio, reaching 272 and 308 mL 12 h−1 (32.0% and 37.1% increase, Fig. 2), in the GO/Fe0 system, respectively. Previous studies have shown that Fe2+ on the surface of the complex could stimulate the synthesis of key enzymes, promote microbial growth and favor bacterial interactions, thus promoting microbial aggregation.22,23 Therefore, microorganisms could maintain normal growth and metabolism even under unfavorable pH conditions in the presence of GO/iron systems. These results suggest that the introduction of the GO/iron series systems can reduce the inhibition of anaerobic systems by pH shock to a certain extent. It was also reported that the mixed addition of NZVI and BC powder (1
:
1) could increase the pH stability of the system and promote the degradation of organic acids.24
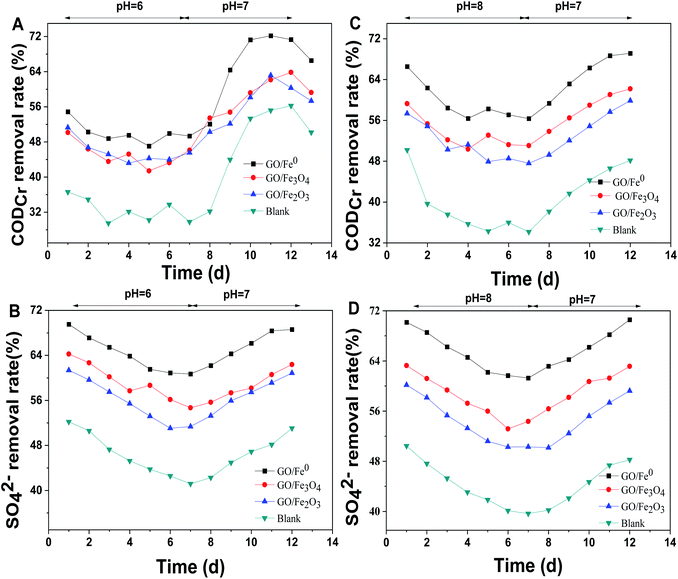 |
| Fig. 1 Effect of pH shock on CODCr and SO42− removal rate ((A): CODCr removal rate of pH = 6 shock, (B): SO42− removal rate of pH = 6 shock, (C): CODCr removal rate of pH = 8 shock, (D): SO42− removal rate of pH = 8 shock). | |
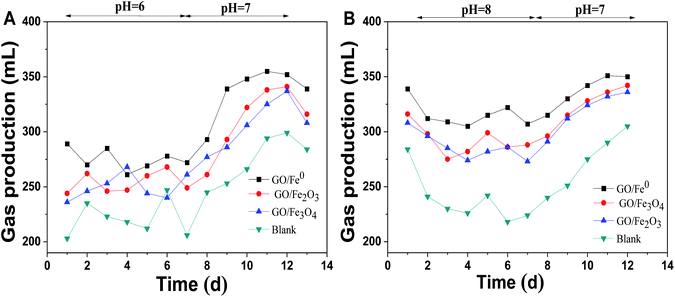 |
| Fig. 2 Effect of pH shock on the gas volume of the effluent ((A): gas volume of pH = 6 shock, (B): gas volume of pH = 8 shock). | |
Moreover, in our experiments, the CODCr removal rate decreased significantly, but the sulfate removal rate remained at a high level after pH shock. This was likely due to methanogenic bacteria being more sensitive to pH changes than sulfate-reducing bacteria, resulting in sulfate-reducing bacteria showing a significant competitive advantage under pH shock.25 In addition, we found that the anaerobic digestion efficiency was better under alkaline shock than under acidic shock. It may be attributed to the different toxicity of sulfides to microorganisms in different states. According to the H2S/HS− conjugated ion distribution curve, when the pH was greater than 8, more than 90% of the sulfide exists in the form of HS−, but about 99% of the sulfide exists in the form of H2S at pH less than 5.26 Because the ionic HS− and S2− do not easily enter the interior of the cell, the tolerance of microorganisms to them was high. Therefore, under the same concentration conditions, the degree of inhibition of H2S, HS−, and S2− to microorganisms was of the order H2S > HS− > S2−, which leads to a difference in alkaline and acidic shock.
The effect of pH shock on the matrix degradation kinetics is shown in Fig. 3. It can be seen that whether it was acid shock or alkaline shock, the degradation rate of the system with the GO/iron system was higher than that of the blank system, and the degradation rate constant k of the GO/Fe0 system was the largest, suggesting that the anaerobic microorganisms in the system with added GO/iron series systems could maintain a high activity under pH shock, with a faster organic matter degradation rate and shorter processing cycles. Therefore, the addition of GO/iron series systems could improve the pH shock resistance of anaerobic systems.
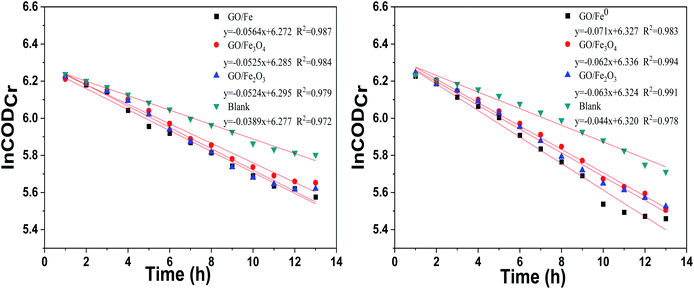 |
| Fig. 3 Fitted curve of first-order kinetics. | |
Changes in effluent pH and VFA content
As depicted in Fig. 4, the pH values in the GO/iron systems were maintained at 6.5–6.8 and 7.4–7.6 after acidic and alkaline shocks, respectively. Compared with the blank group (6.3 and 7.7), it was more conducive to creating the environment required for the growth and reproduction of microorganisms, indicating that the GO/iron series systems were beneficial to improving the resistance of the anaerobic system to pH shock. This was likely due to a formation of FeS precipitates in the presence of iron, as FeS precipitates can effectively buffer the pH value of the system.27 Therefore, the pH in the GO/iron system could be restored to normal values relatively quickly after shock. The changes in the VFA fraction and content after impact are shown in Fig. 5. After the system was subjected to pH shock, VFAs in each anaerobic system accumulated to a certain extent, and the content and proportion of propionic acid increased significantly. However, after 12 h of treatment, propionic acid was almost completely degraded for the GO/iron systems, and the blank group still had a certain accumulation. According to previous research, propionic acid is an important metabolic intermediate in anaerobic systems and is toxic to microorganisms,28 so rapid degradation of propionic acid in the GO/iron systems was beneficial for anaerobic digestion. In addition, we found that the content of propionic acid was lower under alkaline shock than under acidic shock, which was consistent with the results of previous studies.29
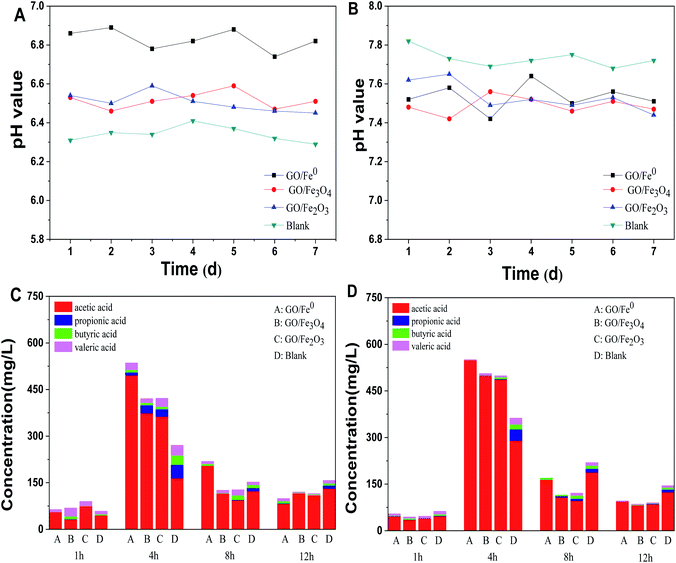 |
| Fig. 4 Effect of pH shock on the pH value and VFA contents of the effluent ((A): pH value of pH = 6 shock, (B): pH value of pH = 8 shock, (C): VFA contents of the effluent of pH = 6 shock, (D): VFA contents of the effluent of pH = 8 shock). | |
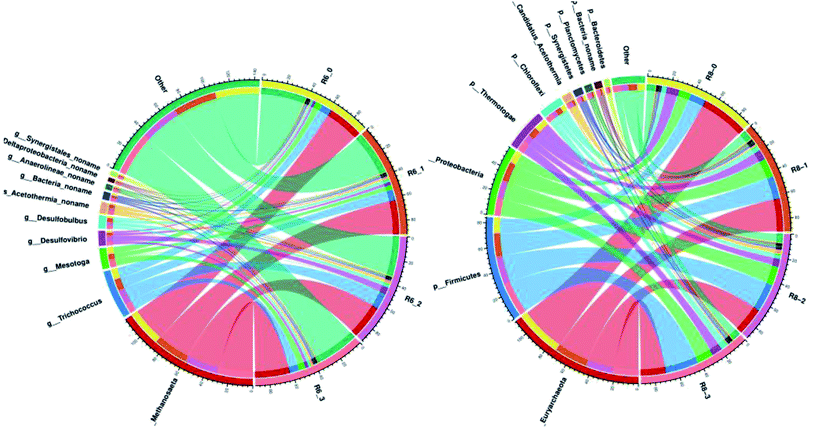 |
| Fig. 5 Collinearity diagram of the community composition of microbial taxa (genus): left: pH = 6; right: pH = 8. | |
The changes in pH and VFA fractions and contents suggested that GO/iron systems can promote the degradation of propionic acid, create a suitable pH environment for microorganisms, and thus strengthen the resistance of anaerobic systems to pH shocks.
Effect of pH shock on microbial flora
The microbial community composition in different systems was analyzed via high-throughput sequencing (Fig. 5 and Table 1). The sequencing results reveal that the microbial flora distribution remarkably changed in the presence of GO/iron series systems after pH shock.
Table 1 The distribution of microflora at the genus level
Microorganism |
pH |
Blank |
GO/Fe0 |
GO/Fe3O4 |
GO/Fe2O3 |
Main function |
Trichococcus |
6 |
9.02% |
9.44% |
13.20% |
8.06% |
Degrade organic matter and produce acid |
8 |
4.22% |
10.95% |
13.34% |
17.01% |
Clostridium |
6 |
0.59% |
0.63% |
0.65% |
0.70% |
8 |
1.03% |
0.84% |
1.11% |
1.22% |
Mesotoga |
6 |
5.90% |
4.28% |
0.57% |
6.03% |
8 |
8.45% |
6.69% |
5.16% |
7.14% |
Methanosaeta |
6 |
30.54% |
29.07% |
28.96% |
29.56% |
Acetic acid type methanogenesis |
8 |
35.65% |
25.62% |
21.65% |
19.72% |
Geobacter |
6 |
0.36% |
0.45% |
0.44% |
0.39% |
Dissimilated iron reduction, DIET |
8 |
0.29% |
0.45% |
0.37% |
0.38% |
Desulfobulbus |
6 |
1.57% |
2.97% |
3.41% |
2.29% |
Sulfate reduction |
8 |
1.41% |
3.42% |
2.10% |
2.99% |
Desulfuromonas |
6 |
0.21% |
0.27% |
0.26% |
0.23% |
8 |
0.16% |
0.29% |
0.22% |
0.23% |
Desulfovibrio |
6 |
1.68% |
2.26% |
5.61% |
2.29% |
8 |
1.44% |
1.69% |
2.14% |
2.00% |
Desulfomicrobium |
6 |
0.31% |
0.16% |
0.15% |
0.19% |
8 |
0.06% |
0.08% |
0.08% |
0.08% |
Geobacter, Clostridium, Desulfobulbus and Desulfovibrio, the main functional bacteria, increased significantly in the experimental group after pH shock. Geobacter, which can exchange electrons via direct interspecies electron transfer with Methanosaeta and regulate electron flux,30,31 had a distinct increase in the GO/Fe0 system by 25.0% and 55.2% after acidic and alkaline shock, respectively, compared with the control. Clostridium plays a key role in the degradation of VFAs and can promote the production of methane,32,33 which had a higher abundance in the GO/iron system after shock. The enriched Geobacter and Phylum Firmicutes further confirm the positive role of GO/iron series systems in promoting electron transfer and the degradation of organic matter even in uncomfortable pH environments. Consequently, the inhibition of excessive accumulation of propionic acid on microorganisms was avoided to some extent. However, the relative abundance of Methanosaeta, important for methane production, decreased slightly after pH shock, which may be because Methanosaeta are more sensitive to pH than other microflora.34 Desulfobulbus and Desulfovibrio belong to the genus of sulfate-reducing bacteria, both of which have high resistance to pH shock and can degrade propionic acid to produce acetic acid.35 In our experiment, the abundance of sulfate-reducing bacteria in the GO/iron systems increased significantly after shock, suggesting that the introduction of the GO/iron series systems can improve the activity of sulfate-reducing bacteria, which was consistent with the results of previous studies.36 Therefore, a high removal rate of SO42− could still be guaranteed even when the anaerobic system was subjected to pH shock. Moreover, changes in the relative abundance of bacterial flora under alkaline shock were more visible than those under acidic shock, which further explained the previous experimental results (i.e., the removal rate of CODCr and SO42− and gas production).
In conclusion, changes in the microbial flora indicate that the introduction of GO/iron series systems is conducive to the formation of a more stable anaerobic co-metabolic system; thus, the pH shock resistance of anaerobic systems can be enhanced.
Strengthening mechanism analysis
The strengthening mechanism of GO/iron systems under pH shock is summarized in Fig. 6. The stability of co-metabolic flora decreased significantly after pH shock, inhibiting the normal degradation of organic matter. However, the addition of GO/iron series systems could accelerate direct interspecies electron transfer (DIET) between Geobacter and Methanosaeta, promoting organic matter degradation and avoiding the inhibition of excessive accumulation of VFAs on the microorganisms. In addition, the formation of FeS precipitates reduced the toxic effect of sulfide in different states on the microbiological flora under pH shock. As a result, the system can form a more stable anaerobic co-metabolizing bacterial community under unfavorable pH conditions, which made the system more resistant to pH shock and improved the treatment efficiency of sulfate-containing wastewater.
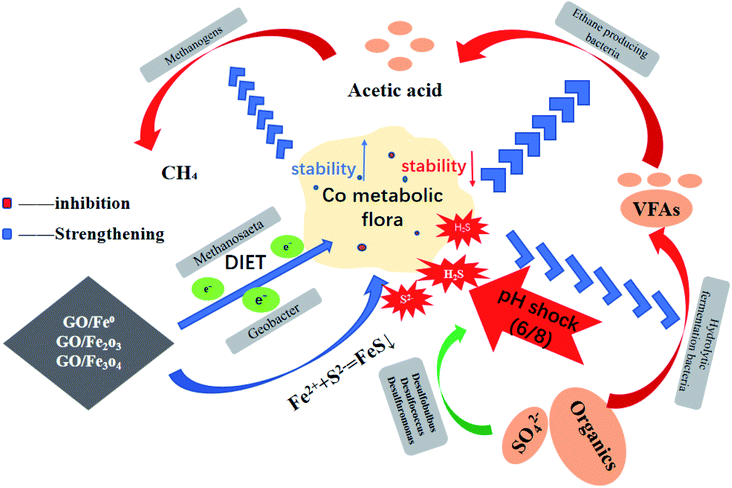 |
| Fig. 6 Analysis of the strengthening mechanism of GO/iron systems under pH shock. | |
Conclusions
In this work, it was found that GO/iron series systems not only promote organic matter degradation by accelerating the DIET between Geobacter and Methanosaeta but also reduce the toxic effect of sulfide on microbiological flora with the formation of FeS precipitates. Therefore, the CODCr removal rate, SO42− removal rate and gas production of the GO/iron series anaerobic systems were better compared to those of the control after pH shock. It was also confirmed that there was no excessive accumulation of propionic acid in the GO/Fe0 group, and the pH was close to 7, which provided a more favorable environment for the co-metabolic flora. In addition, in the presence of GO/iron series systems, acidic and alkaline shock increased the relative abundance of functional flora such as Geobacter, Clostridium, Desulfobulbus and Desulfovibrio, which was conducive to the formation of more efficient and stable co-metabolic flora. Overall, the experimental results demonstrate that the dosing of GO/iron series systems can be applied to enhance the pH shock resistance of anaerobic systems for sulfate-containing organic wastewater treatment, which provides a theoretical reference for the practical engineering application of new wastewater treatment technology.
Conflicts of interest
There are no conflicts to declare.
Acknowledgements
The authors are grateful to the National College Student Innovation and Entrepreneurship Training Program (Approval Number: 202110426100).
References
- G. Lourinho, L. F. T. G. Rodrigues and P. S. D. Brito, Recent advances on anaerobic digestion of swine wastewater, Int. J. Environ. Sci. Technol., 2020, 17(12), 4917–4938 CrossRef CAS.
- N. R. Khandaker, S. S. Islam and U. F. Shakin, Biogas Generation from Rice Cooking Wastewater, J. Environ. Treat. Tech., 2020, 8, 794–796 Search PubMed.
- A. A. Ansari, A. Khalid, C. H. Correia, M. Dheen, R. Talal, Y. Elshamy and F. Doucet, Feasibility Study of Biogas Production and Applications using Rural Biomass in Northern Queensland, Australia, J. Environ. Treat. Tech., 2022, 10(1), 124–133 Search PubMed.
- F. R. Oliveira, K. C. Surendra, D. P. Jaisi, H. Lu, G. Unal-Tosun, S. Sung and S. Kumar Khanal, Alleviating sulfide toxicity using biochar during anaerobic treatment of sulfate-laden wastewater, Bioresour. Technol., 2020, 301, 122711 CrossRef CAS PubMed.
- B. Song, J. Tang and M. Zhen, et al. Influence of graphene oxide and biochar on anaerobic degradation of petroleum hydrocarbons, J. Biosci. Bioeng., 2019, 128(1), 72–79 CrossRef CAS PubMed.
- J. Zhang, Z. Wang and Y. Wang, et al. Effects of graphene oxide on the performance, microbial community dynamics and antibiotic resistance genes reduction during anaerobic digestion of swine manure, Bioresour. Technol., 2017, 245, 850–859 CrossRef CAS PubMed.
- S. Karri, R. Sierra-Alvarez and J. A. Field, Zero valent iron as an electron-donor for methanogenesis and sulfate reduction in anaerobic sludge, Biotechnol. Bioeng., 2005, 92(7), 810–819 CrossRef CAS PubMed.
- D. Ma, J. Wang and T. Chen, et al. Iron-oxide-promoted anaerobic process of the aquatic plant of curly leaf pondweed, Energy Fuels, 2015, 29(7), 4356–4360 CrossRef CAS.
- C. Cruz Viggi, S. Rossetti and S. Fazi, et al. Magnetite particles triggering a faster and more robust syntrophic pathway of methanogenic propionate degradation, Environ. Sci. Technol., 2014, 48(13), 7536–7543 CrossRef CAS PubMed.
- T. Wang, D. Zhang and L. Dai, et al. Effects of metal nanoparticles on methane production from waste-activated sludge and microorganism community shift in anaerobic granular sludge, Sci. Rep., 2016, 6(1), 1–10 CrossRef PubMed.
- He Liu, J. Wang, X. Liu, Bo Fu, J. Chen and Yu Han-Qing, Acidogenic fermentation of proteinaceous sewage sludge: Effect of pH, Water Res., 2012, 46(3), 799–807 CrossRef CAS PubMed.
- Y. Yan, L. Feng, C. Zhang, C. Wisniewski and Z. Qi, Ultrasonic enhancement of waste activated sludge hydrolysis and volatile fatty acids accumulation at pH 10.0, Water Res., 2010, 44(11), 3329–3336 CrossRef CAS PubMed.
- Z. Li, H. Wang, Z. Tang, X. Wang and J. Bai, Effects of pH value and substrate concentration on hydrogen production from the anaerobic fermentation of glucose, Int. J. Hydrogen Energy, 2008, 33(24), 7413–7418 CrossRef CAS.
- Y. Elhenawy, S. El-Kadi, K. Elsawy, A. Abdelmotalip and I. AbdelrahmanIbrahim, Biogas production by anaerobic digestion of cow dung using floating type fermenter, J. Environ. Treat. Tech., 2021, 9(2), 446–451 Search PubMed.
- M. Sun, Z. Zhang and G. Liu, et al. Enhancing methane production of synthetic brewery water with granular activated carbon modified with nanoscale zero-valent iron (NZVI) in anaerobic system, Sci. Total Environ., 2021, 760, 143933 CrossRef CAS PubMed.
- F. Xie, B. Zhao, Y. Cui, Ma Xiao, D. Li and X. Yue, Enhancing nitrogen removal performance of anammox process after short-term pH and temperature shocks by coupling with iron-carbon micro-electrolysis, J. Cleaner Prod., 2020, 289, 125753 CrossRef.
- B. Yu, Z. Dongling, D. Xiaohu, L. Ziyang, Y. Haiping and Z. Nanwen, The synthetic effect on volatile fatty acid disinhibition and methane production enhancement by dosing FeCl3 in a sludge thermophilic anaerobic digestion system, RSC Adv., 2016, 6(25), 21090–21098 RSC.
- J. Zhao, D. Fang, P. Zhang and L. Zhou, Long-term effects of increasing acidity on low-pH sulfate-reducing bioprocess and bacterial community, Environ. Sci. Pollut. Res., 2016, 24(4), 4067–4076 CrossRef PubMed.
- J. Geets, B. Borremans, L. Diels and D. Van der Lelie, Molecular monitoring of SRB community structure and dynamics in batch experiments to examine the applicability of in situ precipitation of heavy metals for groundwater remediation, J. Soils Sediments, 2005, 5, 149–163 CrossRef CAS.
- N. Paepatung, W. Songkasiri, H. Yasui and C. Phalakornkule, Enhancing methanogenesis in fed-batch anaerobic digestion of high-strength sulfate-rich wastewater using zero valent scrap iron, J. Environ. Chem. Eng., 2020, 8(6), 104508 CrossRef CAS.
- X. Liu, W. Gong and L. Liu, Treatment of sulfate-rich and low pH wastewater by sulfate reducing bacteria with iron shavings in a laboratory, Water Sci. Technol., 2014, 69(3), 595–600 CrossRef CAS PubMed.
- G. Zhen, X. Lu, Y. Y. Li, Y. Liu and Y. Zhao, Influence of zero valent scrap iron (ZVSI) supply on methane production from waste activated sludge, Chem. Eng. J., 2015, 263, 461–470 CrossRef CAS.
- C. Cruz Viggi, S. Rossetti, S. Fazi, P. Paiano, M. Majone and F. Aulenta, Magnetite particles triggering a faster and more robust syntrophic pathway of methanogenic propionate degradation, Environ. Sci. Technol., 2014, 48, 7536–7543 CrossRef CAS PubMed.
- Y. Jiao, H. Xue, C. He, Z. Wang, X. Ma, X. Liu, L. Liu, C. Chang, F. Petracchini and P. Li, Effect of combined addition amount of nano zero-valent iron and biochar on methane production by anaerobic digestion of corn straw, Environ. Dev. Sustain., 2021, 24, 4709–4726 CrossRef.
- I. Kushkevych, M. Vitezova, V. Tomas and M. Bartos, Production of biogas: relationship between methanogenic and sulfate-reducing microorganisms, Open Life Sci., 2017, 12(1), 82–91 CAS.
- J. M. Costa, K. C. Castro and R. P. Rodriguez, et al. Anaerobic reactors for the treatment of sulphate and metal-rich wastewater: a review, Int. J. Environ. Anal. Chem., 2020, 1–12 Search PubMed.
- X. Liu, W. Gong and L. Liu, Treatment of sulfate-rich and low pH wastewater by sulfate reducing bacteria with iron shavings in a laboratory, Water Sci. Technol., 2014, 69(3), 595–600 CrossRef CAS PubMed.
- K. Xiao, Z. Yan, G. Chenghong, M. Yogananda and W.-J. Ng, Dynamics of propionic acid degradation in a two-phase anaerobic system, Chemosphere, 2015, 140, 47–53 CrossRef CAS PubMed.
- D. R. Boone and L. Xun, Effects of pH, temperature, and nutrients on propionate degradation by a methanogenic enrichment culture, Appl. Environ. Microbiol., 1987, 53(7), 1589–1592 CrossRef CAS PubMed.
- D. E. Holmes, P. M. Shrestha, D. J. F. Walker, Y. Dang, K. P. Nevin, T. L. Woodard and D. R. Lovley, Metatranscriptomic Evidence for Direct Interspecies Electron Transfer between Geobacter and Methanothrix Species in Methanogenic Rice Paddy Soils, Appl. Environ. Microbiol., 2017, 83(9), e00223-17 CrossRef PubMed.
- C. Valentin, B. Lucille, P. Füeg Michael, K. Maksym, S. Madivoli Edwin, H. Nelly, M. Dantas Joana, A. Salgueiro Carlos, B. Giese and M. Fromm Katharina, Kinetics and Mechanism of Mineral Respiration: How Iron Hemes Synchronize Electron Transfer Rates, Angew. Chem., Int. Ed. Engl., 2019 DOI:10.1002/anie.201914873.
- S. Ai, H. Liu, M. Wu, G. Zeng and C. Yang, Roles of acid-producing bacteria in anaerobic digestion of waste activated sludge, Front. Environ. Sci. Eng., 2018, 12(6), 1–11 CrossRef CAS.
- E. Marie Fykse, T. Aarskaug, E. H. Madslien and M. Dybwad, Microbial community structure in a full-scale anaerobic treatment plant during start-up and first year of operation revealed by high-throughput 16S
rRNA gene amplicon sequencing, Bioresour. Technol., 2016, 222, 380–387 CrossRef PubMed.
- H. H. Chou, J. S. Huang, W. G. Chen and R. Ohara, Competitive reaction kinetics of sulfate-reducing bacteria and methanogenic bacteria in anaerobic filters, Bioresour. Technol., 2008, 99(17), 8061–8067 CrossRef CAS PubMed.
- D. Zeng, Q. Yin and Q. Du, et al., System performance and microbial community in ethanol-fed anaerobic reactors acclimated with different organic carbon to sulfate ratios, Bioresour. Technol., 2019, 278, 34–42 CrossRef CAS PubMed.
- D. Y. Zhang, J. L. Wang, J. Y. Zhao, Y. Y. Cai and Q. H. Lin, Comparative Study of Nickel Removal from Synthetic Wastewater by a SulfateReducing Bacteria Filter and a Zero Valent Iron-Sulfate-Reducing Bacteria Filter, Journal of Geological Microbiology, 2016, 33(3–4), 226–234 Search PubMed.
|
This journal is © The Royal Society of Chemistry 2022 |
Click here to see how this site uses Cookies. View our privacy policy here.