DOI:
10.1039/D2RA01629J
(Paper)
RSC Adv., 2022,
12, 12471-12476
Preparation of quinazolinones using biosynthesized silver nanoparticles†
Received
12th March 2022
, Accepted 6th April 2022
First published on 25th April 2022
Abstract
A silver nanocatalyst has been used as an effective catalyst for the preparation of quinazolinones under reflux conditions in ethanol. The catalyst was characterized by UV-VIS, FT-IR, XRD, SEM and EDS. Amongst the many benefits of this method are atom economy, reusability of the catalyst, low catalyst loading, applicability to a wide range of substrates, high yields of products, environmental friendliness and easy separation of products. Silver nanoparticles (Ag NPs) were prepared using Echium amoenum extract. The structures of the prepared quinazolinones were fully characterized by 1H and 13C NMR, FT-IR spectra and elemental analysis.
1. Introduction
Quinazolinones show many biological activities, including antioxidant,1 antifungal,2 antibacterial,3 anticancer,4 anti-inflammatory5 and analgesic properties.6 Therefore, seeking efficient and simple techniques for the preparation of quinazolinones through multicomponent reactions (MCRs) is an attractive area of research in organic and medicinal chemistry. Many quinazolinones have been synthesized in the presence of diverse catalysts including trimethylsilyl chloride,7 hydrochloric acid,8 iron(III) chloride,9 copper(I) iodide,10 cyanuric chloride,11 zinc(II) perfluorooctanoate,12 indium(III) trifluoromethanesulfonate,13 palladium acetate-bidentate phosphine14 and Bi(TFA)3–[nbp]FeCl4.15 However, some of the reported synthesis methods hold several drawbacks, including prolonged reaction times, low yields, non-reusability of the catalyst and undesirable reaction conditions. Therefore, it is essential to develop easy, suitable and efficient methods for the preparation of a wide range of organic compounds under heterogeneous catalytic conditions.16,17 The development of environmentally benign and green catalysts, which can be easily recycled at the end of a reaction, has obtained great attention in recent years. Given the growing concerns about environmental issues, green chemistry has evolved as a key solution, which is concerned with developing efficient recoverable heterogeneous catalysts and green techniques for application in organic syntheses and industrial procedures. Recently, the green synthesis of metallic nanoparticles (NPs) has attracted much attention. In particular, biosynthetic synthesis methods for metal NPs have several benefits, including simplicity, low toxicity and low cost, as well as suitability for biomedical and pharmaceutical applications. Among biosynthetic routes for the synthesis of metal nanoparticles, plant extracts have received substantial attention, due to simple sampling and environmental friendliness. In addition, many plant extracts have reducing and antioxidant effects.18–20 We wish to report herein a highly efficient method for the preparation of quinazolinones using Ag nanoparticles as an efficient and reusable heterogeneous catalyst under reflux conditions in ethanol (Scheme 1). We also report the green synthesis of silver nanoparticles (Ag NPs) using Echium amoenum (E. amoenum) extract. Recently, silver nanoparticles have been used as a suitable catalyst in many reactions, including the oxidation of alcohol,21 oxidation of styrene,22 synthesis of β-enaminones,23 preparation of tetrazoles,24 oxidation of phenylsilanes to silanols25 and synthesis of propargylamines.26 We found that our Ag NPs produce our desired compounds in high yields (80–97%) with excellent recovery and a simple work-up procedure. In addition, the Ag NPs have good recycling properties, and this advantage is important from an economic point of view.
 |
| Scheme 1 Synthesis of quinazolinones using biosynthesized Ag NPs. | |
2. Results and discussion
In the present study, Ag NPs were synthesized using Echium amoenum extract. In our investigation, we focused on the preparation of nanoparticles in aqueous media using the reducing activities of antioxidant phytochemicals inside the plant, especially polyphenolics as a main reducing agent and polyhydroxyl as a highly polar agent. The UV-VIS absorption spectrum of the plant extract and prepared Ag NPs is given in Fig. 1. It shows a characteristic peak centered at 400 nm in the visible light region.
 |
| Fig. 1 UV-VIS of Ag NPs and plant extract. | |
The powder X-ray diffraction (XRD) pattern of the synthesized Ag NPs is depicted in Fig. 2. The pattern agrees well with the reported pattern for other Ag nanoparticles. The 2θ peaks of 38.64°, 45.06°, 64.51° and 77.01° are related to the Ag NPs (JCPDS No. 01-087-0717).
 |
| Fig. 2 XRD of Ag NPs. | |
Fig. 3 reveals the scanning electron microscopy (SEM) images of the Ag NPs. The images show that the nanostructure is made up of rods with sizes in the nanometer range.
 |
| Fig. 3 SEM of Ag NPs. | |
The elemental composition of the nanocatalyst was studied using Energy Dispersive Spectroscopy (EDS) (Fig. 4). The elements in the extract include carbon, nitrogen, oxygen, and sulfur and the element in the nanostructure was silver.
 |
| Fig. 4 EDS of the nanocatalyst. | |
Fig. 5 shows the FT-IR spectra of Ag NPs synthesized using Echium amoenum extract. The absorption peak at 3430 cm−1 is related to the stretching vibrational absorptions of –OH groups. The peaks at 1670 and 1410 cm−1 correspond to C
O and C
C, respectively, in the extract.
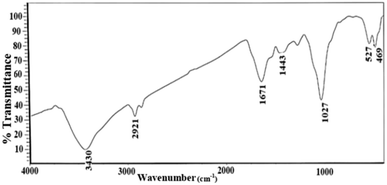 |
| Fig. 5 FT-IR of Ag NPs synthesized using Echium amoenum extract. | |
Initially, we carried out a three-component reaction of benzaldehyde (1.0 mmol), dimedone (1 mmol) and urea (1.2 mmol) as a model reaction. The model reaction was performed using Et3N, p-TSA, CH3COOH, NaHSO4, NiO and Ag NPs. The reactions were tested using diverse solvents including ethanol, acetonitrile, acetone, water and dimethylformamide. The best results were gained in ethanol and we found that the reaction gave convincing results in the presence of Ag NPs (2 mg) under reflux conditions (Table 1). We also applied Ag NPs for the synthesis of quinazolinone derivatives from various aromatic aldehydes under similar conditions, as presented in Table 2. The results in this table indicate that excellent yields were achieved in the presence of Ag NPs (2 mg) under reflux conditions.
Table 1 Optimization of reaction condition using different catalystsa
Entry |
Catalyst (amount) |
Solvent (reflux) |
Time (min) |
Yieldb (%) |
Reaction conditions: benzaldehyde (1.0 mmol), dimedone (1 mmol) and urea (1.2 mmol). Isolated yield. |
1 |
None |
EtOH |
350 |
NR |
2 |
Et3N (5 mol%) |
EtOH |
200 |
12 |
3 |
NaHSO4 (5 mol%) |
EtOH |
200 |
38 |
4 |
CH3COOH (5 mol%) |
EtOH |
250 |
27 |
5 |
pTSA (5 mol%) |
EtOH |
150 |
58 |
6 |
NiO (5 mol%) |
EtOH |
200 |
50 |
7 |
Ag NPs (3 mg) |
DMF |
150 |
61 |
8 |
Ag NPs (3 mg) |
H2O |
150 |
65 |
9 |
Ag NPs (3 mg) |
Acetone |
100 |
73 |
10 |
Ag NPs (3 mg) |
CH3CN |
90 |
79 |
11 |
Ag NPs (1 mg) |
EtOH |
40 |
85 |
12 |
Ag NPs (2 mg) |
EtOH |
30 |
92 |
13 |
Ag NPs (3 mg) |
EtOH |
30 |
92 |
Table 2 Synthesis of quinazolinones using Ag NPs (2 mg) under reflux conditions
Entry |
Product |
R aldehydes |
Time (min) |
Yielda (%) |
MP °C |
MP °C [ref] |
Isolated yield. |
1 |
4a |
4-Cl |
20 |
95 |
298–300 |
297–299 [27] |
2 |
4b |
2-OH-5-Br |
30 |
90 |
352–355 |
— |
3 |
4c |
3-NO2 |
25 |
94 |
303–305 |
295–297 [27] |
4 |
4d |
4-OH |
40 |
82 |
274–276 |
299–300 [28] |
5 |
4e |
4-NO2 |
20 |
97 |
308–310 |
293–294 [28] |
6 |
4f |
H |
30 |
92 |
288–290 |
292–293 [28] |
7 |
4g |
2,4-Cl |
20 |
97 |
270–272 |
270–272 [28] |
8 |
4h |
4-Isopropyl |
40 |
84 |
299–301 |
— |
9 |
4i |
2-Cl |
20 |
94 |
292–294 |
271–273 [13] |
10 |
4j |
4-OCH3 |
40 |
80 |
278–280 |
272–274 [13] |
11 |
4k |
4-CH3 |
40 |
82 |
298–300 |
300–302 [13] |
12 |
4l |
3-Cl |
25 |
94 |
296–298 |
290–292 [13] |
We also assessed the recycling of Ag NPs as a catalyst for the model reaction under reflux conditions in ethanol. The results showed that the nanoparticles can be reused several times without a noticeable loss of catalytic activity (yields from 92 to 90%) (Fig. 6).
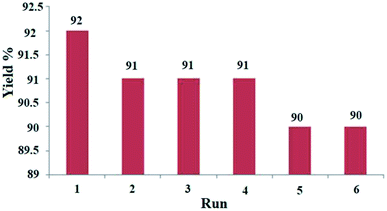 |
| Fig. 6 Reusability of Ag NPs as a catalyst for the synthesis of 4f. | |
A plausible mechanism for the preparation of quinazolinones using Ag NPs is shown in Scheme 2. Firstly, we assumed that the reaction occurs via a condensation between dimedone and aldehyde, to form the intermediate (I) on the active sites of the Ag nanocatalyst. Then, urea added to intermediate I gives the intermediate II. The final product is formed by an intra-molecular cyclization reaction. In this mechanism, the surface atoms of the Ag nanocatalyst activate the C
O groups for better reaction with nucleophiles.
 |
| Scheme 2 Proposed mechanism for the synthesis of quinazolinones. | |
3. Experimental
3.1. General
All materials were commercially purchased from Merck and Sigma-Aldrich. Samples of Echium amoenum were collected from the Jennat Rudbar area (Mazandaran, Iran). Powder X-ray diffraction (XRD) was carried out on a Philips diffractometer from X'pert Company with monochromatized Ag Kα radiation (λ = 1.5406 Å). Electronic spectra of the samples were obtained on a JASCO UV-VIS scanning spectrometer (Model V-670). Scanning Electron Microscopy and Energy Dispersive X-ray analysis (MIRA3-TESCAN FESEM) were used to provide information about the morphology and elemental composition. Fourier transform infrared measurements were carried out on a Magna 550 instrument using potassium bromide (KBr) plates. NMR spectra were recorded on a Bruker 400 MHz spectrometer with DMSO-d6 as a solvent and TMS as an internal standard.
3.2. Biosynthesized Ag NPs
Samples of Echium amoenum were completely powdered. 20 g of Echium amoenum powder was mixed with 200 ml of deionized water in a 500 ml flask. Then, it was placed under a magnetic stirrer for 24 hours at 70 °C and extraction was performed by centrifugation. In the next step, 10 ml of the extract with 90 ml of a 1 mM solution of AgNO3 was placed on a magnetic stirrer for 3 hours and centrifuged again. The resulting precipitate was dried in an oven for 20 hours. XRD, FT-IR, FE-SEM and EDAX analyzes were used to confirm the final product. The supernatant of the centrifuged sample was also used for UV-VIS testing.
3.3. Synthesis of quinazolinones using biosynthesized Ag NPs
A mixture of aryl aldehyde (1 mmol), dimedone (1 mmol), urea (1.2 mmol) and 2 mg of silver nanocatalyst was stirred in ethanol (10 ml) under reflux conditions. The progression of the reaction was assessed by thin layer chromatography (TLC). After completion of the reaction, the catalyst was insoluble in ethanol and it could therefore be recycled by simple filtration. The filtrate solution was crystallized with ethanol. After being placed in a desiccator under vacuum, it was completely dried and prepared for identification experiments.
3.4. Spectral data
4-(4-Chloro-phenyl)-7,7-dimethyl-4,6,7,8-tetrahydro-1H,3H-quinazoline-2,5-dione (4a). White solid, m. p. 298–300 °C, IR (KBr): νmax 3423, 3220, 1627, 1459, 647, 829; 1H NMR (400 MHz, DMSO-d6): δ 0.88 (s, 3H), 1.02 (s, 3H), 2.07 (d, J = 20 Hz, 1H), 2.24 (d, J = 20 Hz, 1H), 2.40 (d, J = 12 Hz, 2H), 5.19 (s, 1H), 7.21–7.24 (m, 2H), 7.41–7.43 (m, 2H), 9.68 (s, NH), 10.60 (s, NH); 13C NMR (100 MHz, DMSO-d6): δ 27.49, 29.18, 32.78, 33.56, 50.32, 53.23, 109.09, 116.32, 126.88, 142.94, 147.36, 151.83, 152.09, 190.07. Anal. calcd for C16H17ClN2O2: C, 63.05; H, 5.62; N, 9.19; found: C, 63.10; H, 5.67; N, 9.10.
4-(2-Hydroxy-5-bromo-phenyl)-7,7-dimethyl-4,6,7,8-tetrahydro-1H,3H-quinazoline-2,5-dione (4b). White solid, m. p. 352–355 °C, IR (KBr): νmax 3430, 3105, 1616, 1376, 1029; 1H NMR (400 MHz, DMSO-d6): δ 0.97 (s, 3H), 1.04 (s, 3H), 2.03 (d, J = 12 Hz, 1H), 2.25 (d, J = 12 Hz, 1H), 2.33 (d, J = 8 Hz, 2H), 5.03 (s, 1H), 6.95 (d, J = 8 Hz, 1H), 7.03 (s, 1H), 7.28 (d, J = 8 Hz, 1H), 7.65 (s, OH), 7.75 (s, NH), 10.62 (s, NH); 13C NMR (100 MHz, DMSO-d6): δ 27.48, 29.10, 32.72, 33.55, 50.30, 53.25, 109.08, 116.30, 126.85, 130.42, 135.22, 142.98, 147.37, 150.80, 152.59, 193.08. Anal. calcd for C16H17BrN2O3: C, 52.62; H, 4.69; N, 7.67; found: C, 52.53; H, 4.55; N, 7.60.
4-(3-Nitro-phenyl)-7,7-dimethyl-4,6,7,8-tetrahydro-1H,3H-quinazoline-2,5-dione (4c). White solid, m. p. 303–305 °C, IR (KBr): νmax 3415, 3266, 3105, 2954, 1704, 1373, 1153; 1H NMR (400 MHz, DMSO-d6): δ 0.88 (s, 3H), 1.02 (s, 3H), 2.04 (d, J = 12 Hz, 1H), 2.21 (d, J = 12 Hz, 1H), 2.31 (s, 1H), 2.41 (s, 1H), 5.31 (s, 1H), 7.63 (d, J = 8 Hz, 1H), 7.70 (d, J = 8 Hz, 1H), 7.94 (s, 1H), 8.11 (d, J = 8 Hz, 1H), 8.06 (s, NH), 9.65 (s, NH); 13C NMR (100 MHz, DMSO-d6): δ 27.42, 29.11, 32.73, 33.54, 50.38, 53.20, 110.05, 116.20, 126.80, 130.45, 135.25, 140.92, 147.33, 151.82, 152.09, 192.12. Anal. calcd for C16H17N3O4: C, 60.94; H, 5.43; N, 13.33; found: C, 60.91; H, 5.37; N, 13.30.
4-(4-Hydroxy-phenyl)-7,7-dimethyl-4,6,7,8-tetrahydro-1H,3H-quinazoline-2,5-dione (4d). White solid, m. p. 274–276 °C, IR (KBr): νmax 3247, 3411, 2960, 1650, 1374; 1H NMR (400 MHz, DMSO-d6): δ 0.89 (s, 3H), 1.00 (s, 3H), 2.00 (d, J = 16 Hz, 1H), 2.17 (d, J = 16 Hz, 1H), 2.24 (d, J = 16 Hz, 1H), 2.38 (d, J = 16 Hz, 1H), 5.02 (s, 1H), 6.66 (d, J = 8 Hz, 2H), 7.01 (d, J = 8 Hz, 2H), 7.65 (s, OH), 9.33 (s, NH), 9.39 (s, NH); 13C NMR (100 MHz, DMSO-d6): δ 27.41, 29.19, 32.79, 33.46, 50.32, 53.22, 110.08, 116.22, 130.44, 141.90, 147.32, 151.85, 152.12, 193.10. Anal. calcd for C16H18N2O3: C, 67.12; H, 6.34; N, 9.78; found: C, 67.08; H, 6.31; N, 9.70.
4-(4-Nitro-phenyl)-7,7-dimethyl-4,6,7,8-tetrahydro-1H,3H-quinazoline-2,5-dione (4e). White solid, m. p. 308–310 °C, IR (KBr): νmax 3327, 3245, 2961, 1671, 1525; 1H NMR (400 MHz, DMSO-d6): δ 0.86 (s, 3H), 1.02 (s, 3H), 2.03 (d, J = 16 Hz, 1H), 2.21 (d, J = 16 Hz, 1H), 2.28 (d, J = 16 Hz, 1H), 2.43 (d, J = 16 Hz, 1H), 5.30 (s, 1H), 7.51 (d, J = 8 Hz, 2H), 7.93 (s, NH), 8.21 (d, J = 8 Hz, 2H), 9.65 (s, NH); 13C NMR (100 MHz, DMSO-d6): δ 27.49, 29.18, 32.78, 33.56, 50.32, 53.23, 110.05, 116.24, 130.25, 141.82, 147.22, 151.65, 152.15, 193.08. Anal. calcd for C16H17N3O4: C, 60.94; H, 5.43; N, 13.33; found: C, 60.90; H, 5.35; N, 13.25.
4-(Phenyl)-7,7-dimethyl-4,6,7,8-tetrahydro-1H,3H-quinazoline-2,5-dione (4f). White solid, m. p. 288–290 °C, IR (KBr): νmax 3259, 2957, 1673, 1445; 1H NMR (400 MHz, DMSO-d6): δ 0.88 (s, 3H), 1.01 (s, 3H), 2.02 (d, J = 16 Hz, 1H), 2.19 (d, J = 16 Hz, 1H), 2.26 (d, J = 16 Hz, 1H), 2.40 (d, J = 20 Hz, 1H), 5.14 (s, 1H), 7.22 (t, J = 8 Hz, 3H), 7.30 (t, J = 8 Hz, 2H), 7.77 (s, NH), 9.47 (s, NH); 13C NMR (100 MHz, DMSO-d6): δ 27.40, 29.28, 32.68, 33.52, 50.31, 53.23, 110.08, 116.24, 130.23, 140.88, 147.25, 151.68, 152.25, 193.07. Anal. calcd for C16H18N2O2: C, 71.09; H, 6.71; N, 10.36; found: C, 71.02; H, 6.65; N, 10.31.
4-(2,4-Dichloro-phenyl)-7,7-dimethyl-4,6,7,8-tetrahydro-1H,3H-quinazoline-2,5-dione (4g). White solid, m. p. 270–272 °C, IR (KBr): νmax: 3329, 3102, 2960, 1700, 1375, 1230; 1H NMR (400 MHz, DMSO-d6): δ 0.93 (s, 3H), 1.02 (s, 3H), 1.98 (d, J = 16 Hz, 1H), 2.16 (d, J = 16 Hz, 1H), 2.31 (d, J = 16 HZ, 1H), 2.43 (d, J = 16 Hz, 1H), 5.35 (s, 1H), 7.29 (d, J = 4 Hz, 1H), 7.39–7.41 (d, J = 4 Hz, 1H), 7.54 (d, J = 4 Hz, 1H), 7.75 (s, NH), 9.58 (s, NH); 13C NMR (100 MHz, DMSO-d6): δ 27.35, 29.25, 32.65, 33.58, 50.30, 53.22, 109.08, 116.31, 126.85, 142.92, 147.36, 151.81, 152.05, 193.03. Anal. calcd for C16H16Cl2N2O2: C, 56.65; H, 4.75; N, 8.26; found: C, 56.58; H, 4.71; N, 8.22.
4-(4-Isopropyl-phenyl)-7,7-dimethyl-4,6,7,8-tetrahydro-1H,3H-quinazoline-2,5-dione (4h). White solid, m. p. 299–301 °C, IR (KBr): νmax: 3254, 2960, 1678, 1374, 1233; 1H NMR (400 MHz, DMSO-d6): δ 0.91 (s, 3H), 1.01 (s, 3H), 1.15 (s, 3H), 1.16 (s, 3H), 2.02 (d, J = 16 Hz, 1H), 2.10 (m, 1H), 2.18 (d, J = 16 Hz, 1H), 2.27 (d, J = 16 Hz, 1H), 2.40 (d, J = 20 Hz, 1H), 5.09 (s, 1H), 7.12–7.17 (m, 4H), 7.71 (s, NH), 9.44 (s, NH); 13C NMR (100 MHz, DMSO-d6): δ 24.33, 24.37, 27.49, 29.18, 32.78, 33.56, 50.32, 52.22, 107.89, 126.65, 126.72, 142.60, 147.71, 151.35, 152.79, 193.34. Anal. calcd for C19H24N2O2: C, 73.05; H, 7.74; N, 8.97; found: C, 73.01; H, 7.65; N, 8.90.
4-(2-Chloro-phenyl)-7,7-dimethyl-4,6,7,8-tetrahydro-1H,3H-quinazoline-2,5-dione (4i). White solid, m. p. 292–294 °C, IR (KBr): νmax 3260, 3094, 2956, 1705, 1377; 1H NMR (400 MHz, DMSO-d6): δ 0.95 (s, 3H), 1.02 (s, 3H), 1.97 (d, J = 16 Hz, 1H), 2.16 (d, J = 16 Hz, 1H), 2.31 (d, J = 20 Hz, 1H), 2.43 (d, J = 16 Hz, 1H), 5.55 (s, 1H), 7.23–7.31 (m, 3H), 7.38 (d, J = 8 Hz, 1H), 7.71 (s, NH), 9.54 (s, NH); 13C NMR (100 MHz, DMSO-d6): δ 27.38, 29.12, 32.75, 33.53, 50.35, 53.20, 110.06, 116.20, 126.82, 130.45, 135.28, 140.96, 147.35, 151.82, 152.09, 193.13. Anal. calcd for C16H17ClN2O2: C, 63.05; H, 5.62; N, 9.19; found: C, 63.01; H, 5.55; N, 9.12.
4-(4-Methoxy-phenyl)-7,7-dimethyl-4,6,7,8-tetrahydro-1H,3H-quinazoline-2,5-dione (4j). White solid, m. p. 287–280 °C, IR (KBr): νmax 3318, 3247, 2954, 1674, 1377, 1237; 1H NMR (400 MHz, DMSO-d6): δ 0.89 (s, 3H), 1.00 (s, 3H), 2.00 (d, J = 16 Hz, 1H), 2.18 (d, J = 16 Hz, 1H), 2.24 (d, J = 20 Hz, 1H), 2.39 (d, J = 20 Hz, 1H), 3.70 (s, 3H), 5.07 (s, 1H), 6.85 (d, J = 8 Hz, 2H), 7.13 (d, J = 8 Hz, 2H), 7.70 (s, NH), 9.43 (s, NH); 13C NMR (100 MHz, DMSO-d6): δ 27.75, 29.15, 32.75, 33.54, 50.31, 53.20, 55.35, 109.08, 116.36, 126.75, 142.92, 147.36, 151.80, 152.02, 193.01. Anal. calcd for C17H20N2O3: C, 67.98; H, 6.71; N, 9.33; found: C, 67.92; H, 6.65; N, 9.25.
4-(4-Methyl-phenyl)-7,7-dimethyl-4,6,7,8-tetrahydro-1H,3H-quinazoline-2,5-dione (4k). White solid, m. p. 298–300 °C, IR (KBr): νmax 3322, 3252, 2959, 1675, 1375, 1233; 1H NMR (400 MHz, DMSO-d6): δ 0.88 (s, 3H), 1.00 (s, 3H), 2.00 (d, J = 16 Hz, 1H), 2.18 (d, J = 16 Hz, 1H), 2.24 (s, 3H), 2.24 (d, J = 16 Hz, 1H), 2.39 (d, J = 20 Hz, 1H), 5.09 (s, 1H), 7.10 (m, 4H), 7.72 (s, NH), 9.44 (s, NH); 13C NMR (100 MHz, DMSO-d6): δ 24.58, 27.35, 29.05, 32.62, 33.52, 50.35, 53.28, 108.89, 116.14, 126.47, 142.94, 147.32, 1531.81, 152.19, 193.12. Anal. calcd for C17H20N2O2: C, 71.81; H, 7.09; N, 9.85; found: C, 71.75; H, 7.02; N, 9.80.
4-(3-Chloro-phenyl)-7,7-dimethyl-4,6,7,8-tetrahydro-1H,3H-quinazoline-2,5-dione (4l). White solid, m. p. 296–298 °C, IR (KBr): νmax 3478, 3360, 3295, 1668, 1371, 1142; 1H NMR (400 MHz, DMSO-d6): δ 0.88 (s, 3H), 1.02 (s, 3H), 2.07 (d, J = 16 Hz, 1H), 2.23 (d, J = 16 Hz, 1H), 2.40 (d, J = 12 Hz, 2H), 5.18 (s, 1H), 7.22 (d, J = 8 Hz, 2H), 7.42 (d, J = 8 Hz, 2H), 9.68 (s, NH), 10.61 (s, NH); 13C NMR (100 MHz, DMSO-d6): δ 27.55, 29.19, 32.76, 33.53, 50.32, 53.19, 111.05, 117.31, 126.82, 130.45, 134.20, 140.96, 147.35, 151.82, 153.19, 192.15. Anal. calcd for C16H17ClN2O2: C, 63.05; H, 5.62; N, 9.19; found: C, 63.01; H, 5.57; N, 9.10.
4. Conclusion
In this study, we described the preparation of quinazolinones using Ag NPs as a superior catalyst under reflux conditions. Ag NPs were prepared using Echium amoenum extract in a green synthesis. The catalyst was characterized by UV-VIS, FT-IR, XRD, SEM and EDS. The current method provides obvious benefits, including environmental friendliness, short reaction time, low catalyst loading, reusability of the catalyst and simple work-up procedure. The catalyst was stable and could tolerate the MCR conditions. The present catalytic procedure is applicable to a wide variety of substrates for the synthesis of a diverse library of quinazolinones.
Conflicts of interest
There are no conflicts to declare.
Acknowledgements
The authors are grateful to the University of Kashan for supporting this work under grant no. 159148/XII.
References
- A. A. Al-Amiery, A. A. H. Kadhum, M. Shamel, M. Satar, Y. Khalid and A. B. Mohamad, Med. Chem. Res., 2014, 23, 236–242 CrossRef CAS.
- Z. Xu, Y. Zhang, H. Fu, H. Zhong, K. Hong and W. Zhu, Bioorg. Med. Chem. Lett., 2011, 21, 4005–4007 CrossRef CAS PubMed.
- S. Gatadi, T. V. Lakshmi and S. Nanduri, Eur. J. Med. Chem., 2019, 170, 157–172 CrossRef CAS PubMed.
- A. A. Noser, M. El-Naggar, T. Donia and A. H. Abdelmonsef, Molecules, 2020, 25, 4780–4800 CrossRef CAS PubMed.
- A. Kumar, S. Sharma, K. Bajaj, S. Sharma, H. Panwar, T. Singh and V. K. Srivastava, Bioorg. Med. Chem., 2003, 11, 5293–5299 CrossRef CAS.
- A. A. M. Abdel-Aziz, L. A. Abou-Zeid, K. E. H. ElTahir, M. A. Mohamed, M. A. A. El-Enin and A. S. El-Azab, Bioorg. Med. Chem., 2016, 24, 3818–3828 CrossRef CAS PubMed.
- J. M. Khurana and S. Kumar, Monatsh. Chem., 2010, 141, 561–564 CrossRef CAS.
- H. Kefayati, K. Rad-Moghadam, M. Zamani and S. Hosseyni, Lett. Org. Chem., 2010, 7, 277–282 CrossRef CAS.
- R. Mekala, R. Akula, R. R. Kamaraju, C. K. Bannoth, S. Regati and J. Sarva, Synlett, 2014, 25, 821–826 CrossRef CAS.
- M. Soheilizad, S. Soroosh and R. Pashazadeh, Monatsh. Chem., 2017, 148, 739–743 CrossRef CAS.
- M. Sharma, S. Pandey, K. Chauhan, D. Sharma, B. Kumar and P. M. S. Chauhan, J. Org. Chem., 2012, 77, 929–937 CrossRef CAS.
- L. M. Wang, L. Hu, J. H. Shao, J. Yu and L. Zhang, J. Fluorine Chem., 2008, 129, 1139–1145 CrossRef CAS.
- B. Karami, S. Khodabakhshi and S. Karami, Croat. Chem. Acta, 2014, 87, 23–27 CrossRef CAS.
- C. Larksarp and H. Alper, J. Org. Chem., 2000, 65, 2773–2777 CrossRef CAS PubMed.
- A. R. Khosropour, I. Mohammadpoor-Baltorka and H. Ghorbankhanib, Tetrahedron Lett., 2006, 47, 3561–3564 CrossRef CAS.
- K. Alorku, M. Manoj and A. Yuan, RSC Adv., 2020, 10, 40923–40939 RSC.
- C. Vanlalveni, S. Lallianrawna, A. Biswas, M. Selvaraj, B. Changmai and S. L. Rokhum, RSC Adv., 2021, 11, 2804–2837 RSC.
- A. Ahmad, Y. Wei, F. Syed, M. Imran, Z. U. H. Khan, K. Tahir, A. U. Khan, M. Raza, Q. Khan and Q. Yuan, RSC Adv., 2015, 5, 99364–99377 RSC.
- F. Qazi, Z. Hussain and M. N. Tahir, RSC Adv., 2016, 6, 60277–60286 RSC.
- M. N. Alam, N. Roy, D. Mandal and N. A. Begum, RSC Adv., 2013, 3, 11935–11956 RSC.
- F. Liu, J. Zhan, Y. Sun and X. Jing, Chin. J. Org. Chem., 2021, 41, 2099–2104 CrossRef.
- R. Xu, D. Wang, J. Zhang and Y. Li, Chem.–Asian J., 2006, 1, 888–893 CrossRef CAS PubMed.
- K. D. Bhatte, P. J. Tambade, K. P. Dhake and B. M. Bhanage, Catal. Commun., 2010, 11, 1233–1237 CrossRef CAS.
- P. Mani, C. Sharma, S. Kumar and S. K. Awasthi, J. Mol. Catal. A: Chem., 2014, 392, 150–156 CrossRef CAS.
- T. Mitsudome, S. Arita, H. Mori, T. Mizugaki, K. Jitsukawa and K. Kaneda, Angew. Chem., 2008, 120, 8056–8058 CrossRef.
- X. Zhou, Y. Lu, L. L. Zhai, Y. Zhao, Q. Liu and W. Y. Sun, RSC Adv., 2013, 3, 1732–1734 RSC.
- L. Moradi and M. Tadayon, J. Saudi Chem. Soc., 2018, 22, 66–75 CrossRef CAS.
- B. Sadeghi, Z. Nasirian and A. Hassanabadi, J. Chem. Res., 2012, 36, 391–392 CrossRef CAS.
|
This journal is © The Royal Society of Chemistry 2022 |
Click here to see how this site uses Cookies. View our privacy policy here.