DOI:
10.1039/D2RA02185D
(Paper)
RSC Adv., 2022,
12, 21129-21134
A turn-on fluorescent probe with high selectivity for Hg2+ and its applications in living cells†
Received
5th April 2022
, Accepted 8th July 2022
First published on 1st August 2022
Abstract
A novel fluorescent probe L with a rhodamine B lactam structure modified with 3-methyl-2-thiophenecarboxaldehyde has been prepared based on the thiophilicity of Hg2+. The probe L exhibits a unique response with an “off–on-type” mode towards Hg2+ among other biologically relevant metal cations. The limit of detection (LOD) for probe L is 1.5 ppb. In addition, in the presence of Hg2+, the probe L shows a colorimetric response from colorless to pink. The recognition behavior of probe L towards Hg2+ has been investigated by 1H NMR titration experiments, Job's plot, and MS and IR analyses. As a result, the ligation between the probe and Hg2+ leads to the scission of the spirolactam bond of free L and the restoration of its conjugated structure, which can give rise to the fluorescence enhancement of the probe L. Besides, it also can be used as a sensitivity probe in living cells for Hg2+ sensing, which can meet various needs in genetic and environmental samples.
Introduction
Mercury is one of the highly toxic heavy metal element for the environment and human health, which is generated by numerous sources such as coal plants, gold production, and mercury lamps.1–3 Because of the strong affinity for the thiol groups of proteins, Hg2+ has been linked to a number of neurological issues such as brain damage and kidney damage.4–8 Since Hg2+ ions are highly toxic and easily penetrate the blood–brain barrier, the EPA has set a limit of 2 × 10−9 M in drinking water9 in the U.S. Therefore, it is critical to find a reliable technique to detect and monitor small amounts of Hg2+ ions in genetic and environmental samples.
Numerous approaches have been applied to detect Hg2+ ions including atomic absorption spectroscopy, spectrophotometry, and voltammetry.10,11 These approaches provide respectable detection limits, whereas the majority of them involve the need for an advanced and expensive instrument as well as lengthy pretreatment operations, which are not suitable for real-time monitoring.12 Fluorescence-based approaches have been gradually developed and become essential instruments in metal ion detection owing to their advantages of straightforward operation, high sensitivity and selectivity.13–15 The development of fluorescent indicators for Hg2+ ions has received considerable interest. There are a number of fluorescence probes for Hg2+. However, some reported probes have several limitations such as exhibited fluorescent “on–off” behavior owing to the intrinsic spectral property of Hg2+,16–18 solubility in organic solvents,19 non-colorimetric20 and difficult synthesis.21–23 Therefore, the development of a colorimetric probe with a simple procedure, low detection limit, and “off–on” behavior in an aqueous solution is still necessary. The rhodamine B lactam with outstanding spectroscopic properties could be modified with 3-methyl-2-thiophenecarboxaldehyde based on the thiophilicity of Hg2+. On the one hand, the probe is formed by the condensation of the aldehyde group with the amino group in the rhodamine lactam; on the other hand, the sulfur atom also potentially acts as a coordinating atom for Hg2+. Therefore, it is expected that the synthesized probe L could be a potential coordinator for Hg2+.
Herein, a new colorimetric probe (E)-3′,6′-bis(diethylamino)-2-(((3-methylthiophen-2-yl)methylene)amino)spiro[isoindoline-1,9′-xanthen]-3-one has been designed and synthesised. It shows a significant fluorescence behaviour and unique response for Hg2+ among other biologically relevant metal cations. In comparison with some reported probe of Hg2+, the colorimetric probe L is easily synthesized, possessing low detection limits and “off–on” behavior in CH3OH–H2O (4
:
1, v/v, Tris–HCl buffer pH = 8.0). Therefore, it has potential applications in various practical samples as a visible probe for Hg2+.
Experimental
Materials
Hydrazine hydrate, 3-methyl-2-thiophenecarboxaldehyde and rhodamine B were used. All chemicals and solvents were purchased commercially. Stock solutions (1 × 10−5 M) of the probe were produced by dissolving 5.65 mg compounds L in the mixed solvent (CH3OH–H2O = 4
:
1, v/v, pH = 8.0). Stock solutions (1.0 × 10−2 M) of Na+, Ag+, Al3+, Ba2+, Ca2+, Cd2+, Cr3+, Cu2+, Co2+, Fe3+, Hg2+, K+, Mg2+, Mn2+, Ni2+, Pb2+, Sr2+ and Zn2+ were made by dissolving their nitrate salts in the above mixed solvent.
Instruments
1H NMR and 13C NMR signals were collected on a Bruker spectrometer using TMS as a standard. Absorption spectra were recorded on a U-3900 spectrophotometer at room temperature. Fluorescent emission was obtained using a Hitachi F-4600 spectrophotometer at room temperature. MS spectra were obtained on an Agilent 6546 Q-TOF LC/MS spectrometer. Fluorescence microscopy images were collected via laser scanning confocal microscopy. IR spectra were recorded in an anhydrous methanol solution using a Bruker TENSOR27.
Measurement
Test solutions were prepared by pouring 2 mL solution of L (10 μM) into the cuvettes with each metal ion stock solutions (20 μL, 10 mM). The prepared samples were shaken for 5 s at 25 °C. The excitation wavelength used for all fluorescence experiments was 490 nm.
Synthesis
The probe was successfully synthesized in two easy steps, as seen in Scheme 1.
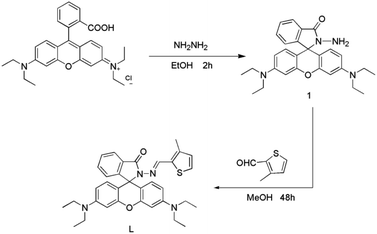 |
| Scheme 1 Synthetic route to probe L. | |
Synthesis of compound 1. Compound 1 was synthesized by a previously reported.24 It was prepared by refluxing rhodamine B (hydrochloride salt, 1.92 g, 4 mM) and excess hydrazine hydrate (85%, 5 mL) in 30 mL ethanol for 2 h at 25 °C. Pinkish solids (1.81 g) has been obtained. Yield: 95%. 1H NMR (500 MHz, DMSO) δ 7.84–7.72 (m, 1H), 7.47 (dd, J = 5.9, 3.2 Hz, 2H), 6.99 (d, J = 6.2 Hz, 1H), 6.37 (s, 2H), 6.32 (d, J = 8.8 Hz, 4H), 4.28 (s, 2H), 3.32 (dd, J = 13.8, 6.8 Hz, 8H), 1.08 (t, J = 6.9 Hz, 12H) (Fig. S1†).
Synthesis of probe L. Compound 1 (1 mM) was dissolved in 30 mL absolute methanol, and then added 3-methyl-2-thiophenecarboxaldehyde (1 mM) under stirring condition. In the reaction system, 2–3 drops of acetic acid were added. A light pink precipitate was formed after refluxed 48 h at 80 °C. Filtered the precipitates were washed with ethanol three times, and pinkish solids (0.49 g, 86%) has been obtained. 1H NMR (500 MHz, DMSO) δ 8.59 (s, 1H), 7.90 (d, J = 7.3 Hz, 1H), 7.58 (dt, J = 21.4, 7.2 Hz, 2H), 7.46 (d, J = 4.9 Hz, 1H), 7.08 (d, J = 7.4 Hz, 1H), 6.84 (d, J = 4.9 Hz, 1H), 6.43 (s, 2H), 6.39 (d, J = 8.8 Hz, 2H), 6.35 (dd, J = 8.9, 1.6 Hz, 2H), 3.32 (dd, J = 14.4, 7.1 Hz, 8H), 2.05 (s, 3H), 1.06 (t, J = 6.9 Hz, 12H) (Fig. S2†). 13C NMR (126 MHz, DMSO) δ 163.87 (s), 152.98 (s), 149.02 (s), 140.42 (s), 134.33 (s), 133.31 (s), 131.28 (s), 129.25 (s), 129.06 (s), 128.57 (s), 128.28 (s), 124.26 (s), 123.35 (s), 108.70 (s), 105.28 (s), 97.59 (s), 65.44 (s), 44.16 (s), 13.61 (s), 12.82 (s) (Fig. S3†). MS(ESI): m/z [M + H]+: calcd for C34H36N4O2S: 565.26, found: 565.40 (Fig. S4†).
Result and discussion
Absorption and fluorescence selectivity of probe L towards Hg2+
The absorption responses of probe L to various cations (Na+, Ag+, Al3+, Ba2+, Ca2+, Cd2+, Cr3+, Cu2+, Co2+, Fe3+, Hg2+, K+, Mg2+, Mn2+, Ni2+, Pb2+, Sr2+ and Zn2+) were examined. As shown in Fig. 1, the colorless probe L displayed weak absorptions at 251 nm and 315 nm, which belong to the thiophene group. In addition, only weak changes were observed when other cations were added. In the presence of Hg2+ ions, the peaks at 251 nm and 315 nm of receptor L significantly increased. Moreover, a new peak at 550 nm was discovered. Simultaneously, the color of the solution changed from colorless to pink. The new absorption band represents the scission of the spirolactam bond, and the restoring conjugated structure of probe L. These results indicated that probe L has a high selectivity to Hg2+ ions and could act as a colorimetric probe for Hg2+.
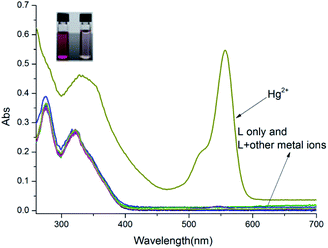 |
| Fig. 1 The UV-vis absorption spectra changes of L (10 μM) in CH3OH–H2O (4 : 1, v/v, Tris–HCl buffer pH = 8.0) upon being treated with 10 equiv. metal ions. | |
In addition, the selectivity of probe L for Hg2+ ions was determined via fluorescence spectroscopy. As shown in Fig. 2, the free probe L exhibited a feeble fluorescence emission at 582 nm upon excitation at 490 nm. In comparison, the solution of probe L has a strong emission band in the presence of the Hg2+ ions at 582 nm but other cations did not cause any noticeable emission at 582 nm. These phenomena agree with the results of the absorption spectrum, suggesting that probe L has a high sensitivity to Hg2+ because a complex was formed between L and Hg2+ ions.
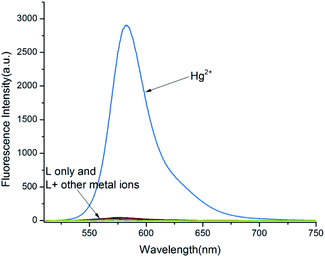 |
| Fig. 2 Fluorescence responses of probe L upon treated with 10 equiv. metal ions in CH3OH–H2O (4 : 1, v/v, Tris–HCl buffer pH = 8.0). | |
Competition experiments
To study the selectivity of probe L towards Hg2+ over other biologically and environment-relevant cations, competitive experiments were conducted, as described in Fig. 3. The Hg2+-induced emission of L was almost unaffected by other metal cations. These findings indicate that L showed a strong selectivity towards Hg2+ over other physiologically significant and environmentally metal ions.
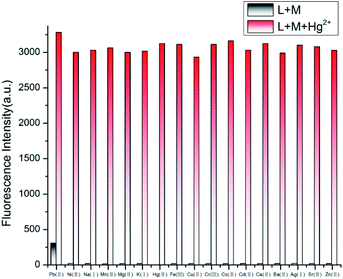 |
| Fig. 3 The fluorescence intensity of L with Hg2+ in the presence of various competitive metal ions in CH3OH–H2O (4 : 1, v/v, Tris–HCl buffer pH = 8.0). | |
Fluorescence titration of Hg2+ with probe L
To further investigate the properties of probe L, fluorescence titration experiments were conducted, as shown in Fig. 4. The emission of receptor L significantly enhanced at 582 nm with the level of Hg2+ increased, and the fluorescence emission was approximately increased to 55 fold when Hg2+ reached to 1.3 × 10−5 M.
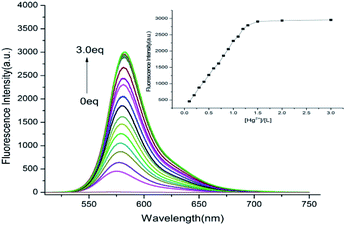 |
| Fig. 4 Fluorescence emission spectra of probe L in the presence of various concentrations of Hg2+ (0–3 equiv.) in CH3OH–H2O (4 : 1, v/v, Tris–HCl buffer pH = 8.0). | |
The association constant (K) for the L–Hg2+ complex was determined based on the Benesi–Hildebrand equation.25 From Fig. S5(a),† K was determined to be 5.4 × 104 M−1. As demonstrated in Fig. S5(b),† working curves were established from titration data, which showed a reliable linear connection at the range of 0–13 μM for the concentration of Hg2+. The LOD was 1.5 × 10−9 M, which is lower than the limit quantity of Hg2+ ion in drinking water approved by the EPA.
Binding stoichiometry between probe L and Hg2+
The stoichiometry of Hg2+ and probe L has been established by holding the quantity of the L and Hg2+ at 10 μM and adjusting the molar ratio of [Hg2+]/[L + Hg2+] from 0 to 1. As showed in Fig. 5, the highest fluorescence value was obtained when the molar fraction approached 0.5. This phenomenon reveals a 1
:
1 stoichiometry for the Hg2+ and probe L. To further clarify the stoichiometry between probe L and Hg2+, the MS analysis was carried out. When existence of 1.0 equiv. Hg2+, a main peak at m/z = 827.259[L + Hg2+ + NO3− + H+] have been shown (Fig. S6†). It can be ascribed to the 1
:
1 L–Hg2+ complex, which agree with the results of the Job's plot. Simultaneously, a nitrate ion participates in the coordination of the Hg2+.
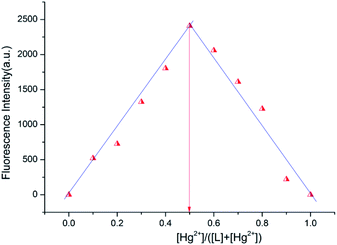 |
| Fig. 5 Job's plot for determining the stoichiometry between L and Hg2+ ion in CH3OH–H2O (4 : 1, v/v, Tris–HCl buffer pH = 8.0). | |
The reversibility and regeneration of probe L toward Hg2+
By introducing the EDTA aqueous solution to the L–Hg2+ complex, the chemical reversibility and regeneration behaviors of L were examined. Probe L treated with Hg2+ showed a solid emission at 582 nm. After 20 equiv. EDTA was added, the fluorescence emission at 582 nm instantly dropped. The results show that EDTA is a better chelating agent than L. However, when the excessive Hg2+ (60 equiv.) were dropped to the above mixture, the emission at 582 nm appeared again. As described in Fig. 6, these findings show that the Hg2+ binding to L is chemically reversible and could be used for practical applications.
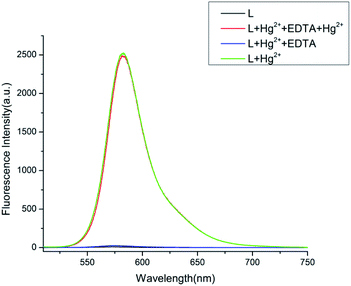 |
| Fig. 6 Effect on fluorescence response of free L and L–Hg2+ complex in CH3OH–H2O (4 : 1, v/v, Tris–HCl buffer pH = 8.0) upon the addition of EDTA. | |
pH effect on free L and L–Hg2+ complex
The impact of pH for the emission bands was investigated (Fig. 7). The emission intensity at 582 nm of receptor L decreased with the rise in the pH value when pH was less than 7.0. This phenomenon might be attributed to the spirolactam ring opening of probe L, which restored its conjugated structure as induced by the high concentration of H+.25,26 Once the pH was greater than 7.0, the emission of probe L disappeared immediately. It might be attributed to the presence of the spirolactam ring, which destroyed its conjugated structure for probe L. However, for the L–Hg2+ complex, the emission at 582 nm gradually enhanced from the pH 3 to 5, and almost did not alter at a pH of 6–8, which were probably attributed to the restoration of the conjugated structure for the probe L induced by Hg2+. These phenomena showed that probe L possesses a significant fluorescence emission toward Hg2+ in the range of 7.0–8.0, which could be potentially applied in some physiologically samples.
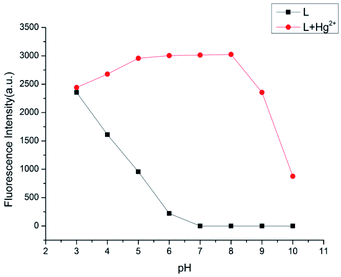 |
| Fig. 7 Fluorescence intensity of probe L (10 μM) in the absence and presence of Hg2+ (10 equiv.) at different pH. | |
Complexation mechanism for L–Hg2+
To understand the complexation behavior of receptor L with Hg2+, 1H NMR titration experiments were carried out in DMSO-d6. The significant spectral differences upon the addition of Hg2+ aer described in Fig. 8. In the presence of 1.0 equiv. Hg2+, the protons of the imine (Hc′) and thiophene ring (Ha′, Hb′) shifted downfield from 8.594 ppm to 8.672 ppm, 7.456 ppm to 7.467 ppm and 7.075 ppm to 7.087 ppm, respectively. This phenomenon suggested that the nitrogen atom in the imine moiety and the sulfur atom in the thiophene moiety might get involved in Hg2+ coordination. In addition, the proton of the benzene (Hd′) was shifted downfield from 7.888 ppm to 7.903 ppm, and the protons in the benzene (e′, f′, and g′) were almost unaffected. This phenomenon indicated that the oxygen atom in the acyl hydrazone moiety might be involved in Hg2+ coordination, which is a significant factor for opening the spirolactam bond of the probe L and generating the xanthene moiety, which is in the conjugated state. To further clarify the sensing behavior of receptor L towards Hg2+, IR analysis was carried out. Compared with free L, when 1.0 equiv. Hg2+was added, the IR peak (Fig. S7†) for the C
O amide bond of probe L at 1684 cm−1 shifted to 1632 cm−1, which was attributed to the oxygen atom of the acyl hydrazone moiety binding to Hg2+; moreover, Hg2+ induced the spirolactam ring opening of probe L.
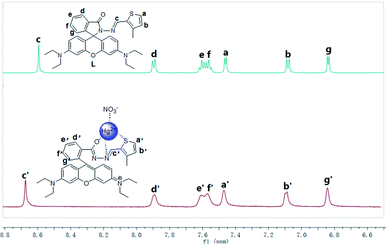 |
| Fig. 8 1H NMR titration of the probe L in the presence of Hg2+ (1 equiv.). | |
For free probe L, the presence of the spirolactam ring destroys its conjugated structure. When excited with 520 nm light, the molecule has no fluorescence emission. Based on 1H NMR titration, Job's plot, MS and IR analysis, a 1
:
1 complex of L and Hg2+ was formed. Besides, the nitrogen atom in the imine moiety, the sulfur atom in the thiophene moiety, the oxygen atom in the acyl hydrazone moiety and a nitrate ion in the solution might get involved in Hg2+ coordination. While coordinating with the above atoms, Hg2+ induces the spirolactam ring opening of probe L to restore its conjugated structure. Once excited with 520 nm light, the L–Hg2+ complex has strong fluorescence emission. Protons can also induce the spirolactam ring opening of probe L when the pH is less than 7. The proposed sensing mechanism is shown in Scheme 2.
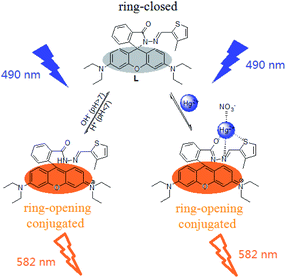 |
| Scheme 2 Proposed complexation mechanism of probe L and Hg2+ ions. | |
Cell imaging in living biological
By using MTT assay, the cytotoxicity of the solution, Hg(NO3)2, probe L and the L–Hg complex for MCF-7 cells were investigated following the reported method.27 The results are shown in Fig. S8.† The viabilities of MCF-7 cells were more than 85% upon treatment with 5% CH3OH–H2O (4
:
1) solution and 10 μM Hg(NO3)2 in CH3OH–H2O (4
:
1) solution for 12 h, respectively. The results indicate that the solution of 5% CH3OH–H2O (4
:
1) and 10 μM Hg(NO3)2 has little effect on the cell activity. When incubation with 10–70 μM probe L and L–Hg complex for 12 h respectively, cell viabilities were greater than 80% even at a concentration of 70 μM, which indicated the satisfactory biocompatibility of the probe L and L–Hg2+ at 10–70 μM. Besides, the determination of Hg2+ ions in MCF-7 cells was used to assess the potential biological application of L. The cells were cultured by the existed procedure.28 As described in Fig. 9a, a red fluorescence was observed after the cells were treated with 10 μM Hg2+ ions. However, cells only supplementing 10 μM probe L showed no fluorescence (Fig. 9b). These findings indicated that L may be used as a Hg2+ probe in biological systems.
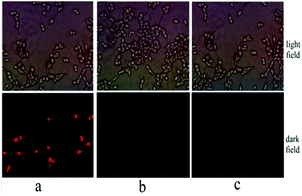 |
| Fig. 9 Fluorescence images in MCF-7 cells. MCF-7 cells incubated with the 10 μM L–Hg2+ ions (a), MCF-7 cells incubated with the 10 μM probe L (b) and free MCF-7 cells (c). | |
Conclusions
In conclusion, a rhodamine-based probe L has been developed. The probe shows a “turn-on” response and clear colorimetric signal toward Hg2+ ions, which could be applied as an indicator. The spectral responses of L toward Hg2+ were robust over competitive ions. The binding mechanism of L–Hg2+ was studied via 1H NMR titration, Job's plot, and MS and IR analyses, which show a 1
:
1 complex of L and Hg2+ and the spirolactam bond was open when the Hg2+ ions were added. Furthermore, L can be utilized as a sensing material to image Hg2+ ions in MCF-7 cells.
Conflicts of interest
There are no conflicts to declare.
Acknowledgements
This work was supported by the Scientific Research Project of Ordos Institute of Technology (Grant No. KYYB2021014).
Notes and references
- L. Feng, Y. Deng, X. Wang and M. Liu, Sens. Actuators, B, 2017, 245, 441–447 CrossRef CAS.
- Y. Ding, Y. Pan and Y. Han, Ind. Eng. Chem. Res., 2019, 58, 7786–7793 CrossRef CAS.
- M. F. Wolfe, S. Schwarzbach and R. A. Sulaiman, Environ. Toxicol. Chem., 1998, 17, 146–160 CrossRef CAS.
- R. Singh, S. K. Rai, M. K. Tiwari, A. Mishra, A. K. Tewari, P. C. Mishra and R. K. Singh, Chem. Phys. Lett., 2017, 676, 39–45 CrossRef CAS.
- Q. Zhu, L. H. Liu, Y. P. Xing and X. H. Zhou, J. Hazard. Mater., 2018, 355, 50–55 CrossRef CAS PubMed.
- Q. Meng, C. He, W. Su, X. Zhang and C. Duan, Sens. Actuators, B, 2012, 174, 312–317 CrossRef CAS.
- E. K. Silbergeld, I. A. Silva and J. F. Nyland, Toxicol. Appl. Pharmacol., 2005, 207, 282–292 CrossRef PubMed.
- Q. Duan, H. Zhu, C. Liu, R. Yuan, Z. Fang, Z. Wang, P. Jia, Z. Li, W. Sheng and B. Zhu, Analyst, 2019, 144, 1426–1432 RSC.
- Mercury Update Impact on Fish Advisories, EPA Fact Sheet EPA-823-F-01-011, EPA Off., Washington, DC, 2001 Search PubMed.
- H. Cheng, C. Wu, L. Shen, J. Liu and Z. Xu, Anal. Chim. Acta, 2014, 828, 9–16 CrossRef CAS PubMed.
- Y. Jia, Q. Mou, Y. Yu, Z. Shi, Y. Huang, S. Ni, R. Wang and Y. Gao, Anal. Chem., 2019, 91, 5217–5224 CrossRef CAS PubMed.
- O. Abollino, A. Giacomino, M. Malandrino, G. Piscionieri and E. Mentasti, Electroanalysis, 2010, 20, 75–83 CrossRef.
- D. Li, C. Y. Li, H. R. Qi, K. Y. Tan and Y. F. Li, Sens. Actuators, B, 2016, 223, 705–712 CrossRef CAS.
- Y. Shiraishi, S. Sumiya, Y. Kohno and T. Hirai, J. Org. Chem., 2008, 73, 8571–8574 CrossRef CAS PubMed.
- X. Chen, Y. Xiang, Z. Li and A. Tong, Anal. Chim. Acta, 2008, 625, 41–46 CrossRef CAS PubMed.
- H. N. Kim, W. X. Ren, J. S. Kim and J. Y. Yoon, Chem. Soc. Rev., 2012, 41, 3210–3244 RSC.
- İ. Aydin, K. Ertekin, S. Oncuoglu and C. G. Hizliates, Opt. Mater., 2021, 115, 111030 CrossRef.
- A. Kaya and J. Russ, Coord. Chem., 2021, 47, 903–908 CrossRef.
- F. Liang, L. Xu, D. Jin, L. Dong, S. Lin, R. Huang, D. Song and P. Ma, Luminescence, 2022, 37, 161–169 CrossRef CAS PubMed.
- H. Li, W. L. Sheng, L. Z. Wang, X. Meng, H. D. Duan and L. Q. Chi, RSC Adv., 2021, 11, 23597–23606 RSC.
- Y. Li, Y. Zhang, M. Wang, D. Wang, K. Chen, P. Lin, Y. Ge, W. Liu and J. Wu, J. Hazard. Mater., 2021, 415, 125712 CrossRef CAS PubMed.
- Y. Cai, J. Wang, L. Niu, Y. Zhang, X. Liu, C. Liu, S. Yang, H. Qi and A. Liu, Analyst, 2021, 146, 4630–4635 RSC.
- S. Chemate and N. Sekar, Sens. Actuators, B, 2015, 220, 1196–1204 CrossRef CAS.
- B. Muthuraj, R. Deshmukh, V. Trivedi and P. K. Iyer, ACS Appl. Mater. Interfaces, 2014, 6, 6562–6569 CrossRef CAS PubMed.
- Y. Li, X. J. Wen, X. Y. Ding, X. Teng, X. H. Xiong and Y. Y. Liu, Res. Chem. Intermed., 2022, 48, 67–83 CrossRef CAS.
- K. Li, Y. Xiang, X. Wang, J. Li, R. Hu, A. Tong and B. Z. Tang, J. Am. Chem. Soc., 2014, 136, 1643–1649 CrossRef CAS PubMed.
- S. P. Wu, T. H. Wang and S. R. Liu, Tetrahedron, 2010, 66, 9655–9660 CrossRef CAS.
- Q. Zhou, Z. M. Wu, X. H. Huang, F. F. Zhong and Q. Y. Cai, Analyst, 2015, 140, 6720–6726 RSC.
|
This journal is © The Royal Society of Chemistry 2022 |
Click here to see how this site uses Cookies. View our privacy policy here.