DOI:
10.1039/D2RA03729G
(Paper)
RSC Adv., 2022,
12, 26090-26098
Optical chemical sensor of Gd(III) based on 5-(2′-bromophenyl- azo)-6-hydroxypyrimidine-2,4-dione immobilized on poly(methyl methacrylate) and 2-nitrophenyloctylether matrix
Received
16th June 2022
, Accepted 7th September 2022
First published on 15th September 2022
Abstract
A novel optical chemical sensor (optode) was fabricated for the determination of Gadolinium ions. The optical sensor was prepared by incorporating a recently synthesized ionophore, 5-(2′-bromophenylazo)-6-hydroxy pyrimidine-2,4-dione (BPAHPD), and 2-nitrophenyloctylether (NPOE) as a plasticizer in poly(methyl methacrylate) (PMMA) membrane. The color of the sensing membrane in contact with Gd(III) ions changed from yellow to red-orange due to the adsorption of Gd(III) with the maximum absorbance (λmax) at 563 nm. The chemical sensor responds optimally towards Gd(III) ions at the optimum conditions of pH 7.5, contact time 10 min, 150 ng mL−1 Gd(III), and 5.0 mL solution. The linear regression equation achieved was A = 4.36C (μg mL−1) – 0.15 (r = 0.9976). A linear Gd(III) calibration curve can be established in the concentration range of 5.0–250 ng mL−1 with R2 = 0.9976. Detection and quantification limits are 1.47 and 4.75 ng mL−1, respectively. The molar absorptivity and Sandell sensitivity are found to be 6.86 × 107 L mol−1 cm−1 and 0.023 ng cm−2, respectively. In addition to its stability and reproducibility, the optode revealed a great selectivity toward Gd(III) ions as compared to other coexisting ions in real samples. The recovery of Gd(III) ions from the sensor material was achieved using 0.4 M HNO3. The offered optode sensor membrane has been employed to monitor Gd(III) in soil, sediments, river water, and urine with an internal standard addition method and compared statistically with the ICP-OES method. The results revealed calculated t-values between 1.11–1.85, whereas F values were in the range of 2.46–3.77 which did not exceed the theoretical values, indicating no significant difference at 95% confidence level. The observed percent recovery is in the range of 97.24–102.52%.
Introduction
Rare-earth elements (REE) are becoming more critical in high-tech ceramics, cutting-edge engineering, and specialized glasses, which has driven the development of reliable and efficient analytical procedures for assessing traces of these metals. Fluorescent bulbs, energy-saving lights, color televisions, and eyeglasses are just a few examples of common objects that contain the rare chemical gadolinium.1 Gadolinium mainly occurs in two different types of ores. Gadolinium is increasingly being used because it is suitable for making catalysts and polishing glass.
Gadolinium can be inhaled as fumes and damps and is often dangerous to the environment. Lung embolisms may arise from this, especially if you are exposed for a prolonged period of time. The liver is put in danger when gadolinium accumulates in the body. There are many ways that gadolinium is released into the environment, but the petroleum sector is the main source. Additionally, when household items are thrown away, it may be discharged into the environment. Gadolinium will gradually accumulate in soils and aquatic environments, increasing concentrations in humans, animals, and soil particles. Gadolinium damages cell membranes in aquatic species, which has a number of negative effects on reproductive and nervous system functions.
Due to the effective use of gadolinium compounds in industry, monitoring of Gd(III) ions has lately become necessary. Diverse techniques have been used for Gd(III) assessment which most of them have been spectroscopic procedures like inductively coupled plasma-mass spectrometry (ICP-MS),2–7 atomic emission spectroscopy,4 electron spin resonance,5–10 laser-based multi-step resonance ionization,11 phosphorescence opto-sensing,12 high-resolution I3-spectroscopy,13 time-resolved fluorimetry,14 spectrophoto-fluorimetric assessment,15,16 quenching of gadolinium fluorescence,17 spectrophotometry.18 Ion selective electrode (ISE),19,20 several nucleic21 and other methodologies.22–24
Each of these procedures demand expensive, sophisticated equipment. Therefore, a practical, affordable approach that can provide the selectivity to detect Gd(III) in the presence of other, extremely similar lanthanides is essential.25–31 In our Laboratory, various strategies for optical sensors for different metal ions in aqueous samples have been investigated,32–37 since they can easily be included in low-cost, user-friendly kits. Additionally, they can offer the required sensitivity and selectivity for environmental checking.39–49
In this study, we have developed a new optode sensor Gd(III) sensing method that is straightforward, secure, affordable, ecologically friendly, and focused on green analytical chemistry. The sensing membrane is advanced constructed on immobilization of BPAHPD in polymethyl methacrylate (PMMA) membrane using 2-nitrophenyl octyl ether (NPOE) as plasticizer. Theoretical and basic concepts of bulk optode membranes relying on reversible mass analyte movement from sample in the bulk of the sensing layer have been explained.50,51 The sensor can be simply regenerated, by using a 0.4 M HNO3 solution. According to the best of our knowledge no manufactured optode has been described in the literature for determining of gadolinium applying the studied reagent.
Experimental
Chemicals and instruments
Cadmium(II) nitrate [Cd(NO3)2], copper(II) chloride (CuCl2), zinc(II) nitrate [Zn(NO3)2], nickel(II) nitrate [Ni(NO3)2], iron(III) chloride (FeCl3), lanthanum nitrate[La(NO3)3], samarium nitrate [Sm(NO3)3], scandium nitrate[Sc(NO3)3], yttrium nitrate [Y(NO3)3], thorium nitrate [Th(NO3)4], uranyl nitrate [UO2(NO3)2], sodium hydroxide (NaOH), hydrochloric acid (HCl), and tetrahydrofuran (THF) were obtained from Merck. BPAHPD was synthesized according to the method described previously.52–54 Poly(methyl methacrylate) (PMMA) was obtained by synthesis with an average molecular weight of about 220
000. The double distilled water was applied as a solvent throughout all assessments. The pH of the solution was adjusted by dropwise addition of either 0.10 M NaOH or 0.10 M HCl. Standard elation of Gd(III) were prepared by dissolution of reasonable amounts of the oxide (99.99% pure, Aldrich) in small volumes of concentrated HCl, followed by dilution with distilled water. Standardization was achieved as ascribed in the literature.55
Instrumentation
The thickness of the optode was assessed by a digital micrometer (Mitutoy, Japan) with an accuracy of ± 0.001 mm. A microprocessor-based pH meter model Orion research model 601 A/digital ionalyzer was used for pH assessments. Atomic absorption spectrometer model 6300 (AAS), Shimadzu (Japan), was done for assessments with flame of N2O–C2H2 and the device settings were according to the manufacturer's recommendations. UV-vis spectrophotometer model V 53 from JASCO (Tokyo, Japan) was employed for recording the spectra and the absorbance measurements. The absorbance measurements were achieved by mounting the optode samples (1 cm × 2 cm) inside a quartz cuvette. The absorbance measurements of the optode samples were accomplished with respect to air as well as blank optode sample.
Preparation of optode sensor membrane
The optode sensor membrane was prepared by dissolving 0.300 g PMMA, 40 mg BPAHPD, and 120 μL NPOE in 10 mL THF. The mixture was stirred at a constant speed for 5.0 h at room temperature. Consequently, the reaction mixture was poured into a casting glass (5 cm × 12 cm) and left at room temperature for 8.0–10 hours, until all the solvent evaporated. The membranes were released from the cast, washed with distilled water, dried at room temperature, and cut into rectangle shape (1 cm × 2 cm). The membrane thickness was c.a. 0.05 mm.
It was important to adjust the various experimental conditions in order to achieve the optimum sensing optode film in terms of uniformity and maximal sensitivity toward Gd(III). The BPAHPD amount was optimized by varying its concentration from 15.0 to 60 mg in the casting solution. The optode films thus gotten were equilibrated with 5.0 mL standard solution of 150 ng mL−1 Gd(III), and the absorbance values at λmax = 563 nm were recorded. The amount of BPAHPD in the optode film that provided the highest absorbance was fixed for rest of the investigates.
Determination of optimum pH
The optimum pH was detected by evaluating the absorbance of membranes that were previously soaked in 5.0 mL solution involving 1.0 mg L−1 of Gd(III) ion in the pH range of 6.0–9.0 for a definite time. The optimum pH was specified by better absorbance at 563 nm.
Interfering ions
The interfering ions including K+, Na+, Ca2+, Mg2+, Cd2+, Cr3+, Ni2+, Cu2+, Pb2+, Fe2+, Fe3+, Al3+, Zn2+ La3+, Sm3+, Er3+, Sc3+, and Y3+, were investigated by assessing the absorbance of the solution in the existence of the interfering cations and Gd(III) in various concentration ratio at 563 nm and pH 7.5.
Accuracy and precision
To investigate the achieved optodes accuracy and precision, solutions including three various concentrations of Gd(III) were prepared. The assay optode was analyzed in six replicates, and the relative standard deviation (RSD) was evaluated as a percentage within the same day to assess the repeatability (intra-assay) and over five days to estimate evaluate the intermediate precision (inter-assay).
Determination of Gd(III) in urine samples
Urine was collected and stored in plastic containers without any preservatives. The urine samples were digested following the procedure previously reported by Moyano et al.56 The resultant clear solution from the previous step was loaded onto a AG50-X8 cation-exchange column, and the chromatographic procedure recommended by Crock and co-worker57 was performed in order to remove Ca(II) and other possible contaminants present in the sample. The elution procedure was as follows: (a) load 15 mL of sample solution; (b) elute with 15 mL of 2.0 M HCl, followed by 15 mL of 2.0 M HNO3, discarding both eluates; (c) elute with 15 mL of 7.5 M HNO3, and collect the eluate for subsequent Gd determination; (d) regenerate the column by washing with 50 mL of 8.0 M HNO3.
In order to demonstrate the validity of the proposed optode method, 150 mL of spiked urine sample was collected and divided into 10 portions of 15 mL each. The proposed method was applied to six portions and the average quantity of Gd(III) obtained was taken as a base value. Then, increasing quantities of gadolinium were added to the other sample aliquots and Gd(III) was determined by the same optode. Using the calibration procedure to assess Gd(III) content was employed by the recommended sensor in the urine samples.
Determination of Gd(III) in water samples
In order to test the applicability of the proposed SFODME method for the analysis of real samples, mineral and river water (obtained of Benha, Egypt) were studied. In order to remove each solid material, all water samples were centrifuged at 6000 rpm for 5.0 min and were filtered with filter paper No. 0.45 μm before use. A reasonable volume of water sample (500–1000 mL) was moved to a 1000 mL beaker and 0.2 mL of concentrated HCl was added. The beaker was heated in a water-bath and the solution was evaporated to 5.0 mL. The concentrated solution was poured quantitatively into a 5.0 mL calibrated flask and diluted with water to the mark. The treated solutions were emptied into the sample optode, and lanthanum assessments were taken under the previously stated circumstances.
Determination of Gd(III) in soils and sediments
Sample (1.0 g) was weighed into a PTFE beaker, and 5.0 mL of 70% HClO4 and 10 mL of 48% HF were added. The sample was heated in sand bath to incipient dryness. The acid attack with HClO4 and HF (1 + 2) was repeated three times to complete digestion of the silicate matrix. Then the samples were moved into flasks and diluted with 5.0 mL of 5.0% NaOH and distilled water to 50 mL (pH = 7.5). Subsequently, the spectra of these solutions were assessed by applying the developed Gd(III) sensor as well as its calibration curve, which was attained after measuring a series of gadolinium ion standard solutions. Then, the gadolinium ion concentration in the samples was evaluated.
Results and discussion
Spectral characteristics
Supporting matrix stability and sensor membrane response toward Gd(III); PMMA was preferable as a supporting matrix for BPAHPD owing to its transparency, hydrophobicity, resistance to acid, base and salt solutions,58 and low absorbance in the UV-vis range.59 These will maintain the sensor membrane stable throughout Gd(III) in water examination and enable for quantitative UV-Vis spectrophoto-metry analysis. As a ligand, BPAHPD can achieve a colorful complex ion with a number of cations, that are Y(III),52 Sm(III),53 and Bi(III).54 The complex ions of BPAHPD with those cations are advanced through the coordination bond amongst –OH and azo-nitrogen groups of the phenolic ring with the cations.
In our Laboratory, various strategies for optical sensors for different metal ions in aqueous samples have been illustrated.32–38 The comparison of UV-vis spectra of optode samples equilibrated with diverse aqueous matrix having same concentration of Gd(III) (150 ng mL−1) is shown in Fig. 1. It is seen from this figure that a pH 7.5 ± 0.2 in the equilibrating solution improved the colour variation in optode sample corresponding to the formation of Gd(III)-BPAHPD complex. The optode response to Gd(III) in seawater was substantially lower than in other aqueous matrices, and it may not be effective for detecting and quantifying Gd(III) in seawater. The absorbance spectra of optode samples equilibrated with solutions containing different Gd(III) samples in pH 7.5 are given (Fig. 1). The comparison of spectra specified that there was great bathochromic shift in the wavelength and absorbance of optode samples from 451 nm (blank) to 563 nm on equilibration with buffer solutions involving Gd(III) concentration ranging from 5.0 to 250 ng mL−1. The absorbance maxima at 563 nm is stated as a characteristic of Gd(III)-BPAHPD complex. This designated that the optode is responsive towards variation in the Gd(III) concentrations in the equilibrating solution. The absorbance of the blank optode sample [without Gd(III)] is expressively lower as compared to that at 563 nm. Hence, the change in the absorbance at 563 nm can be applied for quantitative assessment of Gd(III) in the aqueous samples. It was also observed that red-orange colour of Gd(III)-loaded optode sample varies back to yellow on immersing this optode in well-stirred 0.02 M HNO3 for 10 min. This induced that Gd(III)-BPAHPD complex established in the optode is broken at pH 2.0 to regenerate the BPAHPD in the optode.
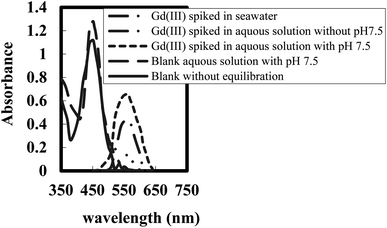 |
| Fig. 1 UV-vis spectra of optode samples (1.0 cm × 2.0 cm) equilibrated with 5.0 mL of different aqueous samples spiked with 150 ng mL−1 of Gd(III) at pH 7.5. | |
Membrane characterization
The FTIR spectra of BPAHPD immobilized in the membrane matrix are seen in Fig. 2. It is clearly displayed that BPAHPD has been effectively incorporated into the membrane. The peak at 3443 cm−1 indicates a stretching vibration of –OH group of naphthol, however the peak at 2336 cm−1 is thanks to the N
N group. The medium peak at 1606 cm−1 is attributed to the N
O bond. Further, the peak at 1250 cm−1 suggests the attendance of caryl–Ocalkyl group. Both peaks reveal the existence of NPOE as a plasticizer in the membrane.
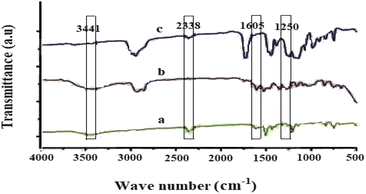 |
| Fig. 2 Infrared spectra of the membrane components. (a) BPAHPD, (b) NPOE, (c) PMMA. | |
The effect of pH on sensing performance
The pH of the test solutions plays an important role in achieving a better membrane performance. The analyte solution has been subjected to pH variation, from 6.0 to 9.0. Fig. 3 recommends that the solution pH of 7.5 provides the best response. Furthermore, at optimum pH, the amount of OH− ion in the solution is larger and the donor atom in the ligand tends to release H+ ion (deprotonated) and thus becomes partially negative-charged species (L−). This condition enhances the reaction between Gd3+ or [Gd(OH)2]+ in the solution and BPAHPD ligand in the matrix to form GdL2. On the other hand, at lower pH, large amount of H+ in the solution causes the construction of free metal ion (Mn+) and the hydroxyl group in the ligand remains protonated. This situation causes the interaction between metal ion and ligand much more problematic to occur due to charge repulsion between positive metal ion and positively protonated ligand. At higher pH (>9.0), the absorbance is getting smaller possibly because of leaching process of BPAHPD ligand from the membrane matrix as indicated by the orange-red color change of the solution. For all of the tested pH, the absorbance has been observed to reach its maximum value after 10 min of reaction.
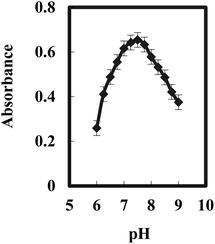 |
| Fig. 3 Effect of pH on the optical sensor of 150 ng mL−1 Gd(III) complexed with BPAHPD at the optimum conditions. | |
Optimization
In this context, it is significant to study the features of plasticizer and their relative concentrations in the optode matrix. It was stated that increase in amount of plasticizer increases the diffusion rate of anions in the plasticized membranes.60 Thus, the optode samples having varying amounts of two diverse plasticizers (e.g. PMMA and NPOE) were prepared and checked for their response time towards Gd(III) ions in pH 7.5 medium.
The absorbance variation of the optode samples, plasticized with varying amounts of PMMA and NPOE, was studied by equilibrating these with well-stirred 5.0 mL buffered sample solutions having 150 ng mL−1 Gd(III) concentrations in pH 7.5 medium (Fig. 3). The optode sensor samples were taken out at regular time intervals to monitor the absorbance of Gd(III)-BPAHPD complex at 563 nm as a function of equilibration time. The variations of absorbance at λmax in the optode samples as a function of equilibration time are shown in Fig. 4. This graph indicates the kinetics of sorption of anionic Gd(III) species in the optode sensor. As can be seen from Fig. 4, the sorption rate of gadolinium anionic species increases with increasing amount of plasticizer in the optode. The matrix establishing PMMA chains in the liquid fraction of optode (plasticizer) matrix produces obstruction in the path of the moving ion-pair.
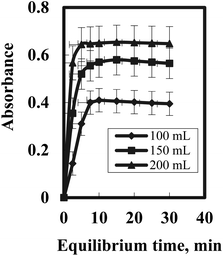 |
| Fig. 4 Gadolenium uptake kinetics as a function of plasticizer NPOE amount in the optode; Gd =150 ng mL−1; sample volume = 5.0 mL; pH 7.5. | |
The plasticizer, which is the major component of liquid phase, acts as a medium for diffusion transport of ions in the optode sensor. The optode could not be plasticized more than 95 wt% of the plasticizer as mechanical strength of the optode was not enough to use it in well-stirred solution Fig. 5. Out of two plasticizer used in the preparation of optode, the optode sample with 1.0 wt% NPOE gave faster increase in absorbance corresponding to Gd(III)-BPAHPD complex than the optode made by TEHP. Although the equilibration time needed for almost complete Gd(III) sorption is longer than 10 min, the shorter equilibration time could be used for quantitative analysis of Gd(III) as % uptake remains constant at fixed equilibration time. The equilibration time of 10 min could be used for constructing calibration graph for aqueous sample including Gd(III) greater than 250 ng mL−1. Nevertheless, the detection limit for Gd(III) would be better after 10 min equilibration of the optode sensor sample as more than 96.5% of Gd(III) sorption in the optode is acquired. Lastly, the optode sensor composition was kept as: 87.2 wt% PMMA + 1.1 wt% NPOE + 11.7 wt% BPAHPD for Gd(III) uptake studies with an equilibration time of 10 min.
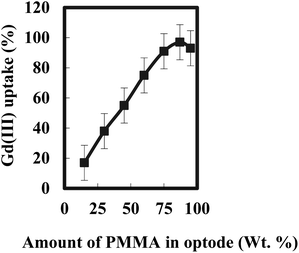 |
| Fig. 5 Variation of Gadolinium uptake with PMMA concentration in the optode; Gd= 150 ng mL−1; sample volume = 5.0 mL; duration = 15 min; pH 7.5. | |
The absorbance at 563 nm examined for a long period over 12 h did not show the evidence of leaching of Gd(III)-BPAHPD complex from the optode sample to equilibrating aqueous medium with pH 7.5. There was no drift in the absorbance when the film used for gadolinium uptake was exposed to light. No appreciable variation in the optode absorbance value was detected when the film was dipped in de-ionized distilled water overnight. These observations recommend that the optode sensor film was quite stable under the circumstances of current studies.
Stoichiometric ratio
The complex nature was accomplished at the optimum conditions described above using the continuous variation and molar ratio methods. The plot of absorbance versus the mole faction of Gd(III), attained by varying the BPAHPD and Gd(III) concentration, revealed inflection at 0.33, indicating the attendance of two BPAHPD molecules in the established complex. Besides, the molar ratio method showed a ratio of BPAHPD to Gd(III) = 2.0. Subsequently, the results indicated that the stoichiometric ratio was (2
:
1) [BPAHPD: Gd(III)]. The conditional formation constant (log
K), calculated using the Harvey and Manning equation applying the data achieved from the above two methods, was achieved to be 7.41, while the true constant was 7.58.
2 BPAHPD + Gd(III) [(BPAHPD)2Gd] |
Response of sensor membrane
The UV-vis spectra of sensing membrane before and after contact with Gd(III) solution are displayed in Fig. 1. Before contact with Gd(III) solution, the sensor membrane has highest absorbance (λmax) at 451 nm, and after contact with Gd(III) solution at pH 7.5, it shifts to 563 nm. The complex formation Gd(III)-BPAHPD in the sensor membrane exhibits substantial bathochromic shift from 451 to 563 nm. It has been described that the λmax of BPAHPD and Gd(III)−BPAHPD complex in chloroform is 456 nm and between 540–570 nm, respectively. The slight variance in our results may be owing to the BPAHPD sensitivity in various environments that lead to shift in λmax when it packs in the solid membrane matrices.44 It is noteworthy to note that the cation permeability in the polymer matrix is lower than that in the solution. This may also cause the shift of λmax. For comparison, it has been stated that the λmax of BPAHPD and Gd(III)−BPAHPD complex in the CTA membrane is observed at 451 and 563 nm, respectively. The membrane was prepared by solvent mixing of 10 mL dichloromethane and 10 mL chloroform, and dried for 48 h. By applying this reported membrane, it has been found that the linear range of Gd(III) calibration curve was in the range of 5.0–275 ng mL−1 and the equilibrium time for complex formation was achieved after 50 minutes of reaction at pH 7.5. It is, hence, obvious that our results is superior, especially in term of response time (10 min), required sample size (5.0 mL only), less usage solvent and shorter evaporation time, meaning that the preparation of the sensing membrane in our study produces less waste, reduces gas emission and needs shorter analysis time. After contact with Gd(III) solution, the membrane colour variations from yellow to red-orange as revealed in Fig. 6. The colour intensity of the membrane increases along with the increase of the Gd(III) concentration in the solution.
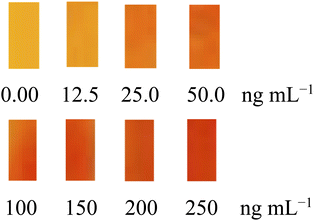 |
| Fig. 6 Progress of optode sensor membrane colour change along with increase in Gd(III) concentration. | |
Regeneration of the sensor
The reversibility of the sensor was examined by washing the applied sensors with 0.2 M iodate and/or thiocyanate solutions. The results confirmed that the sensors were not regenerated to be use one more time for Gd(III) determination. In addition, HCl and/or HNO3 were also tested for the regeneration of the used sensor by immersion of the applied sensor into the acids solution (0.1–0.7 M) for 5.0 min. The results demonstrated that the sensors could be regenerated in 0.4 M HNO3 solution. Hence, each sensor can be used many times for Gd(III) analysis.
Interfering ions
The optode selectivity was checked by equilibrating optode samples with solutions containing various cations (Th4+, La3+, Sc3+, Y3+, Sm3+, Nd3+, Eu3+, Lu3+ and Fe3+) and anions (F−, I−, NO3−, CO32−, PO43− and C2O42−). The absorbance spectra of optode samples did not vary on its equilibration with solution having 5.0–500 μg of Th4+. Conversely, some of these ions were established to affect the Gd(III) sorption in the optode. In order to investigate the effects of these ions on Gd(III) sorption in the optode, the uptake studies were done in the existence of micromolar concentrations of cations like Th4+, Nd3+, and anions such as F− at pH 7.5 with 150 ng mL−1 Gd(III) concentration. In all cases except F−, the absorbance variations in the optode samples were within ± 5.0%, suggesting that existence of these cations/anions in the micro-molar concentrations will not expressively influence the Gd(III) examination. The tolerance limit was taken as 5.0% deviations in the mean absorbance values in Gd(III)– BPAHPD complex at 563 nm in the absence of the competing cations/anions. In case of F−, the absorbance was reduced to 18% which designated that F− ions interfere in the sorption of Gd(III) in the optode. Addition of 1.0 mL of 1.0 × 10−3 M aluminium chloride eliminate the interference of F− up to 500-fold molar excess. It is recommended that all other foreign ions studied can be tolerated in considerable amounts, so that the proposed optode method can be used to determine Gd(III) in environmental samples directly.
Calibration and reproducibility
The optode response, in the form of variation in absorbance at 563 nm, towards gadolinium concentration is up to 275 ng mL−1 after correcting for the blank. The blank absorbance at 563 nm was assessed after equilibrating optode sample with blank solution at pH 7.5, without gadolinium. The absorbance linearly varies as a function of Gd(III) concentration range of 5.0–275 ng mL−1. Nevertheless, the calibration line did not pass through the zero. This may be because of the chemical changes created on sorption of Gd(III) in the matrix that might have varied the absorbance. These chemical alterations may be counter ions or water content in the optode matrix. The water contents before and after Gd(III) sorption in optode sample were created to be within 1.2 wt%. The least concentration of Gd(III) required in the 5.0 mL equilibrating solution to achieve distinct color change of optode (dimensions 2.0 cm × 1.0 cm) was established to be 275 ng mL−1. Though, this detection limit of Gd(III) concentration can be further greater by using larger volume of aqueous sample.
The linear regression equation achieved was A = 4.36C (μg mL−1) – 0.15 (r = 0.9976). The molar absorptivity was accomplished to be 6.86 × 107 L mol−1 cm−1 at 563 nm, whereas the Sandell sensitivity was established to be 0.023 ng cm−2 Table 1. The standard deviations of the absorbance assessments were calculated from a series of 13 blank solutions. The detection (K = 3) and quantification (K = 10) limits of the method were established61 and recorded in Table 1, according to the IUPAC definitions C1 = KSo/s where C1 is the limit of detection, So is the standard error of blank, s is the slope of the standard curve and K is the constant related to the confidence interval. The relative standard deviation was 1.56% attained from a series of 10 standards each including 150 ng mL−1 of Gd(III). For more precise results, Ringbom optimum concentration range were evaluated and recorded in Table 1.
Table 1 Analytical features of the proposed method
Parameters |
Using optode |
Parameters |
Using optode |
pH |
67.5 |
Regression equation |
|
Equilubrium time (min) |
15 |
Slope (μg mL−1) |
4.36 |
Stirring time (min) |
5.0 |
Intercept |
−0.15 |
Beer's range (ng mL−1) |
5.0–250 |
Correlation coefficient (r) |
0.9976 |
Ringbom range (ng mL−1) |
25–225 |
RSD a (%) |
1.56 |
Molar absorptivity (L mol−1 cm−1) |
6.86 × 107 |
Detection limits (ng mL−1) |
1.47 |
Sandell sensitivity (ng cm−2) |
0.0022 |
Quantification limits (ng mL−1) |
4.75 |
The reproducibility of the optode membrane was estimated applying standard gadolinium solution. Reasonable aliquots were spiked from standard gadolinium solution into the medium of uptake investigates, e.g., pH 7.5, volume = 5.0 mL; and [Gd] = 150 ng mL−1. The optode sample was taken out for the absorbance assessments after equilibration for 15 min. The mean absorbance values with the relative standard deviation were established to be 0.0.65 ± 0.03 (n = 6). The small deviations in absorbance values recommend that optode membrane responses are reproducible under the conditions of this study.
The intra-day and inter-day accuracy and precision analytical results represented that the attained approach is highly repeatable and reproducible, with coefficients of variation ranging between 1.33 and 1.96%.
So as to enhance the variation in absorbance at 563 nm in the optode, the optode samples of diverse dimensions were equilibrated with pH = 7.5 solutions involving 1.0 μg of Gd(III) in 5.0 mL medium. The absorbance increases from 0.22 to 0.65 by decreasing area of optode samples from 4.0 to 1.0 cm2. This can be attributed to increase in Gd(III) concentration in the unit volume of the optode. As a consequence, by decreasing the volume of the optode, the Gd(III) detection limit can be enhanced.
The characteristics of the suggested Gd optical sensor were compared with other techniques for evaluation of Gd in the literature in Table 2. It was mentioned that the obtained detection limit is much lower than previously reported procedures [3,6,18–20,39] (Table 2). Although, the proposed method has more advantages through the sensitivity and interference point of view, it safer from a narrow range compared with other methods. Moreover, for the determination of Gd(III) ions in diverse samples, there is a speedy, ecologically safe, and easy procedure that may be used. The broader range of detection, greater accuracy, better stability and lower time consumption demonstrate the advantages of the recommended method over the other techniques.
Table 2 Comparison of Gd(III) optical sensor with previous methods for determination of gadolinium
Method |
DLa (ng mL−1) |
LRb (ng mL−1) |
Ref. |
Detection limit. Linear range. |
SPE-ICP |
78.6 |
— |
3 |
ICP-OES |
4.0 |
15–200 |
6 |
Spectrophotometry |
80 |
100–1400 |
18 |
Ion-selective membrane |
912 |
1100–15850 |
19 |
Ion-selective membrane |
1100 |
2000–16000 |
20 |
Optical sensor |
17.3 |
90–3150 |
39 |
Optical sensor membrane |
1.47 |
5.0–250 |
This work |
Application of the sensor to synthetic sample and real samples
The gadolinium membrane sensor was employed in the fruitful spectrophotometric titration of a gadolinium ion solution (1 × 10−4 M) with EDTA (1 × 10−2 M). The amount of gadolinium can be determined with the proposed sensor.
The recommended Gd(III) optical sensor device was employed to the assessment of gadolinium ions in synthetic and river water samples. Table 3 lists the respective resulting data of these assays. Furthermore, the membrane was applied to assess the Gd(III) ion content of a 50 mL urine sample through spiking method, and the results are shown in Table 3. As can be seen, the amounts of the Gd ions, which were added to the urine sample, could be assessed by the sensor with relatively great accuracy.
Table 3 Results of the Gd(III) ion concentration measurements in spiked samples
Sample |
Gd(III) added ng mL−1 |
Gd(III) founda ng mL−1 |
Recovery% |
t-testb |
F-valueb |
Optode |
ICP-OES |
Optode |
ICP-OES |
Average of six determinations. Theoretical value for t- and F-values for six degrees of freedom and 95% confidence limits are 2.57 and 5.05, respectively. |
Urine 1 |
0.0 |
ND |
ND |
— |
— |
— |
— |
100 |
99.2 |
104.3 |
99.2 |
104.3 |
1.42 |
2.97 |
150 |
151.5 |
147.5 |
101.0 |
98.33 |
1.67 |
3.43 |
200 |
203.4 |
195.5 |
101.7 |
97.75 |
1.12 |
2.46 |
Urine 2 |
0.0 |
ND |
ND |
— |
— |
— |
— |
80 |
79.4 |
81.5 |
99.25 |
101.88 |
1.63 |
3.18 |
160 |
162.1 |
158.2 |
101.31 |
98.88 |
1.85 |
3.61 |
240 |
237.8 |
244.5 |
99.08 |
101.88 |
1.37 |
2.87 |
River water |
0.0 |
ND |
ND |
— |
— |
— |
— |
60 |
60.8 |
59.3 |
101.33 |
98.83 |
1.66 |
3.17 |
120 |
117.9 |
123.4 |
98.25 |
102.83 |
1.32 |
2.58 |
180 |
182.0 |
175.6 |
101.11 |
97.56 |
1.76 |
3.63 |
Mineral water |
0.0 |
ND |
ND |
— |
— |
— |
— |
75 |
76.2 |
72.5 |
101.60 |
96.67 |
1.58 |
2.81 |
150 |
147.6 |
153.4 |
98.40 |
102.27 |
1.79 |
3.72 |
225 |
228.6 |
222.2 |
101.60 |
98.76 |
1.46 |
3.07 |
The offered technique could effectively recover gadolinium ion from soils and sediments including various foreign ions. The concentrations of Gd(III) were evaluated once varying amounts of Gd(III) were spiked into soil and sediment samples. The findings are provided in Table 4.
Table 4 Determination of gadolinium in soil and sediments samples (the results are based on triplicates measurements)
Samples |
Gd(III) founda ng mL−1 |
t-testb |
F-valueb |
Proposed |
ICP-OES |
Average of six determinations. Theoretical value for t- and F-values for six degrees of freedom and 95% confidence limits are 2.57 and 5.05, respectively. |
1 |
46.2 ± 0.2 |
44.5 ± 0.3 |
1.27 |
2.73 |
2 |
32.8 ± 0.2 |
31.0 ± 0.3 |
1.38 |
2.99 |
3 |
37.2 ± 0.4 |
36.3 ± 0.4 |
1.51 |
3.27 |
4 |
68.6 ± 0.2 |
67.4 ± 0.4 |
1.11 |
2.56 |
5 |
59.9 ± 0.5 |
59.0 ± 0.3 |
1.73 |
3.53 |
6 |
49.5 ± 0.3 |
48.3 ± 0.4 |
1.82 |
3.77 |
The performance of the suggested method was evaluated by calculation of the t-value (for accuracy) and F-test (for precision) compared with the ICP-OES method.62 The mean values were gotten in a Student's t- and F-tests at 95% confidence limits for five degrees of freedom.63 The results revealed that the calculated values (Tables 3 and 4) did not exceed the theoretical values.
Conclusions
A PMMA-based optode sensor membrane has been developed for the preconcentration, separation, and evaluation of gadolinium. The optode variations color owing to Gd(III) from to yellow to red-orange uptake at pH 7.5. The color intensity in the optode sample was accomplished to be reliant on the gadolinium uptake from the sample solution as well as the membrane composition. The absorbance linearly varies as a function of Gd(III) concentration range from 5.0–275 ng mL−1. The detection and quantification limits of the optode film (dimension: 2.0 cm × 1.0 cm) were assessed to be 1.47 and 4.75 ng mL−1 Gd(III), respectively. The attendance of micro-molar concentrations of cations like Th4+, Nd3+, Fe3+, and of anions such I−, NO3−, and CO32−, etc. cannot be tolerated throughout gadolinium revealing. With the exception of F− ions, the negative bias was detected at higher concentrations of these ions. The results demonstrated that the sensors could be regenerated in 0.4 M HNO3 solution. Under the circumstances of the existing experiments, the optode film was relatively stable. The outcome of this work reported a non-toxic, economical, stable, accurate, easy-to-use, and novel optical sensor material to assess Gd(III) in synthetic, river water, soil, sediments, and urine water samples and compared statistically with the ICP-OES method.
Ethical statement
All biological studies were carried out in strict accordance with the animal welfare guidelines of the World Organization for Animal Health. All biological experiments were performed using protocols approved by the Laboratory Animal Ethics Committee of “Egypt” was approved by Commission on the Ethics of Scientific Research, Faculty of medicine, Benha University. In all cases, informed written consent was obtained from each participant.
Data availability
All data and materials should be available upon request.
Author contributions
Eslam Moustafa: conceptualization, data curation, investigation, methodology, visualization, validation, writing – original draft, writing – review & editing. Alaa Amin: conceptualization, methodology, data curation, investigation, supervision, validation, writing –original draft, writing – review & editing. Eman Darwish: conceptualization, investigation, methodology, validation, writing – original draft, writing – review & editing.
Conflicts of interest
The authors declare that they have no known competing financial interests or personal relationships that could have appeared to impudence the work reported.
Acknowledgements
The authors' sincere thanks are due to Benha and Port Said Universities, for providing the necessary facilities.
References
- R. Zare-Dorabei, P. Norouzi and M. Ganjali, J. Hazard. Mater., 2009, 171, 601–606 CrossRef CAS PubMed.
- H. Isnard, R. Brennetot, C. Caussignac, N. Caussignac and F. Chartier, Int. J. Mass Spectrom., 2005, 246, 66–73 CrossRef CAS.
- K. Hennebruder, R. Wennrich, J. Mattusch, H. J. Stark and W. Engewald, Talanta, 2004, 63, 309–316 CrossRef PubMed.
- P. T. Normann, P. Joff, I. Martinsen and H. S. Thomsen, J. Pharm. Biomed. Anal., 2000, 22, 939–947 CrossRef CAS.
- D. B. Salem and J.-A. Barrat, Talanta, 2021, 221, 121549 CrossRef PubMed.
- J. A. Salonia, J. A. Gasquez, L. D. Martinez, S. Cerutti, M. Kaplan and R. A. Olsina, Instrum. Sci. Technol., 2006, 34, 305–316 CrossRef CAS.
- X. Cao, M. Yin and B. Li, Talanta, 1999, 48, 517–525 CrossRef CAS.
- C. Miyake and S. Imoto, J. Less-Common Met., 1986, 121, 261–264 CrossRef CAS.
- C. Miyake, M. Kanamaru and S. Imoto, J. Nucl. Mater., 1986, 137, 256–260 CrossRef CAS.
- S. Moyer and W. J. McCarthy, Anal. Chim. Acta, 1969, 48, 79–85 CrossRef.
- K. Blaum, C. Geppert, W. Schreiber, J. Hengstler, P. Muller, W. Nortershauser, K. Wendt and B. Bushaw, Anal. Bioanal. Chem., 2002, 372, 759–764 CrossRef CAS.
- Z. Gong and Z. Zhang, Mikrochim. Acta, 1997, 126, 117–121 CrossRef CAS.
- H. Robins and D. A. Hilton, Int. J. Appl. Radiat. Isot., 1970, 21, 619–623 CrossRef PubMed.
- B. Berg, E. Mainka and H. J. Ache, Anal. Bioanal. Chem., 1989, 333, 766–767 Search PubMed.
- T. Taketatsu and A. Sato, Anal. Chim. Acta, 1978, 98, 397–399 CrossRef CAS.
- S. M. Borisov, R. Fischer, R. Saf and I. Klimant, Adv. Funct. Mater., 2014, 24, 6548–6560 CrossRef CAS.
- R. Reisfeld and E. Biron, Talanta, 1970, 17, 105–108 CrossRef CAS PubMed.
- I. Mori, Y. Fujita, M. Toyoda and K. Tanaka, Anal. Lett., 1992, 25, 2377–2388 CrossRef CAS.
- M. R. Ganjali, M. Emami, M. Rezapour, M. Shamsipur, B. Maddah, M. S. Niasari, M. Hosseini and Z. Talebpoui, Anal. Chim. Acta, 2003, 495, 51–59 CrossRef CAS.
- M. R. Ganjali, P. Norouzi, T. Alizadeh, A. Tajarodi and Y. Hanifehpour, Sens. Actuators, B, 2007, 120, 487–493 CrossRef CAS.
- H. A. Zamani, G. Rajabzadeh, M. R. Ganjali and P. Norouzi, Anal. Chim. Acta, 2007, 598, 51–57 CrossRef CAS.
- H. P. Neves, G. M. D. Ferreira, G. M. D. Ferreira, L. R. de Lemos, G. D. Rodrigues, V. A. Leao and A. B. Mageste, Sep. Purif. Technol., 2022, 282, 120064 CrossRef.
- E. A. El-Sofany, J. Hazard. Mater., 2008, 153, 948–954 CrossRef CAS PubMed.
- M. Soylak and H. Balgunes, J. Hazard. Mater., 2008, 155, 595–600 CrossRef CAS PubMed.
- M. R. Ganjali, A. Daftari, P. Norouzi and M. Salavati-Niasari, Anal. Lett., 2003, 36, 1511–1522 CrossRef CAS.
- M. R. Ganjali, M. Dodangeh, H. Ghorbani, P. Norouzi and M. Adib, Anal. Lett., 2006, 39, 1075–1086 CrossRef CAS.
- M. R. Ganjali, P. Norouzi, M. Adib and A. Ahmadalinezhad, Anal. Lett., 2006, 39, 495–506 CrossRef CAS.
- M. R. Ganjali, P. Norouzi, B. Akabri-Adergani, S. Riahi and B. Larijani, Anal. Lett., 2007, 40, 1923–1938 CrossRef CAS.
- M. R. Ganjali, P. Norouzi, F. Faridbod, N. Hajiabdollah, B. Larijani and Y. Hanifehpour, Anal. Lett., 2007, 40, 2544–2561 CrossRef CAS.
- M. R. Ganjali, P. Norouzi, F. Faridbod, N. Hajiabdollah, R. Dinarv and S. Meghdadi, Anal. Lett., 2008, 41, 3–23 CrossRef CAS.
- H. A. Zamani, M. R. Ganjali, P. Norouzi and S. Meghdadi, Anal. Lett., 2008, 41, 902–916 CrossRef CAS.
- E. M. I. Moustafa, A. S. Amin and M. A. El-Attar, Anal. Biochem., 2022, 654, 114835 CrossRef CAS.
- H. H. El-Feky, A. S. Amin and E. M. I. Moustafa, RSC Adv., 2022, 12, 18431–18440 RSC.
- A. S. Amin, S. El-Bahy and H. H. El-Feky, Anal. Biochem., 2022, 643, 114579 CrossRef CAS.
- A. S. Amin and H. El-Feky, Iran. J. Anal. Chem., 2021, 8, 65–77 CAS.
- H. H. El-Feky, A. M. Askar and A. S. Amin, RSC Adv., 2021, 11, 35300–35310 RSC.
- A. S. Amin, Eurasian J. Anal. Chem., 2018, 13, 5–13 CrossRef.
- Z. Al-Mallah and A. S. Amin, J. Ind. Eng. Chem., 2018, 63, 281–287 CrossRef CAS.
- R. Zare-Dorabei, P. Norouzi and M. R. Ganjali, Anal. Lett., 2009, 42, 190–203 CrossRef CAS.
- R. Seitz, in Fiber Optic Chemical Sensors and Biosensors, ed. O. S. Wolfbeis, CRC Press, Boca Raton, FL, 1991, vol. 2, pp. 1–19 Search PubMed.
- A. Safavi and M. Bagheri, Spectrochim. Acta, Part A, 2008, 70, 735–739 CrossRef PubMed.
- M. Noroozifar, M. K. Motlagh, A. Taheri and R. Zare-Dorabei, Turk. J. Chem., 2008, 32, 249–257 CAS.
- P. Pourali, M. Fazlzadeh, M. Aaligadri, A. Dargahi, Y. Poureshgh and B. Kakavandi, Arabian J. Chem., 2022, 15, 103980 CrossRef CAS.
- A. Dargahi, H. R. Barzoki, M. Vosoughi and S. A. Mokhtari, Arabian J. Chem., 2022, 15, 103801 CrossRef CAS.
- A. Dargahi, M. Vosoughi, S. A. Mokhtari, Y. Vaziri and M. Alighadri, Arabian J. Chem., 2022, 15, 103648 CrossRef CAS.
- S. Ma, S. Lee, K. Kim, J. Im and H. Jeon, Sep. Purif. Technol., 2021, 255, 117715 CrossRef CAS.
- M. R. Samarghandi, A. Ansari, A. Dargahi, A. Shabanloo, D. Nematollahi, M. Khazaei, H. Z. Nasab and Y. Vaziri, J. Environ. Chem. Eng., 2021, 9, 106072 CrossRef CAS.
- A. Dargahi, K. Hasani, S. A. Mokhtari, M. Vosoughi, M. Moradi and Y. Vaziri, J. Environ. Chem. Eng., 2021, 9, 105889 CrossRef CAS.
- M. R. Samarghandi, A. Dargahi, A. Rahmani, A. Shabanloo, A. Ansari and D. Nematollahi, Chemosphere, 2021, 279, 130640 CrossRef CAS PubMed.
- K. Seiler and W. Simon, Anal. Chim. Acta, 1992, 266, 73–78 CrossRef CAS.
- I. Oehme and O. S. Wolfbeis, Mikrochim. Acta, 1997, 126, 177–192 CrossRef CAS.
- A. S. Amin, T. Y. Mohammed and A. A. Mousa, Spectrochim. Acta, Part A, 2003, 59, 2577–2584 CrossRef CAS.
- A. S. Amin and I. S. Ahmed, Anal. Lett., 2010, 43, 2598–2608 CrossRef CAS.
- A. S. Amin and I. A. Zaafarany, J. Taibah Univ. Sci., 2015, 9, 490–497 CrossRef.
- K. Maraenko, Spectrophotometric Determination of Elements, Wiley, New York, 1976, p. 441 Search PubMed.
- S. Moyano, R. G. Wuilloud, R. A. Olsina, J. A. Gasquez and L. D. Martinez, Talanta, 2001, 54, 211–219 CrossRef CAS.
- J. C. Crock, F. E. Lichte, G. O. Riddle and C. L. Beech, Talanta, 1986, 33, 601–606 CrossRef CAS PubMed.
- G. J. Mohr, Polymers for optical sensors, ed. F. Baldini, A. N. Chester, J. Homola and S. Martellucci, Springer, The Netherland, 2004, pp. 297–322.Optical chemical sensor Search PubMed.
- S. L. Hsu, Poly(methyl methacrylate), in Polymer data handbook, ed. J. E. Mark, Oxford University Press, Inc., Oxford, 1999, pp. 655–657 Search PubMed.
- C. Mc. Donagh, C. S. Burke and B. D. MacCraith, Chem. Rev., 2008, 108, 400–422 CrossRef.
- IUPAC, Spectrochim. Acta, Part B, 1978, 33, 241–245 CrossRef.
- P. T. Normann, P. Joff, I. Martinsen and H. S. Thomsen, J. Pharm. Biomed. Anal., 2000, 22, 939–947 CrossRef CAS.
- J. N. Miller and J. C. Miller, Statistics and Chemometrics for Analytical Chemistry, Prentice-Hall, London, 5th edn, 2005 Search PubMed.
|
This journal is © The Royal Society of Chemistry 2022 |
Click here to see how this site uses Cookies. View our privacy policy here.