DOI:
10.1039/D2RA04631H
(Paper)
RSC Adv., 2022,
12, 34730-34739
Acidic tributyl phosphonium-based ionic liquid: an efficient catalyst for preparation of diverse pyridine systems via a cooperative vinylogous anomeric-based oxidation†
Received
25th July 2022
, Accepted 17th November 2022
First published on 6th December 2022
Abstract
In this study, an acidic phosphonium-based ionic liquid, namely tributyl(3-sulfopropyl)phosphonium trifluoroacetate, was designed and synthesized via a facile and green route. From an accurate perspective, the structure of the prepared ionic liquid was investigated using FT-IR, 1H NMR and 13C NMR spectroscopies, and EDX, elemental mapping and TGA/DTG analysis. In this intensive research, catalytic application of tributyl(3-sulfopropyl)phosphonium trifluoroacetate was explored for the preparation of diverse pyridine systems such as triaryl pyridines, 2-amino-3-cyanopyridines and indolyl pyridines via a cooperative vinylogous anomeric-based oxidation (CVABO). The observed results proved that prepared acidic phosphonium-based ionic liquid is a effective catalyst for the multicomponent synthesis of pyridines.
Introduction
Ionic liquids (ILs), as remarkable class of chemical compounds, have attracted a tremendous deal of interest.1–3 Many of researchers in modern sciences are engaged in exploration of the exceptional properties of these versatile systems.4,5 These materials, due to irreplaceable privileges such as suitable chemical and thermal stability, controlled miscibility, tunability, designability, low vapor pressure, high ionic conductivity, etc., are an integral part of catalysis, biotechnology, medical sciences, energetic materials, separation and extraction processes, electrochemistry, surfactants and functional materials.2,6–12 In subsequent and intensive study, acidic ILs have been investigated in catalytic processes such as multicomponent reactions and CO2 fixation, biomass processing, and electrolytes.13,14
The cationic section of ILs plays a decisive role in viscosity, solubility, melting point, density and other characteristic properties of ionic liquids.15 Among varied type ILs, phosphonium-based ionic liquid (PILs) are more thermal and chemical stable and less viscous than other ILs.16,17 PILs due to environment compatibility, low cost and simplicity of synthesis, have an excellent potential for academic and industrial chemical processes.18–26 It goes without saying that there are several reports for variety applications of PILs. Wittig reagents,26 catalytic applications,27 desulfurization and denitrogenation of liquid fuels,18,28 extraction of phenolic compounds from water29 and recovery of metals30 are a selection of applications of PILs. In recent years, catalytic applications of PILs have received much attentions.16 These systems, have a good catalytic response to multicomponent reactions,16 CO and CO2 fixation,31–33 Mukaiyama-aldol reaction,33 hydroformylation acetalization34 and Heck reactions.35 A number of catalytic applications of PILs are shown in Scheme 1.
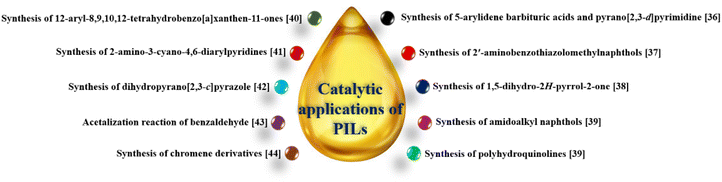 |
| Scheme 1 Catalytic applications of PILs in organic reactions. | |
Triaryl pyridines and nicotinonitriles as the most important subunits of pyridine families are ubiquitous subsets of pyridine families.36–40 Triaryl pyridines are popular building blocks in supramolecular chemistry,41 advanced devices as components of OLEDs,42 sensors43 and photo sensitizers44 and have good medicinal potentials such as antidepressant, anticancer and antimicrobial activities.45,46 Noticeably, nicotinonitrile derivatives by pharmaceutical and medicinal functions, have a crucial role and created new insights in the design of anticancer compounds, antihistaminic, antimicrobial and etc.47–49 Multicomponent strategy for the synthesis of pyridine families has a fundamental attitude of synthetic efficiency, utility high atom economy and selectivity.50–52 A number of pharmaceutical applications of triaryl pyridines and nicotinonitriles are sketched in Scheme 2.
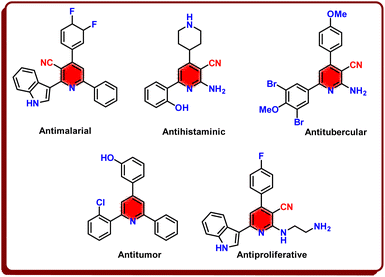 |
| Scheme 2 Selected pharmaceutical applications of triaryl pyridines and nicotinonitriles. | |
In this study, we devoted our focus on the design and synthesis of tributyl(3-sulfopropyl)phosphonium trifluoroacetate and its catalytic application in the preparation of triaryl pyridines, indolyl pyridines and nicotinonitriles (Schemes 3 and 4). In turn, a cooperative vinylogous anomeric-based oxidation was investigated to justify the mechanism of synthesis of pyridines.53–56
 |
| Scheme 3 Experimental routes for the synthesis of acidic tributyl phosphonium-based ionic liquid. | |
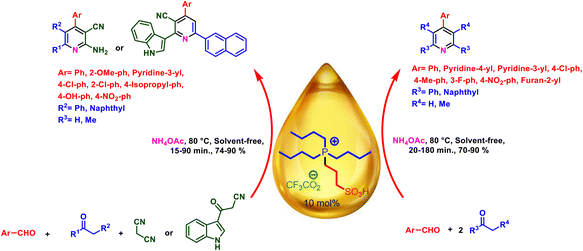 |
| Scheme 4 Experimental procedure for the synthesis of triaryl pyridines, indolyl pyridines and nicotinonitriles. | |
Results and discussion
As it is discussed in detail below, several techniques were applied for determination and characterization of the tributyl(3-sulfopropyl)phosphonium trifluoroacetate.
In FT-IR spectrum of PIL 1, emergence of broad pick at 3479 cm−1, indicates the acidic functional group and the stretching band of aliphatic C–H groups are observed at 2966 to 2882 cm−1. The characteristic peak at 1760 was confirmed the existence of carbonyl group of trifluoroacetate anion. The diagnostic stretching bond of S
O was appeared at 1158 cm−1. Also, 1H NMR and 13C NMR spectra are well consistent with the structure of the catalyst (see ESI†).
Elemental composition of target catalyst was evaluated by using EDX analysis. According to the observed results, the expected elements in the structure of tributyl(3-sulfopropyl)phosphonium trifluoroacetate including C, S, P, O and F are approved (Fig. 1). In addition, elemental mapping analysis was used for further confirmation of the obtained data from EDX analysis. Also, due to this technique, scattering of elements are well observed (Fig. 2).
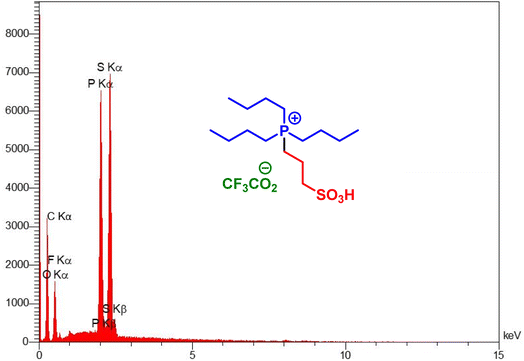 |
| Fig. 1 EDX analysis of PIL 1. | |
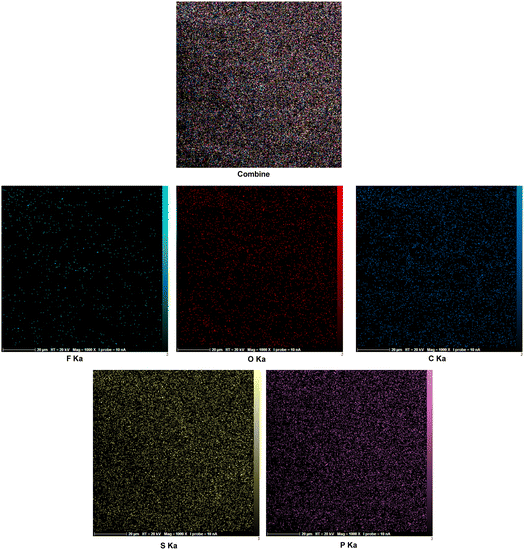 |
| Fig. 2 Elemental mapping analysis of PIL 1. | |
Thermogravimetric/differential thermogravimetric analysis displayed that PIL 1 was stable up to 223 °C. Fiddling weight loss up to 163 °C is related to the separation of trapped solvents. Also, it seems that the two extreme weight loss respectively in 223 and 416 °C which indicate the decomposition of –(CH2)3–SO3H and butyl chains of the catalyst57 (Fig. 3).
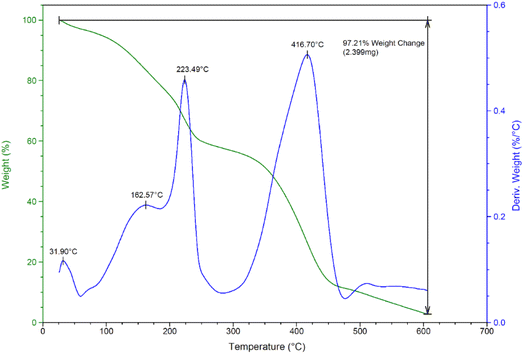 |
| Fig. 3 TGA/DTG curves of PIL 1. | |
In a deliberate study, after characterization of PIL 1, its catalytic application was investigated for the synthesis of diverse pyridine systems. In this respect, 4-chlorobenzaldehyde, 1-(naphthalen-2-yl)ethan-1-one, malononitrile and ammonium acetate as typical compounds were selected for the optimization of the reaction conditions. Initially, the role of temperature was examined and several temperatures consist of 40, 60, 70, 80 and 90 °C were exerted for the model reaction. The obtained experimental data proved that 80 °C is the best operational temperature. After that, the reaction was performed in the presence of different solvents including polar (protic and aprotic) and nonpolar solvents. The reaction has a substantial yield by using EtOH and CHCl3 as solvent. Then, the different amounts of catalyst were used in model reaction that due to the observed results, 10 mol% of catalyst gives the desired product by good yield. As confirmed by the obtained data, the best results for the model reaction achieved by the performing of the reaction in the presence of 10 mol% of catalyst and solvent free conditions at 80 °C (Table 1).
Table 1 Optimizing of the reaction conditions for the synthesis of 1ja
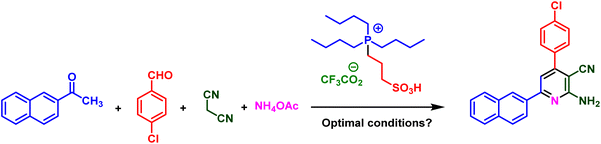
|
Entry |
Solvent |
Temperature (°C) |
Catalyst loading (mol%) |
Time (min) |
Yieldb (%) |
Reaction conditions: 4-chlorobenzaldehyde (1 mmol, 0.140 g), 1-(naphthalen-2-yl)ethan-1-one (1 mmol, 0.170 g), malononitrile (1 mmol, 0.066 g) and ammonium acetate (1 mmol, 0.078 g). Related to isolated yields. Data for the model reaction. |
1c |
— |
80 |
10 |
15 |
85 |
2 |
— |
80 |
20 |
15 |
85 |
3 |
— |
80 |
40 |
15 |
75 |
4 |
— |
80 |
— |
60 |
45 |
5 |
— |
90 |
10 |
10 |
85 |
6 |
— |
70 |
10 |
45 |
62 |
7 |
— |
60 |
10 |
45 |
56 |
8 |
— |
40 |
10 |
60 |
Trace |
9 |
— |
Room temperature |
10 |
120 |
Trace |
11 |
H2O |
Reflux |
20 |
60 |
57 |
12 |
EtOH |
Reflux |
20 |
60 |
85 |
13 |
n-Hexane |
Reflux |
20 |
60 |
75 |
14 |
EtOAc |
Reflux |
20 |
60 |
Trace |
15 |
CHCl3 |
Reflux |
20 |
60 |
80 |
In a discrete study, to expand the substrate scope for the desired molecules, different electron-poor or electron-rich aromatic aldehydes, aromatic ketones, malononitrile or 3-(1H-indol-3-yl)-3-oxopropanenitrile have been used. By using malononitrile or 3-(1H-indol-3-yl)-3-oxopropanenitrile as starting materials nicotinonitrile derivatives were successfully synthesized (Table 2) and in the same conditions without malononitrile or 3-(1H-indol-3-yl)-3-oxopropanenitrile and by using 2 equivalents of ketones triaryl pyridine derivatives were achieved (Table 3).
Table 2 Synthesis of nicotinonitrile derivatives in the presence of PIL 1 as catalysta
Reaction conditions: 1-(naphthalen-2-yl)ethan-1-one (1 mmol, 0.170 g), malononitrile (1 mmol, 0.066 g) or 3-(1H-indol-3-yl)-3-oxopropanenitrile (1 mmol, 0.184), ammonium acetate (1 mmol, 0.078 g), aromatic aldehyde (1 mmol), catalyst (10 mol%), solvent-free, 80 °C. |
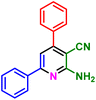 |
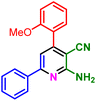 |
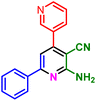 |
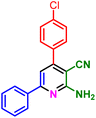 |
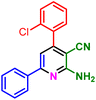 |
1a, 30 min, 80% |
1b, 15 min, 85% |
1c, 30 min, 80% |
1d, 80 min, 85% |
1e, 90 min, 88% |
MP: 179–181 °C |
MP: 195–197 °C |
MP: 199–202 °C |
MP: 198–202 °C |
MP: 184–186 °C |
[180]56 |
[198–201]58 |
[206–210]59 |
[188–190]58 |
[192]56 |
White solid |
White solid |
Yellow solid |
White solid |
White solid |
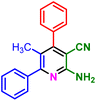 |
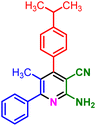 |
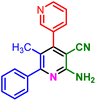 |
 |
|
1f, 25 min, 75% |
1g, 15 min, 75% |
1h, 25 min, 82% |
1i, 15 min, 80% |
|
MP: 198–202 °C |
MP: 239–241 °C |
MP: 221–223 °C |
MP: 265–267 °C |
|
[205]56 |
[New] |
[>300]56 |
[New] |
|
White solid |
White solid |
Yellow solid |
White solid |
|
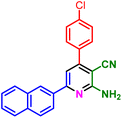 |
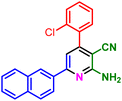 |
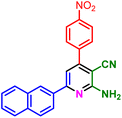 |
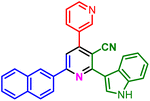 |
|
1j, 15 min, 85% |
1k, 15 min, 75% |
1l, 20 min, 90% |
1m, 45 min, 75% |
|
MP: 205–208 °C |
MP: 166–170 °C |
MP: 193–196 °C |
MP: >300 °C |
|
[218–220]59 |
[New] |
[204–206]60 |
[New] |
|
White solid |
White solid |
Brown solid |
Yellow solid |
|
Table 3 Synthesis of triaryl pyridines by using PIL 1 as a catalysta
Reaction conditions: 1-(naphthalen-2-yl)ethan-1-one (2 mmol, 0.340 g), ammonium acetate (1 mmol, 0.078 g), aromatic aldehyde (1 mmol), catalyst (10 mol%), solvent-free, 80 °C. |
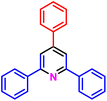 |
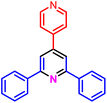 |
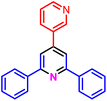 |
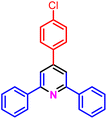 |
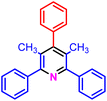 |
2a, 180 min, 75% |
2b, 30 min, 75% |
2c, 60 min, 70% |
2d, 180 min, 83% |
2e, 45 min, 70% |
MP: 117–120 °C |
MP: 232–236 °C |
MP: 140–143 °C |
MP: 112–116 °C |
MP: 125–127 °C |
[136–138]61 |
[187–190]62 |
[Not reported]63 |
[133–135]64 |
[130–133]65 |
White solid |
White solid |
White solid |
White solid |
White solid |
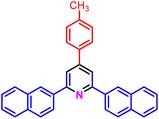 |
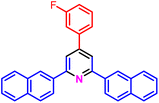 |
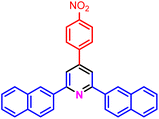 |
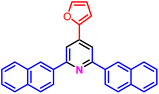 |
|
2f, 120 min, 75% |
2g, 120 min, 80% |
2i, 20 min, 90% |
2j, 30 min, 72% |
|
MP: 150–154 °C |
MP: 149–152 °C |
MP: 193–196 °C |
MP: 215–217 °C |
|
[Not reported]66 |
[New] |
[231–233]66 |
[220–221]67 |
|
White solid |
White solid |
Brown solid |
Brown solid |
|
The plausible mechanistic route for the synthesis of nicotinonitrile derivatives is revealed in Scheme 5. Firstly, activated 1-(naphthalen-2-yl)ethan-1-one by catalyst was reacted by ammonia (from NH4OAc) and leads to imine intermediate. Likewise, Knoevenagel intermediate was achieved by reaction of 4-chlorobenzaldehyde and malononitrile. In the second step, these two intermediates were reacted with each other to produce intermediate a. Then, through an intermolecular cyclization and subsequent tautomerization processes, the intermediate d was produced. After that, in the key step, with the participation of a cooperative vinylogous anomeric-based oxidation and releasing of H2O2 in air condition or releasing of H2 under inert atmosphere (N2 or argon), dihydropyridine structure is oxidized to target pyridine product.
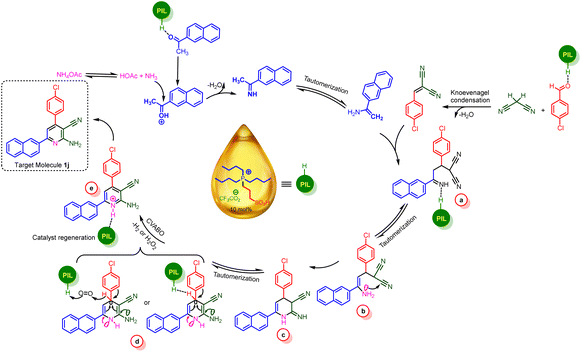 |
| Scheme 5 Plausible mechanism for the synthesis of 1j by using PIL 1 as catalyst. | |
Similarly, we suggested a plausible mechanism for the synthesis of triaryl pyridine systems (Scheme 6). At the first step, 1-(naphthalen-2-yl)ethan-1-one was activated by catalyst and converted to enolic form and reacted by 4-methylbenzaldehyde that leads to intermediate f. Then, due to Michael addition of enolic form of ketone to intermediate f, intermediate g was produced. In the next step, ammonia attacked to intermediate g and by removing one molecule of H2O and tautomerization processes, the enamine h is produced. After this, by intermolecular cyclization reaction and removing of H2O, dihydropyridine intermediate was achieved. Finally, CVABO leads to removing of H2O2 under air condition or H2 in inert atmosphere and desired target molecule was produced.
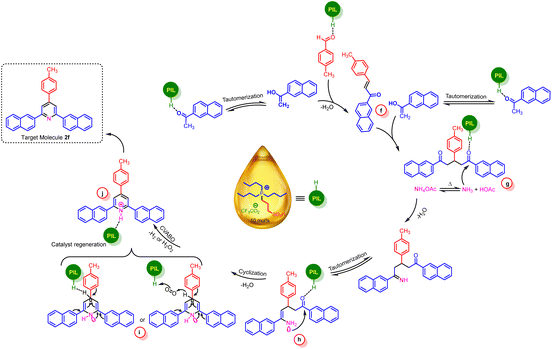 |
| Scheme 6 Plausible mechanism for the synthesis of 2f in the presence of PIL 1. | |
Experimental section
General experimental procedure for the synthesis of PIL 1
At the first, zwitterion 1 was synthesized according to the reported procedure.15 Hereupon, tributyl phosphine (5 mmol, 1.01 g), 1,2-oxathiolane 2,2-dioxide (5 mmol, 0.61 g) and acetonitrile as solvent were successively added into a 50 mL round-bottomed flask and refluxed under N2 atmosphere for 72 h. After completing of the reaction, the solvent was removed and the remained oil was washed with diethyl ether to gives the oily product. In the second step, zwitterion 1 (5 mmol, 1.62 g) and trifluoro acetic acid (5 mmol, 0.57 g) and 30 mL of toluene as catalyst were added into a 50 mL round-bottomed flask and the mixture of reaction was refluxed at 110 °C for 24 h. After that, the oily product was separated from solvent by using extraction with n-hexane to gives PIL 1 as desired product.
Experimental route for the synthesis of nicotinonitrile derivatives
Aromatic aldehyde derivatives (1 mmol), malononitrile (1 mmol, 0.066 g) or 3-(1H-indol-3-yl)-3-oxopropanenitrile (1 mmol, 0.184), 1-(naphthalen-2-yl)ethan-1-one (1 mmol, 0.170 g), ammonium acetate (1 mmol, 0.078 g) and 10 mol% of PIL 1 were charged into a 10 mL round-bottomed flask. The mixture of reaction was stirred at 80 °C. After completing of each of reactions as indicated by TLC technique (n-hexane/ethylacetate, 6/4), 10 mL of H2O was poured into the mixture of reaction to separate the catalyst. After that, hot EtOH was added to the mixture of reaction and remained solid washed three times and dried at 80 °C to give pure products.
General experimental procedure for the synthesis of triaryl pyridine derivatives
Aromatic aldehydes (1 mmol), 1-(naphthalen-2-yl)ethan-1-one (2 mmol, 0.340 g), ammonium acetate (1 mmol, 0.078 g) and 10 mol% of PIL 1 were added into a 10 mL round-bottomed flask and reacted at 80 °C. Then, reaction progress was checked by TLC techniques (n-hexane/ethylacetate, 6/4). After completing of reaction, 10 mL of H2O was added to the flask and the catalyst was separated. After that, the remained solid was washed with hot EtOH and dried at 80 °C to gives a pure product.
Spectral data
Tributyl(3-sulfopropyl)phosphonium trifluoroacetate PIL 1. FT-IR (KBr, ν, cm−1): 3479, 2966, 1760, 1467, 1157, 1038. 1H NMR (301 MHz, DMSOd6) δppm 5.04 (s, 1H), 2.61 (t, J = 7.0 Hz, 2H), 2.52–2.36 (m, 2H), 2.33–2.17 (m, 6H), 1.95–1.72 (m, 2H), 1.63–1.39 (m, 12H), 0.98 (t, J = 7.2 Hz, 9H). 13C NMR (76 MHz, DMSOd6) δppm 159.5, 159.0, 158.5, 158.0, 121.1, 117.3, 113.5, 109.7, 51.4, 23.8, 23.6, 23.1, 23.0, 18.1, 17.4, 13.4.
2-Amino-4-(2-methoxyphenyl)-6-phenylnicotinonitrile (1b). Mp = 195–197 °C. FT-IR (KBr, ν, cm−1): 3482, 3342, 2226, 1622, 1583. 1H NMR (301 MHz, DMSOd6) δppm 8.12–8.09 (m, 2H, aromatic), 7.51–7.49 (3, 4H, aromatic), 7.37 (dd, J = 9, 1.8 Hz, 1H, aromatic), 7.23–7.11 (m, 2H, aromatic), 7.14–7.08 (m, 1H, aromatic), 6.94 (s, 2H, NH2), 3.82 (s, 3H, CH3). 13C NMR (76 MHz, DMSOd6) δppm 160.6, 158.8, 156.5, 153.4, 138.1, 131.4, 130.5, 130.5, 129.2, 127.6, 126.6, 121.1, 117.2, 112.2, 110.7, 89.6, 56.0.
2′-Amino-6′-phenyl-[3,4′-bipyridine]-3′-carbonitrile (1c). Mp = 199–202 °C. FT-IR (KBr, ν, cm−1): 3465, 3311, 2205, 1635, 1582, 1483. 1H NMR (301 MHz, DMSOd6) δppm 8.90 (dd, J = 3, 0.9 Hz, 1H, aromatic), 8.76 (dd, J = 4.9, 1.6 Hz, 1H, aromatic), 8.19–8.13 (m, 3H, aromatic), 7.64–7.60 (m, 1H, aromatic), 7.53–7.51 (m, 3H, aromatic), 7.40 (s, 1H, aromatic), 7.16 (s, 2H, NH2). 13C NMR (76 MHz, DMSOd6) δppm 161.3, 159.4, 152.2, 150.9, 149.2, 137.9, 136.6, 133.3, 130.7, 129.2, 127.8, 124.1, 117.3, 109.8, 87.1.
2-Amino-4-(4-chlorophenyl)-6-phenylnicotinonitrile (1d). Mp = 198–202 °C. FT-IR (KBr, ν, cm−1): 3486, 3362, 2215, 1630, 2215, 1630, 1573, 1546. 1H NMR (301 MHz, DMSOd6) δppm 8.15 (dd, J = 6.7, 3.0 Hz, 2H, aromatic), 7.74 (d, J = 6 Hz, 2H, aromatic), 7.65 (d, J = 6 Hz, 2H, aromatic), 7.53–7.50 (m, 3H, aromatic), 7.31 (s, 1H, aromatic), 7.11 (s, 2H, NH2). 13C NMR (76 MHz, DMSOd6) δppm 161.3, 159.2, 154.1, 137.9, 136.3, 135.0, 130.8, 130.7, 129.3, 129.1, 127.8, 117.4, 109.6, 86.9.
2-Amino-4-(2-chlorophenyl)-6-phenylnicotinonitrile (1e). Mp = 184–186 °C. FT-IR (KBr, ν, cm−1): 3489, 3344, 2229, 1596, 1622, 1478. 1H NMR (301 MHz, DMSOd6) δppm 8.15–8.12 (m, 2H, aromatic), 7.69–7.66 (m, 1H, aromatic), 7.58–7.49 (m, 6H, aromatic), 7.24 (s, 1H, aromatic), 7.15 (s, 2H, NH2). 13C NMR (76 MHz, DMSOd6) δppm 160.6, 159.1, 153.6, 137.8, 136.7, 131.7, 131.4, 131.2, 130.7, 130.2, 129.2, 128.0, 127.7, 116.6, 110.2, 88.9.
2-Amino-5-methyl-4,6-diphenylnicotinonitrile (1f). Mp = 198–202 °C. 1H NMR (301 MHz, DMSOd6) δppm 7.56–7.38 (m, 10H, aromatic), 6.79 (s, 2H, NH2), 1.84 (s, 3H, CH3). 13C NMR (76 MHz, DMSOd6) δppm 161.9, 158.5, 155.7, 140.5, 137.6, 129.3, 129.2, 129.1, 128.9, 128.8, 128.4, 117.1, 117.1, 89.6, 17.2.
2-Amino-4-(4-isopropylphenyl)-5-methyl-6-phenylnicotinonitrile (1g). Mp = 239–241 °C. FT-IR (KBr, ν, cm−1): 3429, 3307, 2211, 1646, 1584. 1H NMR (301 MHz, DMSOd6) δppm 7.50–7.32 (m, 9H), 7.24–7.21 (m, 2H), 2.92 (hept, J = 7.0 Hz, 1H), 1.54 (s, 3H), 1.23 (d, J = 7.0 Hz, 6H). ESI-MS (m/z) = calcd for C22H21N3 (M+) 327.43, found: 328.024.
2′-Amino-5′-methyl-6′-phenyl-[3,4′-bipyridine]-3′-carbonitrile (1h). Mp = 221–223 °C. FT-IR (KBr, ν, cm−1): 3466, 3292, 2213, 1634, 1557, 1456. 1H NMR (301 MHz, DMSOd6) δppm 8.72 (dd, J = 6, 1.6 Hz, 1H, aromatic), 8.66 (d, J = 2.3, 1H, aromatic), 7.93 (dt, J = 9, 3 Hz, 1H, aromatic), 7.63–7.58 (m, 1H, aromatic), 7.56–7.45 (m, 5H, aromatic), 6.90 (s, 2H, NH2), 1.87 (s, 3H, CH3). 13C NMR (76 MHz, DMSOd6) δppm 162.2, 158.6, 152.3, 150.3, 149.2, 140.2, 136.8, 133.5, 129.3, 129.0, 128.5, 124.1, 117.4, 117.0, 89.5, 17.2.
2-Amino-4-(4-hydroxyphenyl)-6-(naphthalen-2-yl)nicotinonitrile (1i). Mp = 265–267 °C. FT-IR (KBr, ν, cm−1): 3474, 3383, 3055, 1638, 1571. 1H NMR (301 MHz, DMSOd6) δppm 10.02 (s, 1H, OH), 8.80–8.72 (m, 1H), 8.29 (dd, J = 8.7, 1.8 Hz, 1H), 8.07–7.97 (m, 3H), 7.63–7.58 (m, 4H), 7.44 (s, 1H), 6.99–6.95 (m, 4H). 13C NMR (76 MHz, DMSOd6) δppm 161.5, 159.4, 158.68, 155.3, 135.6, 134.1, 133.3, 130.4, 129.2, 128.5, 128.0, 128.0, 127.64, 127.47, 127.07, 125.03, 117.97, 115.99, 109.76, 86.78. ESI-MS (m/z) = calcd for C22H15N3O (M+) 337.38, found: 337.969.
2-Amino-4-(2-chlorophenyl)-6-(naphthalen-2-yl)nicotinonitrile (1k). Mp = 206–209 °C. FT-IR (KBr, ν, cm−1): 3493, 3439, 2222, 1622, 1596, 1551. 1H NMR (301 MHz, DMSOd6) δppm 8.76 (s, 1H), 8.31 (dd, J = 9, 3 Hz, 1H, aromatic), 8.06–7.97 (m, 3H, aromatic), 7.78 (d, J = 9 Hz, 2H), 7.67 (d, J = 6 Hz, 2H, aromatic), 7.61–7.58 (m, 2H, aromatic), 7.49 (s, 1H, aromatic), 7.16 (s, 2H, NH2). 13C NMR (76 MHz, DMSOd6) δppm 161.4, 159.1, 154.2, 136.3, 135.3, 135.0, 134.2, 133.3, 130.8, 129.3, 128.6, 128.1, 127.7, 127.7, 127.1, 125.0, 117.4, 110.0, 87.0. ESI-MS (m/z) = calcd for C22H14ClN3 (M+) 355.83, found: 355.918.
2′-(1H-Indol-3-yl)-6′-(naphthalen-2-yl)-[3,4′-bipyridine]-3′-carbonitrile (1m). Mp > 300 °C. FT-IR (KBr, ν, cm−1): 3443, 3133, 2223, 1592, 1567, 1531. 1H NMR (301 MHz, DMSOd6) δppm 11.91 (s, 1H), 8.98 (s, 1H), 8.54–8.49 (m, 2H), 8.44–8.43 (m, 1H), 8.17–8.15 (m, 2H), 8.13–8.09 (m, 1H), 8.05–8.01 (m, 1H), 7.89–7.86 (m, 2H), 7.66–7.60 (m, 5H), 7.31–7.28 (m, 2H). 13C NMR (76 MHz, DMSOd6) δppm 158.6, 157.9, 155.6, 137.4, 136.9, 135.4, 134.3, 133.5, 130.2, 129.5, 129.4, 129.2, 129.1, 128.1, 128.0, 127.3, 126.6, 124.9, 122.9, 121.8, 121.3, 119.4, 117.2, 113.5, 112.6, 102.0. (m/z) = calcd for C29H18N4 (M+) 422.49, found: 423.100.
2,4,6-Triphenylpyridine (2a). Mp = 117–120 °C. FT-IR (KBr, ν, cm−1): 3063, 1692, 1578, 1548, 1494. 1H NMR (301 MHz, DMSOd6) δppm 8.38–8.34 (m, 4H, aromatic), 8.20 (s, 2H, aromatic), 8.07–8.04 (m, 2H, aromatic), 7.62–7.47 (m, 9H, aromatic). 13C NMR (76 MHz, DMSOd6) δppm 157.0, 150.1, 139.3, 138.2, 129.8, 129.7, 129.6, 129.2, 127.8, 127.5, 117.1.
4-(4-Chlorophenyl)-2,6-diphenylpyridine (2d). Mp = 112–116 °C. FT-IR (KBr, ν, cm−1): 3067, 1601, 1544, 1491, 1384. 1H NMR (301 MHz, DMSOd6) δppm 8.37–8.33 (m, 4H, aromatic), 8.21 (s, 2H, aromatic), 8.11 (d, J = 9 Hz, 2H, aromatic), 7.64–7.49 (m, 8H). 13C NMR (76 MHz, DMSOd6) δppm 157.1, 148.7, 139.2, 137.0, 134.7, 129.8, 129.7, 129.5, 129.2, 127.5, 116.9.
2,6-Di(naphthalen-2-yl)-4-(p-tolyl)pyridine (2f). Mp = 150–154 °C. FT-IR (KBr, ν, cm−1): 2922, 1596, 1544, 818. 1H NMR (301 MHz, DMSOd6) δppm 8.98 (d, J = 1.7 Hz, 2H, aromatic), 8.61 (dd, J = 9, 1.8 Hz, 2H, aromatic), 8.42 (s, 2H, aromatic), 8.18–8.01 (m, 8H, aromatic), 7.64–7.60 (m, 4H, aromatic), 7.45 (d, J = 6 Hz, 2H, aromatic), 2.45 (s, 3H, CH3). 13C NMR (76 MHz, DMSOd6) δppm 157.0, 150.0, 139.6, 136.8, 135.2, 133.9, 133.7, 130.2, 129.2, 128.7, 128.1, 127.7, 127.2, 126.9, 126.8, 125.3, 117.1, 21.4.
4-(3-Fluorophenyl)-2,6-di(naphthalen-2-yl)pyridine (2g). Mp = 149–152 °C. FT-IR (KBr, ν, cm−1): 3057, 1598, 1551, 1399, 1183. 1H NMR (301 MHz, DMSOd6) δppm 9.00 (s, 2H), 8.62 (d, J = 8.9 Hz, 2H), 8.47 (s, 2H), 8.17–8.02 (m, 8H), 7.69–7.61 (m, 5H), 7.43 (d, J = 9.0 Hz, 1H). 13C NMR (76 MHz, DMSOd6) δppm 157.1, 148.8, 140.6, 136.5, 133.9, 133.6, 131.6, 131.5, 129.2, 128.7, 128.1, 127.3, 127.0, 126.9, 125.3, 124.1, 117.5, 116.7, 116.5, 115.0, 114.7.
Conclusion
In conclusion, we reported the tributyl(3-sulfopropyl)phosphonium trifluoroacetate as a new phosphonium-based ionic liquid with acidic moiety. The prepared ionic liquid was characterized by using FT-IR, 1H NMR and 13C NMR spectroscopies, EDX, elemental mapping and TGA/DTG analysis. After that, PIL 1 has been shown good potential as an efficient catalyst for the multicomponent synthesis of triaryl pyridines, 2-amino-3-cyanopyridines and indolyl pyridine. In this work, we used diverse starting materials such as different ketones and aldehydes that leads to formation of varied pyridine systems. Moreover, most of synthesized pyridine derivatives have a relatively good yields and short reaction times. In a separate study, a plausible mechanism based on a cooperative vinylogous anomeric-based oxidation was suggested for the synthesis of pyridine derivatives.
Conflicts of interest
There are no conflicts to declare.
Acknowledgements
We thank the Bu-Ali Sina University and Iran National Science Foundation [(INSF), Grant Number: 98001912] for financial support to our research group.
References
- S. Zhang, J. Zhang, Y. Zhang and Y. Deng, Chem. Rev., 2017, 117, 6755 CrossRef CAS.
- K. S. Egorova, E. G. Gordeev and V. P. Ananikov, Chem. Rev., 2017, 117, 7132 CrossRef CAS.
- B. Wang, L. Qin, T. Mu, Z. Xue and G. Gao, Chem. Rev., 2017, 117, 7113 CrossRef CAS.
- Z. Lei, B. Chen, Y. M. Koo and D. R. MacFarlane, Chem. Rev., 2017, 117, 6633 CrossRef.
- F. Ren, J. Wang, F. Xie, K. Zan, S. Wang and S. Wang, Green Chem., 2020, 22, 2162 RSC.
- Y. Tao, R. Dong, I. V. Pavlidis, B. Chen and T. Tan, Green Chem., 2016, 18, 1240 RSC.
- E. Sebastiao, C. Cook, A. Hu and M. Murugesu, J. Mater. Chem. A, 2014, 2, 8153 RSC.
- S. K. Singh and A. W. Savoy, J. Mol. Liq., 2020, 297, 112038 CrossRef CAS.
- P. Sun, S. Zhang, J. Pang, Y. Tan, D. Sun, C. Xia, X. Cheng and X. Xin, J. Mol. Liq., 2018, 272, 180 CrossRef CAS.
- G. R. Zhang and B. J. Etzold, J. Energy Chem., 2016, 25, 199 CrossRef.
- A. J. Greer, J. Jacquemin and C. Hardacre, Molecules, 2020, 25, 5207 CrossRef CAS.
-
(a) M. Kharazi, J. Saien, M. Yarie and M. A. Zolfigol, J. Pet. Res., 2021, 31, 113 Search PubMed;
(b) M. Kharazi, J. Saien, M. Yarie and M. A. Zolfigol, J. Pet. Sci. Eng., 2020, 195, 107543 CrossRef CAS;
(c) M. Kharazi, J. Saien, M. Yarie and M. A. Zolfigol, J. Mol. Liq., 2019, 296, 111748 CrossRef CAS;
(d) J. Saien, M. Kharazi, M. Yarie and M. A. Zolfigol, Ind. Eng. Chem. Res., 2019, 58, 3583 CrossRef CAS.
- A. S. Amarasekara, Chem. Rev., 2016, 116, 6133 CrossRef CAS.
- T. Wang, D. Zheng, J. Zhang, B. Fan, Y. Ma, T. Ren, L. Wang and J. Zhang, ACS Sustainable Chem. Eng., 2018, 6, 2574 CrossRef CAS.
-
(a) D. Dupont, S. Raiguel and K. Binnemans, Chem. Commun., 2015, 51, 9006–9009 RSC;
(b) M. Chen, B. T. White, C. R. Kasprzak and T. E. Long, Eur. Polym. J., 2018, 108, 28 CrossRef CAS.
- S. Khazalpour, M. Yarie, E. Kianpour, A. Amani, S. Asadabadi, J. Y. Seyf, M. Rezaeivala, S. Azizian and M. A. Zolfigol, J. Iran. Chem. Soc., 2020, 17, 1775 CrossRef CAS.
- D. Wei-Li, J. Bi, L. Sheng-Lian, L. Xu-Biao, T. Xin-Man and A. Chak-Tong, Appl. Catal., A, 2014, 470, 183 CrossRef.
- E. Kianpour, S. Azizian, M. Yarie, M. A. Zolfigol and M. Bayat, Chem. Eng. J., 2016, 295, 500 CrossRef CAS.
- N. Noroozi-Shad, M. Gholizadeh and H. Sabet-Sarvestani, J. Mol. Struct., 2022, 1257, 132628 CrossRef CAS.
- H. Shirota, K. Takahashi, M. Ando and S. Kakinuma, J. Chem. Eng. Data, 2019, 64, 4701 CrossRef CAS.
- K. J. Fraser and D. R. MacFarlane, Aust. J. Chem., 2009, 62, 309 CrossRef CAS.
- J. Chen, M. Li, M. Li, X. Lin and T. Qiu, ACS Sustainable Chem. Eng., 2020, 8, 6956 CrossRef CAS.
- M. Stalpaert, N. Peeters and D. De Vos, Catal. Sci. Technol., 2018, 8, 1468 RSC.
- A. H. T. Hang, T. L. H. Nguyen, D. K. N. Chau and P. H. Tran, RSC Adv., 2018, 8, 11834 RSC.
- M. Chen, B. T. White, C. R. Kasprzak and T. E. Long, Eur. Polym. J., 2018, 108, 28 CrossRef CAS.
-
(a) H. B. Phan, Q. B. T. Nguyen, C. M. Luong, K. N. Tran and P. H. Tran, Mol. Catal., 2021, 503, 111428 Search PubMed;
(b) H. B. Phan, C. M. Luong, L. P. Nguyen, B. T. Bui, H. T. Nguyen, B. V. Mai and P. H. Tran, ACS Sustainable Chem. Eng., 2022, 10, 8673 CrossRef CAS;
(c) D. S. Firaha, A. V. Gibalova and O. Holloczki, ACS Omega, 2017, 2, 2901 CrossRef CAS PubMed.
- M. Stalpaert, K. Janssens, C. Marquez, M. Henrion, A. L. Bugaev, A. V. Soldatov and D. De Vos, ACS Catal., 2020, 10, 9401 CrossRef CAS.
-
(a) E. Mosayebi, S. Azizian, M. Yarie and M. A. Zolfigol, J. Mol. Liq., 2021, 326, 115326 Search PubMed;
(b) F. R. Moghadam, E. Kianpour, S. Azizian, M. Yarie and M. A. Zolfigol, R. Soc. Open Sci., 2020, 7, 200803 CrossRef CAS;
(c) F. R. Oghadam, S. Azizian, M. Bayat, M. Yarie, E. Kianpour and M. A. Zolfigol, Fuel, 2017, 208, 214 CrossRef;
(d) F. R. Moghadam, S. Azizian, E. Kianpour, M. Yarie, M. Bayat and M. A. Zolfigol, Chem. Eng. J., 2017, 309, 480 Search PubMed.
- E. Skoronski, M. Fernandes, F. J. Malaret and J. P. Hallett, Sep. Purif. Technol., 2020, 248, 117069 Search PubMed.
- H. Mahandra, F. Faraji and A. Ghahreman, Sustain. Chem. Eng., 2021, 9, 8179 Search PubMed.
- J. Steinbauer, C. Kubis, R. Ludwig and T. Werner, ACS Sustainable Chem. Eng., 2018, 6, 10778 CrossRef CAS.
- F. Liu, Y. Gu, H. Xin, P. Zhao, J. Gao and M. Liu, ACS Sustainable Chem. Eng., 2019, 7, 16674 Search PubMed.
- T. Werner, Adv. Syn. Catal., 2009, 351, 1469 Search PubMed.
- P. Wang, X. Chen, D. L. Wang, Y. Q. Li and Y. Liu, Green Energy Environ., 2017, 2, 419 Search PubMed.
- D. Rauber, F. Philippi and R. Hempelmann, J. Fluorine Chem., 2017, 200, 115 CrossRef CAS.
- C. Allais, J. M. Grassot, J. Rodriguez and T. Constantieux, Chem. Rev., 2014, 114, 10829 CrossRef CAS.
- J. A. Bull, J. J. Mousseau, G. Pelletier and A. B. Charette, Chem. Rev., 2012, 112, 2642 Search PubMed.
- J. S. Carey, D. Laffan, C. Thomson and M. T. Williams, Org. Biomol. Chem., 2006, 4, 2337 Search PubMed.
- R. S. Rohokale, B. Koenig and D. D. Dhavale, J. Org. Chem., 2016, 81, 7121 CrossRef CAS PubMed.
- M. Torabi, M. Yarie, S. Baghery and M. A. Zolfigol, Recent advances in catalytic synthesis of pyridine derivatives, Elsevier Inc., 2023, pp. 503–580, ISBN: 978-0-323-91221-1 Search PubMed.
- Z. Zarnegar, J. Safari and M. Borjian-Borujeni, Chem. Heterocycl. Compd., 2015, 50, 1683 CrossRef CAS.
- L. F. B. Fontes, R. N. da Silva, A. M. S. Silva and S. Guieu, ChemPhotoChem, 2020, 4, 5312 Search PubMed.
- A. G. Fang, J. V. Mello and N. S. Finney, Tetrahedron, 2004, 60, 11075 Search PubMed.
- M. K. Dhinakaran and T. M. Das, Org. Biomol. Chem., 2012, 10, 2077 RSC.
- D. Ashok, M. R. Reddy, R. Dharavath, N. Nagaraju, K. Ramakrishna, S. Gundu and M. Sarasija, J. Chem. Sci., 2021, 133, 1 CrossRef.
- P. Thapa, R. Karki, M. Yun, T. M. Kadayat, E. Lee, H. B. Kwon, Y. Na, W. J. Cho, N. D. Kim, B. S. Jeong, Y. Kwon and E. S. Lee, Eur. J. Med. Chem., 2012, 52, 123 Search PubMed.
- H. Tao, J. Wang, W. Lu, R. Zhang, Y. Xie, Y. C. Liu, R. Liu, L. Yue, K. Chen, H. Jiang, Y. Zhang, X. Xu and C. Luo, RSC Adv., 2019, 9, 4917 RSC.
- C. S. Challa, N. K. Katari, V. Nallanchakravarthula, D. Nayakanti, R. Kapavarapu and M. Pal, J. Mol. Struct., 2021, 1240, 130541 Search PubMed.
- S. B. Goud, Org. Biomol. Chem., 2022, 20, 352 RSC.
- B. H. Rotstein, S. Zaretsky, V. Rai and A. K. Yudin, Chem. Rev., 2014, 114, 8323 CrossRef CAS PubMed.
- T. M. Shaikh, S. Nagarajan and E. Kandasamy, Curr. Organocatal., 2020, 7, 96 Search PubMed.
- B. B. Tour and D. G. Hall, Chem. Rev., 2009, 109, 4439 CrossRef PubMed.
-
(a) F. Karimi, M. Yarie and M. A. Zolfigol, Mol. Catal., 2020, 489, 110924 Search PubMed;
(b) M. Torabi, M. Yarie, M. A. Zolfigol, S. Azizian and Y. Gu, RSC Adv., 2022, 12, 8804 RSC;
(c) P. Ghasemi, M. Yarie, M. A. Zolfigol, A. Taherpour and M. Torabi, ACS Omega, 2020, 5, 3207 Search PubMed;
(d) F. Karimi, M. Yarie and M. A. Zolfigol, RSC Adv., 2020, 10, 25828 Search PubMed;
(e) M. Dashteh, M. A. Zolfigol, A. Khazaei, S. Baghery, M. Yarie, S. Makhdoomi and M. Safaiee, RSC Adv., 2020, 10, 27824 RSC;
(f) F. Karimi, M. Yarie and M. A. Zolfigol, Mol. Catal., 2019, 463, 20 Search PubMed;
(g) M. Torabi, M. Yarie, M. A. Zolfigol, S. Rouhani, S. Azizi, T. O. Olomola, M. Maaza and T. A. M. Msagati, RSC Adv., 2020, 11, 3143 RSC;
(h) N. Zarei, M. Torabi, M. Yarie and M. A. Zolfigol, Polycyclic Aromat. Compd., 2022, 1 DOI:10.1080/10406638.2022.2061531.
-
(a) M. Yarie, Iran. J. Catal., 2017, 7, 85 Search PubMed;
(b) M. Yarie, Iran. J. Catal., 2020, 10, 79 CAS;
(c) M. Torabi, M. A. Zolfigol, M. Yarie and Y. Gu, Mol. Catal., 2021, 516, 110959 Search PubMed;
(d) F. Jalili, M. Zarei, M. A. Zolfigol, S. Rostamnia and A. R. Moosavi-Zare, Microporous Mesoporous Mater., 2020, 294, 109865 Search PubMed;
(e) S. Kalhor, M. Yarie, M. Torabi, M. A. Zolfigol, M. Rezaeivala and Y. Gu, Polycyclic Aromat. Compd., 2021, 11, 17456 Search PubMed;
(f) M. Torabi, M. A. Zolfigol, M. Yarie, B. Notash, S. Azizian and M. M. Azandaryani, Sci. Rep., 2021, 11, 16846 Search PubMed.
-
(a) I. V. Alabugin, L. Kuhn, M. G. Medvedev, N. V. Krivoshchapov, V. A. Vil, I. A. Yaremenko, P. Mehaffy, M. Yarie, A. O. Terent'ev and M. A. Zolfigol, Chem. Soc. Rev., 2021, 50, 10253 Search PubMed;
(b) I. V. Alabugin, L. Kuhn, N. V. Krivoshchapov, P. Mehaffy and M. G. Medvedev, Chem. Soc. Rev., 2021, 50, 10212 RSC.
- M. Rahmati and D. Habibi, Res. Chem. Intermed., 2021, 47, 1643 Search PubMed.
- M. Yarie, M. A. Zolfigol and M. Saeidi-Rad, J. Mol. Liq., 2018, 249, 144 Search PubMed.
- G. M. Ziarani and S. Bahar, J. Iran. Chem. Soc., 2019, 16, 365 CrossRef.
- F. Tamaddon, S. Ghazi and M. R. Noorbala, J. Mol. Catal. B: Enzym., 2016, 127, 89 Search PubMed.
- M. Ashouri, H. Kefayati and S. Shariati, J. Chin. Chem. Soc., 2019, 66, 355 CrossRef CAS.
- M. A. Zolfigol, F. Karimi, M. Yarie and M. Torabi, Appl. Organomet. Chem., 2018, 32, e4063 CrossRef.
- H. Alinezhad, M. Tajbakhsh and N. Ghobadi, Res. Chem. Intermed., 2015, 41, 9113 Search PubMed.
- H. Huang, X. Ji, W. Wu, L. Huang and H. Jiang, J. Org. Chem., 2013, 78, 3774 Search PubMed.
- J. Han, X. Guo, Y. Liu, Y. Fu, R. Yan and B. Chen, Adv. Syn. Catal., 2017, 359, 2676 CrossRef CAS.
- X. Zhang, Z. Wang, K. Xu, Y. Feng, W. Zhao, X. Xu, Y. Yan and W. Yi, Green Chem., 2016, 18, 2313 RSC.
- H. Behmadi, S. Naderipour, S. M. Saadati, M. Barghamadi, M. Shaker and N. Tavakoli-Hoseini, J. Heterocycl. Chem., 2011, 48, 1117 Search PubMed.
- V. G. Kharchenko, N. M. Kupranets and M. N. Tilichenko, Chem. Heterocycl., 1970, 4, 10 CrossRef.
|
This journal is © The Royal Society of Chemistry 2022 |
Click here to see how this site uses Cookies. View our privacy policy here.