DOI:
10.1039/D2RA05339J
(Paper)
RSC Adv., 2022,
12, 28490-28493
[3+2] click chemistry approach to tetrazine containing polymers†
Received
25th August 2022
, Accepted 27th September 2022
First published on 6th October 2022
Abstract
We report a [3+2] cycloaddition using 3,6-bis-propargyloxy-1,2,4,5-tetrazine and azides to synthesize energetic polymers containing 1,2,4,5-tetrazine within the scaffold. This work also includes [3+2] cycloaddition to crosslink azide containing glycidyl azide polymer (GAP). These reactions provide pathways for incorporation of 1,2,4,5-tetrazine into novel energetic materials using click-chemistry and provide an alternative polymer curing approach.
Due to their versatility and unique chemical and physical properties, tetrazines have found utility in organic solar cells,1,2 sensors,3,4 on/off fluorescence5–8 and energetic materials applications.9,10 A significant increase in 1,2,4,5-tetrazine chemistry synthesis has also occurred over the past several years.11–14 A number of investigations have focused in large part on bioorthogonal, inverse electron demand hetero-Diels–Alder reactions with 1,2,4,5-tetrazines for numerous applications, such as in situ synthesis of fluorogenic probes for live cell imaging, and near infrared fluorogenic probes.8 Unfortunately, the inverse electron demand hetero-Diels–Alder reaction of 1,2,4,5-tetrazines is not a promising pathway in energetic materials chemistry as a concomitant reduction in the inherent energy of the molecule results during these types of transformations due to the loss of N2. Thus alternative pathways for the incorporation of 1,2,4,5-tetrazine into novel energetic materials are warranted.
One very interesting possibility for the preparation of new energetics is to employ a [3+2] cycloaddition (click-chemistry) strategy. In this case, a Huisgen 1,3-dipolar cycloaddition between 1,3-dipoles such as azides, and 1,2-dipolarophiles such as acetylenes could be incorporated onto the tetrazine ring system at the 3 and 6 positions either directly or through linkers of varying length. The challenge presented with this approach is the possibility for the 1,2,4,5-tetrazine system to undergo competitive inverse electron demand [4+2] hetero-Diels–Alder chemistry with acetylene functional groups. Indeed, Rusinov and co-workers found that the reaction of but-3-yn-1-ol with 3,6-bis(3,5-dimethylpyrazol-1-yl)-1,2,4,5-tetrazine (1) in the presence of triethylamine in acetonitrile led to the two products 2 and 3 (5
:
1) as shown in Scheme 1.15 These two products both are a result of inverse electron demand hetero-Diels–Alder processes. Changing the leaving group from 3,5-dimethylpyrazole to imidazole led to the exclusive isolation of the bicyclic product 2.
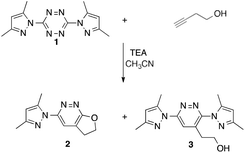 |
| Scheme 1 But-3-yn-1-ol reaction with 1 in the presence of TEA. | |
While these literature results were not encouraging, we decided to pursue the synthesis of 3,6-bispropargyloxy-1,2,4,5-tetrazine (4). We found that shortening the distance between the alcohol group and the acetylene moiety allowed for nucleophilic addition to proceed exclusively with no evidence of inter- or intramolecular hetero-Diels–Alder cyclization occurring. Thus propargyl alcohol reacted with 1 in the presence of potassium carbonate to provide 4 in excellent yield (Scheme 2). Ultimately, we were able to perform the reaction neat, using propargyl alcohol as the solvent, although only in minimal amounts.
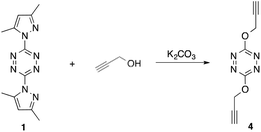 |
| Scheme 2 The synthesis of 4. | |
To confirm the structure of 4, X-ray quality crystals were obtained from slow diffusion of diethyl ether into a solution of 4 in acetone. The compound was found to have the monoclinic crystal system with a P21/c space group.7 It displayed a density of 1.46 g cm−3 at 293 K (see ESI†). The Ortep representation of the crystal structure is displayed in Fig. 1. Overall, the transformation resulting in 4 is unprecedented and allowed for a “click” chemical approach to make energetic, tetrazine containing polymers.
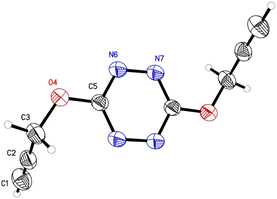 |
| Fig. 1 Molecular conformation of 4, nonhydrogen atoms are shown as 50% probability displacement ellipsoids. Ellipsoids of 4 shown at 50% probability. | |
With 4 in hand, we attempted to perform the Huisgen cycloaddition reaction with azide containing materials employing the Cu(I)-catalyzed azide/alkyne cycloaddition approach (CuAAC) to accomplish prepolymer curing, polymerization and self-polymerization.8 We first investigated a [3+2] cross-linking strategy using 4 as a curing agent for azide containing prepolymers, such as the glycidyl azide (GAP) prepolymer. A similar approach has been described with other polyacetylene compounds.9 Typically GAP is cured using a urethane crosslinking approach, but this method presents challenges, such as sensitivity to moisture and the use of reactive isocyanates. A [3+2] curing approach with a high energy cross linker could provide higher energy GAP polymers. Initial results found that 5 wt% 4 was successful at curing the GAP prepolymer (5) in the presence of a catalytic amount of CuCl, to produce polymer 6 (Scheme 3).
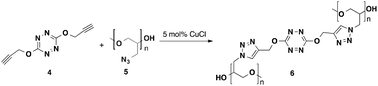 |
| Scheme 3 Curing of glycidyl azide prepolymer. | |
These results were expanded on to include a curing study of GAP (5) and GAP plasticizer (GAPp, 5p) with varying weight percentages of 4 in the presence of a copper halide species to produce polymer 6 and 6p, respectively. The GAP (also called GAP 5527-Polyol) is a high molecular weight prepolymer with a hydroxyl equivalent between 2.5–3 used in urethane cures, while the GAPp is a lower viscosity prepolymer with an average MW of 700, and a hydroxyl equivalent close to 1. As the click-chemistry cure for these GAP pre-polymers is independent of hydroxyl terminal group content, the two materials were utilized to evaluate rheological properties over the course of reaction. Rheometry measurements were conducted on samples containing 3% of 4 for both polymers (see ESI†). The transition from liquid to solid, or gel point, for 6 occurred at roughly 83 minutes compared to 6p at about 4 hours.
After demonstrating that 4 could be used to cure GAP, we turned our attention to polymerization studies of 4 with diazide compounds. One such material was 2,2-bisazidomethyl-1,3-propanediol (7).16 Since 7 is a liquid, we performed the cycloaddition neat by thoroughly mixing 4, 7 with a catalytic amount of a copper halide (CuCl or CuI). Heating for several hours at 60 °C led to the polymerized product (8) (Scheme 4). Similarly, when 4 was reacted with 2-hydroxy-1,3-diazidopropane (9)17,18 in the presence of a catalytic amount of CuCl, the desired polymerization product (10) was observed (Scheme 4).
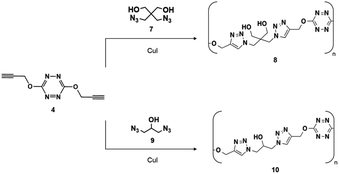 |
| Scheme 4 Polymerization of 4 with diazides. | |
Polymers 8 and 10 were found to have molecular weights of 3602 ± 136.71 g mol−1 and 6796 ± 132.17 g mol−1, respectively. As expected, Fourier transform infrared spectroscopy (FT-IR) comparisons of 4 to both polymers showed significant reduction in the intensity of the terminal acetylene stretch at 3261 cm−1 (C–H) and 2126 cm−1 (C
C) (see ESI†).
Further studies for single compound self-polymerization were explored by incorporation of the azide group and an acetylene group on the same tetrazine ring. We began with the reaction of propargyl alcohol with 3,6-dichloro-1,2,4,5-tetrazine (11) in the presence of 2,4,6-collidine, using tert-butyl methyl ether as a solvent. This provided 12 in good yield (Scheme 5). Subsequent treatment of 12 with sodium azide in acetone provided the 3-azido-6-propargyloxy-1,2,4,5-tetrazine (13).10 This material melts at 50 °C and begins to polymerize if left in the melt for several hours to provide polymer 14. Unfortunately, the extreme sensitivity of 13 precluded further studies on the properties of the resultant polymer.
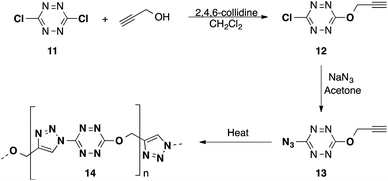 |
| Scheme 5 Synthesis of 13 and subsequent thermal polymerization to 14. | |
Calculations for compounds 4, 7, and 9 are provided in Table 1, and include: theoretical maximum density (ρ), heat of formation (ΔHf), and oxygen balance (OB).19–21
Table 1 Theoretical calculations
|
4 |
7 |
9 |
ρ (g cm−3) |
1.40 |
1.39 |
1.40 |
ΔHf (kcal mol−1) |
128.04 |
44.91 |
87.87 |
OB (%) |
−143 |
−155.7 |
−90.1 |
Additionally, sensitivity measurements were conducted on compounds 6, 6p, 8, 9, 10 and are compared to pentaerythritol tetranitrate (PETN) in Table 2. Measurements include: impact sensitivity (IS), friction sensitivity (FS), and electrostatic discharge (ESD).
Table 2 Sensitivity measurements
|
ISa [cm] |
FSb [N] |
ESDc [J] |
LANL type 12, 50% drop height, 2.5 kg weight. 50% load Neyer d-optimal method. ABL spark, 3.4% threshold initiation level (TIL). |
4 |
20.7 |
225 |
0.0625 |
6 |
177 |
>360 |
0.0625 |
6p |
194 |
>360 |
0.0625 |
8 |
79.4 |
>360 |
0.125 |
9 |
21.5 |
>360 |
0.125 |
10 |
>320 |
>360 |
0.125 |
PETN |
12.9 |
58 |
0.0625 |
Illustrated in Table 2 polymers 6, 6p, 8, and 10 all have impact sensitivities less than PETN. However, 6 and 6p both are comparable to PETN with respect to spark sensitivities.
In conclusion, propargyl alcohol and 1 reacted to give the unprecedented product 4 which maintained the 1,2,4,5-tetrazine ring system intact. With this result in hand, we implemented a [3+2] cycloaddition strategy using alkyne and azide moieties to accomplish prepolymer curing, polymerization and self-polymerization reactions. The resultant energetic polymers contain the 1,2,4,5-tetrazine within the polymer scaffolds. All polymers presented here display explosive sensitivity properties either less than or equal to PETN. Further work on studying these energetic polymers and developing new energetic materials are currently being pursued.
Experimental section
General methods
Unless otherwise noted, all 1H– and 13C–NMR spectra were recorded in DMSO-d6 at ambient temperature using a Bruker Avance 400 spectrometer operating at 400 MHz for H and 100 MHz for 13C. Chemical shift values (δ) are reported in ppm, referenced to the residual DMSO solvent signal (1H: 2.50 ppm & 13C: 39.52 ppm). FT-IR spectra were measured with a Thermo–Nicolet iS5 FTIR spectrophotometer using the OMNIC software, and data collection are reported in reciprocal centimeters (cm−1). IR spectra measurements were made using an ATR cell. Elemental Analyses (EA) were performed with a PerkinElmer series II 2400 CHNS/O analyzer. All reagents and solvents were used as received (Sigma–Aldrich, Fluka, Acros Organics and Mach 1 Specialty Chemicals). 2,2-Diazidomethyl-1,3 propanediol (7)16 and 2-hydroxy-1,3-diazido-propane (9)17,18 were synthesized according to literature procedure.
Caution! The compounds and polymers presented here are highly energetic with sensitivity to various stimuli. While we encountered no issues while working with this material, proper protective measures (Kevlar® gloves, face shield, and grounded equipment) should still be used at all times. The [3+2] cycloaddition between alkyne and azide moieties is highly exothermic and should only be undertaken in small amounts with sufficient heat-dissipation to avoid ignition during reaction.
4. To propargyl alcohol (10 mL) is added freshly powdered K2 CO3 (0.40 g, 2.89 mmol). The mixture was stirred at ambient temperature. 3,6-Bis(3,5-dimethyl-pyrazol-1-yl)-1,2,4,5-tetrazine (5.4 g, 20 mmol) was then added. The mixture was heated to 70 °C and the mixture became a solution. The reaction was stirred for 30 min at 70 °C, cooled to ambient temperature and water (50 mL) was added. A red-orange precipitate was formed. The product was filtered, washed with water and air-dried to provide 2.8 g (74% yield) of product. FTIR cm−1 3260, 2130, and 1320. 1H NMR (DMSO-d6, 400 MHz): δ 3.64 (d, J = 2.4 Hz, alkyne CH) and 5.72 (d, J = 2.4 Hz, CH2)·13C NMR (DMSO-d6, 100 MHz): δ 67.1, 87.3, 176.2, 215.7 ppm. Elemental analysis calcd C, 50.53, H, 3.18, 29.46. Found C, 50.51, H, 3.19, N, 29.448.
8. To 2,2-bisazidomethyl-1,3-propanediol (7) (0.093 g, 0.500 mmol) 3,6-bispropargyloxy-1,2,4,5-tetrazine (4) (0.086 g, 0.452 mmol) was added, followed by copper iodide (0.002 g, 0.010 mmol). The mixture was stirred for one minute (X2) at ambient temperature. The mixture was slowly heated in increments of 10 °C to 60 °C. The reaction was held at 60 °C for 24 hours. A dark burgundy solid was formed. The product was air-dried to provide 0.078 g of material. FTIR cm−1 3417, 2102, 1710, and 1419. GPC/SEC 3.602 ± 136.71 g mol−1. DSC onset 115.98 °C, Tg at 15.4 °C.
10. To a vessel containing 3,6-bispropargyloxy-1,2,4,5-tetrazine (4) (0.203 g, 1.07 mmol), 2-hydroxy-1,3-diazidopropane (9) (0.167 g, 1.17 mmol) was added, followed by copper iodide (0.005 g, 0.026 mmol). The mixture was stirred for one minute (X2) at ambient temperature. The mixture was slowly heated in increments of 10 °C to 60 °C. The reaction was held at 60 °C for 24 hours. A dark burgundy solid was formed. The product was air-dried to provide 0.332 g of material. FTIR cm−1 3435, 2102, and 1642. GPC/SEC 6.796 ± 132.17 g mol−1. DSC onset 143.67 °C, Tg at 24.7 °C.
Conflicts of interest
The authors have no conflicts to declare.
Acknowledgements
We would like to thank Stephanie Hagelberg (elemental analysis) for characterization, Hongzhao Tian (DSC), Jose G. Archuleta, Lisa Klamborowski (sensitivity testing), Taylor Dehner (GPC, DSC) and Mary Sandstrom (thermal analysis). We would also like to thank the Joint Munitions Technology Development Program for funding this work. Los Alamos National Laboratory is operated by Triad National Security, LLC for the U.S. Dept. of Energy's NNSA. The authors also thank the Office of Naval Research (Award No. C.). This work has been approved for public release under LA-UR-22-22149.
Notes and references
- C. Quinton, V. Alain-Rizzo, C. Dumas-Verdes, G. Clavier, L. Vignau and P. Audebert, Triphenylamine/Tetrazine Based π-Conjugated Systems as Molecular Donors for Organic Solar Cells, New J. Chem., 2015, 39(12), 9700–9713 Search PubMed
. - Z. Li, J. Ding, N. Song, J. Lu and Y. Tao, Development of a New s-Tetrazine-Based Copolymer for Efficient Solar Cells, J. Am. Chem. Soc., 2010, 132(38), 13160–13161 Search PubMed
. - S. A. A. Razavi, M. Y. Masoomi and A. Morsali, Double Solvent Sensing Method for Improving Sensitivity and Accuracy of Hg(II) Detection Based on Different Signal Transduction of a Tetrazine-Functionalized Pillared Metal–Organic Framework, Inorg. Chem., 2017, 56(16), 9646–9652 Search PubMed
. - I. Ortiz-Gomez, M. Ortega-Muñoz, A. Salinas-Castillo, J. A. Álvarez-Bermejo, M. Ariza-Avidad, I. de Orbe-Payá, F. Santoyo-Gonzalez and L. F. Capitan-Vallvey, Tetrazine-Based Chemistry for Nitrite Determination in a Paper Microfluidic Device, Talanta, 2016, 160, 721–728 Search PubMed
. - C. Allain, J. Piard, A. Brosseau, M. Han, J. Paquier, T. Marchandier, M. Lequeux, C. Boissière and P. Audebert, Fluorescent and Electroactive Low-Viscosity Tetrazine-Based Organic Liquids, ACS Appl. Mater. Interfaces, 2016, 8(31), 19843–19846 Search PubMed
. - L. Chen, F. Li, M. Nandi, L. Huang, Z. Chen, J. Wei, W. Chi, X. Liu and J. Yang, Towards Tetrazine-Based near-Infrared Fluorogenic Dyes: Is There a Wavelength Limit?, Dyes Pigments, 2020, 177, 108313–108318 Search PubMed
. - S.-K. Choi, J. Kim and E. Kim, Overview of Syntheses and Molecular-Design Strategies for Tetrazine-Based Fluorogenic Probes, Molecules, 2021, 26(7), 1868 Search PubMed
. - Y. Lee, W. Cho, J. Sung, E. Kim and S. B. Park, Monochromophoric Design Strategy for Tetrazine-Based Colorful Bioorthogonal Probes with a Single Fluorescent Core Skeleton, J. Am. Chem. Soc., 2018, 140(3), 974–983 Search PubMed
. - D. E. Chavez, M. A. Hiskey and R. D. Gilardi, 3,3′-Azobis(6-Amino-1,2,4,5-Tetrazine): A Novel High-Nitrogen Energetic Material, Angew. Chem., Int. Ed., 2000, 39(10), 1791–1793 Search PubMed
. - D. E. Chavez and M. A. Hiskey, 1,2,4,5-Tetrazine Based Energetic Materials, J. Energ. Mater., 1999, 17(4), 357–377 Search PubMed
. - M. Guan, H. Yang, C. Lu and C. Guang-bin, Synthesis and Characterization of Trinitroethyl Compounds Derived from Tetrazine, Hanneng Cailiao, 2014, 22(6), 736–740 Search PubMed
. - M. H. V. Huynh, M. A. Hiskey, D. E. Chavez, D. L. Naud and R. D. Gilardi, Synthesis, Characterization, and Energetic Properties of Diazido Heteroaromatic High-Nitrogen C–N Compound, J. Am. Chem. Soc., 2005, 127(36), 12537–12543 Search PubMed
. - M. H. V. Huynh, M. A. Hiskey, J. G. Archuleta, E. L. Roemer and R. Gilardi, 3,6-Di(Azido)-1,2,4,5-Tetrazine: A Precursor for the Preparation of Carbon Nanospheres and Nitrogen-Rich Carbon Nitrides, Angew. Chem., 2004, 116(42), 5776–5779 Search PubMed
. - D. Chen, H. Yang, Z. Yi, H. Xiong, L. Zhang, S. Zhu and G. Cheng, C8N26H4: An Environmentally Friendly Primary Explosive with High Heat of Formation, Angew. Chem., Int. Ed., 2018, 57(8), 2081–2084 Search PubMed
. - R. I. Ishmetova, N. I. Latosh, I. N. Ganebnykh, N. K. Ignatenko, S. G. Tolshchina and G. L. Rusinov, Replacement of Dimethylpyrazolyl Group in 1,2,4,5-Tetrazines by Aliphatic Alcohols and Water, Russ. J. Org. Chem., 2009, 45(7), 1102–1107 Search PubMed
. - L. Yang, H. Xiao, L. Yan, R. Wang, Y. Huang, Z. Xie and X. Jing, Lactose Targeting Oxaliplatin Prodrug Loaded Micelles for More Effective Chemotherapy of Hepatocellular Carcinoma, J. Mater. Chem. B, 2014, 2(15), 2097 Search PubMed
. - K. G. Priyanka, A. K. Mishra, S. Kantheti, R. Narayan and K. V. S. N. Raju, Synthesis of Triazole Ring-Containing Pentol Chain Extender and Its Effect on the Properties of Hyperbranched Polyurethane-Urea Coatings, J. Appl. Polym. Sci., 2012, 126(6), 2024–2034 Search PubMed
. - R. R. Farias, A. J. S. Mascarenhas, T. d. J. Santos and M. M. Victor, Are Diazides Really Dangerous Compounds under Ordinary Conditions?, Tetrahedron Lett., 2020, 61(48), 152574–152577 Search PubMed
. - E. F. C. Byrd and B. M. Rice, Improved Prediction of Heats of Formation of Energetic Materials Using Quantum Mechanical Calculations, J. Phys. Chem. A, 2006, 110(3), 1005–1013 Search PubMed
. - E. F. C. Byrd and B. M. Rice, Additions and corrections, J. Phys. Chem. A, 2009, 113, 5813 (J. Phys. Chem. A, 2022, 126, 1787) Search PubMed
. - L. A. Fried, W. M. Howard, S. Bastea, K. Glaesmann, P. C. Souers, P. A. Vitello and L. F. Kuo, CHEETAH Thermochemical Code, Lawrence Livermore National Laboratory, Livermore, CA, 1994 Search PubMed
.
Footnote |
† Electronic supplementary information (ESI) available: For experimental details, including characterization, X-ray crystallographic and rheometry data. CCDC 1846069. For ESI and crystallographic data in CIF or other electronic format see https://doi.org/10.1039/d2ra05339j |
|
This journal is © The Royal Society of Chemistry 2022 |
Click here to see how this site uses Cookies. View our privacy policy here.