DOI:
10.1039/D2RA05387J
(Paper)
RSC Adv., 2022,
12, 28800-28803
PIFA-mediated selenylative spirocyclization of indolyl ynones: facile access to selenated spiro[cyclopentenone-1,3′-indoles]†
Received
28th August 2022
, Accepted 3rd October 2022
First published on 10th October 2022
Abstract
A fast selenylative spirocyclization of indolyl ynones mediated by PIFA has been developed. This transformation was enabled by the reactive RSeOCOCF3 species generated in situ from diselenides with PIFA, involving an electrophilic dearomative cascade cyclization. This protocol provides a facile and efficient method for the synthesis of selenated spiro[cyclopentenone-1,3′-indoles] and tolerates broad functional groups.
Introduction
Spirocycles are ubiquitous in numerous pharmaceuticals, natural products, and valuable materials.1 Among the spirocycles, spiroindolenines have aroused great interest from organic chemists lately because of their diverse biological activities.2 Hence, development of efficient protocols for the direct construction of spiroindolenines is highly valuable in modern organic synthesis.3 In recent years, dearomative cascade cyclization with indolyl ynones has emerged as a powerful tool for the synthesis of sophisticated spiroindolines.4 For example, Unsworth4a–c and Van der Eycken4d described an efficient transition metal-catalyzed or trifluoroacetic acid (TFA)-promoted monofunctionalization of indolyl ynones to access spiroindolines, respectively. Subsequently, some elegant bifunctionalizations of indolyl ynones for the construction of functionalized spiroindolenines have been disclosed by organic chemists.4e–j Although several methods for the synthesis of the spiroindolenine scaffold have been well established, the facile and efficient construction of diverse functionalized spiroindolines is still in high demand.
On the other hand, organoselenides are an important class of bioactive molecules extensively found in pharmaceuticals and biologically active compounds.5 So far, a great deal of effort has been devoted to investigating the preparation of organoselenides.6 Among which, the diselenides are the most commonly used selenization reagents for these transformations.7 Considering the importance of spiroindoline and selenium in medicinal chemistry, efficient strategies for the synthesis of selenated spiroindolenines with potential biological interest are valuable. Recently, Xu's group realized an elegant visible light-promoted selenylative spirocyclization of indolyl ynones toward the formation of seleno-spiroindolenines via an arylselenyl radical process (Scheme 1a).8 Despite simple and mild reaction conditions, this method suffers from several limitations: (1) low atom economy of the reaction since only half equivalent of diselenide was utilized and the other half equivalent was wasted. (2) the diselenide bearing an alkane moiety was incompatible in the current transformation. Therefore, developing a new synthetic methodology with low loading of diselenide and high functional group tolerability to access seleno-spiroindolenines was necessary. Very recently, Zhao and Du disclosed that the reactive RSeOCOCF3 species generated in situ from diselenides with PIFA, which could participates in an electrophilic cyclization as an electrophile.9 Inspired by above results and our continuing interest in the construction of spirocycles and nitrogen-containing heterocyclics,10 herein, we report a fast PIFA-mediated selenylative spirocyclization of indolyl ynones for the construction of selenated spiroindolenines under mild conditions, involving an ionic pathway with a electrophilic cyclization (Scheme 1b). This reaction was enabled by RSeOCOCF3 generated in situ from diselenides with PIFA, followed by an electrophilic dearomative cascade cyclization of indolyl ynones.
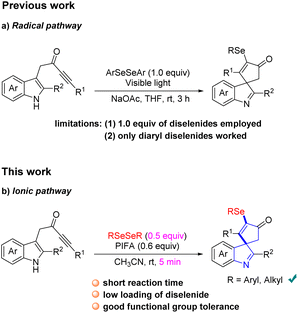 |
| Scheme 1 Synthesis of selenated spiroindolenines via radical or ionic process. | |
We commenced our investigation with the reaction of indole ynone 1a (0.2 mmol), diphenyl diselenide 2a (0.5 equiv.), and PIFA (0.6 equiv.) at room temperature under air atmosphere in DCM. To our delight, the desired product 3aa was afforded in 71% yield (Table 1, entry 1). First, various solvents were tested (entries 2–7), and acetonitrile (CH3CN) was found to be optimal (entry 4). Other hypervalent iodine oxidants including PIDA, PICl2 gave inferior yields of 3aa (entries 8–9). In addition, tuning the loading of PIFA did not further improve the reaction (entries 10 and 11). Control experiment indicated that no reaction occurred in the absence of PIFA (entry 12).
Table 1 Optimization of the reaction conditionsa,b
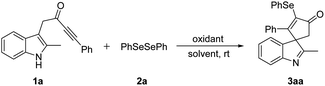
|
Entry |
Oxidant |
Solvent |
Yield(%)b |
Reaction conditions: 1a (0.2 mmol), 2a (0.1 mmol), and oxidant (0.12 mmol) in solvent (2 mL) at rt for 5 min. Isolated yield. PIFA (0.1 mmol) was used. PIFA (0.16 mmol) was used. |
1 |
PIFA |
DCM |
71 |
2 |
PIFA |
DCE |
74 |
3 |
PIFA |
THF |
56 |
4 |
PIFA |
CH3CN |
89 |
5 |
PIFA |
DMSO |
33 |
6 |
PIFA |
MeOH |
Trace |
7 |
PIFA |
Toluene |
65 |
8 |
PIDA |
CH3CN |
NR |
9 |
PhICl2 |
CH3CN |
75 |
10c |
PIFA |
CH3CN |
66 |
11d |
PIFA |
CH3CN |
86 |
12 |
— |
CH3CN |
NR |
After identifying the optimal reaction conditions, the substrate scope in this PIFA-mediated selenylative spirocyclization was investigated. The scope of diselenides 2 was first surveyed to react with indole ynone 1a (Table 2). Diselenides 2 bearing electron-donating groups, such as me, et, meo, and t-Bu at the para position of the phenyl ring, leading to the corresponding products in 87–89% yield (3ab–3ae). The reaction could also be scaled up to 4 mmol to give 1.42 g (83%) of product 3aa. para-Halogen-substituted substrates, such as F, Cl, and Br, afforded the desired products in 84–91% yield (3af–3ah). meta-Substituted diselenides with either electron-donating group (MeO) or electron-withdrawing group (CF3) were also worked well, giving spiroindolenines 3ai and 3aj in 80 and 85% yields, respectively. Furthermore, substituent at the ortho-position also proved to be tolerated and the corresponding product 3ak was generated in 74% yield. Diselenide bearing a thiophene moiety was also tolerated, giving 3al in 77% yield. The naphthyl-substituted selenated spiroindolenine 3am was afforded in 73% yield. In addition, dialkyl diselenides also served as suitable reaction partners with indole ynone 1a, giving the desired products 3an and 3ao in excellent yields.
Table 2 Scope of diselenidesa,b
Reaction conditions: 1a (0.2 mmol), 2 (0.1 mmol), and PIFA (0.12 mmol) in CH3CN (2 mL) at rt for 5 min. Isolated yield. 4 mmol scale. |
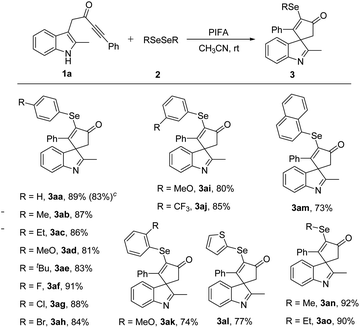 |
Next, various indolyl ynones 1 were tested with diphenyl diselenide 2a (Table 3). The effect of the substituents on the phenyl ynones was first explored. In general, this procedure was compatible with methyl, methoxy, halide, and trifluoromethyl groups, which gave the desired products 3ba–3ga in 80–90% yield. However, ortho-substituted aryl alkynes as substrates, such as ortho-bromo-substituted aryl alkyne, there was no reaction occurred and no desired product was obtained. Additionally, 2-thienyl in the alkyne reacted smoothly to provide 3ha in satisfactory yield. When the aryl group of ynones was replaced by an alkyl group, such as cyclopropyl, no desired product 3ia was detected. Then, the effect of the substituents on the indole ring (R2) was studied, the substrates with a group at the C5 or C7 position of the indole ring were all tolerated (3ja–3ka). The substrate with a methoxy group at the C4 position of the indole ring treated with 2a affording a trace amount of desired product, perhaps due to the steric hindrance at the C4-position was unfavorable for the dearomatizing spirocyclization. Meanwhile, when the methyl group on the indole skeleton rings replaced with a phenyl group, the substrate 1 failed to transform into desired product, perhaps aslo due to the steric hindrance at the C2-position was unfavorable for the transformation. Unfortunately, when non-two-substituted indole in the substrate 1 was used, the reaction failed to give the desired product 3la.
Table 3 Scope of indolyl ynonesa,b
Reaction conditions: 1 (0.2 mmol), 2a (0.1 mmol), and PIFA (0.12 mmol) in CH3CN (2 mL) at rt for 5 min. Isolated yield. |
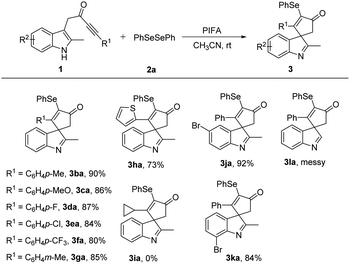 |
To understand the reaction mechanism, we carried out several control experiments. First, when PIFA and diphenyl diselenide reacted at rt in the absence of substrate 1a, leading to compound 4, which was detected by HRMS (see the ESI†) (Scheme 2, eqn 1). Moreover, when substrate 1a was treated with PhSeCl and silver trifluoroacetate in acetonitrile at room temperature,11 the desired product 3aa was obtained in 86% yield (Scheme 2, eqn 2). Both control experiments indicated that this transformation was enabled by the reactive RSeOCOCF3 species generated in situ from diselenides with PIFA.
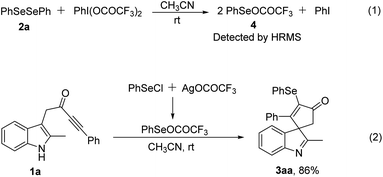 |
| Scheme 2 Control experiments. | |
Base on the above results and previous reports,9,12 a plausible reaction pathway is shown in Scheme 3. First, the oxidation of diselenide 2a by PIFA promotes the generation of reactive PhSeOCOCF3 through the attack of selenium of diselenide on the iodine center of PIFA to form intermediate A, which was converted to selenium salt B after the elimination of PhI. Then trifluoroacetic acid anion attacked the selenium atom of salt B to produce two molecules of PhSeOCOCF3. Next, PhSeOCOCF3 reacted with the alkyne group of indolyl ynone 1a leaded the formation of selenium ion C. Finally, the electrophilic dearomative cyclization of C at 3-position of indole to give the desired product 3aa.
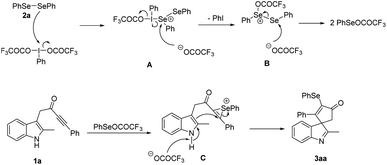 |
| Scheme 3 Proposed reaction mechanism. | |
Conclusions
In summary, a selenylative spirocyclization of indolyl ynones mediated by PIFA has been developed. This protocol provides a simple, high-yielding approach for the quick formation of selenated spiroindolenines. The reaction requires mild conditions and shows broad reactivity. Exploration of the reaction mechanism suggests that an electrophilic RSeOCOCF3 -induced dearomative cascade cyclization is the operative reaction.
Conflicts of interest
There are no conflicts to declare.
Acknowledgements
This work was supported by the NSF of Fujian Province (2021J01965, 2020J01769) and Quanzhou Science and Technology Foundation (2020C034R).
Notes and references
-
(a) J.-F. Hu, H. Fan, J. Xiong and S.-B. Wu, Chem. Rev., 2011, 111, 5465 CrossRef PubMed
;
(b) W.-T. Wu, L. Zhang and S.-L. You, Chem. Soc. Rev., 2016, 45, 1570 RSC
;
(c) E. M. Antunes, B. R. Copp, M. T. Davies-Coleman and T. Samaai, Nat. Prod. Rep., 2005, 22, 62 RSC
;
(d) H. Wang and X. Luan, Org. Biomol. Chem., 2016, 14, 9451 RSC
;
(e) W.-C. Yang, M.-M. Zhang and J.-G. Feng, Adv. Synth. Catal., 2020, 362, 4446 CrossRef
;
(f) Y. Zheng, C. M. Tice and S. B. Sing, Bioorg. Med. Chem. Lett., 2014, 24, 3673 CrossRef
;
(g) Z. Zuo, J. Wang, J. Liu, Y. Wang and X. Luan, Angew. Chem., Int. Ed., 2020, 59, 653 CrossRef PubMed
. -
(a) J. Bariwal, L. G. Voskressensky and E. V. Van der Eycken, Chem. Soc. Rev., 2018, 47, 3831 RSC
;
(b) E. Vitaku, D. T. Smith and J. T. Njardarson, J. Med. Chem., 2014, 57, 10257 CrossRef CAS
;
(c) Y. Zheng, C. M. Tice and S. B. Singh, Bioorg. Med. Chem. Lett., 2014, 24, 3673 CrossRef CAS
;
(d) R. Jadulco, R. A. Edrada, R. Ebel, A. Berg, K. Schaumann, V. Wray, K. Steube and P. Proksch, J. Nat. Prod., 2004, 67, 78 CrossRef CAS
. -
(a) W. Zi, Z. Zuo and D. Ma, Acc. Chem. Res., 2015, 48, 702 CrossRef CAS
;
(b) J. Bariwal, L. Voskressensky and E. Vandereycken, Chem. Soc. Rev., 2018, 47, 3831 RSC
;
(c) G. Huang and B. Yin, Adv. Synth. Catal., 2019, 361, 405 CrossRef CAS
;
(d) C.-X. Zhuo, C. Zheng and S.-L. You, Acc. Chem. Res., 2014, 47, 2558 CrossRef CAS PubMed
;
(e) Q.-F. Wu, C. Zheng and S.-L. You, Angew. Chem., Int. Ed., 2012, 51, 1680 CrossRef CAS PubMed
;
(f) M. J. James, P. O'Brien, R. J. K. Taylor and W. P. Unsworth, Chem.–Eur. J., 2016, 22, 2856 CrossRef
. -
(a) M. J. James, J. D. Cuthbertson, P. O'Brien, R. J. K. Taylor and W. P. Unsworth, Angew. Chem., Int. Ed., 2015, 54, 7640 CrossRef PubMed
;
(b) A. K. Clarke, M. J. James, P. O'Brien, R. J. K. Taylor and W. P. Unsworth, Angew. Chem., Int. Ed., 2016, 55, 13798 CrossRef PubMed
;
(c) J. T. R. Liddon, A. K. Clarke, R. J. K. Taylor and W. P. Unsworth, Org. Lett., 2016, 18, 6328 CrossRef CAS PubMed
;
(d) P. Fedoseev and E. K. Van der Eycken, Chem. Commun., 2017, 53, 7732 RSC
;
(e) P. Fedoseev, G. Coppola, G. M. Ojeda and E. K. Van der Eycken, Chem. Commun., 2018, 54, 3625 RSC
;
(f) H. E. Ho, T. C. Stephens, T. J. Payne, P. O'Brien, R. J. K. Taylor and W. P. Unsworth, ACS Catal., 2019, 9, 504 CrossRef CAS
;
(g) H. E. Ho, A. Pagano, J. A. Rossi-Ashton, J. R. Donald, R. G. Epton, J. C. Churchill, M. J. James, P. O'Brien, R. J. K. Taylor and W. P. Unsworth, Chem. Sci., 2020, 11, 1353 RSC
;
(h) B. Zhang, X. Li, Z. Ai, B. Zhao, Z. Yu and Y. D, Org. Lett., 2022, 24, 390 CrossRef CAS
;
(i) N. Inprung, H. E. Ho, J. A. Rossi-Ashton, R. G. Epton, A. C. Whitwood, J. M. Lynam, R. J. K. Taylor, M. J. James and W. P. Unsworth, Org. Lett., 2022, 24, 668 CrossRef CAS
;
(j) G. Ru, M. Zhang, T. Zhang, X. Jiang, G. Gao, X. Zhu, S. Wang, C. Fan, X. Li and W. Shen, Org. Chem. Front., 2022, 9, 2621 RSC
. -
(a) Z. Chen, H. Lai, L. Hou and T. Chen, Chem. Commun., 2020, 56, 179 RSC
;
(b) L. S. Galant, J. Rafique, A. L. Braga, F. C. Braga, S. Saba, R. Radi, J. B. T. da Rocha, C. Santi, M. Monsalve, M. Farina and A. F. de Bem, Neurochem. Res., 2021, 46, 120 CrossRef CAS
;
(c) Z. Jin, X. Du, Y. Xu, Y. Deng, M. Liu, Y. Zhao, B. Zhang, X. Li, L. Zhang, C. Peng, Y. Duan, J. Yu, L. Wang, K. Yang, F. Liu, R. Jiang, X. Yang, T. You, X. Liu, X. Yang, F. Bai, H. Liu, X. Liu, L. W. Guddat, W. Xu, G. Xiao, C. Qin, Z. Shi, H. Jiang, Z. Rao and H. Yang, Nature, 2020, 582, 289 CrossRef CAS
;
(d) N.-B. Li, L. Xu, R. Ma, Q. Fan, B. Li, J. Qiao, R. Guo and X.-H. Xu, J. Inorg. Chem., 2021, 41, 2723 Search PubMed
;
(e) B. Banerjee and M. Koketsu, Coord. Chem. Rev., 2017, 339, 104 CrossRef
;
(f) L. Sancineto, A. Mariotti, L. Bagnoli, F. Marini, J. Desantis, N. Iraci, C. Santi, C. Pannecouque and O. Tabarrini, J. Med. Chem., 2015, 58, 9601 CrossRef
. -
(a) C. An, C.-Y. Li, X.-B. Huang, W.-X. Gao, Y.-B. Zhou, M.-Z. Liu and H.-Y. Wu, Org. Lett., 2019, 21, 6710 CrossRef
;
(b) Y. Wu, J.-Y. Chen, J. Ning, X. Jiang, J. Deng, Y. Deng, R. Xu and W.-M. He, Green Chem., 2021, 23, 3950 RSC
;
(c) A. D. Sonawane, R. A. Sonawane, M. Ninomiya and M. Koketsu, Adv. Synth. Catal., 2020, 362, 3485 CrossRef CAS
;
(d) Q.-B. Zhang, Y.-L. Ban, P.-F. Yuan, S.-J. Peng, J.-G. Fang, L.-Z. Wu and Q. Liu, Green Chem., 2017, 19, 5559 RSC
;
(e) S. Wu, J. Shi and C.-P. Zhang, Org. Biomol. Chem., 2019, 17, 7468 RSC
;
(f) I. P. Beletskaya and V. P. Ananikov, Chem. Rev., 2011, 111, 1596 CrossRef CAS
. -
(a) Y. Xu, C. Li, J. Meng, Y. Huang, J. Fu, B. Liu, Y. Liu and N. Chen, J. Inorg. Chem., 2021, 41, 1012 Search PubMed
;
(b) H. Ishitani, Z. Yu, T. Ichitsuka, N. Koumura, S. Onozawa, K. Sato and S. Kobayashi, Adv. Synth. Catal., 2022, 364, 1 CrossRef
;
(c) C.-F. Zhou, Y.-Q. Zhang, Y. Ling, L. Ming, X. Xi, G.-Q. Liu and Y. Zhang, Org. Biomol. Chem., 2022, 20, 420 RSC
;
(d) Z. Zhang, S. Wang, P. Tan, X. Gu, W. Sun, C. Liu, J. Chen, J. Li and K. Sun, Org. Lett., 2022, 24, 2288 CrossRef CAS PubMed
;
(e) K. Sun, X. Wang, C. Li, H. Wang and L. Li, Org. Chem. Front., 2020, 7, 3100 RSC
;
(f) Z. Guan, Y. Wang, H. Wang, Y. Huang, S. Wang, H. Tang, H. Zhang and A. Lei, Green Chem., 2019, 21, 4976 RSC
;
(g) Z. Chen, X. Zheng, S.-F. Zhou and X. Cui, Org. Biomol. Chem., 2022, 20, 5779 RSC
. - X. Zhou, H. Liu, Z. Mo, X. Ma, Y. Chen, H. Tang, Y. Pan and Y. Xu, Chem.–Asian J., 2020, 15, 1536 CrossRef CAS
. - Z. Ai, J. Xiao, Y. Li, B. Guo, Y. Du and K. Zhao, Org. Chem. Front., 2020, 7, 3935 RSC
. -
(a) Z. Chen, H. Zhang, S.-F. Zhou and X. Cui, Org. Lett., 2021, 23, 7992 CrossRef PubMed
;
(b) Z. Chen, H. Zhang, S.-F. Zhou and X. Cui, Org. Chem. Front., 2022, 9, 364 RSC
;
(c) Z. Chen, H. Zhang, S.-F. Zhou and X. Cui, J. Inorg. Chem., 2020, 40, 3866 Search PubMed
;
(d) Z. Song, Z. Yang, P. Wang, Z. Shi, T. Li and X. Cui, Org. Lett., 2020, 22, 6272 CrossRef
;
(e) T. Yuan, C. Pi, C. You, X. Cui, S. Du, T. Wan and Y. Wu, Chem. Commun., 2019, 55, 163 RSC
. -
(a) H. J. Reich, J. Org. Chem., 1974, 39, 428 CrossRef
;
(b) D. M. Browne, O. Niyomura and T. Wirth, Org. Lett., 2007, 9, 3169 CrossRef PubMed
. -
(a) L. Xing, Y. Zhang, B. Li and Y. Du, Org. Lett., 2019, 21, 3620 CrossRef PubMed
;
(b) S. Ortgies and A. Breder, ACS Catal., 2017, 7, 5828 CrossRef CAS
;
(c) F. V. Singh and T. Wirth, Org. Lett., 2011, 13, 6504 CrossRef CAS PubMed
;
(d) A. G. Kutateladze, J. L. Kice, T. G. Kutateladze, N. S. Zefirov and N. V. Zyk, Tetrahedron Lett., 1992, 33, 1949 CrossRef CAS
.
Footnote |
† Electronic supplementary information (ESI) available: Experimental details and spectral data, copies of 1H and 13C NMR spectra. See DOI: https://doi.org/10.1039/d2ra05387j |
|
This journal is © The Royal Society of Chemistry 2022 |
Click here to see how this site uses Cookies. View our privacy policy here.