DOI:
10.1039/D2RA05704B
(Paper)
RSC Adv., 2022,
12, 34946-34950
An expedient metal-free cascade route to chromonyl diene scaffolds: thermodynamic vs. kinetic control†
Received
10th September 2022
, Accepted 29th November 2022
First published on 7th December 2022
Abstract
A piperidine-catalyzed reaction between 3-formylchromone, 1,3-dimethyl barbituric acid, and ylidenemalononitriles is developed that offers chromonyl diene products in good yields. This cascade reaction proceeds via the insertion of ylidenemalononitriles between the Knoevenagel adduct obtained from 3-formylchromone and 1,3-dimethylbarbituric acid, where the pyrimidine-based enaminone is integrated with the chromone through the central diene linker. Similarly, introducing pyrimidine-based enaminone into the terminal part of the chromonyl diene scaffold gave an equilibrium mixture of rotational isomers in DMSO, which could be separated and isolated by crystallization. The computational analysis confirmed the role of barbiturate in directing the type of final chromonyl diene via kinetic or thermodynamic control. Moreover, computations revealed that one of these species, observed in the NMR spectra, is produced by the bond cleavage in the spirocyclic intermediate.
The barbituric acid derivatives have attracted extensive attention because of their unique therapeutic properties such as antioxidant,1 antibacterial,2 anti-proliferative,3 antitubercular,4 sedative,5 antispasmodic,6 anti-inflammatory,7 anticonvulsant,8 anticancer,9 and hypnotic activities.10 Although barbituric acid is, on its own, pharmacologically inactive, structural modification at its pyrimidinone C5 atom allows potential biological activities11 due to conjugated carbon–carbon double bonds.12 Such 5-arylidene barbiturates are described as antimicrobials against different bacteria and fungi13 and are potent tyrosinase inhibitors.14 In addition, by adding the alkenylamine moiety to the structure, they can strongly chelate various metal ions in biological systems.15 On the other hand, a naturally occurring oxygen-containing chromone nucleus has emerged as an essential pharmacophore of many biological compounds16 including neuroprotective, anticancer, HIV-inhibitory, antioxidant activities, and are effective in inhibiting α-glucosidase.17 From a pharmacological point of view, one route to modifying chromone is the introduction of barbituric acid derivatives, on which many biologically active compounds are based.18 On the other hand, chromones bearing a functionalized conjugated dienes unit at the C3 position are potential intermediates for synthesizing biologically active molecules.19 Furthermore, integrating one terminal chromone, one barbiturate, and a central diene linear linker can be an efficient way for the synthesis of π-conjugated compounds and a new strategy for biologically active product discovery (Fig. 1). Few reactions allow the direct synthesis of π-conjugated compounds through the formation of carbon–carbon bonds. The reported reactions of 1,3-butadiene derivatives containing chromone moiety have been prepared mainly using condensation of Wittig reagents20 and Pd-catalyzed cross-coupling reaction.21 Also, the aldol reaction of ylidenemalononitriles with 3-formylchromones led to the formation of electron-deficient dienes connected to the chromone moiety.22 Along these lines, herein, we report the ylidenemalononitriles insertion reaction between the Knoevenagel adduct obtained from 3-formylchromone and 1,3-dimethylbarbituric acid in the synthesis of chromone-barbiturate hybrid structure linked by a linear diene (Scheme 1).
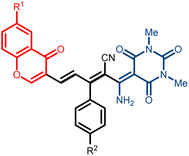 |
| Fig. 1 The design of the proposed hybrid chromone-barbiturate linked by a central diene. | |
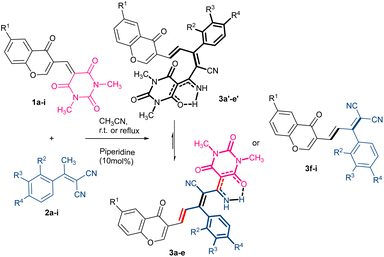 |
| Scheme 1 One-pot sequential synthesis of substituted chromonyl triene barbiturate and chromonyl dienes. | |
We began our study with model reactants chromonyl barbituric's acid 1b and ylidenemalononitrile 2b in the presence of a suitable base. The acetic acid-mediated synthesis involving Knoevenagel condensation of 3-formylchromone with 1,3-dimethylbarbituric acid in water is known.23 We found that the resulting multifunctional synthon 1b could in situ react with ylidenemalononitrile 2b, which have been used extensively as vinylogous donors in Michael reactions.24 Indeed, the chromonyl triene product containing barbiturate moiety 3b was obtained in a 65% yield within 24 hours. Various bases such as 1,8-diazabicyclo[5.4.0]undec-7-ene (DBU), piperidine, Et3N, and 1,4-diazabicyclo[2.2.2]octane (DABCO) were first evaluated in CH3CN. Screening the amount of the selected base showed that the catalytic amount of piperidine (10 mol%) provided a good yield of 3b. It is important to note that changing the CH3CN solvent with EtOH led to inseparable reaction mixtures. Therefore, various solvents such as THF, DCM, CH3CN, DMF, and toluene were examined, and the result showed that CH3CN played a significant role in improving the product yield (Table 1). Electron-donating substituents (OMe and 2,3-di-OMe) at suitable positions of the phenyl ring were introduced, and the products 3b and 3c were afforded in excellent yields at room temperature. Note that electron-withdrawing chlorine atoms at both position 2 of the phenyl ring and within the chromone ring also worked well in this insertion process, so that, in the absence of chlorine of chromone, the chromonyl diene product 3f was formed. Interestingly, the reaction provided the chromonyl diene product 3g at room temperature and the desired chromonyl triene 3d at reflux. To establish the scope and limitations, we found that in the case of 2,3-di-OMe and OMe substituents and 6-chloro-3-formylchromone, the reaction gave chromonyl diene products 3i and 3h, even at a reflux temperature. Also, in cases of 4-bromophenyl, 4-methylphenyl, 4-fluorophenyl and 4-chlorophenyl analogues, the reaction did not lead to desired products, and the formed yellow precipitate could not be analyzed due to its low solubility. Investigating spectral data of synthesized chromonyl trienes revealed two stereoisomers, which led us to assume that two s-trans,s-trans, and s-trans,s-cis-conformers exists as an equilibrium mixture in DMSO. In the 1H NMR spectra of 3a, the appearance of the singlet at δ 8.65 and 8.67 ppm indicated that the pyrone ring remained intact in both isomers. The chemical shifts of olefinic protons of major isomer at δ 6.26 and 7.97 ppm with the vicinal coupling constants 3J = 15.7–15.8 Hz revealed the E-configuration of chromonyl chalcone double bond. It is established that cis H-atoms with respect to the phenyl group appear in the higher field due to a long-range anisotropy effect of the non-coplanar benzene ring, which could be the reason for the shielding effect of olefinic H-11 proton.25
Table 1 Scope of substituted chromonyl diene 3
The plausible mechanism of further transformations for all products can be readily rationalized from Scheme 2. It seems that the carbonyl groups of barbiturate in In1a–1i provided two possible routes for the formation of final products by the [1,5]-H-transfer from the methylene substitution at the γ position of two carbonyl groups to the barbiturate moiety to form the intermediates In2a–2e, or the [1,3]-H-transfer with the same proton to barbiturate to from In2f–2i. After the H-transfer, the negative charge on the methylene site would liberate a barbiturate to generate the desired chromonyl diene products P3f–3i through the formation of intermediates In2f–2i (path A) or would take part in an annulation reaction to form P3a–3e by passing from the intermediates In2a–2e (path B).
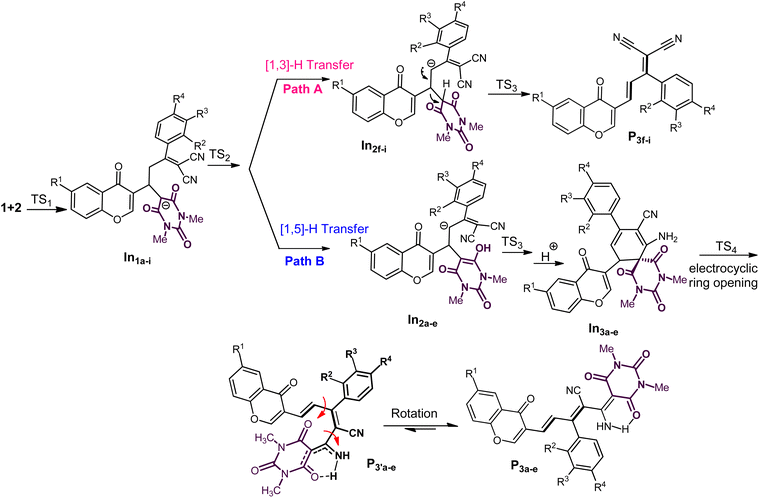 |
| Scheme 2 The mechanistic proposal for the formation of chromonyl diene derivatives. | |
Considering that two proton transfer mechanisms competed for the final product, we individually examined the affecting factors including thermodynamics and kinetics in this section. In comparing the energy barriers of hydrogen transfers, it was found that the transfer of [1,5] with an energy between 10 and 14 kcal mol−1 is kinetically faster than that of [1,3] (29–32 kcal mol−1). However, the thermodynamic difference between the intermediates and transition state (0.5–1 kcal mol−1) in [1,5]-H-transfer, indicated a possibility of reaction reversal.
This issue could account for the formation of P3f–3g in reflux conditions since the reflux condition not only provided the needed energy for [1,3]-H-transfer but also created more stable intermediates (Fig. 3). In order to verify that temperature can increase the instability of the intermediates In2a–2i, and promote the more favorable inverse pathway over the In3a–3i formation, we selected 3d as an example in reflux and the ambient temperature. The results showed that In2d in path A had a significant instability among intermediates, and the reflux conditions have influenced on the reverse reaction more than passing the reaction through TS3 which was confirmed by experiments. In other words, unstable structures in reflux conditions tended to be at their optimal energy level, and favorable kinetic conditions could not influence the direction of reactivity. Thus, both sets of results confirmed that the decisive step in selecting the reaction pathway was related to proton transfer under thermodynamic control. Finally, breaking the spiro bond would proceed through 6π electrocyclic ring-opening due to the torsional strain of the formed spirocyclic In3b (Fig. 2). It should be noted that the 1C–2C bond dissociation enthalpy (ΔHR = −6.24 kcal mol−1, for details, see ESI†) of spirocyclic In3 confirms the 6π electrocyclic ring-opening.
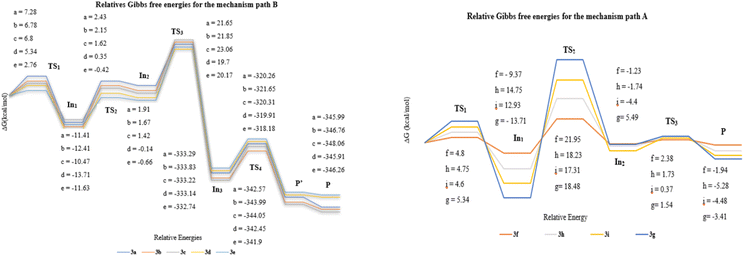 |
| Fig. 2 Relative Gibbs free energies for the mechanism of path A and path B (all values in kcal mol−1). | |
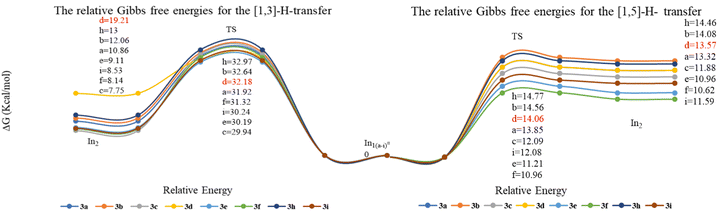 |
| Fig. 3 Relative Gibbs free energies for the mechanism of [1,3] and [1,5] H-transfer (all values in kcal mol−1). | |
The experimental 1H NMR evidence supported the existence of two products as an equilibrium mixture in DMSO in P3a–3e. According to the NMR computational data (for details, see ESI†), these are related to compounds P3a′–3e′, resulting from the ring-opening, and linear structures P3a–3e. In addition, the energy difference between P3b and P3b′ is 0.45 kcal mol−1, which shows that both stereoisomers could be in the equilibrium with each other. Considering the importance of the pyrimidine-based enaminone and chromonyl dienes and a unique orientation of the functional substituents on a rigid heterocyclic system, the highly selective cascade method for the synthesis of substituted chromonyl dienes in satisfactory yields has been described.
This piperidine-catalyzed reaction proceeds via the ylidenemalononitrile insertion between the Knoevenagel adduct obtained from 3-formylchromone and 1,3-dimethylbarbituric acid, while the pyrimidine-based enaminone is integrated with the chromone through the central diene linker. Computations showed that the barbiturate unit can control the direction of reaction through a single bond rotation and can lead to the diversity of linear chromonyl diene products with and without a pyrimidine-based enaminone unit.
Conflicts of interest
There are no conflicts to declare.
Acknowledgements
Financial support of this research from Tarbiat Modares University, Iran, is gratefully acknowledged. Also, we would like to thank Dr Alcides Simao from the Deutsches Elektronen-Synchrotron DESY (Hamburg) for his technical support on the ORCA calculation.
Notes and references
-
(a) B. B. Sokmen, S. Ugras, H. Y. Sarikaya, H. I. Ugras and R. Yanardag, Appl. Biochem. Biotechnol., 2013, 171, 2030 CrossRef CAS PubMed;
(b) I. Chaaban, A. Mohsen, M. E. Omar and M. A. Maharan, Sci. Pharm., 1984, 52, 51 CAS.
-
(a) J. Figueiredo, J. L. Serrano, M. Soares, S. Ferreira, F. C. Domingues, P. Almeida and S. Silvestre, Eur. J. Pharm. Sci., 2019, 137, 104964 CrossRef CAS PubMed;
(b) Q. Yan, R. Cao, W. Yi, Z. Chen, H. Wen, L. Ma and H. Song, Eur. J. Med. Chem., 2009, 44, 4235 CrossRef CAS PubMed.
- J. Figueiredo, J. L. Serrano, E. Cavalheiro, L. Keurulainen, J. Yli-Kauhaluoma, V. M. Moreira, S. Ferreira, F. C. Domingues, S. Silvestre and P. Almeida, Eur. J. Med. Chem., 2018, 143, 829 CrossRef CAS PubMed.
- S. V. Laxmi, Y. T. Reddy, B. S. Kuarm, P. N. Reddy, P. A. Crooks and B. Rajitha, Bioorg. Med. Chem. Lett., 2011, 21, 4329 CrossRef PubMed.
-
(a) A. R. Zarei and F. Gholamian, Anal. Biochem., 2011, 412, 224 CrossRef CAS PubMed;
(b) T. L. Lemke and D. A. Williams, Foye's Principle of Medicinal Chemistry, Wolters Kluwer Health, 2012, p. 485 Search PubMed;
(c) F. Lopez-Munoz, R. Ucha-Udabe and C. Alamo, Neuropsychiatr. Dis. Treat., 2005, 1, 329 CAS.
- J. S. Lundy, Anesth. Analg., 1929, 8, 360 Search PubMed.
-
(a) M. A. A. Radwan, E. A. Ragab, N. M. Sabry and S. M. El-Shenawy, Bioorg. Med. Chem., 2007, 15, 3832 CrossRef CAS PubMed;
(b) F. Shaheen, M. Ahmad, M. T. H. Khan, S. Jalil, A. Ejaz, M. N. Sultankhodjaev, M. Arfan and M. I. Choudhary, Phytochemistry, 2005, 66, 935 CrossRef CAS PubMed.
-
(a) D. Kalaivani, R. Malarvizhi and R. Subbalakshmi, Med. Chem. Res., 2008, 17, 369 CrossRef CAS;
(b) D. S. Cox, K. R. Scott, H. Gao and N. D. Eddington, J. Pharmacol. Exp. Ther., 2002, 302, 1096 CrossRef CAS PubMed.
-
(a) A. Barakat, S. M. Soliman and Y. A. M. M. Elshaier, J. Mol. Struct., 2017, 1134, 99 CrossRef CAS;
(b) N. R. Penthala, A. Ketkar, K. R. Sekhar, M. L. Freeman, R. L. Eoff, R. Balusu and P. A. Crooks, Bioorg. Med. Chem., 2015, 23, 7226 CrossRef CAS PubMed;
(c) P. Bhatt, M. Kumar and A. Jha, ChemistrySelect, 2018, 3, 7060 CrossRef CAS.
-
(a) F. López-Muñoz, R. Ucha-Udabe and C. Alamo, Neuropsychiatr. Dis. Treat., 2005, 1, 329 Search PubMed;
(b) A. S. Girgis, H. Farag, N. S. M. Ismail and R. F. George, Eur. J. Med. Chem., 2011, 46, 4964 CrossRef CAS PubMed.
-
(a) B. S. Jursic, F. Douelle and E. D. Stevens, Tetrahedron, 2003, 59, 3427 CrossRef CAS;
(b) E. Giziroglu, M. Aygün, C. Sarikurkcu, D. Kazar, N. Orhan, E. Firinci, H. Soyleyici and C. Gokcen, Inorg. Chem. Commun., 2013, 36, 199 CrossRef CAS.
-
(a) G. M. Ziarani, F. Aleali and N. Lashgari, RSC Adv., 2016, 6, 50895 RSC;
(b) N. D. Moirangthem and W. S. Laitonjam, Beilstein J. Org. Chem., 2010, 6, 1056 CrossRef CAS PubMed;
(c) M. A. Ismail, S. Al-Shihry, R. K. Arafa and U. J. El-Ayaan, J. Enzyme Inhib. Med. Chem., 2013, 28, 530 CrossRef CAS PubMed.
- K. M. Khan, M. Khan, A. Ahmad, A. Irshad, L. B. S. Kardono, F. Rahim, S. M. Haider, S. Ahmed and S. Parveen, J. Chem. Soc. Pak., 2014, 36, 1153 CAS.
-
(a) Z. Chen, D. Cai, D. Mou, Q. Yan, Y. Sun, W. Pan, Y. Wan, H. Song and W. Yi, Bioorg. Med. Chem., 2014, 22, 3279 CrossRef CAS PubMed;
(b) S. Ranjbar, P.-S. Shahvaran, N. Edraki, M. Khoshneviszadeh, M. Darroudi, Y. Sarrafi, M. Hamzehloueian and M. Khoshneviszadeh, Arch. Pharm., 2020, 353, 2000058 CrossRef CAS PubMed;
(c) Q. Yan, R. Cao, W. Yi, Z. Chen, H. Wen, L. Ma and H. Song, Eur. J. Med. Chem., 2009, 44, 4235 CrossRef CAS PubMed;
(d) Q. Yan, R. Cao, W. Yi, L. Yu, Z. Chen, L. Ma and H. Song, Bioorg. Med. Chem. Lett., 2009, 19, 4055 CrossRef CAS PubMed.
- R. Fırıncı, E. Fırıncı, G. Başbülbül, M. B. Dabanca, D. B. Celepci and M. E. Günay, Transition Met. Chem., 2019, 44, 391 CrossRef.
- R. S. Keri, S. Budagumpi, R. K. Pai and R. G. Balakrishna, Eur. J. Med. Chem., 2014, 78, 340 CrossRef CAS PubMed.
-
(a) R. Larget, B. Lockhart, P. Renard and M. Largeron, Bioorg. Med. Chem. Lett., 2000, 10, 835 CrossRef CAS PubMed;
(b) P. Valenti, A. Bisi, A. Rampa, F. Belluti, S. Gobbi, A. Zampiron and M. Carrara, Bioorg. Med. Chem., 2000, 8, 239 CrossRef CAS PubMed;
(c) D. Yu, C. H. Chen, A. Brossi and K. H. Lee, J. Med. Chem., 2004, 47, 4072 CrossRef CAS PubMed;
(d) M. Kuroda, S. Uchida, K. Watanabe and Y. Mimaki, Phytochemistry, 2009, 70, 288 CrossRef CAS PubMed;
(e) G. Wang, M. Chen, J. Qiu, Z. Xie and A. Cao, Bioorg. Med. Chem. Lett., 2018, 28, 113 CrossRef CAS PubMed.
-
(a) T. N. M. Musthafa, Z. N. Siddiqui, F. M. Husain and I. Ahmad, Med. Chem. Res., 2011, 20, 1473 CrossRef CAS;
(b) K. A. Krasnov, V. G. Kartsev and A. S. Gorovoi, Chem. Nat. Compd., 2000, 36, 192 CrossRef CAS.
-
(a) S. Kumar, B. K. Singh, A. K. Pandey, A. Kumar, S. K. Sharma, H. G. Raj, A. K. Prasad, E. Van der Eycken, V. S. Parmar and B. Ghosh, Bioorg. Med. Chem., 2007, 15, 2952 CrossRef CAS PubMed;
(b) H. Chen, F. Xie, J. Gong and Y. Hu, J. Org. Chem., 2011, 76, 8495 CrossRef CAS PubMed;
(c) A.-T. Dang, D. O. Miller, L. N. Dawe and G. Bodwell, Org. Lett., 2008, 10, 233 CrossRef CAS PubMed;
(d) Y. Zhang, Z. Lv, M. Zhang and K. Li, Tetrahedron, 2013, 69, 8839 CrossRef CAS;
(e) Y.-F. Yu, C. Zhang, Y.-Y. Huang, S. Zhang, Q. Zhou, X. Li, Z. Lai, Z. Li, Y. Gao, Y. Wu, L. Guo, D. Wu and H.-B. Luo, ACS Chem. Neurosci., 2020, 11, 1058 CrossRef CAS PubMed;
(f) W. Sun, P. J. Carroll, D. R. Soprano and D. J. Canney, Bioorg. Med. Chem., 2009, 19, 4339 CrossRef CAS PubMed;
(g) Q.-H. Chen, K. Yu, X. Zhang, G. Chen, A. Hoover, F. Leon, R. Wang, N. Subrahmanyam, E. A. Mekuria and L. H. Rakotondraibe, Bioorg. Med. Chem. Lett., 2015, 25, 4553 CrossRef CAS PubMed.
- W. Sun, P. J. Carroll, D. R. Soprano and D. J. Canney, Bioorg. Med. Chem. Lett., 2009, 19, 4339 CrossRef CAS PubMed.
-
(a) H. Horiguchi, K. Hirano, T. Satoh and M. Miura, Adv. Synth. Catal., 2009, 351, 1431 CrossRef CAS;
(b) A. T. Lindhardt, M. L. H. Mantel and T. Skrydstrup, Angew. Chem., Int. Ed., 2008, 47, 2668 CrossRef CAS PubMed;
(c) C. Chen, K. Wilcoxen, Y.-F. Zhu, K. Kim and J. R. McCarthy, J. Org. Chem., 1999, 64, 3476 CrossRef CAS PubMed;
(d) L. Zhao and X. Lu, Org. Lett., 2002, 4, 3903 CrossRef CAS PubMed.
- V. Y. Korotaev, A. Y. Barkov, I. B. Kutyashev, A. V. Safrygin and V. Y. Sosnovskikh, Tetrahedron, 2014, 70, 3584 CrossRef CAS.
- A. Alizadeh, A. Bagherinejad, J. Kayanian and R. Vianello, New J. Chem., 2022, 46, 7242 RSC.
-
(a) A. Alizadeh, H. Sedighian and F. Bayat, Synlett, 2014, 25, 389 CrossRef CAS;
(b) A. Alizadeh, S. Y. Hosseini, H. Sedighian, F. Bayat, Z. Zhu and M. Dusek, Tetrahedron, 2015, 71, 7885 CrossRef CAS;
(c) A. Alizadeh and A. Bagherinejad, ChemistrySelect, 2020, 5, 1547 CrossRef CAS;
(d) A. Alizadeh, A. Bagherinejad, F. Bayat, S. Y. Hosseini and L. G. Zhu, Mol. Divers., 2019, 23, 651 CrossRef CAS PubMed;
(e) S. Noritake, N. Shibata, S. Nakamura, T. Toru and M. Shiro, Eur. J. Org. Chem., 2008, 3465 CrossRef CAS;
(f) E. Magnier, J. C. Blazejewski, M. Tordeux and C. Wakselman, Angew. Chem., Int. Ed., 2006, 45, 1279 CrossRef CAS PubMed;
(g) T. Umemoto and S. Ishihara, J. Am. Chem. Soc., 1993, 115, 2156 CrossRef CAS;
(h) J. A. Ma and D. Cahard, Chem. Rev., 2004, 104, 6119 CrossRef CAS PubMed.
- T. Hayashi, J. Org. Chem., 1966, 31, 3253 CrossRef CAS.
Footnote |
† Electronic supplementary information (ESI) available: Experimental and computational details; single crystal X-ray diffraction analysis; 1H and 13C NMR spectra. CCDC 1970238. For ESI and crystallographic data in CIF or other electronic format see DOI: https://doi.org/10.1039/d2ra05704b |
|
This journal is © The Royal Society of Chemistry 2022 |
Click here to see how this site uses Cookies. View our privacy policy here.