DOI:
10.1039/D2SC00419D
(Edge Article)
Chem. Sci., 2022,
13, 4103-4108
Enantioselective [1,2]-Stevens rearrangement of thiosulfonates to construct dithio-substituted quaternary carbon centers†
Received
21st January 2022
, Accepted 10th March 2022
First published on 11th March 2022
Abstract
An enantioselective [1,2] Stevens rearrangement was realized by using chiral guanidine and copper(I) complexes. Bis-sulfuration of α-diazocarbonyl compounds was developed through using thiosulfonates as the sulfenylating agent. It was undoubtedly an atom-economic process providing an efficient route to access novel chiral dithioketal derivatives, affording the corresponding products in good yields (up to 90% yield) and enantioselectivities (up to 96
:
4 er). A novel catalytic cycle was proposed to rationalize the reaction process and enantiocontrol.
Introduction
Organosulfur compounds, including thioethers and the oxidized derivatives such as sulfoxides or sulfones, have attracted significant attention owing to their wide occurrence in natural products and biologically active molecules.1 Furthermore, they could also serve as useful reagents and ligands in organic synthesis.2 Thiosulfonate is considered the line of defense against cyanide intoxication,3 and could be used for both sulfenylation and sulfonylation.4 For example, sulfenylation of α-sulfonyl carboxylic esters with S-methyl methanethiosulfonate resulted in dithioketals containing two sulfur substitutions with different oxidation states at the same carbon center4a (Scheme 1a). The carbene insertion reaction of α-diazoesters to the S–S bond of thiosulfonates could simultaneously introduce two different sulfur-groups into the stereogenic carbon center in the presence of the bpy/CuTc4b or dppp/Rh2(OAc)4 (ref. 4c) catalyst (Scheme 1b). The reaction occurred via a [1,2]-Stevens rearrangement of sulfonium ylide,5,6 but enantiocontrol remains difficult. Xu's group explored the possibility of an asymmetric version by screening a variety of bisoxazoline ligands, and the highest ee of 55% was obtained.4b Tang and co-workers demonstrated an efficient catalytic asymmetric [1,2]-Stevens rearrangement of sulfonium ylide using side-armed bisoxazoline/Cu(OTf)2 as the catalyst (Scheme 1c),6a but the stereogenic center lied in an O-based carbon center rather than the carbon of metal carbenoid species.7
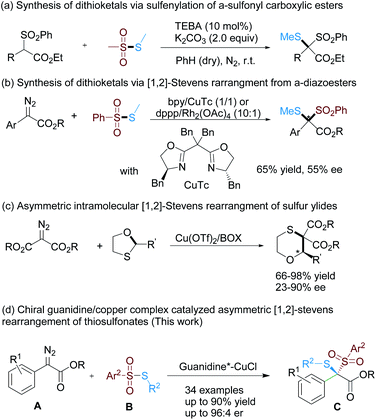 |
| Scheme 1 Synthesis of dithioketals from thiosulfonates and asymmetric catalytic [1,2]-Stevens rearrangements of sulfonium ylides. | |
Sigmatropic rearrangements of ylides represent a powerful set of reactions for the construction of heterocycles and interesting small molecules with functional groups. Different from asymmetric [2,3]-sigmatropic rearrangements and other transformations of onium ylides which have been well studied,8,9 the enantioselective [1,2]-Stevens rearrangement of onium ylides is limited and challenging.10 The intricacy mainly lies in the unclear mechanism where diradical pairs, concerted or ion pair propositions have an unpredictable influence on the stereoselectivity, regardless of chiral metal-bound or free ylide intermediates.
Recently, our group has realized several asymmetric catalytic rearrangements9b,d,e and carbene–insertion reactions12d,e,13a based on α-diazo carbonyl compounds. Guanidines as a kind of multi-nitrogen-containing compound have versatile functionality as organocatalysts and ligands for asymmetric catalytic reactions.11 Previously, we utilized chiral amino acids to construct bifunctional acyclic guanidine-amide compounds for organocatalysis12a–c and metal complex catalysis.13 Their combination with copper(I) salt13 showed an obvious accelerating effect in carbenoid insertion of terminal alkynes13a or HCN,13e enabling enantioselective construction of both axial and center chirality. In view of these studies and the characteristics of readily modified subunits of guanidine-amides, we explored their application in [1,2]-Stevens rearrangement between thiosulfonates and α-diazoesters (Scheme 1d). Herein we describe the results of enantioselective synthesis of dithio-substituted carbon centers via [1,2]-Stevens rearrangement to simultaneously introduce two C–S bonds with a chiral guanidine/CuCl catalyst.
Results and discussion
Initially, we carried out the reaction with S-methyl benzenesulfonothioate B1 as the dithio-source, and (R)-2-pipecolic acid derived guanidine-amide G5 and CuCl as the catalyst, and the selected results are listed in Table 1. The reaction with α-diazoesters A1–A4 tethered to different ester groups could be performed with moderate to good yield and enantioselectivity in CH2Cl2 at 40 °C (Table 1, entries 1–4). Especially, 4-fluorobenzyl 2-diazo-2-phenylacetate A4 could get better results with 75% yield and 91.5
:
8.5 er (entry 4). In comparison with the weak transformation in the presence of CuCl salt itself, the addition of guanidine also dramatically increased the reactivity. The use of other metal salts, such as CuBr, CuI, or CuTc, resulted in sluggish reactivity even with the assistance of guanidine G5 (entries 5–7). The dithio-substituted racemic product C4 was generated with low yield in the presence of metal salts such as Rh2(OAc)4, Pd2(dba)3, or AgOTf (entry 8; see the ESI† for details). Moreover, it was found that the steric hindrance of the substitution on the amide unit of guanidines had a dramatic influence on the enantioselectivity. Only the racemic product C4 was isolated if aniline-based G1 was used as the ligand (entry 9). The enantioselectivity gradually enhanced as the steric hindrance of substitutions at the 2,4,6-positions of anilines increased (G1–G5, entries 9–12 vs. entry 4). Using isopropyl groups instead of cyclohexyl groups at the N-substituents of the amidine part of guanidine afforded similar er values but slightly lower yields (G6vs.G5; entry 13 vs. entry 4). The amino acid backbone was also critical, for tetrahydroisoquinoline-3-carboxylic acid-based guanidine G7 decreased the enantioselectivity a lot (entry 14). A screening of other chiral guanidines with variation at different subunits identified that the combination of CuCl and guanidine G5 was the optimal catalyst for the reaction (see the ESI† for details). Increasing the catalyst loading to 15 mol% led to a slightly higher yield and enantioselectivity (entry 15, 78% yield with 92
:
8 er).
Table 1 Optimization of reaction conditionsa
With the optimized reaction conditions in hand, the substrate scope was then explored (Table 2). The established optimal reaction conditions exhibited similar tolerance to phenyl substituted α-diazo compounds with substituted benzyl esters (C5–C8), or more sterically hindered benzhydryl ester (C20). However, the electronic nature of substitution at the ortho-, meta-, or para-positions of the aromatic rings of α-diazoesters had a dramatic influence on the enantioselectivity. Products with electron-deficient aryl groups (C12–C18, and C20–C28) were universally generated in moderate to good yields (65–90%) with higher enantioselectivities (90
:
10–96
:
4 er), nevertheless, the products containing electron-donating substituents (C9–C11, C29 and C30) were accessible with high yields (75–86%) but with decreased enantioselectivities (only 58.5
:
41.5 er for 4-MeO substituted C30). The electronic effect of substitutions on the enantioselectivity was uniform no matter what the ester group, implying that the stability of the sulfonium ylide intermediate was significant to the enantiocontrol. Additionally, 2-naphthyl substituted dithioketal C19 was obtained with a decreased er value due to the steric hindrance. Unfortunately, the reaction of ethyl 2-diazopropanoate delivered the desired product with 42% yield as a racemate, and other alkyl α-diazoesters resulted in poor reactivity under the current conditions (see the ESI† for details).
Table 2 Substrate scope for thiosulfonates and α-diazoestersaa
Unless otherwise noted, all reactions were performed with A (0.20 mmol), B (0.10 mmol), G5/CuCl (1 : 1, 15 mol%) in CH2Cl2 (0.1 mL) at 40 °C. Isolated yield. The er was determined by HPLC analysis on a chiral stationary phase.
|
|
Subsequently, we turned our attention to the scope of thiosulfonates. Not surprisingly, benzenesulfonyl groups with substitutions, such as 4-Me, 4-F, 4-Cl, and 4-Br, were tolerated well in the reaction to yield the corresponding products smoothly (C31–C34, 72–90% yields, and 88
:
12–90.5
:
9.5 er). The product C35 deriving from 2-naphthyl thiosulfonate could also be generated in 89% yield with 89
:
11 er. However, S-ethyl and S-propyl-substituted thiosulfonates delivered the related products C36 and C37 with unsatisfied results, highlighting the steric bias of sulfur substitution on enantioselectivity.
The oxidation of the dithioketal derivative C20 led to the construction of the derivative D1 (Scheme 2) with a disulfonyl substituted quaternary center, whose absolute configuration was determined to be S based on X-ray single crystal analysis.14 Accordingly, the absolute configuration of the product C20 was assigned as the same. Next, we carried out a cross-over experiment by subjecting α-diazoester A4 and two kinds of thiosulfonate to the same catalytic system. It was found that two direct rearrangement products were detected, and there was no scrambling of the substituents in dithioketals. Primary theoretical calculations about the natural bond orbital charges of thiosulfonate B1 showed that the sulfonyl center has a strong positive charge while the other thio-atom has a negative charge (Scheme 3), indicating that the homolytic cleavage of the S–S bond to generate a diradical intermediate is difficult. We proposed that the reaction is likely to proceed in a concerted ionic pathway. The above results are in consist with the electronic effect of aryl substituents of diazoesters on the reactivity. The electron-donating aryl group is advantageous for the final rearrangement to increase the yield but disadvantageous for the initial ylide formation step, which might determine the enantioselectivity.
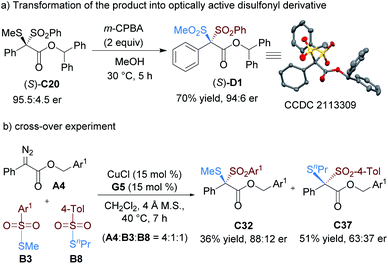 |
| Scheme 2 Transformation of the products and the control experiment. | |
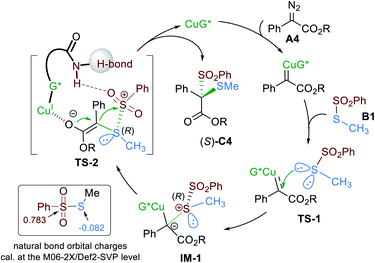 |
| Scheme 3 Possible asymmetric catalytic process. | |
In connection with the coordination ability of the guanidine functional group, we propose that it might act as a bifunctional ligand to form a chiral copper complex catalyst. As shown in Scheme 3, upon the addition of α-diazoester A, chiral copper carbenoid species are generated that undergo nucleophilic attack by thiosulfonate B. Different from the oxonium ylides, there is a S-based central chirality in the sulfonium ylide intermediate, generating from the discrimination of the two lone pair electrons of thiosulfonate which is extremely challenging, similar to asymmetric Sommelet–Houser rearrangement and 2,3-Stevens rearrangements.9b,d Moreover, if the reaction follows a stepwise 1,2-sigmatropic rearrangement or free ylide intermediate, the stereo-chemistry at sulfur disappears or racemizes, leading to a new, difficult to reconstruct stereogenic center at carbon.
We rationalize that the steric hindrance between the sulfonate group of thiosulfonate B and ester group of α-diazoester A would lead to selective attack of sulfur viaTS-1 to set up the chiral sulfonium ylide IM-1. Meanwhile, the hydrogen-bond between the amide of G5 and sulfonate unit might also direct the approach of thiosulfonate. Next, in view of the instability of the SO2Ph cation intermediate and the cross-over experiment, a stepwise process is ruled out. Thus, a concerted rearrangement occurs with the shift of sulfonate from the Si-face of the enolate viaTS-2 during which the chiral copper-bond enolate might prevent racemization of the sulfur center and assist the approach of the sulfonyl from the rear to construct the second C–S bond. Finally, the desired (S)-dithioketal derivative C is generated to recycle the chiral copper catalyst.
Conclusions
In summary, we have reported an efficient asymmetric catalytic [1,2]-Stevens rearrangement by using a type of chiral guanidine-copper(I) complex. The catalytic system enabled the direct introduction of two C–S bonds in an enantioselective manner, yielding various chiral dithioketal derivatives in good yield and enantioselectivity, shedding a shaft of light on the asymmetric 1,2-sigmatropic rearrangement. More research about the [1,2]-Stevens rearrangement and the application of the chiral guanidine/copper(I) catalytic system are undergoing.
Data availability
Further details of experimental procedure, 1H, 13C{1H} and 19F{1H} NMR, HPLC spectra, X-ray crystallographic data for D1 are available in the ESI.†
Author contributions
L. F. H. performed the experiments. J. Z. L. repeated data. X. M. F. and X. H. L. supervised the project. X. M. F., X. H. L., Y. Y. Z. and L. F. H. co-wrote the manuscript.
Conflicts of interest
There are no conflicts to declare.
Acknowledgements
We appreciate the National Natural Science Foundation of China (21625205) and Sichuan University (2020SCUNL204) for financial support. The authors also acknowledge Dr Yuqiao Zhou (Sichuan University) for his assistance in X-ray crystallographic analysis and calculations.
Notes and references
- For selected reviews and examples, see:
(a) S. W. Kaldor, V. J. Kalish, J. F. Davies, B. V. Shetty, J. E. Fritz, K. Appelt, J. A. Burgess, K. M. Campanale, N. Y. Chirgadze, D. K. Clawson, B. A. Dressman, S. D. Hatch, D. A. Khalil, M. B. Kosa, P. P. Lubbehusen, M. A. Muesing, A. K. Patick, S. H. Reich, K. S. Su and J. H. Tatlock, J. Med. Chem., 1997, 40, 3979 CrossRef CAS PubMed;
(b) T. Nakazawa, J. Z. Xu, T. Nishikawa, T. Oda, A. Fujita, K. Ukai, R. E. P. Mangindaan, H. Rotinsulu, H. Kobayashi and M. Namikoshi, J. Nat. Prod., 2007, 70, 439 CrossRef CAS PubMed;
(c) A. Y. Kovalevsky, A. K. Ghosh and I. T. Weber, J. Med. Chem., 2008, 51, 6599 CrossRef CAS PubMed;
(d) A. Z. Rys, I. A. Abu-Yousef and D. N. Harpp, Tetrahedron Lett., 2008, 49, 6670 CrossRef CAS;
(e) C. S. Jiang, W. E. G. Müller, H. C. Schröder and Y. W. Guo, Chem. Rev., 2012, 112, 2179 CrossRef CAS PubMed;
(f) E. K. Davison and J. Sperry, J. Nat. Prod., 2017, 80, 3060 CrossRef CAS PubMed;
(g) D. H. Scharf, J. D. Dworschak, P. Chankhamjon, K. Scherlach, T. Heinekamp, A. A. Brakhage and C. Hertweck, ACS Chem. Biol., 2018, 13, 2508 CrossRef CAS PubMed;
(h) E. O. N'Nang, G. Bernadat, E. Mouray, B. Kumulungui, P. Grellier, E. Poupon, P. Champy and M. A. Beniddir, Org. Lett., 2018, 20, 6596 CrossRef PubMed;
(i) E. O. N'Nang, P. L. Pogam, T. N. Mba, C. S. Obiang, E. Mouray, P. Grellier, B. Kumulungui, P. Champy and M. A. Beniddir, J. Nat. Prod., 2021, 84, 1409 CrossRef PubMed.
- For selected reviews and examples, see:
(a) M. Mellah, A. Voituriez and E. Schulzas, Chem. Rev., 2007, 107, 5133 CrossRef CAS PubMed;
(b) G. Sipos, E. E. Drinkel and R. Dorta, Chem. Soc. Rev., 2015, 44, 3834 RSC;
(c) F. L. Lam, F. Y. Kwong and A. S. C. Chan, Chem. Commun., 2010, 46, 4649 RSC;
(d) W. Y. Qi, T. S. Zhu and M. H. Xu, Org. Lett., 2011, 13, 3410 CrossRef CAS PubMed;
(e) Q. Y. Zhao, S. K. Li, K. X. Huang, R. Wang and X. M. Zhang, Org. Lett., 2013, 15, 4014 CrossRef CAS PubMed;
(f) Y. Li and M. H. Xu, Chem. Commun., 2014, 50, 3771 RSC;
(g) B. J. Wang, C. Lin, Y. Liu, Z. L. Fan, Z. X. Liu and Y. H. Zhang, Org. Chem. Front., 2015, 2, 973 RSC;
(h) Y. Wei, L. Q. Lu, T. R. Li, B. Feng, Q. Wang, W. J. Xiao and H. Alper, Angew. Chem., Int. Ed., 2016, 55, 2200 CrossRef CAS PubMed;
(i) I. V. Chuchelkin, K. N. Gavrilov, V. K. Gavrilov, S. V. Zheglov, I. D. Firsin, A. M. Perepukhov, A. V. Maximychev, N. E. Borisova, I. A. Zamilatskov, V. S. Tyurin, C. Dejoie, V. V. Chernyshev, V. S. Zimarev and N. S. Goulioukina, Organometallics, 2021, 40, 3645 CrossRef CAS.
-
(a) J. P. Weidner and S. S. Block, J. Med. Chem., 1964, 7, 671 CrossRef CAS PubMed;
(b) E. Block, Angew. Chem., Int. Ed. Engl., 1992, 31, 1135 CrossRef;
(c) M. L. Birsa, M. Cherkinsky and S. Braverman, Tetrahedron Lett., 2002, 43, 9615 CrossRef CAS;
(d) M. L. Alcaraz, S. Atkinson, P. Cornwall, A. C. Foster, D. M. Gill, L. A. Humphries, P. S. Keegan, R. Kemp, E. Merifield, R. A. Nixon, A. J. Noble, D. O'Beirne, Z. M. Patel, J. Perkins, P. Rowan, P. Sadler, J. T. Singleton, J. Tornos, A. J. Watts and I. A. Woodland, Org. Process Res. Dev., 2005, 9, 555 CrossRef CAS;
(e) G. G. Liang, M. C. Liu, J. X. Chen, J. C. Ding, W. X. Gao and H. Y. Wu, Chin. J. Chem., 2012, 30, 1611 CrossRef CAS;
(f) Y. Zheng, F. L. Qing, Y. G. Huang and X. H. Xu, Adv. Synth. Catal., 2016, 358, 3477 CrossRef CAS;
(g) Z. Y. Mo, T. R. Swaroop, W. Tong, Y. Z. Zhang, H. T. Tang, Y. M. Pan, H. B. Sun and Z. F. Chen, Green Chem., 2018, 20, 4428 RSC;
(h) G. Q. Li, Z. Y. Gan, K. X. Kong, X. M. Dou and D. S. Yang, Adv. Synth. Catal., 2019, 361, 1808 CrossRef CAS;
(i) Y. F. Lv, J. Y. Luo, Y. C. Ma, Q. Dong and L. He, Org. Chem. Front., 2021, 8, 2461 RSC.
- For selected examples, see:
(a) B. Wladislaw, L. Marzorati, N. F. Claro Jr and C. D. Vitta, Synthesis, 1997, 4, 420 CrossRef;
(b) H. R. Yuan, T. Nuligonda, H. Y. Gao, C. H. Tunga and Z. H. Xu, Org. Chem. Front., 2018, 5, 1371 RSC;
(c) C. Q. Rao, S. Y. Mai and Q. L. Song, Chem. Commun., 2018, 54, 5964 RSC;
(d) J. Li, S. Y. Wang and S. J. Ji, J. Org. Chem., 2019, 84, 16147 CrossRef CAS PubMed;
(e) P. Mampuys, C. R. McElroy, J. H. Clark, R. V. A. Orru and B. U. W. Maesa, Adv. Synth. Catal., 2020, 362, 3 CrossRef CAS;
(f) J. Y. Luo, M. Z. Lin, L. F. Wu, Z. H. Cai, L. He and G. F. Du, Org. Biomol. Chem., 2021, 19, 9237 RSC;
(g) J. L. Qi, F. Wei, S. Huang, C. H. Tung and Z. H. Xu, Angew. Chem., Int. Ed., 2021, 60, 4561 CrossRef CAS PubMed.
- For selected examples, see:
(a) M. Ioannou, M. J. Porter and F. Saez, Chem. Commun., 2002, 346 RSC;
(b) K. K. Ellis-Holder, B. P. Peppers, A. Yu. Kovalevsky and S. T. Diver, Org. Lett., 2006, 8, 2511 CrossRef CAS PubMed;
(c) A. J. P. Mortimer, A. E. Aliev, D. A. Tocher and M. J. Porter, Org. Lett., 2008, 10, 5477 CrossRef CAS PubMed;
(d) X. F. Xu, C. Li, M. T. Xiong, Z. H. Tao and Y. J. Pan, Chem. Commun., 2017, 53, 6219 RSC;
(e) R. Fan, C. Tan, Y. G. Liu, Y. Wei, X. W. Zhao, X. Y. Liu, J. J. Tan and H. Yoshida, Chin. Chem. Lett., 2021, 32, 299 CrossRef CAS.
- For selected examples, see:
(a) J. P. Qu, Z. H. Xu, J. Zhou, C. L. Cao, X. L. Sun, L. X. Dai and Y. Tang, Adv. Synth. Catal., 2009, 351, 308 CrossRef CAS;
(b) Z. Song, Y. Z. Wu, T. Xin, C. Jin, X. A. Wen, H. B. Sun and Q. L. Xu, Chem. Commun., 2016, 52, 6079 RSC;
(c) R. R. Lin, L. Y. Cao and F. G. West, Org. Lett., 2017, 19, 552 CrossRef CAS PubMed;
(d) X. B. Xu, Z. H. Lin, Y. Y. Liu, J. Guo and Y. He, Org. Biomol. Chem., 2017, 15, 2716 RSC;
(e) Z. Yang, Y. J. Guo and R. M. Koenigs, Chem. Commun., 2019, 55, 8410 RSC.
- For reviews:
(a) T. Ye and M. A. McKervey, Chem. Rev., 1994, 94, 1091 CrossRef CAS;
(b)
M. P. Doyle, M. A. Mckervey and T. Ye, in Modern Catalytic Methods for Organic Synthesis with Diazo Compounds, Wiley, New York, 1998 Search PubMed;
(c) Z. H. Zhang and J. B. Wang, Tetrahedron, 2008, 64, 6577 CrossRef CAS;
(d) H. M. L. Davies and D. Morton, Chem. Soc. Rev., 2011, 40, 1857 RSC;
(e) S. F. Zhu and Q. L. Zhou, Acc. Chem. Res., 2012, 45, 1365 CrossRef CAS PubMed;
(f) X. Guo and W. H. Hu, Acc. Chem. Res., 2013, 46, 2427 CrossRef CAS PubMed;
(g) A. Ford, H. Miel, A. Ring, C. N. Slattery, A. R. Maguire and M. A. McKervey, Chem. Rev., 2015, 115, 9981 CrossRef CAS PubMed;
(h) N. R. Candeias, R. Paterna and P. M. P. Gois, Chem. Rev., 2016, 116, 2937 CrossRef CAS PubMed;
(i) Y. Xia, D. Qiu and J. B. Wang, Chem. Rev., 2017, 117, 13810 CrossRef CAS PubMed.
- For selected examples about asymmetric rearrangements of onium ylides, see:
(a) W. Kirmse and M. Kapps, Chem. Ber., 1968, 101, 994 CrossRef CAS;
(b) M. P. Doyle, W. H. Tamblyn and V. J. Bagheri, J. Org. Chem., 1981, 46, 5094 CrossRef CAS;
(c) M. Ma, L. L. Peng, C. K. Li, X. Zhang and J. B. Wang, J. Am. Chem. Soc., 2005, 127, 15016 CrossRef CAS PubMed;
(d) D. Yadagiri and P. Anbarasan, Chem.?Eur. J., 2013, 19, 15115 CrossRef CAS PubMed;
(e) T. H. West, S. S. M. Spoehrle, K. Kasten, J. E. Taylor and A. D. Smith, ACS Catal., 2015, 5, 7446 CrossRef CAS;
(f) X. F. Xu, C. Li, Z. H. Tao and Y. J. Pan, Green Chem., 2017, 19, 1245 RSC;
(g) D. Kaldre, B. Maryasin, D. Kaiser, O. Gajsek, L. Gonzlez and N. Maulide, Angew. Chem., Int. Ed., 2017, 56, 2212 CrossRef CAS PubMed;
(h) H. Kim, J. Jang and S. Shin, J. Am. Chem. Soc., 2020, 142, 20788 CrossRef CAS PubMed.
- For selected examples about asymmetric rearrangements of onium ylides, see:
(a) Z. K. Zhang, Z. Sheng, W. Z. Yu, G. J. Wu, R. Zhang, W. D. Chu, Y. Zhang and J. B. Wang, Nat. Chem., 2017, 9, 970 CrossRef CAS PubMed;
(b) X. B. Lin, Y. Tang, W. Yang, F. Tan, L. L. Lin, X. H. Liu and X. M. Feng, J. Am. Chem. Soc., 2018, 140, 3299 CrossRef CAS PubMed;
(c) D. Kaldre, I. Klose and N. Maulide, Science, 2018, 361, 664 CrossRef CAS PubMed;
(d) X. B. Lin, W. Yang, W. K. Yang, X. H. Liu and X. M. Feng, Angew. Chem., Int. Ed., 2019, 58, 13492 CrossRef CAS PubMed;
(e) W. Yang, X. B. Lin, Y. Y. Zhang, W. D. Cao, X. H. Liu and X. M. Feng, Chem. Commun., 2020, 56, 10002 RSC;
(f) S. S. Li and J. B. Wang, J. Org. Chem., 2020, 85, 12343 CrossRef CAS PubMed;
(g) Z. Y. Liu, X. J. Jin and Y. F. Dang, ACS Catal., 2021, 11, 691 CrossRef CAS;
(h) S. X. Dong, X. H. Liu and X. M. Feng, Acc. Chem. Res., 2022, 55, 415 CrossRef CAS PubMed.
- For selected examples, see:
(a) F. G. West, B. N. Naidu and R. W. Tester, J. Org. Chem., 1994, 59, 6892 CrossRef CAS;
(b) K. W. Glaeske and F. G. West, Org. Lett., 1999, 1, 31 CrossRef CAS;
(c) G. Ghigo, S. Cagnina, A. Maranzana and G. Tonachini, J. Org. Chem., 2010, 75, 3608 CrossRef CAS PubMed;
(d) B. Xu and U. K. Tambar, J. Am. Chem. Soc., 2016, 138, 12073 CrossRef CAS PubMed;
(e) B. Xu, J. A. Gartman and U. K. Tambar, Tetrahedron Lett., 2017, 73, 4150 CrossRef CAS PubMed;
(f) D. Baidilov, Synthesis, 2020, 52, 21 CrossRef CAS;
(g) V. N. Nair, V. Kojasoy, C. J. Laconsay, W. Y. Kong, D. J. Tantillo and U. K. Tambar, J. Am. Chem. Soc., 2021, 143, 9016 CrossRef CAS PubMed;
(h) C. Empel, S. Jana and R. M. Koenigs, Synthesis, 2021, 53, 4567 CrossRef CAS.
- For selected reviews, see:
(a) D. S. Leow and C. H. Tan, Synlett, 2010, 2010, 1589 CrossRef;
(b) L. L. Zong and C. H. Tan, Acc. Chem. Res., 2017, 50, 842 CrossRef CAS PubMed;
(c) S. X. Dong, X. M. Feng and X. H. Liu, Chem. Soc. Rev., 2018, 47, 8525 RSC;
(d) X. Y. Cui, C. H. Tan and D. S. Leow, Org. Biomol. Chem., 2019, 17, 4689 RSC;
(e) J. Francos and V. Cadierno, Dalton Trans., 2019, 48, 9021 RSC;
(f) L. Steuer, E. Kaifer and H. J. Himmel, Dalton Trans., 2021, 50, 9467 RSC.
-
(a) S. X. Dong, X. H. Liu, X. H. Chen, F. Mei, Y. L. Zhang, B. Gao, L. L. Lin and X. M. Feng, J. Am. Chem. Soc., 2010, 132, 10650 CrossRef CAS PubMed;
(b) S. X. Dong, X. H. Liu, Y. L. Zhang, L. L. Lin and X. M. Feng, Org. Lett., 2011, 13, 5060 CrossRef CAS PubMed;
(c) S. X. Dong, X. H. Liu, Y. Zhu, P. He, L. L. Lin and X. M. Feng, J. Am. Chem. Soc., 2013, 135, 10026 CrossRef CAS PubMed;
(d) Y. Zhu, X. H. Liu, S. X. Dong, Y. H. Zhou, W. Li, L. L. Lin and X. M. Feng, Angew. Chem., Int. Ed., 2014, 53, 1636 CrossRef CAS PubMed;
(e) F. Tan, X. H. Liu, X. Y. Hao, Y. Tang, L. L. Lin and X. M. Feng, ACS Catal., 2016, 6, 6930 CrossRef CAS.
-
(a) Y. Tang, Q. G. Chen, X. H. Liu, G. Wang, L. L. Lin and X. M. Feng, Angew. Chem., Int. Ed., 2015, 54, 9512 CrossRef CAS PubMed;
(b) Q. G. Chen, Y. Tang, T. Y. Huang, X. H. Liu, L. L. Lin and X. M. Feng, Angew. Chem., Int. Ed., 2016, 55, 5286 CrossRef CAS PubMed;
(c) Y. Tang, J. Xu, J. Yang, L. L. Lin, X. M. Feng and X. H. Liu, Chem, 2018, 4, 1658 CrossRef CAS;
(d) S. S. Guo, P. Dong, Y. S. Chen, X. M. Feng and X. H. Liu, Angew. Chem., Int. Ed., 2018, 57, 16852 CrossRef CAS PubMed;
(e) S. Ruan, X. Zhong, Q. G. Chen, X. M. Feng and X. H. Liu, Chem. Commun., 2020, 56, 2155 RSC.
- CCDC 2113309 (D1) contains the supplementary crystallographic data for this paper.†.
Footnote |
† Electronic supplementary information (ESI) available: 1H,13C{1H} and19F{1H}NMR, HPLC spectra (PDF). X-ray crystallographic data for D1. CCDC D12113309. For ESI and crystallographic data in CIF or other electronic format see DOI: 10.1039/d2sc00419d |
|
This journal is © The Royal Society of Chemistry 2022 |
Click here to see how this site uses Cookies. View our privacy policy here.