DOI:
10.1039/D2SC04913A
(Edge Article)
Chem. Sci., 2022,
13, 13117-13121
A copper-catalyzed four-component reaction of arylcyclopropanes, nitriles, carboxylic acids and N-fluorobenzenesulfonimide: facile synthesis of imide derivatives†
Received
3rd September 2022
, Accepted 26th October 2022
First published on 27th October 2022
Abstract
An unprecedented copper-catalyzed four-component reaction of arylcyclopropanes, nitriles, carboxylic acids and N-fluorobenzenesulfonimide (NFSI) has been successfully developed, which represents the first example of a four-component reaction of non-donor–acceptor cyclopropanes. A wide range of imide derivatives were efficiently synthesized in excellent yields under mild conditions.
Introduction
Cyclopropanes, as the smallest carbocyclic compounds, have been emerging as very useful synthetic building blocks in modern organic synthesis due to their high π-character, inherent angle strain and intrinsic torsional strain, which confer versatile possibilities for ring-opening and elaboration.1 On the other hand, multicomponent reactions (MCRs) have been recognized as a very powerful tool for the facile and efficient construction of complex and valuable compounds,2 in particular the incorporation of substantial portions of more than three reactants in the same product through a one-pot procedure, owing to its high step-economy. Therefore, multicomponent reactions involving cyclopropanes have attracted much attention of synthetic chemists. So far, some impressive approaches for the three-component reaction of cyclopropane, especially ring-opening 1,3-difunctionalization have been investigated intensively. Various three-component reactions of cyclopropane with either one nucleophile and one electrophile,3 or one nucleophile and one radical trapping agent or one radical species,4 or even with two nucleophiles,5 have been well established in the past few years. In sharp contrast, the corresponding four-component reactions which have many more difficulties in delicately controlling the subtle balance between reactivity and selectivity of each component, are still very scarce.6 In this context, Studer and coworkers6a pioneered a Lewis acid-mediated four-component 1,3-bifunctionalization of donor–acceptor (D–A) cyclopropanes with arenes, nitrosoarenes and AlBr3 to provide γ,γ-disubstituted N-arylated α-amino ester derivatives in 2016 (Scheme 1A). Subsequently, the same group6b has also achieved an analogous four-component reaction involving D–A cyclopropanes, alkyl halides, 2-lithioindoles and boronic ester, and thereby provided a convenient route to synthetically valuable 2,3-disubstituted-2-boronated indoline derivatives. In spite of these impressive advances, to the best of our knowledge, the four-component reaction of non-D–A cyclopropanes has never been reported to date.
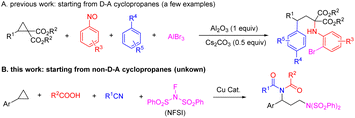 |
| Scheme 1 Four-component 1,3-difunctionalization of cyclopropanes. | |
Imides are commonly occurring in natural products and pharmacologically active compounds7 and have been widely applied in polymer chemistry8 as well as in organic synthesis.9 Therefore, their synthesis has evolved into one of the important topics in modern synthesis, resulting in numerous methods, including condensation of amides and carboxylic acid derivatives (such as carboxylic acids, carboxylic acid salts, acyl chlorides, esters, etc.),10 the oxidation of N,N-dialkyl amides or N-alkyl amides,11 the Ritter-type reaction of nitriles with anhydrides or carboxylic acids,12 oxidative ring-opening of oxazole or imidazole derivatives,13 and hydroamidocarbonylation of 1,3-dienes.14 Recently, an electrochemical four-component 1,2-alkyloxylimidation of alkenes has been successfully established for the synthesis of imides.15 Herein, we describe a novel copper-catalyzed four-component 1,3-diimidization of arylcyclopropanes, N-fluorobenzenesulfonimide (NFSI), nitriles and carboxylic acids, efficiently synthesizing a wide range of imide derivatives in good to excellent yields under mild conditions (Scheme 1B).
Results and discussion
At the beginning, we chose the reaction of phenylcyclopropane (1a), NFSI (2), 4-chlorobenzoic acid (3a) and acetonitrile as the model reaction to optimize the reaction conditions (for details, see ESI Tables S1–S3†). After the reaction was conducted in the presence of 10 mol% Cu(CH3CN)4PF6 and 12 mol% 6,6′-dimethylbipyridine (L1) in anhydrous acetonitrile at 40 °C under a nitrogen atmosphere for 24 hours, we are delighted to find that the desired imide 4a was obtained in an almost quantitative yield (Table 1, entry 1). The ligand could significantly affect the efficiency of this reaction and L1 was proved to be the most efficient ligand (entries 2–5). The absence of LiOtBu reduced the yield of 4a to 75% (entry 6). Anhydrous acetonitrile and the nitrogen atmosphere are favourable for the reaction (entries 7 and 8). The lower reaction temperature only slightly decreased the yield of 4a, while the higher temperature led to a dramatic reduction in 4a yield (entries 9 and 10).
Table 1 Optimization of the reaction conditionsa
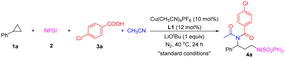
|
Entry |
Variation from the standard conditions |
Yieldb (%) |
Reaction was performed with 1a (0.4 mmol, 2 equiv.), NFSI (2, 0.5 mmol, 2.5 equiv.), 3a (0.2 mmol), Cu(CH3CN)4PF6 (0.02 mmol, 10 mol%), ligand (0.024 mmol, 12 mol%) and LiOtBu (0.2 mmol, 1 equiv.) in anhydrous CH3CN (2 mL) under a N2 atmosphere.
Yield was determined by 1H NMR with α-methylstyrene as an internal standard. n.r. = no reaction.
|
1 |
None |
99 |
2 |
Without the ligand |
Trace |
3 |
L2 instead of L1 |
n.r. |
4 |
L3 instead of L1 |
n.r. |
5 |
L4 instead of L1 |
73 |
6 |
Without LiOtBu |
75 |
7 |
Air instead of N2 |
20 |
8 |
Commercial CH3CN instead of anhydrous CH3CN |
73 |
9 |
25 °C instead of 40 °C |
96 |
10 |
60 °C instead of 40 °C |
40 |
|
With the optimized conditions in hand, we turned to investigate the scope of arylcyclopropanes in the novel four-component reaction (Table 2). The arylcyclopropanes with an electron-withdrawing group at the para-position of the benzene ring, such as chloride and carboxylic ester, could smoothly react with 2, 3a and acetonitrile, furnishing desired imides 4b and 4c. The arylcyclopropanes bearing an electron-donating group were also tested. para-Tetrabutyl substituted arylcyclopropanes 1d afforded imide 4d in 80% yield. para-Methyl substituted 1e produced the desired 4e in 32% yield; meanwhile a three-component aminoesterfication reaction of 1g with 2 and 3a took place and produced 4e′ in 24% yield. para-Methoxyl substituted arylcyclopropane 1f only underwent three-component aminooxygenation to give the aminoesterfication product 4f′, and no desired imide 4f was observed. When arylcyclopropanes with either an electron-withdrawing or electron-donating group were at the meta- or ortho-position of the benzene ring, the desired four-component reaction worked well and formed imide derivatives 4g–4m in 47–95% yields. Furthermore, a gram-scale reaction (3 mmol of 1a) readily produced 4a in 75% yield.
Table 2 Scope of arylcyclopropanesa
Reaction was performed with 1 (0.4 mmol, 2 equiv.), NFSI (2, 0.5 mmol, 2.5 equiv.), 3a (0.2 mmol), Cu(CH3CN)4PF6 (0.02 mmol, 10 mol%), L1 (0.024 mmol, 12 mol%) and LiOtBu (0.2 mmol, 1 equiv.) in anhydrous CH3CN (2 mL) under a N2 atmosphere at 40 °C. Isolated yields are given.
5 mol% Cu(CH3CN)4PF6 and 6 mol% L1 were used.
|
|
Subsequently, the scope of carboxylic acids 3 was investigated (Table 3). A wide range of benzoic acid derivatives with different substituents at the para-position of the benzene ring, including electron-withdrawing groups such as bromine, iodine, formyl, acetyl, nitro group, and cyano were satisfactorily compatible with the four-component reaction, furnishing imides 5a–5f in excellent yields (91–99%). Benzoic acid was also a suitable substrate to afford 5g in 81% yield. para-Electron-donating group substituted benzoic acids such as methoxyl and ethyl can smoothly participate in the reaction to deliver the corresponding imides 5h and 5i in 46% and 74% yields. The reactions of meta-substituted benzoic acids could efficiently proceed and afforded the desired products 5j and 5k in 81% and 53% yields, respectively. ortho-Chlorobenzoic acid and meta-,ortho-dichlorobenzoic acid could be converted to the desired 5l and 5m in 45% and 72% yields. While using ortho-methyl substituted benzoic acid or meta-, ortho-dimethylbenzoic acid as the substrates, the expected imides were detected in very low yields (<10%). In addition, α,β-unsaturated carboxyl acid (E-crotonic acid) was also viable and provided 5n in 57% yield.
Table 3 Scope of carboxylic acidsa
Reaction was performed with 1a (0.4 mmol, 2 equiv.), NFSI (2, 0.5 mmol, 2.5 equiv.), 3 (0.2 mmol), Cu(CH3CN)4PF6 (0.02 mmol, 10 mol%), L1 (0.024 mmol, 12 mol%) and LiOtBu (0.2 mmol, 1 equiv.) in anhydrous CH3CN (2 mL) under a N2 atmosphere at 40 °C. Isolated yields are given.
|
|
Interestingly, during this reaction, the C–C double bond is retained intact. Aliphatic carboxylic acids, such as 4-clorophenylacetic acid, and especially, drug molecule isoxepac acid, could smoothly participate in this reaction to form products 5o and 5p in moderate yields. This result demonstrated the four-component reaction's potential to perform late-stage functionalization and provided facile access to amine-containing drug analogues. Decanoic acid could undergo the four-component reaction to afford desired imide 5q, albeit with a low yield.
Additionally, the scope of nitriles was also examined. After slightly adjusting the reaction conditions, we were pleased to find that using dichloromethane (DCM) as the reaction solvent, the four-component reaction of 1a, 2, 3a and propionitrile (6a, 15 equiv.) worked well, producing the desired imide 7a in good yield. As seen in Table 4, the reaction of isobutyronitrile formed the corresponding product 7b in 90% yield. Other alkyl-substituted nitriles, such as benzyl cyanide and cyclopropylacetonitrile were suitable substrates to form imides 7c and 7d, albeit in diminished yields. Aryl nitriles with either electron-donating or electron-withdrawing groups at the para-, meta- or ortho-position of the benzene ring were tolerated, affording products 7e–7k in 65–99% yields. When cinnamonitrile was subjected to the reaction, the desired product 7l was obtained in 92% yield.
Table 4 Scope of nitrilesa
Reaction was performed with 1a (0.4 mmol, 2 equiv.), NFSI (2, 0.5 mmol, 2.5 equiv.), 3a (0.2 mmol), 6 (3 mmol, 15 equiv.) Cu(CH3CN)4PF6 (0.02 mmol, 10 mol%), L1 (0.024 mmol, 12 mol%) and LiOtBu (0.2 mmol, 1 equiv.) in anhydrous DCM (2 mL) under a N2 atmosphere at 40 °C for 48 h. Isolated yields are given.
|
|
To gain insight into the reaction mechanism, several control experiments were performed (Scheme 2). The addition of 2,2,6,6-tetramethylpiperidine-1-oxyl (TEMPO) sharply decreased the yield of 4a to 10% (Scheme 2a) and the use of 2,6-ditert-butyl-4-methylphenol (BHT) completely suppressed the formation of 4a (Scheme 2b). These results suggested that the reaction might involve radical intermediates. In previous reports, arylcyclopropane 1 can react with NFSI and acetonitrile to form diaminative product 8.16 Thus, the pre-prepared compound 8 was treated with carboxylic acid 3a under the standard conditions, but no desired 4a was observed (Scheme 2c). Additionally, the possible intermediate imide 9,17 was also prepared and subjected to the reaction of 1a with NFSI under standard conditions, but no desired 5g was detected (Scheme 2d).
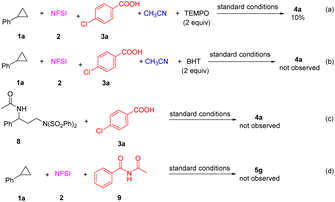 |
| Scheme 2 Control experiments. | |
Based on the above experimental results and previously reported researche studies,5a–c,15,18,19 a plausible mechanism is depicted in Scheme 3. A single electron oxidation between arylcyclopropane 1 and the Cu(III)–N species A or the radical species C occurred to form the radical cation E and the nitrogen anion D, followed by nucleophilic ring-opening and oxidization to generate the benzylic carbocation intermediate G.4a Subsequently, this intermediate was intermolecularly trapped by acetonitrile to produce nitrilium ion H. Then, a cascade nucleophilic attack of H by carboxyl acid 3a and a 1,3-acyl transfer19 occurred to furnish the desired product 4. When the para-position of the benzene ring contained a strong electron-donating group, such as the methoxyl group (1f) as the starting material, the corresponding carbocation intermediate G could be trapped by the para-chlorobenzoate anion rather than nitrile to afford aminooxylation product 4f′.20
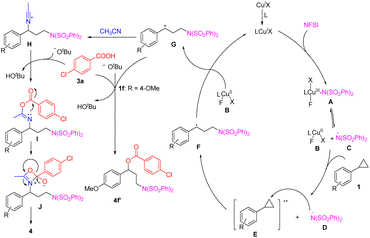 |
| Scheme 3 Proposed mechanism. | |
Conclusions
In summary, we have developed a copper-catalyzed four-component 1,3-diimidization of arylcyclopropanes with commercially available NFSI, carboxyl acids and nitriles, which represents the first example of a four-component reaction of non-D–A cyclopropanes. This strategy provides a new and effective way for synthesizing a series of imides in good to excellent yields under mild conditions. This strategy opens a new way for the multiple-component reaction of cyclopropanes.
Data availability
All experimental and characterization data in this article are available in the ESI.†
Author contributions
Y. Li, S. Yang and Q. Zhang designed the research. S. Yang and C. Liu carried out the experiments and carried out the analysis. Y. Li and Q. Zhang supervised the project. Y. Li and S. Yang co-wrote the paper. Q. Zhang guided and revised the paper. All authors read and commented on the manuscript.
Conflicts of interest
There are no conflicts to declare.
Acknowledgements
We thank the National Natural Science Foundation of China (22193012 and 21831002), the Fundamental Research Funds for the Central Universities (2412022ZD049) and the Ten Thousand Talents Program for generous financial support.
Notes and references
-
(a) H. N. C. Wong, M.-Y. Hon, C.-W. Tse, Y.-C. Yip, J. Tanko and T. Hudlicky, Chem. Rev., 1989, 89, 165–198 CrossRef CAS;
(b) C. Ebner and E. M. Carreira, Chem. Rev., 2017, 117, 11651–11679 CrossRef CAS PubMed;
(c) Y. Qin and P. Tang, Synthesis, 2012, 44, 2969–2984 CrossRef.
-
(a)
J. Zhu and H. Bienaymé, Multicomponent Reactions, Wiley-VCH, Weinheim, 2005 CrossRef;
(b) E. Ruijter, R. Scheffelaar and R. V. Orru, Angew. Chem., Int. Ed., 2011, 50, 6234–6246 CrossRef CAS PubMed;
(c) A. Domling, W. Wang and K. Wang, Chem. Rev., 2012, 112, 3083–3135 CrossRef CAS PubMed;
(d) B. H. Rotstein, S. Zaretsky, V. Rai and A. K. Yudin, Chem. Rev., 2014, 114, 8323–8359 CrossRef CAS PubMed.
-
(a) C. Sparr and R. Gilmour, Angew. Chem., Int. Ed., 2011, 50, 8391–8395 CrossRef CAS PubMed;
(b) S. Das, C. G. Daniliuc and A. Studer, Angew. Chem., Int. Ed., 2017, 56, 11554–11558 CrossRef CAS PubMed;
(c) C. H. U. Gregson, V. Ganesh and V. K. Aggarwal, Org. Lett., 2019, 21, 3412–3416 CrossRef CAS PubMed;
(d) K. Singh, T. Bera, V. Jaiswal, S. Biswas, B. Mondal, D. Das and J. Saha, J. Org. Chem., 2019, 84, 710–725 CrossRef CAS PubMed;
(e) B. Mondal, D. Das and J. Saha, Org. Lett., 2020, 22, 5115–5120 CrossRef CAS PubMed;
(f) N. O. Ilchenko, M. Hedberg and K. J. Szabó, Chem. Sci., 2017, 8, 1056–1061 RSC;
(g) V. M. Y. Leung, M. H. Gieuw, Z. Ke and Y.-Y. Yeung, Adv. Synth. Catal., 2020, 362, 2039–2044 CrossRef CAS.
-
(a) D. Petzold, P. Singh, F. Almqvist and B. König, Angew. Chem., Int. Ed., 2019, 58, 8577–8580 CrossRef CAS PubMed;
(b) L. Ge, D.-X. Wang, R. Xing, D. Ma, P. J. Walsh and C. Feng, Nat. Commun., 2019, 10, 4367–4375 CrossRef PubMed;
(c) Z. Zuo, C. G. Daniliuc and A. Studer, Angew. Chem., Int. Ed., 2021, 60, 25252–25257 CrossRef CAS PubMed.
-
(a) S. Yang, L. Wang, H. Zhang, C. Liu, L. Zhang, X. Wang, G. Zhang, Y. Li and Q. Zhang, ACS Catal., 2018, 9, 716–721 CrossRef;
(b) L. Wang, X. Wang, G. Zhang, S. Yang, Y. Li and Q. Zhang, Org. Chem. Front., 2019, 6, 2934–2938 RSC;
(c) X. Wang, L. Wang, S. Yang, L. Zhang, Y. Li and Q. Zhang, Org. Biomol. Chem., 2020, 18, 4932–4935 RSC;
(d) C. R. Pitts, B. Ling, J. A. Snyder, A. E. Bragg and T. Lectka, J. Am. Chem. Soc., 2016, 138, 6598–6609 CrossRef CAS PubMed;
(e) Y. Wang and J. M. Tanko, J. Chem. Soc., Perkin Trans. 1, 1998, 2, 2705–2712 RSC.
-
(a) S. Das, C. G. Daniliuc and A. Studer, Org. Lett., 2016, 18, 5576–5579 CrossRef CAS PubMed;
(b) S. Das, C. G. Daniliuc and A. Studer, Angew. Chem., Int. Ed., 2018, 57, 4053–4057 CrossRef CAS PubMed.
-
(a) P. J. Voorstad, J. M. Chapman, J. H. Cocolas, S. D. Wyrick and I. H. Hall, J. Med. Chem., 1985, 28, 9–12 CrossRef CAS PubMed;
(b) G. R. Pettit, Y. Kamano, C. Dufresne, R. L. Cerny, C. L. Herald and J. M. Schmidt, J. Org. Chem., 1989, 54, 6005–6006 CrossRef CAS;
(c) S. Hinterberger, O. Hofer and H. Greger, Tetrahedron, 1994, 50, 6279–6286 CrossRef CAS;
(d) D. G. Nagle, V. J. Paul, M. A. Roberts and M. A. Ypaoamide, Tetrahedron Lett., 1996, 37, 6263–6266 CrossRef CAS;
(e) A. Kamal, N. L. Gayatri, D. R. Reddy, P. S. M. Mohan Reddy, M. Arifuddin, S. G. Dastidar, A. K. Kondapi and M. Rajkumar, Bioorg. Med. Chem., 2005, 13, 6218–6225 CrossRef CAS PubMed;
(f) T. Pacher, A. Raninger, E. Lorbeer, L. Brecker, P. P.-H. But and H. Greger, J. Nat. Prod., 2010, 73, 1389–1393 CrossRef CAS PubMed;
(g) G. M. Keating, Drugs, 2017, 77, 459–472 CrossRef CAS PubMed;
(h) A. V. Bogolubsky, Y. S. Moroz, O. Savych, S. Pipko, A. Konovets, M. O. Platonov, O. V. Vasylchenko, V. V. Hurmach and O. O. Grygorenko, ACS Comb. Sci., 2017, 20, 35–43 CrossRef PubMed;
(i) S. K. Misra, T. L. Kampert and D. Pan, Bioconjugate Chem., 2018, 29, 1419–1427 CrossRef CAS PubMed;
(j) C. Zhao, Z. Ye, Z.-X. Ma, S. A. Wildman, S. A. Blaszczyk, L. Hu, I. A. Guizei and W. Tang, Nat. Commun., 2019, 10, 4015 CrossRef PubMed;
(k) R. Sunnapu, S. N. Banoth, R. S. Reyno, A. Thomas, N. Venugopal and G. Rajendar, J. Org. Chem., 2020, 85, 4103–4113 CrossRef CAS PubMed.
-
(a) X. Chen, C. Xu, T. Wang, C. Zhou, J. Du, Z. Wang, H. Xu, T. Xie, G. Bi, J. Jiang, X. Zhang, J. N. Demas, C. O. Trindle, Y. Luo and G. Zhang, Angew. Chem., Int. Ed., 2016, 55, 9872–9876 CrossRef CAS PubMed;
(b) L. I. Buruiana, A. I. Barzic, I. Stoica and C. Hulubei, J. Polym. Res., 2016, 23, 217 CrossRef;
(c) S. Naqvi, M. Kumar and R. Kumar, ACS Omega, 2019, 4, 19735–19745 CrossRef CAS PubMed;
(d) D. Çakal, A. Cihaner and A. M. Önal, J. Electroanal. Chem., 2020, 862, 114000 CrossRef;
(e) K. Yang, X. Zhang, A. Harbuzaru, L. Wang, Y. Wang, C. Koh, H. Guo, Y. Shi, J. Chen, H. Sun, K. Feng, M. C. Ruiz Delgado, H. Y. Woo, R. P. Ortiz and X. Guo, J. Am. Chem. Soc., 2020, 142, 4329–4340 CrossRef CAS PubMed.
-
(a) M. S. Taylor and E. N. Jacobsen, J. Am. Chem. Soc., 2003, 125, 11204–11205 CrossRef CAS PubMed;
(b) M. P. Sibi, N. Prabagaran, S. G. Ghorpade and C. P. Jasperse, J. Am. Chem. Soc., 2003, 125, 11796–11797 CrossRef CAS PubMed.
-
(a)
O. H. Wheeler and O. Rosado, Chemistry of imidic com-pounds, in The Chemistry of Amides, 1970, pp. 335–381 Search PubMed;
(b) M. B. Andrus, W. Li and R. F. Keyes, Tetrahedron Lett., 1998, 39, 5465–5468 CrossRef CAS;
(c) N. Mushtaq, Q. Wang, G. Chen, B. Bashir, H. Lao, Y. Zhang, L. R. Sidra and X. Fang, Polymer, 2020, 190, 122218–122228 CrossRef CAS.
-
(a) J. Sperry, Synthesis, 2011, 22, 3569–3580 CrossRef;
(b) W. Huang and M. L. Xu, J. Chem. Res., 2013, 37, 77–79 CrossRef CAS;
(c) I. Itoh, Y. Matsusaki, A. Fujiya, N. Tada, T. Miura and A. Itoh, Tetrahedron Lett., 2014, 55, 3146–3148 Search PubMed;
(d) W. Lu, C. Mei and Y. Hu, Synthesis, 2018, 50, 2999–3005 CrossRef.
-
(a) K. M. Majerski, R. Margeta and J. Veljkoviç, Synlett, 2005, 2089–2091 CrossRef;
(b) E. M. Nasr, M. Montazerozohori and N. Filvan, J. Serb. Chem. Soc., 2012, 77, 415–421 CrossRef;
(c) M. M. Khodaei and E. Nazari, Tetrahedron Lett., 2012, 53, 2881–2884 CrossRef CAS.
-
(a) R. B. Bates, F. A. Fletcher, K. D. Janda and W. A. Miller, J. Org. Chem., 1984, 49, 3038 CrossRef CAS;
(b) D. A. Evans, P. Nagorny and R. Xu, Org. Lett., 2007, 8, 5669–5671 CrossRef PubMed;
(c) Y. Peng, C. T. Feng, Y. Q. Li, F. X. Chen and K. Xu, Org. Biomol. Chem., 2019, 17, 6570–6573 RSC.
- H. Li, X. Fang, R. Jackstell, H. Neumann and M. Beller, Chem. Commun., 2016, 52, 7142–7145 RSC.
- X. Zhang, T. Cui, X. Zhao, P. Liu and P. Sun, Angew. Chem., Int. Ed., 2020, 59, 3465–3469 CrossRef CAS PubMed.
-
(a) H. Zhang, W. Pu, T. Xiong, Y. Li, X. Zhou, K. Sun, Q. Liu and Q. Zhang, Angew. Chem., Int. Ed., 2013, 52, 2529–2533 CrossRef CAS PubMed;
(b) M. Skvorcova, L. T. Lukasevics and A. Jirgensons, J. Org. Chem., 2019, 84, 3780–3792 CrossRef CAS PubMed.
-
(a) R. H. Wiley and W. B. Guerrant, J. Am. Chem. Soc., 1949, 71, 981–982 CrossRef CAS;
(b) K. Mlinarić-Majerski, R. Margeta and J. Veljković, Synlett, 2005, 13, 2089–2091 CrossRef;
(c) M. M. Khodaei and E. Nazari, Tetrahedron Lett., 2012, 53, 2881–2884 CrossRef CAS.
-
(a) Y. Shen, Q. Li, G. Xu and S. Cui, Org. Lett., 2018, 20, 5194–5197 CrossRef CAS PubMed;
(b) J. Chen, Y. Shao, L. Ma, M. Ma and X. Wan, Org. Biomol. Chem., 2016, 14, 10723–10732 RSC;
(c) L. Vanoye, A. Ham-moud, H. Gérard, A. Barnes, R. Philippe, P. Fongarland, C. de Bellefon and A. Favre-Réguillon, ACS Catal., 2019, 9, 9705–9714 CrossRef CAS.
-
(a) D. Y. Curtin and L. L. Miller, Tetrahedron Lett., 1965, 6, 1869–1876 CrossRef;
(b) J. S. P. Schwarz, J. Org. Chem., 1972, 37, 2906–2908 CrossRef CAS;
(c) K. Brady and A. F. Hegarty, J. Chem. Soc., Perkin Trans. 1, 1980, 2, 121–126 RSC.
- D. Wu, S. S. Cui, Y. Lin, L. Li and W. Yu, J. Org. Chem., 2019, 84, 10978–10989 CrossRef CAS PubMed.
|
This journal is © The Royal Society of Chemistry 2022 |
Click here to see how this site uses Cookies. View our privacy policy here.