DOI:
10.1039/D2SD00119E
(Communication)
Sens. Diagn., 2022,
1, 977-982
Ultrafast screening of serum for early prediction of diabetes by fractalkine detection†
Received
12th July 2022
, Accepted 13th August 2022
First published on 24th August 2022
Abstract
Fractalkine is a key biomarker for early diagnosis of diabetes. A smartphone-based mobile device was proposed for the fast screening of serum samples for fractalkine in order to detect the prediabetes stage. The smart device is able to send the data to the cellphone of the patient and of the medical doctor using bluetooth. A screen-printed stochastic electrode was used as the detection tool in the cellular-based mobile device. The sensitivity of the screen-printed stochastic electrode is very high (3.58 × 103 s−1 g−1 mL), and the limit of determination is very low (2.05 fg mL−1). The screening method is able to perform a qualitative analysis as well as a highly reliable quantitative analysis of fractalkine in serum samples with a recovery (%) vs. ELISA (the standard method) of 99.96 and a relative standard deviation of 3.28. The cost per analysis is very low. No special training is needed for operation of the device.
Introduction
Fractalkine (FKN) is a peptide chemokine and the only member of the CX3C family that is produced as a membrane-anchored protein. FKN has proved to be an excellent biomarker for prediabetes,1 at a stage where diabetes (a disease with increased prevalence all over the world2,3) can still be reversed. It is involved in promoting integrin-independent adhesion4 and could be a mediator of local inflammatory cell attraction in pancreatic beta cells. The increased levels of inflammatory cells together with chemokines or adipocytokines5 could lead to beta cells' damage. This translates into altered insulin secretion and diabetes onset. Previous studies have shown that serum FKN levels are higher in prediabetes (only impaired glucose tolerance included) and type 2 diabetes, compared to the normal levels. The levels of FKN were similar between prediabetes and diabetes groups. This could be explained by a compensatory serum increase before diabetes starts.6 To date, the only method proposed for the assay of FKN is the one proposed by Bio-Rad Laboratories Inc. (Bio-Plex Pro™ system) based on ELISA.
Given the importance of the determination of FKN, we developed a smart cellphone-based mobile device for the fast screening of serum samples for FKN. The tool used for the smart device is a screen-printed stochastic sensor based on a MWCNT matrix chemically modified with –COOH and also physically impregnated with maltodextrin. The choice of the stochastic electrode as a tool is based on the performance of stochastic electrodes when used for biomedical analysis.7–10 The main advantages of these electrodes are: they perform reliable qualitative analysis based on the signature of the analytes; they also perform reliable quantitative analysis which does not depend on the complexity of the matrix, where amounts up to fg mL−1 are able to be detected from very complex matrices.7–10
Utilization of a 2D stochastic sensor for screening tests of serum for FKN versus conventional techniques such as ELISA has the following advantages: ultrafast detection, higher sensitivity, and cost-effectiveness. Furthermore, involvement of new technology based on miniaturized instrumentation/potentiostat controlled by a phone, able to perform data acquisition and data processing, makes the method accessible for any user, from patients to nurses and to medical doctors. The new technology supports the new challenge in the biomedical analysis where highly reliable portable instruments controlled by smart phones are requested.11 Sensor technology has improved in the past few years: new nanomaterials were synthesized and used,11–13 new techniques were employed in order to increase the selectivity
:
sensitivity ratio,11 and smart devices were constructed for wireless data acquisition and processing.13 Therefore, this paper is in line with the new technology, by proposing a miniaturized device driven by a smartphone for screening tests of serum for FKN, a biomarker which can be used for early diagnosis of diabetes, at the stage where diabetes is reversible.
Experimental
Materials and reagents
All chemicals were of analytical grade. Fractalkine, maltodextrin (MD) and bovine serum albumin (BSA) were purchased from Sigma Aldrich. Deionized water, obtained from a Millipore Direct-Q 3 system, was used for the preparation of the fractalkine solutions, with different concentrations (2.5 μg mL−1 to 1.0 fg mL−1) using the serial dilution method. All fractalkine solutions were prepared in a mixture of phosphate buffer solution (PBS, pH = 7.5) and BSA (0.1%). When not in use, the solutions were kept at a temperature of −20 °C. The screen-printed electrode was purchased from Methrom, DropSens.
Apparatus and methods
In order to record all measurements, a miniaturized Sensit/BT potentiostat (from PalmSens) connected to a cellphone using PSTrace (version 5.6) software was used. A screen-printed carbon electrode (from Metrohm DropSens), containing a three-electrode system, was also employed. The three-electrode system contains the following: the working electrode which is made out of carbon (MWCNT-COOH), the auxiliary electrode made out of carbon, and the reference electrode made out of silver.
ELISA
The standard method of analysis was ELISA used by the Bio-Plex Pro™ system (Bio-Rad Laboratories Inc). In this system, the reactions take place at the level of magnetic spheres/beads, working similarly to the sandwich ELISA reaction. Capture antibodies are bound to magnetic spheres in order to bind the biomarker (chemokine) of interest. After a series of washes in order to remove other proteins that have not bound to specific antibodies, another biotinylated antibody is added which has the role of further fixing the streptavidin–phycoerythrin conjugate (streptavidin–phycoerythrin SA–PE). SA–PE will ultimately serve as a marker of fluorescence. This signal is detected by the Bio-Plex system. When a multiplex reaction suspension is introduced into the Bio-Plex Reader200, a red (635 nm) laser will illuminate the fluorescent marker on each sphere to classify and identify it. At the same time, a green laser (532 nm) will stimulate the PE to generate a reporter signal that will be detected by the PMT (photomultiplier tube). A high-speed digital processor will record the data obtained, and the Bio-Plex Manager system will present them as median fluorescence (MFI), respectively, with the concentration in pg mL−1. The concentration of the analyte that will bind to each sphere is proportional to the MFI.
Design of the stochastic screen-printed electrode
The surface of the stochastic screen-printed electrode is based on carbon modified with carboxyl functionalised multi-walled carbon nanotubes (MWCNT–COOH); the selection of the MWCNT type of matrix for the design of the sensor is due to the stability that it gives to the channels of the molecules like maltodextrins for stochastic detection. The diameter of the ceramic substrate was L33 × W10 × H0.5 mm. The active surface of the stochastic screen-printed electrode was modified using a solution of maltodextrin (MD, MD is a molecule able to provide the necessary channels needed in stochastic sensing); 10 μL solution of MD (10−3 mol L−1) was dropped onto the active surface and left overnight (for 24 h) at room temperature to dry. Before and after each measurement, the sensor was cleaned with deionized water. When not in use, the stochastic screen-printed electrode was kept at room temperature, in a dry place.
Stochastic mode
The stochastic mode was used in order to perform all of these measurements. The principle of the stochastic sensors is based on the channel conductivity, when a constant potential of 125 mV was applied and the current was recorded.10 Screening of 1 pg mL−1 FKN solution was done at potentials ranging from 10 mV to 200 mV; the criterion for the selection of the optimum potential was the value of toff (signature of FKN) which should be on the order of ms to s; values recorded for potentials lower than 125 mV were on the order of minutes while values recorded for potentials higher than 125 mV were on the order of ns; therefore the optimum potential chosen was 125 mV. The measured parameters were toff and ton. Using the toff value, which is specific to FKN, the FKN was identified in the recorded diagrams. The ton values determined for concentrations in the range between 2.5 μg mL−1 and 1.0 fg mL−1 were used to find the linear concentration range and the equation of calibration of the proposed sensor; in this regard, the linear regression method was used. The unknown concentrations for FKN were determined using the ton values, which were identified for FKN in the obtained diagrams.
Design of the measurement using the smart cellphone-based device
The designed stochastic screen-printed electrode was inserted into the smart device (Fig. 1), a drop of serum was added on top of the stochastic screen printed electrode (step 1), and the measurement was started (step 2); the diagram was automatically sent to a cellphone by using the bluetooth mode. After the measurement was finalized, the signature of FKN was identified using the software developed in our laboratory. The same software was used to measure the ton value between two toff values (Fig. 2) in which also the ton values were automatically inserted in the equation of calibration to obtain the concentration of FKN.
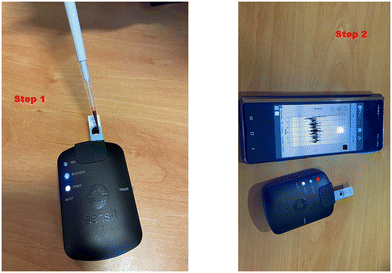 |
| Fig. 1 Measurements with a smart cellphone-based device. | |
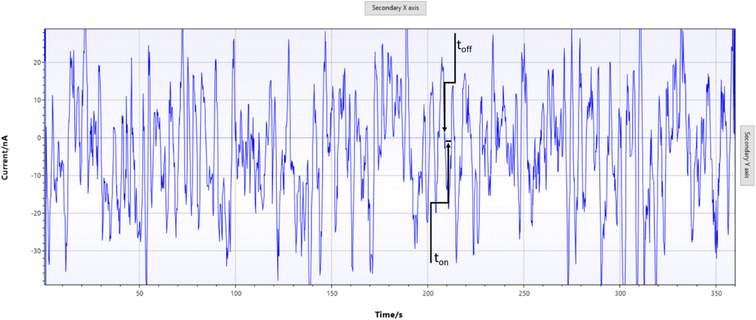 |
| Fig. 2 Pattern recognition of fractalkine in serum samples using stochastic screen-printed electrodes. | |
Samples
Serum samples were received from the university hospitals from Bucharest. Samples from confirmed subjects with diabetes as well as from subjects that had a family history of diabetes were collected according to the procedures specified in the Ethics Committee approval number 75/2015 awarded by the University of Medicine and Pharmacy “Carol Davila” from Bucharest. Written consent was obtained from all patients. No sample pre-treatment was performed. The samples were chosen from the biobank of a clinical study regarding levels of FKN in the categories of subjects mentioned above; the clinical study was performed using both methods ELISA and the fast screening method based on the utilization of stochastic screen-printed electrodes.
Results and discussion
Response characteristics of the stochastic screen-printed electrode
When a constant potential of 125 mV is applied, the current which flows through a channel is modified when FKN goes through the channel and binds with the wall channel. The current development takes place in two phases: in the first phase (known as the molecular recognition phase), the FKN enters the channel blocking it, and the intensity of the current decreases at around the 0 value for a certain period of time, which is known as the signature of FKN, and it is marked in the diagram as toff (Fig. 2); its value is used for the qualitative analysis of FKN. The second phase is known as the reaction phase, due to the fact that FKN interacts with the wall channel10 when the following equation of equilibrium takes place:
Ch(i) + fractalkine(i) ⇔ Ch·fractalkine(i) |
where Ch represents the channel and i represents the interface, and redox processes also take place. The quantitative parameter, also known as ton, represents the equilibrium time necessary for the reaction phase; the concentration of FKN is dependent on its value.
The signature (toff value) recorded for FKN was 0.3 s. The equation of calibration of the stochastic screen-printed electrode is:
1/ton = 0.34 + 3.5 × 103 × Conc.FKN 〈ton〉 = s; 〈Conc.FKN〉 = g mL−1 |
with an
r value of 0.9999.
The sensitivity of the stochastic screen-printed electrode was very high 3.58 × 103 s−1 g−1 mL, while the limit of quantification (the limit of quantification is given by the lowest concentration found in the linear concentration range accordingly with the new IUPAC recommendation (paragraph 3.36, Note 3)14 see the ESI†) of FKN was very low: 2.05 fg mL−1. The stochastic screen-printed electrode can be used in a wide linear concentration range, between 2.05 fg mL−1 and 0.5 μg mL−1.
The selectivity of stochastic sensors is given by the signatures (toff) recorded for the supposed interferences versus the signature (toff) of the biomarker (FKN). D-Glucose, leptin, insulin, proinsulin C peptide, and adiponectin were chosen as the possible interfering species. Their signatures determined using the proposed sensor were: 1.1 s for D-glucose, 0.7 s for leptin, 1.6 s for insulin, 2.0 s for proinsulin C peptide, and 2.8 s for adiponectin. There are significant differences between the signature obtained for FKN and the signatures determined for D-glucose, leptin, insulin, proinsulin C peptide, and adiponectin proving the selectivity of the proposed stochastic sensor.
The reliability and stability of the stochastic screen-printed electrode
Ten stochastic screen-printed electrodes coupled to the smart cellphone-based device were separately designed and tested for one year. During this period of time, their sensitivity varied with 0.98%, proving that the design is reliable, and the stability in time is good.
Screening tests of the serum samples using the stochastic screen-printed electrode coupled to the smart cellphone-based device
Serum samples were screened as described above (Fig. 1). For the validation, taking into account that the standard method (ELISA) was able to determine FKN only from serum samples, we will show the results obtained for the screening of serum samples using the stochastic screen-printed electrode coupled to the smart cellphone-based device. The results obtained using the proposed screening instrumentation and method were compared with the results obtained using the ELISA (Table 1). In order to determine FKN from the diagrams (Fig. 2), first we performed the molecular recognition step based on the identification of the signature of FKN in the diagram. The reading of ton was done just after the toff of FKN (Fig. 2).
Table 1 Determination of fractalkine in serum samples using the proposed method and the standard method (ELISA)
Sample no. |
Fractalkine, pg mL−1 |
Standard method, ELISA |
Screening tests of the serum samples using the stochastic screen-printed electrode coupled to the smartphone-based device |
1 |
690.39 |
689.27 ± 0.07 |
2 |
328.12 |
320.18 ± 0.07 |
3 |
242.44 |
241.56 ± 0.04 |
4 |
1928.49 |
1915.43 ± 0.05 |
5 |
163.50 |
160.00 ± 0.03 |
6 |
233.01 |
255.08 ± 0.07 |
7 |
211.11 |
238.46 ± 0.04 |
8 |
271.95 |
249.25 ± 0.08 |
9 |
221.93 |
225.39 ± 0.04 |
10 |
4204.33 |
4201.76 ± 0.06 |
11 |
236.76 |
255.09 ± 0.07 |
12 |
221.93 |
254.36 ± 0.08 |
13 |
316.76 |
320.00 ± 0.04 |
14 |
278.08 |
255.08 ± 0.07 |
15 |
85.28 |
80.22 ± 0.08 |
16 |
74.98 |
75.36 ± 0.02 |
17 |
128.76 |
120.78 ± 0.08 |
18 |
206.67 |
205.09 ± 0.07 |
19 |
100.07 |
105.44 ± 0.07 |
20 |
101.56 |
106.46 ± 0.04 |
21 |
149.05 |
159.82 ± 0.03 |
22 |
174.17 |
160.00 ± 0.05 |
23 |
299.16 |
255.10 ± 0.06 |
24 |
103.05 |
105.43 ± 0.08 |
25 |
76.45 |
76.40 ± 0.05 |
26 |
113.54 |
159.16 ± 0.08 |
27 |
127.23 |
160.00 ± 0.09 |
28 |
177.50 |
160.00 ± 0.08 |
29 |
131.84 |
140.39 ± 0.04 |
30 |
233.94 |
285.02 ± 0.09 |
31 |
133.39 |
132.72 ± 0.04 |
32 |
142.74 |
140.39 ± 0.07 |
33 |
115.05 |
120.78 ± 0.08 |
34 |
158.64 |
160.00 ± 0.02 |
35 |
244.35 |
255.08 ± 0.04 |
36 |
191.88 |
160.40 ± 0.08 |
37 |
387.04 |
382.77 ± 0.05 |
38 |
76.45 |
70.22 ± 0.08 |
39 |
231.14 |
255.08 ± 0.07 |
40 |
133.39 |
132.72 ± 0.04 |
41 |
88.23 |
91.24 ± 0.07 |
42 |
181.68 |
162.03 ± 0.08 |
43 |
134.94 |
132.72 ± 0.04 |
44 |
419.64 |
417.97 ± 0.07 |
45 |
121.12 |
120.78 ± 0.05 |
46 |
188.46 |
207.54 ± 0.08 |
47 |
112.04 |
105.44 ± 0.07 |
48 |
116.56 |
105.43 ± 0.08 |
49 |
162.69 |
180.26 ± 0.07 |
50 |
454.73 |
452.50 ± 0.09 |
51 |
250.12 |
255.08 ± 0.04 |
52 |
323.54 |
328.77 ± 0.03 |
53 |
202.28 |
205.74 ± 0.07 |
54 |
176.66 |
185.51 ± 0.08 |
55 |
190.16 |
190.00 ± 0.03 |
56 |
263.89 |
255.28 ± 0.08 |
57 |
160.26 |
160.00 ± 0.02 |
A good correlation between the results of the screening tests of the serum samples using the stochastic screen-printed electrode coupled to the smart cellphone-based device and those of ELISA was obtained. This proved that the proposed method and instrumentation can be used reliably for the screening tests of serum in order to determine FKN. The average recovery (%) vs. ELISA (the standard method) was 99.96 with a relative standard deviation of 3.28.
Clinical studies performed by Sindhu et al.15 using immunofluorescence showed concentrations of FKN significantly higher in type 2 diabetes subjects (higher than 166 ± 14.22 pg mL−1) versus healthy subjects (lower than 118 ± 8.90 pg mL−1). We also performed some clinical studies regarding the level of concentrations in prediabetes, obese, and healthy subjects using immunofluorescence and the screen-printed stochastic sensor developed by us;16 elevated levels of FKN were observed in prediabetes subjects (higher than 221.93 ± 18.46 pg mL−1) versus obese subjects (between 182.89 and 191.5 pg mL−1) or healthy subjects (less than 150.22 ± 67.14 pg mL−1). The linear concentration range (2.05 fg mL−1 and 0.5 μg mL−1) in which the stochastic sensor can be used covered well all categories: diabetic and prediabetic subjects, as well as healthy subjects.
Conclusions
A smartphone-based mobile device was proposed for the fast screening of serum samples for fractalkine in order to detect the prediabetes stage. The linear concentration range and the sensitivity of the stochastic screen-printed electrode made possible the determination of FKN in prediabetes and diabetes stages. The system is highly reliable when used for the screening tests of serum; the analysis are cost-effective (very low cost per analysis compared with ELISA). No special training is needed for the operation of the device. The results can be obtained within 6 min. The main feature of the instrumentation and method is their utilization for screening tests of whole blood especially for people prone to develop diabetes, in order to have a diagnosis as early as possible for prediabetes, at a stage where it is still reversible.
Author contributions
Conceptualization: R. I. S. vS.; methodology: R. I. S. vS., C. D. G., and R. A. S.; validation: R. I. S. vS., C. D. G., and R. A. S.; formal analysis: R. I. S. vS., C. D. G., and R. A. S.; investigation: C. D. G. and R. A. S.; writing – original draft preparation: C. D. G. and R. A. S.; writing – review and editing: R. I. S. vS., C. D. G., and R. A. S.; supervision: R. I. S. vS.; funding acquisition: R. I. S. vS. All authors have read and agreed to the published version of the manuscript.
Conflicts of interest
The authors declare no competing interest.
Acknowledgements
This work was supported by the UEFISCDI, PNCDI III framework, PCE project, Stochastic (micro)sensors used for determination of biomarkers specific for diabetes (SCREENSTOCDIA) contract nr 46/2017.
References
- American Diabetes Association (ADA). Classification and Diagnosis of Diabetes: Standards of Medical Care in Diabetes-2020, Diabetes Care, 2020, 43, S14–S31 Search PubMed.
- M. Mota, S. G. Popa, E. Mota, A. Mitrea, D. Catrinoiu, D. M. Cheta, C. Guja, N. Hancu, C. Ionescu-Tirgoviste, R. Lichiardopol, B. M. Mihai, A. R. Popa, C. Zetu, C. G. Bala, G. Roman, C. Serafinceanu, V. Serban, R. Timar, I. A. Veresiu and A. R. Vlad, Prevalence of diabetes mellitus and prediabetes in the adult Romanian population: PREDATORR study, J. Diabetes, 2016, 8, 336–344 CrossRef CAS PubMed.
- A. G. Tabák, C. Herder, W. Rathmann, E. J. Brunner and M. Kivimäki, Prediabetes: a high-risk state for diabetes development, Lancet, 2012, 379, 2279–2290 CrossRef.
- K. W. Kim, A. Vallon-Eberhard, E. Zigmond, J. Farache, E. Shezen, G. Shakhar, A. Ludwig, S. A. Lira and S. Jung, In vivo structure/function and expression analysis of the CX3C chemokine fractalkine, Blood, 2011, 118, e156–e167 CrossRef CAS PubMed.
- S. J. Dunmore and J. E. Brown, The role of adipokines in β-cell failure of type 2 diabetes, J. Endocrinol., 2013, 216, T37–T45 CAS.
- S. Baldane, S. H. Ipekci, A. Ekin, S. Abusoglu, A. Unlu and L. Kebapcilar, Evaluation of fractalkine (FKN) and secreted frizzled-related protein 4 (SFRP-4) serum levels in patients with prediabetes and type 2 diabetes, Bratisl. Lek. Listy, 2018, 119, 112–115 CAS.
- R. I. Stefan-van Staden, R. M. Ilie-Mihai, F. Pogacean and S. Pruneanu, Graphene-based stochastic sensors for pattern recognition of gastric cancer biomarkers in biological fluids, J. Porphyrins Phthalocyanines, 2019, 23, 1365–1370 CrossRef CAS.
- R. M. Ilie-Mihai, R. I. Stefan-van Staden, A. Lungu-Moscalu, F. Pogacean and S. M. Pruneanu, Sulphur Doped Graphenes Based 3D-Needle Stochastic Sensors as New Tools for Biomedical Analysis, J. Electrochem. Soc., 2021, 168, 037509 CrossRef CAS.
- R. M. Ilie-Mihai, S. S. Gheorghe, R. I. Stefan-van Staden and A. Bratei, Electroanalysis of interleukins 1β, 6, and 12 in biological samples using a needle stochastic sensor based on nanodiamond paste, Electroanalysis, 2021, 33, 6–10 CrossRef CAS.
- R. I. Stefan-van Staden, C. C. Negut, S. S. Gheorghe and A. Ciorita, 3D stochastic microsensors for molecular recognition and determination of heregulin-α in biological samples, Anal. Bioanal. Chem., 2021, 413, 3487–3492 CrossRef CAS PubMed.
- R. I. Stefan-van Staden, Challenges in Biomedical Analysis - From Classical Sensors to Stochastic Sensors, ECS Sens. Plus, 2022, 1, 011603 CrossRef.
- V. Chaudhary, N. Ashraf, M. Khalid, R. Walvekar, Y. Yang, A. Kaushik and Y. K. Mishra, Emergence of mxene-polymer hybrid nanocomposites as high-performance next generation chemiresistor for efficient air quality monitoring, Adv. Funct. Mater., 2022, 211913 Search PubMed.
- V. Chaudary, A. Kaushik, H. Furukawa and A. Khosla, Review-towards 5th generation AI and IoT driven sustainable intelligent sensors based on 2D mxenes and borophene, ECS Sens. Plus, 2022, 1, 013601 CrossRef.
- D. B. Hibbert, E. H. Korte and U. Örnemark, IUPAC Recommendations. Metrological and quality concepts in analytical chemistry (IUPAC Recommendations 2021), Pure Appl. Chem., 2021, 93, 997–1048 CrossRef CAS.
- S. Sindhu, N. Akhter, H. Arefanian, A. A. Al-Roub, S. Ali, A. Wilson, A. Al-Hubail, S. Al-Beloushi, S. Al-Zanki and R. Ahmad, Increased circulatory levels of fractalkine (CX3CL1) are associated with inflammatory chemokines and cytokines in individuals with type-2 diabetes, J. Diabetes Metab. Disord., 2017, 16, 15 CrossRef PubMed.
- R. A. Stoica, N. Drăgana, R. Ancuceanu, O. I. Geicu, C. Guja, A. Pantea-Stoian, D. C. Gheorghe, R. I. Stefan-van Staden, C. Serafinceanu, A. Costache and C. Ionescu-Tîrgovişte, Interleukin-8, CXCL10, CXCL11 and their role in insulin resistance in adult females with subclinical hypothyroidism and prediabetes, J. Clin. Transl. Endocrinol., 2022, 28, 100299 CAS.
|
This journal is © The Royal Society of Chemistry 2022 |
Click here to see how this site uses Cookies. View our privacy policy here.