DOI:
10.1039/D0CS01051K
(Review Article)
Chem. Soc. Rev., 2023,
52, 1697-1722
The role of the light source in antimicrobial photodynamic therapy
Received
16th June 2022
First published on 13th February 2023
Abstract
Antimicrobial photodynamic therapy (APDT) is a promising approach to fight the growing problem of antimicrobial resistance that threatens health care, food security and agriculture. APDT uses light to excite a light-activated chemical (photosensitiser), leading to the generation of reactive oxygen species (ROS). Many APDT studies confirm its efficacy in vitro and in vivo against bacteria, fungi, viruses and parasites. However, the development of the field is focused on exploring potential targets and developing new photosensitisers. The role of light, a crucial element for ROS production, has been neglected. What are the main parameters essential for effective photosensitiser activation? Does an optimal light radiant exposure exist? And finally, which light source is best? Many reports have described the promising antibacterial effects of APDT in vitro, however, its application in vivo, especially in clinical settings remains very limited. The restricted availability may partially be due to a lack of standard conditions or protocols, arising from the diversity of selected photosensitising agents (PS), variable testing conditions including light sources used for PS activation and methods of measuring anti-bacterial activity and their effectiveness in treating bacterial infections. We thus sought to systematically review and examine the evidence from existing studies on APDT associated with the light source used. We show how the reduction of pathogens depends on the light source applied, radiant exposure and irradiance of light used, and type of pathogen, and so critically appraise the current state of development of APDT and areas to be addressed in future studies. We anticipate that further standardisation of the experimental conditions will help the field advance, and suggest key optical and biological parameters that should be reported in all APDT studies. More in vivo and clinical studies are needed and are expected to be facilitated by advances in light sources, leading to APDT becoming a sustainable, alternative therapeutic option for bacterial and other microbial infections in the future.
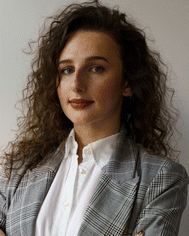
Marta Piksa
| Marta Piksa obtained her BSc and MSc degrees from Wrocław University of Science and Technology (Faculty of Chemistry). Currently, she is pursuing her PhD degree at the Wrocław Doctoral School of Institutes of Polish Academy of Sciences at the Hirszfeld Institute of Immunology and Experimental Therapy PAS. Since her master's studies, her research interest is antimicrobial photodynamic therapy (APDT), and her PhD thesis is focused on the mechanisms of bacterial cell photoinactivation. |
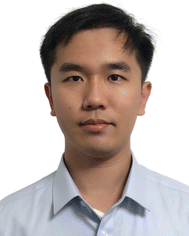
Cheng Lian
| Cheng Lian obtained his BEng in Light sources and Illumination from Taiyuan University of Technology. He completed an MSc in Green Technology from Hong Kong Baptist University. In 2022, he received his PhD from the University of St Andrews for a thesis on “Organic light-emitting diodes for biomedical applications” supervised by Prof. Ifor Samuel. Currently, he is working in BOE Technology Group Co., Ltd. |
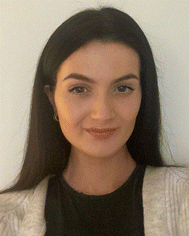
Imogen C. Samuel
| Imogen Samuel is currently a final year medical student at the University of Manchester. She completed an intercalated degree in Biomedical Science in 2021. She developed an interest in photodynamic therapy and dermatology during placements at Ninewells Hospital in Dundee and Salford Royal Hospital. |
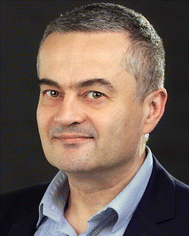
Krzysztof j. Pawlik
| Krzysztof Jakub Pawlik earned his PhD from the Hirszfeld Institute of Immunology and Experimental Therapy in 2002. In the years 2002-2005 he got postdoc fellowship at University of Paris Sud IX and stayed as a research fellow at Department of Microbiology at University of Tübingen. His research interests focus on the molecular basis of secondary metabolites synthesis. He is particularly interested in the synthesis of polyketides by Streptomyces bacteria. Since 2018 he cooperates with Prof. K. Matczyszyn from Wrocław University of Science and Technology to develop an application of photodynamic therapy against pathogens of skin infections. |
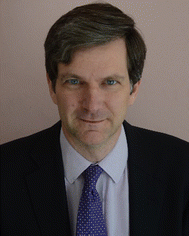
Ifor D. W. Samuel
| Ifor Samuel is Professor of Physics at the University of St Andrews. He received his MA and PhD from the University of Cambridge, working on optical spectroscopy of organic semiconductors. He was a Research Fellow at Christ's College, Cambridge and also performed postdoctoral work at CNET-France Telecom in Paris, before setting up his own research group on light-emitting polymers at the University of Durham. In 2000 he moved to the University of St Andrews where he founded and leads the Organic Semiconductor Centre. His current work concerns the photophysics of organic semiconductor materials and devices, together with their applications including photodynamic therapy. |
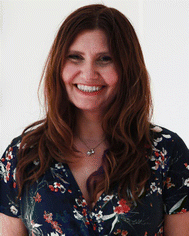
Katarzyna Matczyszyn
| Katarzyna Matczyszyn is a physical chemist and Wroclaw University of Science and Technology professor. Prior to her position in Poland she worked at CEA Saclay, Ecole Normale Superieure de Cachan and Université Pierre et Marie Curie in France. Her major scientific interest falls into light-matter interactions, especially on biologically significant materials. She works with photoactive molecules (mostly photochromes), various types of nanoparticles (including plasmonic and carbon nanodots), lyotropic liquid crystals and in the field of nonlinear optics. She is particularly keen on novel approaches to photodynamic therapy. |
1. Introduction
Due to the widespread use of antibiotics in various areas of our life, from medicine to farming, we are currently facing an ever-increasing threat from antimicrobial resistance (AMR)1–6 which, is so severe that it has been called the “post-antibiotic apocalypse”.7,8 We need to explore alternative ways to kill infectious bacteria, as otherwise many diseases, routine surgery and injuries could lead to serious infections, sepsis and death. The problem applies not only to bacterial infections, but also those caused by fungi and parasites. The dynamic expansion of AMR has reached even the most remote and uninhabited areas of the planet, such as High Arctic soils where in 2019 antibiotic resistance genes were discovered.9 It has been shown that antibiotic resistance can develop very quickly – in one study wild type Escherichia coli took just eleven days to develop mutations allowing them to survive in an antibiotic at 1000 times the concentration that was initially lethal.10 A serious effect of this worldwide crisis is increasing mortality among patients infected by drug resistant pathogens.11–15 Importantly, AMR can also lead to amputation in some cases of incurable bacterial infections.16,17
Photodynamic therapy (PDT) provides an alternative way of killing bacteria.18 It uses light in combination with a light-activated chemical (photosensitiser) in the presence of oxygen to generate reactive oxygen species (ROS) that kill nearby cells. It is commonly used to treat many skin cancers, and is also used for the treatment and palliative care of internal cancers.19–21 There is now great interest in the ability of PDT to kill bacteria and other microbes. Antimicrobial Photodynamic Therapy (APDT) is known by many names such as Antimicrobial Photodynamic Inactivation (aPDI/APDI)22–26 Antimicrobial Photoinactivation,27–29 Antimicrobial Photodynamic Therapy (aPDT/APDT),30–37 lethal photosensitization,38–40 photoactivated disinfection (PAD)41–43(in the dental field), Photodynamic Antimicrobial Chemotherapy (PACT)44,45 and Photodynamic Inactivation of Bacteria (PIB).46–48 All these therapies differ only in the name as they all work in the same way i.e. by using light to activate a photosensitiser that generates ROS that kill the pathogen. In this review, the term APDT will be used for the rest of the article, regardless of whether the studies were conducted in vivo or in vitro.
Photosensitisers can undergo two types of photochemical reactions, both using triplet oxygen (3O23Σg−) as a reagent. Type I, is based on the production of superoxide anions (O2−), leading to the formation of various free radicals (including hydroxyl radicals HO˙, peroxyl radicals ROO˙ and alkoxyl radicals RO˙) and radical ions (radical cation of thymine or guanine). Type II reactions involve the production of singlet oxygen (1O21Δg), which is highly reactive.28,29,49,50 Inactivation of pathogens via photodynamic processes is classified as a type of Photodynamic Therapy (PDT) and poses an attractive and most importantly effective alternative treatment against viruses,51–56 fungi,57–63 parasites64–68 and bacteria.23,27,37,69–71
Over the last 20 years most of the work towards APDT has been focused on the properties of photosensitisers. The biological diversity of microorganisms is a challenge to the efficacy of this therapy. One of the main problems is the composition of pathogen cell walls. Gram-positive bacteria have a thicker peptidoglycan layer (20–80 nm) with polysaccharides, peptidoglycolipids and teichoic acids attached covalently, whereas Gram-negative bacteria have a thinner peptidoglycan layer (5–10 nm) with Braun's lipoprotein, in the outer membrane.49 Therefore, a great effort has been undertaken to optimise the properties of photosensitisers, which would allow the treatment of both a wide range of infections, and specific targeted infections. The short lifetime of singlet oxygen (3 μs in a metabolically-functioning single cell72 or H2O73) is the reason why the immediate proximity of the photosensitiser to the microbial cell is needed for the best results.28
As PDT involves the interaction of light with photosensitisers, the light source is just as important as the photosensitiser. In fact, the development of the field has been largely shaped by the availability of light sources. As photosensitisers have been recently reviewed,74,75 our focus is on the role of light and light sources in APDT. It is timely because advances in optoelectronics, including lasers, LEDs and organic LEDs have transformed lighting and are poised to transform photodynamic therapy. In order to keep the review a manageable size, we focus on the simple, long-established, widely available photosensitiser methylene blue (MB) that is approved by the Federal Drugs Administration for the treatment of methemoglobinemia and is widely used off-label for PDT.76 For the first time we bring together and analyse data for all published APDT studies using MB in the period 2000–2022. We explore whether parameters such as light source, irradiance (incident light intensity per unit area, usually measured in W cm−2) and radiant exposure (incident light energy per unit area, usually measured in J cm−2) influence the effectiveness of APDT. We then discuss the implications for the future of the field.
2. The meaning of light in antimicrobial photodynamic therapy
Light is an essential element for APDT, and the characteristics of the light required, in turn, define the most suitable light sources. In this section we consider the role of the wavelength of light and light-tissue interactions in APDT.
2.1 The optimal treatment wavelength
APDT studies in vitro have shown that this method can be effective for killing a wide range of pathogens, both prokaryotes and eukaryotes.28,77,78 There are more constraints to consider when performing APDT in vivo. The wavelength of the photoactivation light, crucial for ROS production, has to be safe for host (human) cells. Consequently, ultraviolet light (<400 nm) should be avoided in APDT treatment, due to its high potential for DNA mutagenesis leading to oncogenesis and forming toxic products of tryptophan, tyrosine and riboflavin.79–82 UV light exposure (7–25 J cm−2) during in vivo studies resulted in drastic changes in the dermis and epidermis and promoted oxidative stress. In vitro, it led to the apoptosis of fibroblasts, the formation of the tumour suppressor p53 and thymine dimers, the occurrence of sunburn cells and skin pigmentation increase.83,84 However, UV-B sunlight is crucial for humans to produce cholecalciferol (vitamin D3), the production of which is required for calcium and phosphate homeostasis.85 Moreover, PUVA (psoralen and ultraviolet A) and narrowband UVB (NB-UVB) therapy are based on UV light and are widely used to treat psoriasis. Nevertheless, prolonged use of the above treatments has been reported to have carcinogenic effects, including non-melanoma skin cancers (NMSC) squamous cell carcinomas (SCC) and basal cell carcinomas (BCC).86–88
The possible toxicity of blue light has been explored in studies on cell lines. In 2010 Liebmann et al. investigated the influence of solar irradiation at longer wavelengths on human skin cells.89 Their research demonstrated the cytotoxic effect of blue light at high fluences (412, 419, 426 nm: 66–100 J cm−2 and 453 nm: >500 J cm−2) on keratinocytes, endothelial cells and proliferating T lymphocytes (proliferation reduction). The significant proliferation decrease caused by blue light irradiation is the basis of hyperproliferation treatment, for example against psoriasis as an alternative to PUVA therapy. Opländer et al., also studied the effect of blue light on skin. They showed the dependence of cytotoxicity on the applied wavelength and defined the range of blue light (400–460 nm) characterised by antiproliferative properties.90 Masson-Meyers et al., in turn, have suggested that light of a wavelength of 470 nm (at 5 J cm−2 fluence only) does not diminish wound healing (in vitro) and might even stimulate the tissue recovery process.91 A similar effect of a low-dose blue light was observed by Mignon et al. in 2016, and was attributed to enhancement of collagen production, but further research (Mignon et al. 2017) with several light-exposures applied (more than one irradiation) showed a toxic impact of light with wavelength between 450 and 590 nm on cellular metabolic activity.92,93 Blue light has been reported to be harmful to human retinal pigment epithelial (RPE) cells, causing so-called blue light-induced apoptosis (BLIA).94–97 Blue-green light is also considered to be damaging to the retina cells,98,99 however, Arnault et al. showed that green light with wavelength of 550 nm does not exhibit toxic effects on RPE.100 There is no evidence of green light being cytotoxic to skin, and it may be applied in skin lesion treatment.100 However, overall the effect of blue light remains a matter of debate as studies on patients have not shown toxicity of blue light, and according to the best available clinical research evidence, blue filters are not needed to protect eyes.101
Interestingly, in the case of red light (632, 648 nm) and NIR light (850, 940 nm) no negative effects are observed.89 In the last 50 years red light therapies have been extensively investigated and successfully applied in the treatment of various conditions such as wound healing, skin diseases, traumatic brain injuries, Alzheimer's and Parkinson's disease, depression and inflammatory diseases.92,102–106 Wavelengths in the red and NIR are preferred for photodynamic therapy (whether antimicrobial or cancer) for several reasons. Firstly, there are well-known photosensitizers, such as derivatives of chlorins, bacteriochlorins, phthalocyanines, and phenothiazine-based dyes, absorbing this range of light very efficiently.107–109 Secondly, red and NIR light penetrate skin significantly deeper than shorter wavelengths, and finally, so far there is no evidence that they are toxic to cells.
2.2 Light propagation in human tissue
The propagation of light in tissue is a key step in PDT as light must reach the photosensitiser in the target tissue. Light can be reflected from the surface of tissue, and once inside it can be strongly scattered and absorbed. In fact, tissue (including skin) scatters light so strongly that each ray (or photon) can be scattered many times before it is absorbed, making light propagation in tissue a complex problem to study. The regular reflectance of skin illuminated by light at normal incidence is around 4–7%, and the rest of incident light is either scattered or absorbed in the tissue.110 Therefore, light absorption and light scattering are the dominant processes to understand in light-tissue interaction.
The absorption of light in tissue results from different components including water, oxyhaemoglobin and haemoglobin in the epidermis, melanosome, vessel wall, and whole blood.111 It depends on the wavelength, and can be characterized by absorption coefficients μa(λ) as shown in Fig. 1.
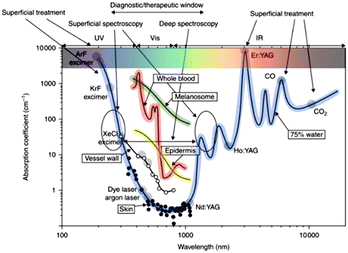 |
| Fig. 1 Primary absorption spectra of biological tissues and wavelengths of lasers commonly used in medicine. Reprinted with permission from Altschuler and Tuchin.111 | |
Light scattering is a photon-matter interaction which alters the direction of propagation of the photon. It occurs at the interface of different tissue components due to the differences in refractive index. Structures much smaller than a wavelength of light lead to Rayleigh scattering, whilst structures comparable to or larger than the wavelength of light lead to Mie scattering.112 The scattering coefficient is also wavelength-dependent and can be represented as μs(λ). In modelling, the reduced scattering coefficient
is often used, and is given by
where the anisotropy parameter g is the average of cosine of the scattering angle. Fig. 2 shows the wavelength-dependent reduced scattering coefficient for different scattering types.113 Different simulations have been used for calculating the light propagation in tissue for sensing or calculation of absorbed dose of light.114–116
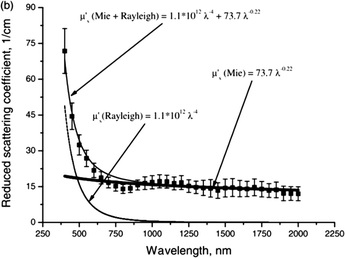 |
| Fig. 2 Reduced scattering coefficient of human skin as a function of wavelength. The symbols show the averaged experimental data and standard deviation represented by vertical lines. The contribution of Mie and Rayleigh scattering, and their combination are also presented. Reprinted with permission from A. Bashkatov.113 | |
Monte Carlo simulations are the most widely used method for simulating light transport in tissue. Photon packets are generated, and their trajectory through the tissue is recorded.117 Once launched, a photon will travel a certain distance and come across different events (absorption, scattering, reflection or transmission). The photon changes direction many times until it is terminated by escaping from the tissue or absorption in the tissue. Reflection or transmission is recorded when the photon escapes from the tissue, and the position of the photon is recorded when it is absorbed by the tissue. The recorded ray history is then analysed after the simulation is completed. As the number of photon trajectories studied increases, the simulation becomes more accurate. In combination with measured values of the optical properties of tissue, Monte Carlo simulations provide a detailed understanding of light propagation in tissue.
The penetration depth of light is a key parameter in evaluating the absorbed dose of light in photodynamic therapy. It is normally defined as the depth at which the incident intensity (irradiance) decreases to 1/e of its initial value. The light penetration depth can be calculated using the absorption and reduced scattering coefficients,113 giving a relationship between wavelength and light penetration depth into the skin as shown in Fig. 3. For photodynamic therapy, a commonly used wavelength range is from 600–900 nm because these wavelengths are safe and can propagate further into tissue than shorter wavelengths.110,113 We can see that the calculated penetration depth at 600, 700, 800 and 900 nm are 1.5, 2.0, 2.3 and 2.5 mm respectively.113 Also, Monte Carlo simulations have shown that beam width can affect the penetration depth. An increase in beam width from 1 to 5 mm can significantly increase the penetration depth, but a further increase in beam width has little effect on it.112
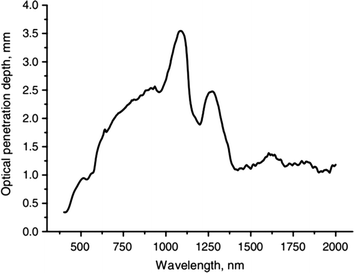 |
| Fig. 3 Optical penetration depth of light into skin at wavelengths from 400 to 2000 nm. Reprinted with permission from A. Bashkatov.113 | |
3. Light sources and their properties
Light is crucial for PDT as it excites the photosensitiser, which then leads to the generation of reactive oxygen species. Hence the light source is very important and needs to fulfil several requirements to achieve effective PDT. First, the wavelength(s) of the light source needs to be absorbed by the photosensitiser, and so needs to be in the region of the absorption spectrum of the photosensitiser. Second, as explained above, the wavelength must be able to propagate far enough in tissue for the desired treatment. Third, the light source needs to deliver sufficient irradiance of light to perform PDT, but should not be so high as to cause unnecessary pain due to heat generation.118 In addition to irradiance and spectrum, there are many other parameters such as light uniformity, equipment size, cost and safety issues which should also be considered. In this section, the advantages and disadvantages of different light sources that are used in antimicrobial photodynamic therapy will be discussed.
3.1 Lasers
LASER is an acronym for Light Amplification by Stimulated Emission of Radiation and is the most widely reported light source for APDT. Lasers generate monochromatic, coherent and collimated light of high irradiance. There are several popular choices of lasers for APDT such as argon ion lasers, metal vapour-pumped dye lasers, Nd: YAG lasers and diode lasers.119,120 They share the benefit of being able to deliver high irradiance from hundreds of mW cm−2 to several W cm−2. These irradiances are higher than for other light sources, but limited to a small area of irradiation. Their narrow emission bandwidth can specifically target the absorption peak of photosensitisers. However, the available wavelengths are limited, hence, the use of dye lasers which use organic dye molecules as the gain medium enabling a wide range of wavelengths to be generated to match the absorption of various photosensitisers. Although lasers are widely installed in clinics, they have some limitations including being expensive, cumbersome, the emitted beam usually being rather small and potential eye safety problems. Sometimes lasers are coupled into fibre bundles, which can be more convenient for delivering light to a patient. The unique feature of laser light, namely its coherence is not needed for PDT and so a range of other light sources is also suitable for PDT.
3.2 Lamps
Lamps have a very long history in light therapy, dating back to the late nineteenth century when the “Finsen lamp” was first used to treat lupus vulgaris.121 Today there are several lamps in use for APDT including tungsten filament, Xenon arc, metal halide, sodium and fluorescent lamps.140 Lamps are normally less expensive and easier to handle than lasers and they deliver light irradiance from several to hundreds of mW cm−2. Unlike lasers, lamps such as sodium lamps and fluorescent lamps can be used for large-area treatment without coupling to fibres.120 Furthermore, the broadband lamps emit a wide range of wavelengths which can cover the whole visible spectrum including the absorption of many commonly used photosensitisers. However, adverse effects such as heat generation by infrared light and tissue damage by ultraviolet light can cause problems.138 For this reason, spectral filters are usually applied to cut off wavelengths not matching the absorption of the photosensitiser. The spectra of filtered lamps for APDT will finally depend on the filters applied, the bandwidth typically ranges from 10 to 100 nm. There are a few limitations of filtered lamps such as large equipment size, expensive spectral filters, and low efficiency due to a combination of the limited efficiency of the lamp itself and to a significant fraction of the light output being blocked by the filters.
3.3 Light-emitting diodes (LEDs)
LEDs are a common light source in which a voltage is applied to a semiconductor, leading to injection of charges and emission of light. The semiconductor can be an inorganic material (such as GaAs or GaN), or an organic semiconductor. However, in practice the term “LED” is used for inorganic semiconductor LEDs, and organic LEDs are called “OLEDs”. LEDs are widely used in lighting and displays, and are also used as light sources for medical treatment. Commonly used materials for LEDs are InGaN, AlGaInP, AlGaAs and GaP. The emission wavelength is determined by the band gap of the semiconductor and can range from the UV to infrared regions of the spectrum, depending on the material. Many LEDs (especially visible and near infra-red) are low-cost in comparison to other light sources, and they can deliver high irradiances up to hundreds of mW cm−2 in an energy-efficient way. Like lamps, LEDs are neither monochromatic nor coherent, but their emission spectrum is much narrower – typically 20–40 nm wide. The available wavelengths of emission of LEDs cover most photosensitisers, and so LEDs can be selected to match the absorption of a photosensitiser, and used without a filter.
LEDs can be mounted as arrays for large-area treatment. Currently, PDT devices based on LEDs are used in hospitals and clinics, but they are still large and cumbersome. However, as individual LEDs are small, arrays of them can be made into smaller, wearable devices.122 An important consideration in all LED based devices is how to achieve uniform illumination.123 This is because LEDs are effectively point sources and so an array of them will naturally be brighter at each LED than in between. For the large hospital-based LED light sources this problem is overcome by placing the array at a distance (∼10 cm) from the patient so that the light from neighbouring LEDs overlaps when it reaches the patient. For a wearable device the light needs to be spread by diffusers which lead to some loss, and also means the light source needs some thickness (∼1 cm) to allow the light to spread out.
3.4 Organic light-emitting diodes (OLEDs)
As well as the inorganic semiconductors discussed above, there are organic semiconductors. These are carbon-based materials which are conjugated, leading to extensive electron delocalisation and semiconducting properties. In contrast to inorganic semiconductors which are rigid, brittle, crystalline materials grown epitaxially, organic semiconductors are often amorphous and flexible. They can readily be deposited from solution or by evaporation and their properties can be tuned by changing their chemical structure. This combination of simple fabrication, flexibility and scope to tune emission across the visible means that OLEDs are now widely used to make vivid and attractive mobile phone displays, and increasingly used for televisions.
OLEDs are lightweight, thin and easy to fabricate by evaporation or solution-based processes. They are intrinsically area light sources (in contrast to inorganic LEDs which are generally point light sources) and so are very suitable for illuminating an area uniformly, as desired in PDT. OLEDs can also be flexible, allowing them to conform to human skin. The available emission wavelengths range from near UV to NIR with a typical emission bandwidth of 60–100 nm. The wavelength can be tuned by changing the chemical structure of the emitter, and fine-tuned using microcavity structures.124–129 The first studies of OLED mediated photodynamic therapy are encouraging and suggest that OLEDs could become the ideal light source for ambulatory PDT.123,125 So far OLEDs give lower emission irradiance (around 5 mW cm−2) than most other light sources but progress is being made towards higher light outputs. The lower irradiance requires a longer exposure time to achieve a given radiant exposure of light, but this is acceptable for an ambulatory device, and has the potential to reduce pain commonly experienced in PDT.
3.5 Daylight
Apart from artificial light sources, PDT using daylight has been promoted in recent years.130–134 Daylight PDT makes use of sunlight which has a broad-spectral range from UV to IR region, so there are many available photosensitisers that can be used. It has some attractive features: it is free of cost, can be accessed without visiting clinics and can illuminate a very large area with high uniformity.135 These features mean daylight PDT has been successfully used to treat actinic keratosis on the scalp.136–139 However, as sunlight is very variable in irradiance, radiant exposure is poorly controlled in daylight PDT. As APDT is likely to be used in situations such as wound healing, and wounds can be on many areas of the body, daylight PDT is likely to be less suitable for APDT than for the treatment of actinic keratosis.
3.6 Other light sources
In addition to the light sources described above, some APDT research simply reports using “non-coherent” or “non-thermal” light sources without giving details of the actual light source used. Even if essential light parameters such as radiant exposure, light irradiance, power output, or wavelength are presented, this may leave questions about the type of light source, mode of operation, appearance of the device, or size of irradiation area. There are also occasional reports of other light sources such as endoscopy systems, photopolymerisers and supra-luminous diodes (SLD).
4. Light sources for antimicrobial photodynamic therapy – approaches to searching and comparison
4.1 Methylene blue as a reference photosensitiser
Singlet oxygen plays a very important role in photochemistry and PDT. One of the most commonly used reference compounds to appraise quantum yield of singlet oxygen generation φ(1O2), which is the essential parameter of the photosensitiser, is methylene blue (MB) known also as methylthioninium chloride. For MB in water, φ(1O2) is 0.52.140 In aqueous solution methylene blue can form dimers, and there is an equilibrium between monomers and dimers that depends on factors such as, for instance, pH and temperature.141 Methylene blue was synthesised for the first time in 1867 by Heinrich Caro with the main purpose of cotton staining. A breakthrough was Guttmann and Ehrlich's discovery of its antimalarial properties, thus methylene blue has become the first clinical synthetic antiseptic.142,143 It has become a widely used diagnostic agent and drug to treat congenital and induced methemoglobinemia, vasoplegic syndrome and ifosfamide-induced encephalopathy.144–146 MB is water soluble but via the uncharged and lipophilic intermediate called leucomethylene blue (LMB/MBH), passes through the cell membrane and promotes non-enzymatic reduction of Fe3+ methaemoglobin ions results in normal haemoglobin.147 Inside the cell LMB is oxidized to MB, where high intracellular concentrations (20–100 μM) lead to the increase of phosphogluconate activity, oxygen consumption and glutathione oxidation.148 Dihydronicotinamide-adenine dinucleotide phosphate (NADPH) is then involved in the reduction of oxidized MB (MB+) to its active reduced form MBH, which causes activation of the glucose 6-phosphate dehydrogenase and therefore pentose phosphate pathway stimulation.149
MB is also involved in other biological processes, such as enhancing cell proliferation in HGPS cell lines and improving cellular respiration of astrocytes.150,151 The regular clinical concentration of methylene blue solution is 10 mg mL−1 (1%) and the therapeutic dose is <2 mg kg−1 body weight.143 MB is widely available and has been very broadly used in PDT, so has been chosen as the focus of this review, and the basis for comparing usefulness of various light sources.
4.2 The protocol of searching
This literature review includes over 330 publications and papers published from January 2000 to September 2022 collected from the platform Web of Science (formerly ISI Web of Knowledge) across all databases. The research was based on a topic search of methylene AND blue AND photodynamic, thus titles, abstracts and keywords were searched and 1892 articles and proceedings were identified for further consideration. From this search, original papers and proceedings about methylene blue mediated APDT (MB-APDT), with a water, phosphate-buffered saline (PBS) or physiological saline (0.9%NaCl w/v) solution of a photosensitiser, were analysed. Both in vitro and in vivo experiments (animal models and human studies) were included. Only articles in the English language were considered. In the end 503 published articles and proceedings were identified from which 330 have sufficient information to use for further analysis. The major aim was to identify the parameters of light used. Additional information collected includes photosensitiser concentration, APDT target, reduction in pathogen cells, and various experimental details such as initial number of cells, the distance between sample and light source, pre-irradiation incubation time (preincubation), and method of cell survival determination.
5. Results
The results of the search on APDT using methylene blue are shown in Table 1. The table summarises the main types of light sources used, together with the key experimental conditions for APDT.
Table 1 The parameters of light sources used in studies of methylene blue mediated APDT published between 2000 and 2022
Light source (according to the number of research) |
Light parameters |
PS concentration [μg mL−1] range modemedian |
No. of papers |
Ref. |
Power output [W] range modemean |
Irradiance [mW cm−2] range modemean |
Radiant exposure [J cm−2] range modemean |
Wavelength [nm] |
Irradiation time [min] range modemedian |
LASER |
0.021–7 |
0.35–2.1 × 106 |
0.24–6048 |
630–690 |
0.17–120 |
0.001–3.2 × 105 |
163 |
33, 57, 71 and 152–311
|
0.040
|
100
|
30
|
|
5
|
100
|
|
0.25
|
26 789
|
128
|
|
4
|
24.47
|
|
LED |
0.1–0.69 |
0.15–1100 |
0.24–305 |
560–780 |
0.33 – 200 |
0.0003–5 × 104 |
108 |
59, 60, 64, 67, 68, 128, 194, 231, 233, 275, 301 and 311–407
|
0.24
|
100
|
10
|
|
10
|
31.98
|
|
0.27
|
104
|
47
|
|
10
|
17.59
|
|
Lamp |
0.165–2.8 |
1.6–7 × 103 |
0.0075–1010 |
350–IR |
2 – 360 |
0.003–5 × 103 |
49 |
22, 70, 174, 181, 185, 193, 205, 217, 281, 316, 320, 401 and 408–444
|
n/d
|
40
|
200
|
|
10
|
3.2
|
|
1222
|
306
|
55
|
|
20
|
6.4
|
|
Other in-/non-coherent light |
0.3–6.88 |
32.5–400 |
0.9–360 |
400–800 |
0.2–120 |
0.032–511.76 |
10 |
46 and 445–453
|
0.70
|
100
|
40
|
|
4
|
6.4
|
|
0.7
|
117
|
45
|
|
6.4
|
6.4
|
|
OLED organic light-emitting diode |
|
1.5–9,44 |
2–129.6 |
570–750 |
47–180 |
1.25; 5 |
3 |
125, 128 and 129
|
|
n/d
|
n/d
|
|
n/d |
|
|
|
6
|
36
|
|
104 |
|
|
Solar radiation |
|
2.9–579.2 |
|
UV–IR |
30; 540 |
0.05–63.97 |
|
454–456
|
|
n/d
|
|
|
|
11.7
|
3 |
|
174
|
|
|
|
|
|
OPO oscillator optical parametric |
|
10; 250 |
30; 90 |
665 |
2; 3.3 |
16 |
2 |
157 and 457
|
Endoscopy system |
|
150 |
|
|
5; 10; 15 |
20; 200; 400 |
1 |
458
|
HHP hand-held photopolymerizer |
|
350–500 |
2 |
400–500 |
1; 3 |
0.032; 3.2 |
1 |
459
|
Non-termal light |
|
|
|
664 |
24 |
1; 500 |
1 |
189
|
SLD supra-luminous diodes |
5.1 |
7.8; 120 |
3; 12; 24; 40; 60 |
625 |
0.5; 2.3; 4.6; 7.8; 11.6 |
90 |
1 |
460
|
Data from each group was converted to the most frequent measurement units: watts (W) for power output, milliwatts per square centimetre (mW cm−2) for illumination irradiance, joules per square centimetre (J cm−2) for radiant exposure, nanometres (nm) for wavelength, and minutes (min) for exposure time. The leading unit for photosensitiser concentration is milligram per millilitre, however, due to a large range of data including very low concentrations, we have used micrograms per millilitre (μg mL−1). For all parameters, the range of values used in the papers identified is presented. Where relevant, the mean is also shown. As there is a wide spread of many parameters, but with some tendency to concentrate around particular values, the mode (i.e. most frequently occurring value) was also calculated. Empty spaces in the table mean lack of literature data. In this review, no attempt was made to calculate any parameters due to the use of only data explicitly provided in the literature.
5.1 The targets of methylene blue-mediated antimicrobial photoinactivation
From the analysis of 330 published articles and proceedings, 84% are in vitro studies, 8.5% in animals and 7% on patients. For animal studies, the murine model is most common and used in approximately half of the studies. The targets of APDT were mainly bacteria (56% of all cases) followed by fungi (29% of cases), virus (8% of all cases), parasites (4.5% of cases), plants (1.5% of all cases) and animals (1% of all cases) (Fig. 4).
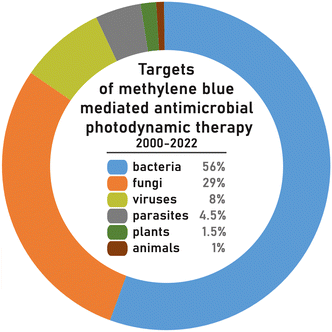 |
| Fig. 4 Targets of methylene blue mediated antimicrobial photodynamic therapy in studies conducted between years 2000–2022 based on the species diversity. | |
The calculations of the percentage distribution of MB-APDT targets were based on the biological diversity (variety of species) of the organisms studied over the years 2000–2022. The most frequently studied bacterium of bacteria targets was Staphylococcus aureus (23%), including antibiotic resistant varieties such as Methicillin-resistant Staphylococcus aureus (MRSA). The next most popular bacterial targets were Escherichia coli (12.5%), Enterococcus faecalis (10.5%), Pseudomonas aeruginosa (9%) and Streptococcus mutans (8%) (Fig. 5a). A possible reason for S. aureus being a more common target is that it is a widespread skin pathogen of major clinical concern that is often antibiotic resistant and therefore an attractive target for APDT.169,239,397,452
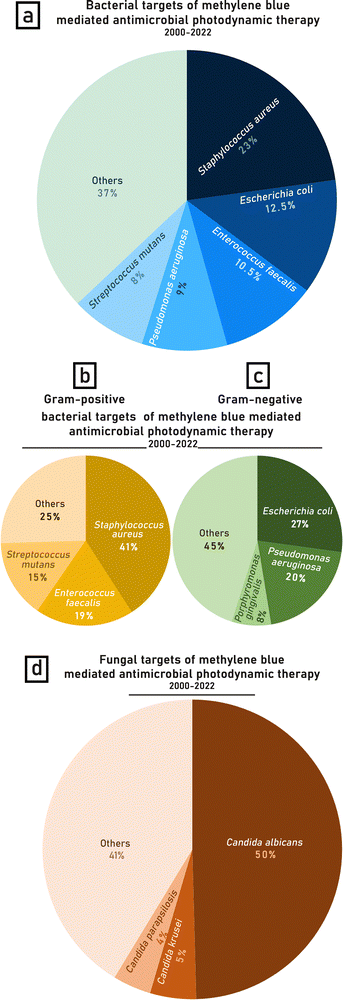 |
| Fig. 5 Targets of MB-mediated antimicrobial photodynamic therapy in studies conducted between years 2000–2022 based on the number of publications. (a) all bacteria, (b) Gram-positive bacteria, (c) Gram-negative bacteria and (d) fungi. | |
Gram-positive bacteria account for 55% of the bacterial species tested, while 45% are Gram-negative. Considering this division of bacterial targets, the % distribution of the most frequently studied species, Staphylococcus aureus, constitutes almost half (41%) of the Gram-positive bacteria studied (Fig. 5b), whilst Escherichia coli is investigated in nearly 1/3 (27%) of the papers regarding APDT against Gram-negative bacteria (Fig. 5c). Amongst fungi, the most commonly investigated fungus genus was Candida spp. (C. albicans, C. krusei, C. parapsilosis). The leading species were Candida albicans (50%), Candida krusei (5%) and Candida parapsilosis (4%) (Fig. 5b). The tested microorganisms were mostly obtained from American Type Culture Collection but in some cases pathogens, especially S. aureus, were isolants or clinical strains (Table 1).60,195,210,211,251,363,421,461 Several studies focused on APDT of oral microflora, in particular, bacteria found in dentin, usually isolated from patients with deep caries lesions153,216,257,263,282,284,362,385
Some studies also investigated MB-APDT targeted towards viruses, including vesicular stomatitis virus (VSV), bovine viral diarrhea virus (BVDV), dengue virus, West Nile virus (WNV), hepatitis C virus (HCV), herpes simplex virus type 1 (HSV-1) and SARS-CoV2.215,297,299,300,311,314,321,331,403,408,409,411
Anti-parasitic photodynamic therapy investigations were dominated by Leishmania spp. (L. braziliensis, L. amazonensis, L. major, L. mazonensis)67,128,196,198,238,324,340,356,368 and only two publications studied MB mediated photoinactivation against an alternative parasite species, which were Trichomonas vaginalis and Trypanosoma cruzi.64,286
5.2 The concentration of methylene blue in APDT
The concentration of MB used in APDT of a range of pathogens is shown in Fig. 6. The photosensitiser concentration was reported in three different ways as molar concentration (mostly μM), mass concentration (mostly mg mL−1) or a percentage (%). The reported molar concentrations ranged from 10 nM (3.2 × 10−6 g L−1) to 1 M (319.84 g L−1), the mass concentrations ranged between 0.001 μg mL−1 (3.12 nM) and 50 mg mL−1 (0.16 M) and the percentage concentrations ranged from 0.0001% to 2%. The highest concentration (319.84 g L−1 = 1 M) was tested on Herpes simplex viruses cultured in infected monolayers of cell lines, and the lowest on Mycoplasma salivarium in vitro.332,353 We note that the highest concentrations reported seem unreasonable as the solubility of methylene blue in water is reported to be up to 0.13 M (43 g L−1) thus concentrations of MB greater than its water solubility were rejected from further analysis.462 For antifungal and antibacterial PDT the methylene blue concentration range is very broad but accumulation of values is observed between 0.31–0.031 mM (0.1–0.01 g L−1) (Fig. 6). Based on all the presented concentration values, the mode for methylene blue concentration is 0.1 mM (0.032 g L−1).
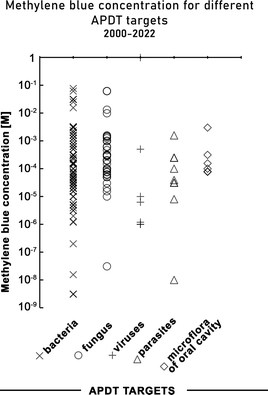 |
| Fig. 6 Methylene blue concentrations (on a log scale) used in MB-mediated antimicrobial photodynamic therapy in studies conducted between 2000–2022. | |
The APDT effect is also highly dependent on the concentration of photosensitiser thus the analysis of log reduction as a function of MB concentration was performed (Fig. 7). In general, no correlation between reduction and MB concentration is observed either for fungi or bacteria. A reduction higher than 6 logs can be obtained in a broad range of photosensitizer concentration (32–320 μg mL−1). For some bacterial targets significant reduction (8 log
10) is achievable with relatively low concentration <60 μg mL−1 (Fig. 7). Some studies show evidence of improved reduction by increasing photosensitizer concentrations, but this effect depends on the pathogen species and is not linear.290
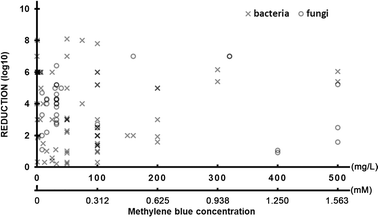 |
| Fig. 7 The reduction of targeted pathogen number via antimicrobial photodynamic therapy as a function of applied methylene blue concentration in studies conducted between 2000–2022. | |
Since methylene blue toxicity in the dark is observed in case of many pathogens, preincubation time, defined as a time of pathogen incubation with photosensitizer without light, appears to play important role in APDT effect. The longer preincubation time the higher log
10 reduction in case of bacteria is observed (Fig. 8a).
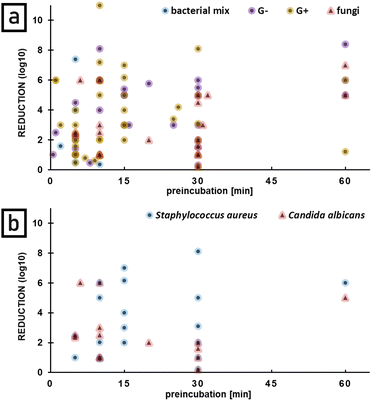 |
| Fig. 8 Log reduction of targeted pathogen number as a function of preincubation time for bacteria (bacterial mix – mixture of bacteria or unidentified bacterial microflora; G(−) – Gram-negative bacteria; G(+) – Gram-positive bacteria) and fungi (a) and the two major pathogens Staphylococcus aureus and Candida albicans (b) investigated in studies concerning antimicrobial photodynamic therapy using methylene blue conducted between 2000 and 2022 based on species diversity. | |
There is a correlation observed especially in case of Staphylococcus aureus which analysis is based on the biggest number of data collected for one bacterial species. For Candida albicans no correlation was observed and longer preincubation (up to 30 min) reduces the final reduction. The same trend was observed in case of other fungal species. No data are available for preincubation between 35 and 60 minutes (Fig. 8b).
5.3 The light sources used for methylene blue mediated APDT
The types of light sources used are shown in Fig. 9. The most common light sources reported for MB-mediated APDT were lasers (48.5%), LEDs (32%), and lamps (13%). The other light sources category (6.5% in total) includes so-called non-coherent light sources (name taken from publication methodologies), solar radiation,454–456 optical parametric oscillator (OPO),457 hand-held photopolymerizer (HHP),459 endoscopy system,458 superluminescent diodes (SLED or SLD)460 and organic light-emitting diodes OLEDs125,128,129 (Fig. 9).
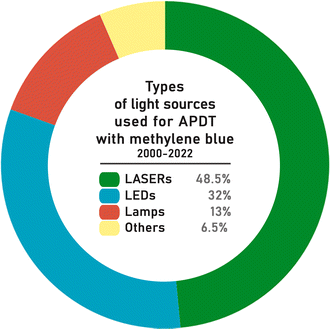 |
| Fig. 9 Diversity of applied light sources for MB-mediated antimicrobial photodynamic therapy in studies conducted between years 2000–2022 based on the number of publications. | |
The key irradiation parameters for APDT are shown in Fig. 10. The issue of power output is quite problematic. Many publications, especially in the case of lamps, do not specify if the reported power is the optical output power or the electrical input power. For preparing Fig. 10a only values of power output were used, limiting us to only three reports of lamps. Consequently, the highest power output was observed for a laser (7 W).
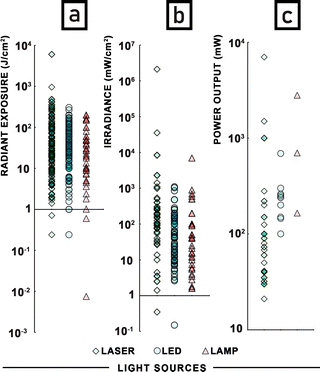 |
| Fig. 10 The irradiation parameters in MB-mediated antimicrobial photodynamic therapy in studies conducted between years 2000–2022. The graphs show the power output (a), irradiance (b) and radiant exposure (c) for lasers, LEDs and lamps, which are the three most common light sources in MB-mediated APDT. All values are in log scale due to their broad ranges. The overlapping of the markers on the graphs shows the most common values. | |
Due to heat generation, lamps require a cooling element (water tank or ice), commonly assembled between the light source and irradiated sample.408,409,413,442–444 Heating is much less of a problem for LEDs because of their much higher efficiency. Lasers, as the most common light source are characterized by the broadest range of power output values.
The most common power output (mode of the distribution) was 40 mW for lasers and 240 mW for LEDs (Fig. 10c). However, when arithmetic means are considered, the average power output of lasers was 257 mW and 274 mW for LEDs. In case of lamps, there are only three reports that explicitly mention power output. The irradiance was the second most often reported parameter (180 publications) ranging from 0.15 mW cm−2 (LED)275 to 2123 W cm−2 (laser).256 For lasers and LEDs the most frequent irradiance value was 100 mW cm−2, for lamps 40 mW cm−2 (Fig. 10b). Unfortunately, in the case of many studies this parameter was disregarded, nevertheless, strong tendency is observed here. The radiant exposure, referring to the irradiated surface area as well as irradiance and directly depending on irradiation time, was the quantity most commonly reported (256 publications). The maximum radiant exposure found was directed against Candida albicans murine models in vivo and was 6048 J cm−2 (laser).264 The most common radiant exposure (mode of the distribution) was 30 J cm−2 for lasers, 10 J cm−2 for LEDs, and 200 J cm−2 for lamps for both in vivo and in vitro studies (Fig. 10a). Because of methylene blue, almost all research used red light for the photoactivation process. Studies using a laser with a wavelength exceeding the absorbance spectrum of the dye were excluded from further analysis. Lasers as monochromatic light were described by only one wavelength. For LEDs the maximum wavelength of emission was usually presented. In the case of lamps, investigators usually marked the presence of filters that allowed them to obtain light with a wavelength usually longer than 630 nm or the range of light wavelengths was presented (Table 1).
The distance between the light source and illuminated area during the photodynamic inactivation process should be reported to understand the geometry of the study. When the light source releases large amounts of energy in the form of heat, the temperature of the surface under direct light may drastically increase, leading to a thermal (not photodynamic) lethal effect. The largest distance between the sample and light source of 3 meters was used in APDT of a virus, and photoinactivation was still observed.314 In the dental field optical fibres were usually introduced into the tooth, as close to the pathogen as possible, reducing the distance between the light source and the irradiated object to a minimum.206,207,241 The range of distances between the irradiated area and the light source was in the range of 0.1–300 cm, with the most common value being 1 cm. It should be noted that only 20.9% of research reported the distance of the light source from the sample.
5.4 The effectiveness of different light sources in microbial load reduction
Many different light sources were applied in antimicrobial photodynamic therapy with a broad range of power output, emission properties, applicability, and availability. Therefore, a question arises: is there any difference in APDT effectiveness dependent on the applied light source and its properties. The reduction of microbial load shown as a logarithm is the commonest approach to present the antimicrobial effect of photoinactivation. For this reason, it was chosen as the basis for the comparison of the effectiveness of different light sources in APDT. There is no doubt that bacteria are the most frequent study target of photoinactivation thus the greatest amount of data is collected in the area of antibacterial action of APDT. Fig. 11 shows that although there is a broad range of reductions achieved, all light sources can achieve a large reduction, and that the reduction does not appear to depend strongly on the light source. The figure does not include parasites because (apart from one exception) the publications on this topic did not include all the information needed (reduction in microbial load, irradiance and radiant exposure).
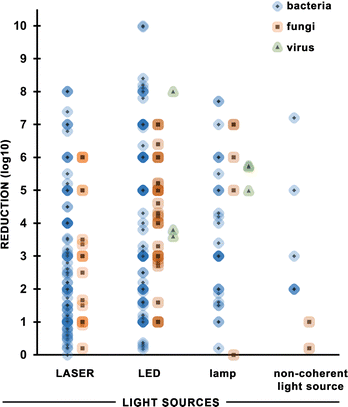 |
| Fig. 11 The reduction of targeted pathogen number via antimicrobial photodynamic therapy with the use of different light sources. | |
For laser APDT, there is a high density of points around 1 log
10 reduction (90% mortality of bacteria), indicating this is the most common value. The average reduction is higher for LEDs and lamps.
Fig. 12 plots the effectiveness of APDT as a function of radiant exposure (J cm−2) of light delivered. Fig. 12a shows results for bacteria, fungi and viruses, and it can be seen that APDT can be effective in greatly reducing the numbers of all these microbes. Fig. 12b shows the same data, but divided into type of light source. It can be seen that LEDs, lasers and lamps can all be very effective sources for APDT, with the highest reductions seen for LEDs. An important point is that large reductions are seen for low radiant exposure of light and that the highest radiant exposures do not give the largest reductions. There is not a clear optimal radiant exposure, because there is not enough data at low radiant exposure to identify the minimum that is still effective. Further studies at low radiant exposure would therefore be useful. Fig. 13 is similar to Fig. 12, but shows the effectiveness of APDT as a function of the irradiance of light used. Fig. 13a shows that effective reduction of pathogens can be achieved using a wide range of irradiance, including low irradiance – there is no clear correlation with light irradiance. Fig. 13b shows that lasers, lamps and LEDs can all be effective, and again there is no clear correlation with irradiance.
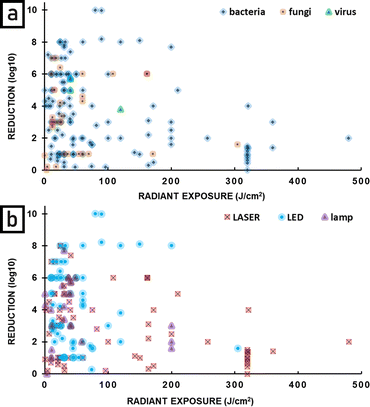 |
| Fig. 12 The reduction of targeted pathogens number via antimicrobial photodynamic therapy as a function of applied radiant exposure and pathogen (a) and light source (b). The overlapping of the markers on the graphs shows the most common values. | |
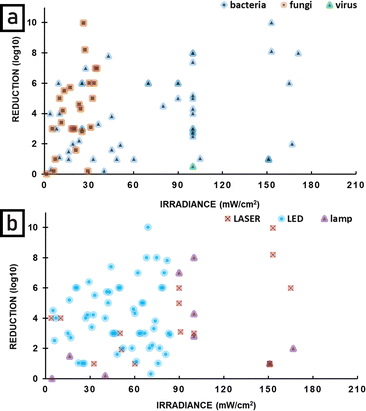 |
| Fig. 13 The reduction of targeted pathogen number via antimicrobial photodynamic therapy as a function of applied irradiance and pathogen (a) and light source (b). The overlapping of the markers on the graphs shows the most common values. | |
Since in the literature statements concerning the difference in sensitivity of Gram-positive and Gram-negative bacteria are common, an analysis depending on this classification was performed. For both, a broad range of radiant exposure (Fig. 14) and irradiance (Fig. 15) was applied. Up to 90 J cm−2 no correlation between radiant exposure and bacteria reduction was observed. Above 90 J cm−2 Gram-positive bacteria show better reduction, however, the gap in research between 90 and 160 J cm−2 for Gram-negative bacteria should be here taken into account (Fig. 14a).
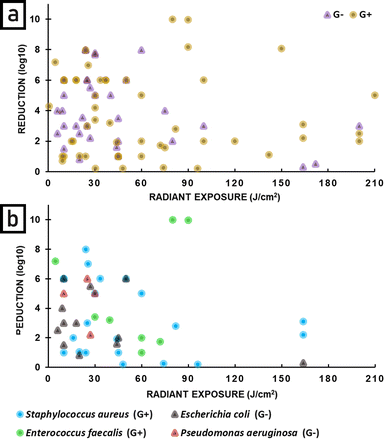 |
| Fig. 14 The reduction of targeted pathogen number via antimicrobial photodynamic therapy as a function of applied radiant exposure divided into Gram-positive and Gram-negative bacteria (a), and the four most popular bacterial species in APDT (b). | |
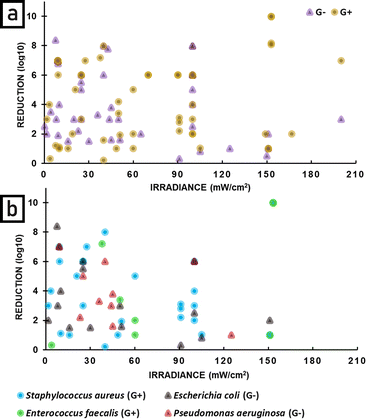 |
| Fig. 15 The reduction of targeted pathogen number via antimicrobial photodynamic therapy as a function of applied irradiance and pathogen (a), and four the most popular bacterial species in APDT (b). The overlapping of the markers on the graphs shows the most common values. | |
Additional analysis of reduction as a function of radiant exposure (Fig. 14b) and irradiance (Fig. 15b) was performed for the four most common bacterial species. A relatively wide range of reduction is observed for Staphylococcus aureus and Escherichia coli at radiant exposures up to 50 J cm−2. Higher radiant exposures do not appear to significantly improve the reduction of Gram-positive bacteria. A similar pattern is observed for irradiance, where values above 60 mW cm−2 do not increase the bactericidal effect of APDT.
5.5 Implications for study design and reporting
Upon reviewing the literature, we have noticed key parameters are often missing in APDT studies, making comparison between studies difficult. APDT studies should be appropriately designed and methods fully described to enable comparison and ensure that other researchers can independently repeat the procedure.
Light parameters.
As light plays an essential role in APDT, causing photosensitiser photoactivation, the reporting of light source parameters is of vital importance. Due to the direct influence of the light source on APDT results, its description should be full (appropriate calculations and units) and clear (exact values if possible) to avoid mistakes that could dramatically change experiment outcomes. For this reason we suggest reporting the following six parameters in the methodology:
1. Radiant exposure (J cm−2) – the main parameter, reported in nearly 50% of analysed publications,
2. Irradiance (mostly mW cm−2) – the second most reported parameter, allows the calculation of radiant exposure if the time of irradiation is known,
3. Irradiation time – reported in most publications, but needs the other light parameters to be useful. Whilst two out of three of radiant exposure, irradiance and irradiation time enable the third parameter to be calculated, it is helpful to the reader to provide all three parameters.
4. Fractionation – in some cases the radiant exposure of light is delivered by turning the light source on and off. If this is done the details should be provided.
5. Spectrum of the light source or wavelength if a laser (nm) – its overlap with the photosensitiser is crucial for determining the amount of light absorbed. In the case of lasers, the exact wavelength is shown, studies using other light sources tend to present ranges,
6. area illuminated and distance between light source and sample – these are helpful for understanding the experimental configuration including likelihood of heat transfer from the source to the sample.
Biological parameters.
The APDT effect also strongly relies on the biological (target) and chemical (photosensitiser) parameters of the study. Not only the targeted pathogen but also the amount of pathogen used (if in vitro) affect the final reduction, understood as a decrease of the target amount in comparison to the untreated group/sample. Another issue is methylene blue toxicity even in dark conditions. The biological and chemical parameters listed below should be presented in the methodology due to their direct influence on outcomes:
1. Initial amount of photoinactivation target (cells, CFU or PFU) – strongly influences the result, some studies present it as the amount of target before photosensitiser addition which leads to its dilution,
2. Photosensitiser concentration at the start of illumination – studies should present the amount of photosensitiser during irradiation, some publications refer only to the initial concentration,
3. Solvent used for photosensitiser solution – some solvents (including methanol and ethanol) may be toxic for the pathogen itself, their use requires additional control,
4. Preincubation time – its presence depends on an additional step where samples are stored in the dark with photosensitiser before irradiation, sometimes with additional shaking,
5. Additional washing before irradiation – included usually when preincubation is implicated.
6. Appropriate control experiments – In the case of APDT, three control experiments are needed. The most fundamental is a control sample without light and photosensitisers stored in the same environmental conditions as the sample exposed to APDT. In addition, due to methylene blue having some toxicity in the dark, a control experiment without light but with photosensitiser is needed. This is often referred to as a “dark control.” The dark control should not be the main basis for sample comparison or calculations due to the actual or potential toxicity of the photosensitiser even without light. The third control that should be performed is a “light control” in which the sample is illuminated, but no photosensitiser is present. The high radiant exposure of energy delivered to the target in a short period of time is a contentious issue. It may lead to pathogen death, which is the primary goal of APDT and an indisputable benefit. However, it may also lead to microbe destruction without photodynamic process participation and promote photobleaching which results in the photosensitisers permanent inability to produce ROS.
Details of temperature and temperature control – The temperature of the experiment needs to be reported and the effect of heating by the light source also needs to be considered and mitigated. This may involve having appropriate distance between the light source and the sample and/or active temperature control of the sample.
6. Conclusions
There is growing interest in APDT due to the steep rise in antibiotic resistant pathogens. Light is a crucial component of PDT because it excites the photosensitiser, leading to the generation of reactive oxygen species. This review has shown that PDT can be effective against bacteria, fungi and viruses, including the emerging problem of SARS-Cov2.299,463,464 A large range of light sources, irradiance and radiant exposure values have been used for APDT. By collecting all these results together, we have seen that effective APDT can be achieved using many different light sources. We have also seen that low radiant exposure of light can be at least as effective as high radiant exposure, and also that low irradiance of light can lead to effective PDT. We found some problems comparing published work, and have therefore suggested key optical and biological parameters that should always be reported. At present the vast majority of studies are in vitro, making it a priority for the field to move towards clinical studies in the future.
The fact that a wide range of light sources, irradiance and radiant exposure values can be effective is important for the future development of APDT and opens a path to light sources that are more cost-effective and convenient for clinical studies. It means that advances in the rapidly developing field of optoelectronics can feed into the development of APDT. We expect a move away from lasers to more robust, efficient, simpler and less expensive light sources, such as those based on LEDs, thereby removing a crucial barrier to the adoption of APDT. This trend is already apparent in the recent literature where in the period 2020–2022, there are more reports of LEDs for APDT than of lasers. Furthermore, finding that high powers are not required for effective APDT creates the possibility of using compact and even wearable light sources for APDT. In this context organic light-emitting diodes (OLEDs) are particularly attractive as they provide flexible light sources that emit over an area. The ready availability of practical light sources can be expected to facilitate the adoption of APDT, providing a powerful tool in the battle against microbial resistance. Reaching this goal will require not only advances in light sources, but also a move from in vitro studies to clinical trials.
Conflicts of interest
IDWS is a founder and shareholder of Lustre Skin Ltd which sells products for skincare, including a wearable light source for the treatment of acne.
Acknowledgements
This work was financially supported by the European Union's Horizon 2020 research and innovation programme under the Marie Sklodowska-Curie grant (agreement no. 764837) and NCN Opus grant no. 2019/35/B/ST4/03280. We are also grateful to the Engineering and Physical Sciences Research Council of the UK for financial support from grants EP/R511778/1 and EP/L015110/1.
Notes and references
-
J. D. D. Aga, S. Gandra, B. Kasprzyk-Hordern, J. Larsson, J. McLain, A. Singer, J. Snape, H. Slijkhuis, A. Sweetman and N. Voulvoulis, Initiatives for addressing antimicrobial resistance in the environment: current situation and challenges, Wellcome Trust, London, England, 2018 Search PubMed.
-
OECD and ECDC, Antimicrobial Resistance Tackling the Burden in the European Union, 2019 Search PubMed.
-
IACG, No Time to Wait: Securing the future from drug-resistant infections, 2019 Search PubMed.
- S. Hernando-Amado, T. M. Coque, F. Baquero and J. L. Martínez, Nat. Microbiol., 2019, 4, 1432–1442 CrossRef CAS PubMed.
- E. Tacconelli and M. D. Pezzani, Lancet Infectious Diseases, 2019, 19, 4–6 CrossRef PubMed.
- P. Padiyara, H. Inoue and M. Sprenger, Infect. Dis.: Res. Treat., 2018, 11, 1–4 Search PubMed.
- C. T. Kåhrström, Nat. Rev. Microbiol., 2013, 11, 146 Search PubMed.
- J. H. Kwon and W. G. Powderly, Science, 2021, 373, 471 CrossRef CAS PubMed.
- C. M. McCann, B. Christgen, J. A. Roberts, J.-Q. Su, K. E. Arnold, N. D. Gray, Y.-G. Zhu and D. W. Graham, Environ. Int., 2019, 125, 497–504 CrossRef CAS PubMed.
- M. Baym, T. D. Lieberman, E. D. Kelsic, R. Chait, R. Gross, I. Yelin and R. Kishony, Science, 2016, 353, 1147–1151 CrossRef CAS PubMed.
- L. Liu, S. Oza, D. Hogan, Y. Chu, J. Perin, J. Zhu, J. E. Lawn, S. Cousens, C. Mathers and R. E. Black, The Lancet, 2016, 388, 3027–3035 CrossRef PubMed.
- J. P. Burnham, M. A. Olsen and M. H. Kollef, Infect. Control Hosp. Epidemiol., 2019, 40, 112–113 CrossRef PubMed.
- U. Hofer, Nat. Rev. Microbiol., 2019, 17, 3 CrossRef CAS PubMed.
- S. Gandra, K. K. Tseng, A. Arora, B. Bhowmik, M. L. Robinson, B. Panigrahi, R. Laxminarayan and E. Y. Klein, Clin. Infect. Dis., 2019, 69, 563–570 CrossRef PubMed.
- A. Fox-Lewis, J. Takata, T. Miliya, Y. Lubell, S. Soeng, P. Sar, K. Rith, G. McKellar, V. Wuthiekanun, E. McGonagle, N. Stoesser, C. E. Moore, C. M. Parry, C. Turner, N. P. J. Day, B. S. Cooper and P. Turner, Emerging Infect. Dis., 2018, 24, 841–851 CrossRef CAS PubMed.
- Y. Carmeli, G. Eliopoulos, E. Mozaffari and M. Samore, Arch. Intern. Med., 2002, 162, 2223–2228 CrossRef PubMed.
- S. E. Cosgrove, Clin. Infect. Dis., 2006, 42, S82–S89 CrossRef PubMed.
- M. R. Hamblin and T. Hasan, Photochem. Photobiol. Sci., 2004, 3, 436–450 CrossRef CAS PubMed.
- D. E. J. G. J. Dolmans, D. Fukumura and R. K. Jain, Nat. Rev. Cancer, 2003, 3, 380–387 CrossRef CAS PubMed.
- A. F. D. Santos, D. R. Q. D. Almeida, L. F. Terra, M. S. Baptista and L. Labriola, J. Cancer Metastasis Treat., 2019, 5, 1–20 Search PubMed.
- M. M. Kim and A. Darafsheh, Photochem. Photobiol., 2020, 96, 280–294 CrossRef CAS PubMed.
- M. Grinholc, B. Szramka, J. Kurlenda, A. Graczyk and K. P. Bielawski, J. Photochem. Photobiol., B, 2008, 90, 57–63 CrossRef CAS PubMed.
- T. Maisch, S. Hackbarth, J. Regensburger, A. Felgenträger, W. Bäumler, M. Landthaler and B. Röder, Z. Hautkrankh., 2011, 9, 360–366 Search PubMed.
- A. Wozniak and M. Grinholc, Front. Microbiol., 2018, 9, 930 CrossRef PubMed.
- L. Huang, G. Szewczyk, T. Sarna and M. R. Hamblin, ACS Infect. Dis., 2017, 3, 320–328 CrossRef CAS PubMed.
- S. Furgeri Godinho Vilela, J. Campos Junqueira, J. Oliveira Barbosa, M. Majewski, E. Munin, A. Olavo and C. Jorge, Arch. Oral Biol., 2011, 57, 704–710 CrossRef PubMed.
- K. O'Riordan, D. S. Sharlin, J. Gross, S. Chang, D. Errabelli, O. E. Akilov, S. Kosaka, G. J. Nau and T. Hasan, Antimicrob. Agents Chemother., 2006, 50, 1828–1834 CrossRef PubMed.
- T. N. Demidova and M. R. Hamblin, Antimicrob. Agents Chemother., 2005, 49, 2329–2335 CrossRef CAS PubMed.
- M. Grinholc, A. Rodziewicz, K. Forys, A. Rapacka-Zdonczyk, A. Kawiak, A. Domachowska, G. Golunski, C. Wolz, L. Mesak, K. Becker and K. P. Bielawski, Appl. Microbiol. Biotechnol., 2015, 99, 9161–9176 CrossRef CAS PubMed.
- J. Y. Nagata, N. Hioka, E. Kimura, V. R. Batistela, R. S. S. Terada, A. X. Graciano, M. L. Baesso and M. F. Hayacibara, Photodiagn. Photodyn. Therapy, 2012, 9, 122–131 CrossRef CAS PubMed.
- L. Marciel, L. Teles, B. Moreira, M. Pacheco, L. M. O. Lourenço, M. G. P. M. S. Neves, J. P. C. Tomé, M. A. F. Faustino and A. Almeida, Future Med. Chem., 2017, 9, 365–379 CrossRef CAS PubMed.
- G. B. Kharkwal, S. K. Sharma, Y.-Y. Huang, T. Dai and M. R. Hamblin, Lasers Surg. Med., 2011, 43, 755–767 CrossRef PubMed.
- T.-C. Huang, C.-J. Chen, S.-J. Ding and C.-C. Chen, Photodiagn. Photodyn. Therapy, 2019, 25, 7–16 CrossRef CAS PubMed.
- M. N. Alasqah, Photodiagn. Photodyn. Therapy, 2019, 25, 349–353 CrossRef CAS PubMed.
- N. Masiera, A. Bojarska, I. Gawryszewska, E. Sadowy, W. Hryniewicz and J. Waluk, J. Photochem. Photobiol., B, 2017, 174, 84–89 CrossRef CAS PubMed.
- J. C. Junqueira, M. A. Ribeiro, R. D. Rossoni, J. O. Barbosa, S. M. R. Querido and A. O. C. Jorge, Photomed. Laser Surg., 2010, 28, S67–S72 CrossRef CAS PubMed.
- L. Huang, Y. Xuan, Y. Koide, T. Zhiyentayev, M. Tanaka and M. R. Hamblin, Lasers Surg. Med., 2012, 44, 490–499 CrossRef PubMed.
- M. Wilson, Photochem. Photobiol. Sci., 2004, 3, 412–418 CrossRef CAS PubMed.
- M. Wilson and J. Pratten, Lasers Surg. Med., 1995, 16, 272–276 CrossRef CAS PubMed.
- Y. P. Krespi, M. Slatkine, T. Dagan and M. Marchenko, Otolaryngol.--Head Neck Surg., 2004, 131, P109–P109 CrossRef PubMed.
- L. Bergmans, P. Moisiadis, B. Huybrechts, B. Van Meerbeek, M. Quirynen and P. Lambrechts, Int. Endodontic J., 2008, 41, 227–239 CrossRef CAS PubMed.
- S. Schlafer, M. Vaeth, P. Hørsted-Bindslev and E. V. G. Frandsen, Oral Surg., Oral Med., Oral Pathol., Oral Radiol. Endodontol., 2010, 109, 634–641 CrossRef PubMed.
- J. A. Williams, G. J. Pearson and M. John Colles, J. Dent., 2006, 34, 363–371 CrossRef CAS PubMed.
- M. Wainwright, J. Antimicrob. Chemother., 1998, 42, 13–28 CrossRef CAS PubMed.
- M. Wainwright and A. McLean, Dyes Pigm., 2017, 136, 590–600 CrossRef CAS.
- F. Cieplik, A. Pummer, C. Leibl, J. Regensburger, G. Schmalz, W. Buchalla, K. A. Hiller and T. Maisch, Front. Microbiol., 2016, 7, 929 Search PubMed.
- A. Späth, C. Leibl, F. Cieplik, K. Lehner, J. Regensburger, K. A. Hiller, W. Bäumler, G. Schmalz and T. Maisch, J. Med. Chem., 2014, 57, 5157–5168 CrossRef PubMed.
- T. Maisch, A. Eichner, A. Späth, A. Gollmer, B. König, J. Regensburger and W. Bäumler, PLoS One, 2014, 9, e111792 CrossRef PubMed.
- M. S. Baptista, J. Cadet, P. Di Mascio, A. A. Ghogare, A. Greer, M. R. Hamblin, C. Lorente, S. C. Nunez, M. S. Ribeiro, A. H. Thomas, M. Vignoni and T. M. Yoshimura, Photochem. Photobiol., 2017, 93, 912–919 CrossRef CAS PubMed.
- A. P. Castano, T. N. Demidova and M. R. Hamblin, Photodiagn. Photodyn. Therapy, 2004, 1, 279–293 CrossRef CAS PubMed.
- L. Xu, X. Zhang, W. Cheng, Y. Wang, K. Yi, Z. Wang, Y. Zhang, L. Shao and T. Zhao, Retrovirology, 2019, 16, 5 CrossRef PubMed.
- J. Lenard, A. Rabson and R. Vanderoef, Proc. Natl. Acad. Sci. U. S. A., 1993, 90, 158–162 CrossRef CAS PubMed.
- H. Majiya, O. O. Adeyemi, M. Herod, N. J. Stonehouse and P. Millner, J. Photochem. Photobiol., B, 2018, 189, 87–94 CrossRef CAS PubMed.
- M. A. Namvar, M. Vahedi, H. R. Abdolsamadi, A. Mirzaei, Y. Mohammadi and F. Azizi Jalilian, Photodiagn. Photodyn. Therapy, 2019, 25, 87–91 CrossRef CAS PubMed.
- L. Costa, M. A. F. Faustino, M. G. P. M. S. Neves, Â. Cunha and A. Almeida, Viruses, 2012, 4, 1034–1074 CrossRef PubMed.
- D. R. Alvarado, D. S. Argyropoulos, F. Scholle, B. S. T. Peddinti and R. A. Ghiladi, Green Chem., 2019, 21, 3424–3435 RSC.
- L. de Carvalho Leonel, M. L. Carvalho, B. M. da Silva, S. Zamuner, C. Alberto-Silva and M. Silva Costa, Photodiagn. Photodyn. Therapy, 2019, 26, 316–323 CrossRef PubMed.
- K. Sueoka, T. Chikama, Y. D. Pertiwi, J. A. Ko, Y. Kiuchi, T. Sakaguchi and A. Obana, Lasers Med. Sci., 2019, 34, 743–747 CrossRef PubMed.
- Q. Lu, Y. Sun, D. Tian, S. Xiang and L. Gao, Mycopathologia, 2017, 182, 1037–1043 CrossRef CAS PubMed.
- Z. Liu, J. Tang, Y. Sun and L. Gao, Mycopathologia, 2019, 184, 315–319 CrossRef PubMed.
- R. F. Donnelly, P. A. McCarron, M. M. Tunney and A. David Woolfson, J. Photochem. Photobiol., B, 2007, 86, 59–69 CrossRef CAS PubMed.
- L. D. M. Baltazar, B. M. Soares, H. C. S. Carneiro, T. V. Avila, L. F. Gouveia, D. G. Souza, M. V. L. Ferreira, M. Pinotti, D. D. A. Santos and P. S. Cisalpino, J. Antimicrob. Chemother., 2013, 68, 354–361 CrossRef CAS PubMed.
- D. A. N. Mario, L. B. Denardi, D. I. B. Pereira, J. M. Santurio and S. H. Alves, Med. Mycol., 2014, 52, 770–773 CrossRef CAS PubMed.
- T. H. Silva Fonseca, M. Alacoque, F. M. Silva Oliveira, B. M. Soares, H. V. Leite, M. V. Caliari, M. A. Gomes and H. Busatti, Photodiagn. Photodyn. Therapy, 2018, 22, 91–95 CrossRef CAS PubMed.
- H. J. Roh, A. Kim, G. S. Kang, B. S. Kim and D. H. Kim, Aquaculture, 2018, 493, 176–185 CrossRef.
- J. Fagundes, K. K. Sakane, T. Bhattacharjee, J. G. Pinto, I. Ferreira, L. J. Raniero and J. Ferreira-Strixino, Spectrochim. Acta, Part A, 2019, 207, 229–235 CrossRef CAS PubMed.
- D. P. Aureliano, J. A. L. Lindoso, S. R. de Castro Soares, C. F. H. Takakura, T. M. Pereira and M. S. Ribeiro, Photodiagn. Photodyn. Therapy, 2018, 23, 1–8 CrossRef CAS PubMed.
- T. H. S. Fonseca, J. M. S. Gomes, M. Alacoque, M. A. Vannier-Santos, M. A. Gomes and H. G. N. O. Busatti, Acta Trop., 2019, 190, 112–118 CrossRef CAS PubMed.
- M. Tim, J. Photochem. Photobiol., B, 2015, 150, 2–10 CrossRef CAS PubMed.
- M. Grinholc, A. Kawiak, J. Kurlenda, A. Graczyk and K. P. Bielawski, J. Photochem. Photobiol., B, 2008, 90, 57–63 CrossRef CAS PubMed.
- P. S. Zolfaghari, S. Packer, M. Singer, S. P. Nair, J. Bennett, C. Street and M. Wilson, BMC Microbiol., 2009, 9, 27 CrossRef PubMed.
- S. Hatz, J. D. C. Lambert and P. R. Ogilby, Photochem. Photobiol. Sci., 2007, 6, 1106–1116 CrossRef CAS PubMed.
- M. Bregnhøj, M. Westberg, F. Jensen and P. R. Ogilby, Phys. Chem. Chem. Phys., 2016, 18, 22946–22961 RSC.
- H. Abrahamse and M. R. Hamblin, Biochem. J., 2016, 473, 347–364 CrossRef CAS PubMed.
- M. Wainwright and K. B. Crossley, J. Chemother., 2013, 14, 431–443 CrossRef PubMed.
- Food and Drug Administration, HHS., Federal Register Volume 83, Issue 231 (November 30, 2018), Office of the Federal Register, National Archives and Records Administration, 2018.
- X. Zhang, G. Lu, M. Sun, M. Mahankali, Y. Ma, M. Zhang, W. Hua, Y. Hu, Q. Wang, J. Chen, G. He, X. Qi, W. Shen, P. Liu and G. Chen, Nat. Chem., 2018, 10, 540–548 CrossRef CAS PubMed.
- R. Yin, M. Wang, Y. Y. Huang, G. Landi, D. Vecchio, L. Y. Chiang and M. R. Hamblin, Free Radical Biol. Med., 2015, 79, 14–27 CrossRef CAS PubMed.
- J. Stoien and R. J. Wang, Proc. Natl. Acad. Sci. U. S. A., 1974, 71, 3961–3965 CrossRef CAS PubMed.
- J. S. Lebkowski, S. Clancy, J. H. Miller and M. P. Calos, Proc. Natl. Acad. Sci. U. S. A., 1985, 82, 8606–8610 CrossRef CAS PubMed.
- B. M. Sutherland, J. S. Cimino, N. Delihas, A. G. Shih and R. P. Oliver, Cancer Res., 1980, 40, 1934–1939 CAS.
- V. M. Maher, L. M. Ouellette, R. D. Curren and J. J. Mccormick, Nature, 1976, 261, 593–595 CrossRef CAS PubMed.
- C. Marionnet, C. Tricaud and F. Bernerd, Int. J. Mol. Sci., 2015, 16, 68–90 CrossRef PubMed.
- D. R. Green and G. Kroemer, Nature, 2009, 458, 1127–1130 CrossRef CAS PubMed.
- M. F. Holick, Am. J. Clin. Nutr., 1995, 61, 638S–645S CrossRef CAS PubMed.
- T. Y. Chuang, L. A. Heinrich, M. D. Schultz, G. T. Reizner, R. C. Kumm and D. J. Cripps, J. Am. Acad. Dermatol., 1992, 26, 173–177 CrossRef CAS PubMed.
- T. E. C. Nijsten and R. S. Stern, J. Am. Acad. Dermatol., 2003, 49, 644–650 CrossRef PubMed.
- C. Pelli, Y. Futagawa, K. Frampton and G. Associates, Global Architecture, 1981, 40 Search PubMed 46 p. of plates.
- J. Liebmann, M. Born and V. Kolb-Bachofen, J. Invest. Dermatol., 2010, 130, 259–269 CrossRef CAS PubMed.
- C. Opländer, S. Hidding, F. B. Werners, M. Born, N. Pallua and C. V. Suschek, J. Photochem. Photobiol., B, 2011, 103, 118–125 CrossRef PubMed.
- D. S. Masson-Meyers, V. V. Bumah and C. S. Enwemeka, J. Photochem. Photobiol., B, 2016, 160, 53–60 CrossRef CAS PubMed.
- C. Mignon, N. E. Uzunbajakava, B. Raafs, N. V. Botchkareva and D. J. Tobin, Sci. Rep., 2017, 7, 2797 CrossRef CAS PubMed.
- C. Mignon, N. E. Uzunbajakava, B. Raafs, M. Moolenaar, N. V. Botchkareva and D. J. Tobin, Mech. Photobiomodulation Therapy XI, 2016, 9695, 969508 Search PubMed.
- J. A. van Best, B. J. Putting, J. A. Oosterhuis, R. C. Zweypfenning and G. F. Vrensen, Microsc. Res. Tech., 1997, 36, 77–88 CrossRef CAS PubMed.
- B.-L. L. Seagle, E. M. Gasyna, W. F. Mieler and J. R. Norris, Proc. Natl. Acad. Sci. U. S. A., 2006, 103, 16644–16648 CrossRef CAS PubMed.
- A. King, E. Gottlieb, D. G. Brooks, M. P. Murphy and J. L. Dunaief, Photochem. Photobiol., 2007, 79, 470–475 CrossRef.
- A. Wenzel, C. Grimm, M. Samardzija and C. E. Remé, Prog. Retinal Eye Res., 2005, 24, 275–306 CrossRef CAS PubMed.
- T. G. Gorgels and D. van Norren, Invest. Ophthalmol. Visual Sci., 1995, 36, 851–863 CAS.
- J. Vicente-Tejedor, M. Marchena, L. Ramírez, D. García-Ayuso, V. Gómez-Vicente, C. Sánchez-Ramos, P. De La Villa and F. Germain, PLoS One, 2018, 13, e0194218 CrossRef PubMed.
- E. Arnault, C. Barrau, C. Nanteau, P. Gondouin, K. Bigot, F. Viénot, E. Gutman, V. Fontaine, T. Villette, D. Cohen-Tannoudji, J. A. Sahel and S. Picaud, PLoS One, 2013, 8, e71398 CrossRef CAS PubMed.
- L. E. Downie, R. Wormald, J. Evans, G. Virgili, P. R. Keller, J. G. Lawrenson and T. Li, JAMA Ophthalmol., 2019, 137, 694–697 CrossRef PubMed.
- H. T. Whelan, E. V. Buchmann, A. Dhokalia, M. P. Kane, N. T. Whelan, M. T. Wong-Riley, J. T. Eells, L. J. Gould, R. Hammamieh, R. Das and M. Jett, J. Clin. Laser Med. Surg., 2003, 21, 67–74 CrossRef PubMed.
-
K. DeSmet, E. Buchmann, M. Henry, M. Wong-Riley, J. Eells, J. VerHoeve and H. Whelan, Near-infrared light as a possible treatment option for Parkinson's disease and laser eye injury, SPIE, 2009 Search PubMed.
- F. Darlot, C. Moro, N. El Massri, C. Chabrol, D. M. Johnstone, F. Reinhart, D. Agay, N. Torres, D. Bekha, V. Auboiroux, T. Costecalde, C. L. Peoples, H. D. T. Anastascio, V. E. Shaw, J. Stone, J. Mitrofanis and A.-L. Benabid, Ann. Neurol., 2016, 79, 59–75 CrossRef PubMed.
- F. Salehpour, S. H. Rasta, G. Mohaddes, S. Sadigh-Eteghad and S. Salarirad, Lasers Surg. Med., 2016, 48, 695–705 CrossRef PubMed.
- K. A. Muili, S. Gopalakrishnan, S. L. Meyer, J. T. Eells and J.-A. Lyons, PLoS One, 2012, 7, e30655 CrossRef CAS PubMed.
- B. Pucelik, A. Sułek and J. M. Dąbrowski, Coord. Chem. Rev., 2020, 416, 213340 CrossRef CAS.
- L. Cincotta, J. W. Foley and A. H. Cincotta, Photochem. Photobiol., 1987, 46, 751–758 CrossRef CAS PubMed.
- G. Jori, J. Photochem. Photobiol., A, 1992, 62, 371–378 CrossRef CAS.
- R. R. Anderson and J. A. Parrish, J. Invest. Dermatol., 1981, 77, 13–19 CrossRef CAS PubMed.
-
G. B. Altshuler and V. V. Tuchin, in Cosmetics Applications of Laser & Light-Based Systems, ed. G. S. Ahluwalia, William Andrew Publishing, Boston, USA, 2009 Search PubMed.
- C. Ash, M. Dubec, K. Donne and T. Bashford, Lasers Med. Sci., 2017, 32, 1909–1918 CrossRef PubMed.
- A. N. Bashkatov, E. A. Genina, V. I. Kochubey and V. V. Tuchin, J. Phys. D: Appl. Phys., 2005, 38, 2543–2555 CrossRef CAS.
- B. C. Wilson and G. Adam, Med. Phys., 1983, 10, 824–830 CrossRef CAS PubMed.
- T. Binzoni, T. S. Leung, R. Giust, D. Rüfenacht and A. H. Gandjbakhche, Comput. Methods Programs in Biomed., 2008, 89, 14–23 CrossRef CAS PubMed.
- I. V. Meglinski and S. J. Matcher, Physiol. Measurement, 2002, 23, 741–753 CrossRef PubMed.
-
S. Prahl, A Monte Carlo model of light propagation in tissue, SPIE, 1989 Search PubMed.
- S. Bouillaguet, G. Caillot, J. Forchelet, M. Cattani-Lorente, J. C. Wataha and I. Krejci, J. Biomed. Mater. Res., Part B, 2005, 72, 260–267 CrossRef PubMed.
- T. S. Mang, Photodiagn. Photodyn. Therapy, 2004, 1, 43–48 CrossRef PubMed.
- L. Brancaleon and H. Moseley, Lasers Med. Sci., 2002, 17, 173–186 CrossRef CAS PubMed.
- S. H. Yun and S. J. J. Kwok, Nat. Biomed. Eng., 2017, 1, 0008 CrossRef CAS PubMed.
- J. Hempstead, D. P. Jones, A. Ziouche, G. M. Cramer, I. Rizvi, S. Arnason, T. Hasan and J. P. Celli, Sci. Rep., 2015, 5, 10093 CrossRef CAS PubMed.
- S. K. Attili, A. Lesar, A. McNeill, M. Camacho-Lopez, H. Moseley, S. Ibbotson, I. D. W. Samuel and J. Ferguson, Br. J. Dermatol., 2009, 161, 170–173 CrossRef CAS PubMed.
- S. Hofmann, M. Thomschke, P. Freitag, M. Furno, B. Lüssem and K. Leo, Appl. Phys. Lett., 2010, 97, 253308 CrossRef.
- C. Lian, M. Piksa, K. Yoshida, S. Persheyev, K. J. Pawlik, K. Matczyszyn and I. D. W. Samuel, npj Flexible Electron., 2019, 3, 1–6 CrossRef CAS.
- S.-C. Lo, R. N. Bera, R. E. Harding, P. L. Burn and I. D. W. Samuel, Adv. Funct. Mater., 2008, 18, 3080–3090 CrossRef CAS.
- P. L. Burn, S.-C. Lo and I. D. W. Samuel, Adv. Mater., 2007, 19, 1675–1688 CrossRef CAS.
- F. V. Cabral, C. Lian, S. Persheyev, T. K. Smith, M. S. Ribeiro and I. D. W. Samuel, Adv. Mater. Technol., 2021, 6, 2100395 CrossRef CAS.
- U. Melendez-Celis, T. Spezzia-Mazzocco, S. Persheyev, C. Lian, I. Samuel, J. C. Ramirez-San-Juan and R. Ramos-Garcia, Photodiagn. Photodyn. Therapy, 2021, 36, 102567 CrossRef CAS PubMed.
- S. Ibbotson, R. Stones, J. Bowling, S. Campbell, S. Kownacki, M. Sivaramakrishnan, R. Valentine and C. A. Morton, J. Dermatol. Treat., 2017, 28, 360–367 CrossRef CAS PubMed.
- P. O’Mahoney, M. Khazova, E. Eadie and S. Ibbotson, Pharmaceuticals, 2019, 12, 143 CrossRef PubMed.
- C. D. Enk, A. Nasereddin, R. Alper, M. Dan-Goor, C. L. Jaffe and H. C. Wulf, Br. J. Dermatol., 2015, 172, 1364–1370 CrossRef CAS PubMed.
- S. R. Wiegell, H. C. Wulf, R. M. Szeimies, N. Basset-Seguin, R. Bissonnette, M. J. P. Gerritsen, Y. Gilaberte, P. Calzavara-Pinton, C. A. Morton, A. Sidoroff and L. R. Braathen, J. Eur. Acad. Dermatol. Venereol., 2012, 26, 673–679 CrossRef CAS PubMed.
- H. Cordey, R. Valentine, A. Lesar, H. Moseley, E. Eadie and S. Ibbotson, Scott. Med. J., 2017, 62, 48–53 CrossRef PubMed.
- S. R. Wiegell, V. Skødt and H. C. Wulf, J. Eur. Acad. Dermatol. Venereol., 2014, 28, 169–175 CrossRef CAS PubMed.
- C. Nissen, I. Heerfordt, S. Wiegell, C. Mikkelsen and H. Wulf, Acta Dermato Venereologica, 2017, 97, 617–621 CrossRef CAS PubMed.
- M. C. Fargnoli, S. H. Ibbotson, R. E. Hunger, G. Rostain, M. T. W. Gaastra, L. Eibenschutz, C. Cantisani, A. W. Venema, S. Medina, N. Kerrouche and B. Pérez-Garcia, J. Eur. Acad. Dermatol. Venereol., 2018, 32, 757–762 CrossRef CAS PubMed.
- I. M. Heerfordt and H. C. Wulf, J. Eur. Acad. Dermatol. Venereol., 2019, 33, 2058–2061 CrossRef CAS PubMed.
- S. Wiegell, U. Johansen and H. Wulf, Acta Derm.-Venereol., 2019, 99, 242–243 CrossRef CAS PubMed.
- U. Yoshiharu, Chem/Lett., 1973, 2, 743–744 CrossRef.
- K. Bergmann and C. T. O'Konski, J. Phys. Chem., 1963, 67, 2169–2177 CrossRef CAS.
- P. Kaufmann, Dtsch. Med. Wochenschr., 1919, 45, 1365 CrossRef.
- M. Oz, D. E. Lorke, M. Hasan and G. A. Petroianu, Med. Res. Rev., 2011, 31, 93–117 CrossRef CAS PubMed.
- A. Küpfer, C. Aeschlimann, B. Wermuth and T. Cerny, The Lancet, 1994, 343, 763–764 CrossRef PubMed.
- M. Cawein, C. H. Behlen, E. J. Lappat and J. E. Cohn, Arch. Intern. Med., 1964, 113, 578–585 CrossRef CAS PubMed.
- G. Shanmugam, Eur. J. Cardiol.-Thoracic Surg., 2005, 28, 705–710 CrossRef PubMed.
- PROVEPHARM SAS, PROVAYBLUE® (methylene blue) injection USP, for intravenous useInitial U.S. Approval: 2016, 2016.
- E. N. Metz, S. P. Balcerzak and A. L. Sagone, J. Clin. Invest., 1976, 58, 797–802 CrossRef CAS PubMed.
- J. M. May, Z. C. Qu and R. R. Whitesell, Biochem. Pharmacol., 2003, 66, 777–784 CrossRef CAS PubMed.
- Z.-M. Xiong, J. Y. Choi, K. Wang, H. Zhang, Z. Tariq, D. Wu, E. Ko, C. LaDana, H. Sesaki and K. Cao, Aging Cell, 2016, 15, 279–290 CrossRef CAS PubMed.
- G. Roy Choudhury, A. Winters, R. M. Rich, M.-G. Ryou, Z. Gryczynski, F. Yuan, S.-H. Yang and R. Liu, PLoS One, 2015, 10, e0123096 CrossRef PubMed.
- A. Dube, H. Bansal and P. K. Gupta, Indian J. Biochem. Biophys., 2000, 37, 245–250 CAS.
- I. S. Ovchinnikov, G. M. Shoub and V. V. Tuchin, Saratov Fall Meeting '99: Optical Technologies in Biophysics and Medicine, 2000, 3910, 30–34 Search PubMed.
- I. S. Ovchinnikov and V. V. Tuchin, Laser-Tissue Interactions, Therapeutic Applications, and Photodynamic Therapy, 2001, 4433, 160 CrossRef.
- M. C. Teichert, J. W. Jones, M. N. Usacheva and M. A. Biel, Oral Surg., Oral Med., Oral Pathol., Oral Radiol. Endodontol., 2002, 93, 155–160 CrossRef CAS PubMed.
- Y. Chan and Á. C.-H. Lai, Lasers Med. Sci., 2003, 18, 51–55 CrossRef PubMed.
-
T. Yamaguchi, S. Sato, S. Kawauchi, M. Terakawa, N. Shinomiya, D. Saitoh, H. Ashida and M. Obara, 2006.
- S. C. de Souza, J. C. Junqueira, I. Balducci, C. Y. Koga-Ito, E. Munin and A. O. C. Jorge, J. Photochem. Photobiol., B, 2006, 83, 34–38 CrossRef CAS PubMed.
- N. S. Soukos, P. S. Y. Chen, J. T. Morris, K. Ruggiero, A. D. Abernethy, S. Som, F. Foschi, S. Doucette, L. Luschke Bammann, C. R. Fontana, A. G. Doukas and P. P. Stashenko, J. Endodontics, 2006, 32, 979–984 CrossRef PubMed.
- F. Foschi, C. R. Fontana, K. Ruggiero, R. Riahi, A. Vera, A. G. Doukas, T. C. Pagonis, R. Kent, P. P. Stashenko and N. S. Soukos, Lasers Surg. Med., 2007, 39, 782–787 CrossRef PubMed.
- M. Ogura, A. D. Abernethy, R. D. Blissett, K. Ruggiero, S. Som, J. M. Goodson, R. Kent, A. G. Doukas and N. S. Soukos, World J. Microbiol. Biotechnol., 2007, 23, 1637–1646 CrossRef CAS.
- E. Munin, L. M. Giroldo, L. P. Alves and M. S. Costa, J. Photochem. Photobiol., B, 2007, 88, 16–20 CrossRef CAS PubMed.
- S. George and A. Kishen, J. Endodontics, 2007, 33, 599–602 CrossRef PubMed.
-
R. A. Prates, E. G. da Silva, J. Yamada, M. Aécio, L. C. Suzuki, C. R. Paula and M. S. Ribeiro, 2008.
- A. M. Yamada Júnior, R. A. Prates, S. Cai and M. S. Ribeiro, Mech. Low-Light Therapy III, 2008, 6846, 68460F Search PubMed.
- S. George and A. Kishen, J. Endodontics, 2008, 34, 1119–1123 CrossRef PubMed.
- J. L. Fimple, C. R. Fontana, F. Foschi, K. Ruggiero, X. Song, T. C. Pagonis, A. C. R. Tanner, R. Kent, A. G. Doukas, P. P. Stashenko and N. S. Soukos, J. Endodontics, 2008, 34, 728–734 CrossRef PubMed.
- C. R. Fontana, A. D. Abernethy, S. Som, K. Ruggiero, S. Doucette, R. C. Marcantonio, C. I. Boussios, R. Kent, J. M. Goodson, A. C. R. Tanner and N. S. Soukos, J. Periodontal Res., 2009, 44, 751–759 CrossRef CAS PubMed.
- S. Tubby, M. Wilson and S. P. Nair, BMC Microbiol., 2009, 9, 1–10 CrossRef PubMed.
- J. C. Junqueira, J. Da Silva Martins, R. L. Faria, C. E. D. Colombo and A. O. C. Jorge, Lasers Med. Sci., 2009, 24, 877–884 CrossRef PubMed.
- G. G. Carvalho, M. P. Felipe and M. S. Costa, J. Microbiol., 2009, 47, 619–623 CrossRef CAS PubMed.
- R. A. Prates, E. G. da Silva, P. F. Chaves, A. J. S. Santos, C. R. Paula and M. S. Ribeiro, Mech. Low-Light Therapy IV, 2009, 7165, 71650H Search PubMed.
- R. A. Prates, E. G. Silva, A. M. Yamada, L. C. Suzuki, C. R. Paula and M. S. Ribeiro, Laser Phys., 2009, 19, 1038–1044 CrossRef CAS.
- R. F. Donnelly, C. M. Cassidy, R. G. Loughlin, A. Brown, M. M. Tunney, M. G. Jenkins and P. A. McCarron, J. Photochem. Photobiol., B, 2009, 96, 223–231 CrossRef CAS PubMed.
- L. A. Pedigo, A. J. Gibbs, R. J. Scott and C. N. Street, Photodyn. Ther., 2009, 7380, 7380H Search PubMed.
- C. N. Street, A. Gibbs, L. Pedigo, D. Andersen and N. G. Loebel, Photochem. Photobiol., 2009, 85, 137–143 CrossRef CAS PubMed.
- C. N. Street, L. Pedigo, A. Gibbs and N. G. Loebel, Photodyn. Ther., 2009, 7380, 73803B Search PubMed.
- L. M. Giroldo, M. P. Felipe, M. A. De Oliveira, E. Munin, L. P. Alves and M. S. Costa, Lasers Med. Sci., 2009, 24, 109–112 CrossRef PubMed.
- C. N. Street, L. A. Pedigo and N. G. Loebel, Photomed. Laser Surg., 2010, 28, S61–S66 CrossRef CAS PubMed.
- R. C. Souza, J. C. Junqueira, R. D. Rossoni, C. A. Pereira, E. Munin and A. O. C. Jorge, Lasers Med. Sci., 2010, 25, 385–389 CrossRef PubMed.
- F. P. Gonzales, S. H. Da Silva, D. W. Roberts and G. U. L. Braga, Photochem. Photobiol., 2010, 86, 653–661 CrossRef CAS PubMed.
- L. C. Souza, P. R. R. Brito, J. C. Machado de Oliveira, F. R. F. Alves, E. J. L. Moreira, H. R. Sampaio-Filho, I. N. Rôças and J. F. Siqueira, J. Endodontics, 2010, 36, 292–296 CrossRef PubMed.
- S. C. Núñez, M. S. Ribeiro, A. Silva Garcez and W. Miyakawa, Biophotonics, 2010, 7715, 77151L Search PubMed.
- A. Hirao, S. Sato, M. Terakawa, D. Saitoh, N. Shinomiya, H. Ashida and M. Obara, Optical Methods for Tumor Treatment and Detection: Mechanisms and Techniques in Photodynamic Therapy XIX, 2010, 7551, 75510V Search PubMed.
- M. H. Shin and F. C. Huang, Invest. Ophthalmol. Visual Sci., 2011, 52, 223–229 CrossRef PubMed.
- I. T. Kato, R. A. Prates, G. P. Tegos, M. R. Hamblin and M. S. Ribeiro, Mech. Low-Light Therapy VI, 2011, 7887, 78870B Search PubMed.
- A. S. Queiroga, V. N. Trajano, E. O. Lima, A. F. M. Ferreira, A. S. Queiroga and F. A. Limeira, Photodiagn. Photodyn. Therapy, 2011, 8, 332–336 CrossRef CAS PubMed.
- R. A. Prates, A. Cio, M. Yamada, L. C. Suzuki, C. M. Franç, S. Cai, M. Rcia, P. A. Mayer, A. C. Ribeiro and M. S. Ribeiro, Photomed. Laser Surg., 2011, 29, 835–844 CrossRef CAS PubMed.
- M. A. Biel, C. Sievert, M. Usacheva, M. Teichert and J. Balcom, Int. Forum Allergy Rhinol., 2011, 1, 329–334 CrossRef PubMed.
- C. d A. B. Guglielmi, M. R. L. Simionato, K. M. Ramalho, J. C. P. Imparato, S. L. Pinheiro and M. A. A. C. Luz, J. Biomed. Opt., 2011, 16, 088003 CrossRef PubMed.
- M. Miyabe, J. C. Junqueira, A. C. Borges, P. D. Costa, A. O. C. Jorge, M. S. Ribeiro, I. S. Feist and A. C. B. P. D. Costa, Braz. Oral Res., 2011, 25, 230–234 CrossRef PubMed.
- A. R. Scwingel, A. R. P. Barcessat, S. C. Núñez and M. S. Ribeiro, Photomed. Laser Surg., 2012, 30, 429–432 CrossRef CAS PubMed.
- S.-l Lin, J.-m Hu, S.-s Tang, X.-y Wu, Z.-q Chen and S.-z Tang, Photochem. Photobiol., 2012, 88, 985–991 CrossRef CAS PubMed.
- M. Wainwright, H. Smalley, O. Scully and E. Lotfipour, Photochem. Photobiol., 2012, 88, 523–526 CrossRef CAS PubMed.
- N. Kashef, G. Ravaei Sharif Abadi and G. E. Djavid, Photodiagn. Photodyn. Therapy, 2012, 9, 11–15 CrossRef CAS PubMed.
- A. F. Barbosa, B. B. Sangiorgi, S. L. Galdino, M. Barral-Netto, I. R. Pitta and A. L. Pinheiro, Lasers Surg. Med., 2012, 44, 850–855 CrossRef PubMed.
- C. Komine and Y. Tsujimoto, J. Endodontics, 2013, 39, 411–414 CrossRef PubMed.
- A. F. S. Barbosa, B. B. Sangiorgi, S. L. Galdino, I. R. Pitta, M. Barral-Netto and A. L. B. Pinheiro, Mecha. Low-Light Therapy VIII, 2013, 8569, 85690N Search PubMed.
- S. C. P. S. Oliveira, G. M. P. Santos, J. S. C. Monteiro, A. F. S. Miranda, F. J. P. Sampaio, M. F. M. Gesteira, F. A. A. Zanin, M. A. V. Santos and A. L. B. Pinheiro, Mech. Low-Light Therapy VIII, 2013, 8569, 85690P Search PubMed.
- I. T. Kato, R. A. Prates, C. P. Sabino, B. B. Fuchs, G. P. Tegos, E. Mylonakis, M. R. Hamblin and M. S. Ribeiro, Antimicrob. Agents Chemother., 2013, 57, 445–451 CrossRef CAS PubMed.
- A. S. Garcez, S. C. Núñez, N. Azambuja, E. R. Fregnani, H. M. H. Rodriguez, M. R. Hamblin, H. Suzuki and M. S. Ribeiro, Photomed. Laser Surg., 2013, 31, 519 CrossRef CAS PubMed.
- S. Tubby, M. Wilson, J. A. Wright, P. Zhang and S. P. Nair, BMC Microbiol., 2013, 13, 201 CrossRef PubMed.
- S. Stojicic, H. Amorim, Y. Shen and M. Haapasalo, Int. Endodontic J., 2013, 46, 649–659 CrossRef CAS PubMed.
- N. Kashef, M. Akbarizare and S. K. Kamrava, Photodiagn. Photodyn. Therapy, 2013, 10, 368–373 CrossRef CAS PubMed.
- I. Maliszewska, A. Leśniewska, J. Olesiak-Bańska, K. Matczyszyn and M. Samoć, J. Nanopart. Res., 2014, 16, 2457 CrossRef.
- G. C. Ghinzelli, M. A. Souza, D. Cecchin, A. P. Farina and J. A. P. de Figueiredo, Photodiagn. Photodyn. Therapy, 2014, 11, 472–478 CrossRef PubMed.
- Y. Wang and X. Huang, Photochem. Photobiol., 2014, 90, 1084–1088 CrossRef CAS PubMed.
- R. D. Rossoni, J. O. Barbosa, F. E. de Oliveira, L. D. de Oliveira, A. O. C. Jorge and J. C. Junqueira, Lasers Med. Sci., 2014, 29, 1679–1684 CrossRef PubMed.
- R. M. Machado-de-Sena, L. Corrêa, I. T. Kato, R. A. Prates, A. M. Senna, C. C. Santos, D. A. Picanço and M. S. Ribeiro, Photodiagn. Photodyn. Therapy, 2014, 11, 275–282 CrossRef CAS PubMed.
- T. Ye, B. Chen, B. Yu, Q. Zhong, G. Huang, X. Hu and W. Zhang, Photochem. Photobiol., 2015, 91, 917–922 CrossRef CAS PubMed.
- F. Freire, P. P. de Barros, D. da Silva Ávila, G. N. B. Brito, J. C. Junqueira and A. O. C. Jorge, Lasers Med. Sci., 2015, 30, 1511–1518 CrossRef PubMed.
- L. López-Jiménez, E. Fusté, B. Martínez-Garriga, J. Arnabat-Domínguez, T. Vinuesa and M. Viñas, Lasers Med. Sci., 2015, 30, 1519–1526 CrossRef PubMed.
- L. P. Rosa, F. C. Da Silva, S. A. Nader, G. A. Meira and M. S. Viana, Arch. Oral Biol., 2015, 60, 675–680 CrossRef CAS PubMed.
- L. P. Rosa, F. C. d Silva, S. A. Nader, G. A. Meira and M. S. Viana, Photodiagn. Photodyn. Therapy, 2015, 12, 276–281 CrossRef CAS PubMed.
- K. M. Ramalho, R. G. Rocha, A. C. Correa-Aranha, S. R. D. B. Cunha, A. Simões, L. Campos and C. D. P. Eduardo, Photodiagn. Photodyn. Therapy, 2015, 12, 321–323 CrossRef PubMed.
- C. Steiner-Oliveira, P. L. Longo, A. C. C. Aranha, K. M. Ramalho, M. P. A. Mayer and C. de Paula Eduardo, J. Biomed. Opt., 2015, 20, 108003 CrossRef PubMed.
- A. Donauerová, J. Bujdák, M. Smolinská and H. Bujdáková, J. Photochem. Photobiol., B, 2015, 151, 135–141 CrossRef PubMed.
- I. M. A. Diniz, I. D. Horta, C. S. Azevedo, T. R. Elmadjian, A. B. Matos, M. R. L. Simionato and M. M. Marques, Photodiagn. Photodyn. Therapy, 2015, 12, 511–518 CrossRef CAS PubMed.
- L. De Sordi, M. Adil Butt, H. Pye, D. Kohoutova, C. A. Mosse, G. Yahioglu, I. Stamati, M. Deonarain, S. Battah, D. Ready, E. Allan, P. Mullany and L. B. Lovat, PLoS One, 2015, 10, e0135039 CrossRef PubMed.
- C. P. Sabino, A. S. Garcez, S. C. Núñez and M. R. Hamblin, Lasers Med Sci, 2015, 30, 1657–1665 CrossRef CAS PubMed.
- P. A. M. Neves, L. A. Lima, F. C. N. Rodrigues, T. J. Leitão and C. C. C. Ribeiro, Braz. Oral Res., 2016, 30, S1806–83242016000100246 CrossRef PubMed.
- E. Darabpour, N. Kashef and S. Mashayekhan, Photodiagn. Photodyn. Therapy, 2016, 14, 211–217 CrossRef CAS PubMed.
- F. Freire, C. Ferraresi, A. Olavo, C. Jorge and M. R. Hamblin, J. Photochem. Photobiol., B, 2016, 157, 161–168 CrossRef PubMed.
- A. Azizi, Z. Amirzadeh, M. Rezai, S. Lawaf and A. Rahimi, J. Photochem. Photobiol., B, 2016, 158, 267–273 CrossRef CAS PubMed.
- A. Azizi, S. Shademan, M. Rezai, A. Rahimi and S. Lawaf, Photodiagn. Photodyn. Therapy, 2016, 16, 66–71 CrossRef CAS PubMed.
- L. M. de Freitas, G. M. F. Calixto, M. Chorilli, J. S. M. Giusti, V. S. Bagnato, N. S. Soukos, M. M. Amiji and C. R. Fontana, Int. J. Mol. Sci., 2016, 17, 769 CrossRef PubMed.
- Y. Long, Z. Li, Q. Bi, C. Deng, Z. Chen, S. Bhattachayya and C. Li, Int. J. Pharm., 2016, 502, 232–241 CrossRef CAS PubMed.
- M. Asnaashari, M. Godiny, S. Azari-Marhabi, F. S. Tabatabaei and M. Barati, J. Lasers Med. Sci., 2016, 7, 99–104 CrossRef PubMed.
- J. A. Soares, S. M. C. Santos Soares, C. A. Santos César, M. A. R. de Carvalho, M. Brito-Júnior, G. R. de Sousa, B. M. Soares and L. de Macêdo Farias, Photodiagn. Photodyn. Therapy, 2016, 13, 123–127 CrossRef CAS PubMed.
- M. G. Corrêa, D. H. Oliveira, C. H. C. Saraceni, F. V. Ribeiro, S. P. Pimentel, F. R. Cirano and R. C. V. Casarin, Lasers Surg. Med., 2016, 48, 944–950 CrossRef PubMed.
- H. D. de Menezes, L. Tonani, L. Bachmann, M. Wainwright, G. Ú. L. Braga and M. R. von Zeska Kress, J. Photochem. Photobiol., B, 2016, 164, 1–12 CrossRef CAS PubMed.
- C. A. Fabio, M. B. Yolanda, G. M. Carmen, C. Francisco, B. Antonio Julián, P. L. Leonor and S. Jesús, J. Oral Pathol. Med., 2016, 45, 627–633 CrossRef CAS PubMed.
- A. F. Forte Giacobone, M. F. Ruiz Gale, E. N. Hogert and O. J. Oppezzo, Photochem. Photobiol., 2016, 92, 702–707 CrossRef CAS PubMed.
- M. Pourhajibagher, N. Chiniforush, S. Shahabi, R. Ghorbanzadeh and A. Bahador, Photodiagn. Photodyn. Therapy, 2016, 15, 159–166 CrossRef CAS PubMed.
- M. Pourhajibagher and A. Bahador, Photodiagn. Photodyn. Therapy, 2017, 20, 1–5 CrossRef CAS PubMed.
- K. F. Grego, M. P. N. d Carvalho, M. P. V. Cunha, T. Knöbl, F. C. Pogliani, J. L. Catão-Dias, S. S. Sant'Anna, M. S. Ribeiro and F. P. Sellera, Photodiagn. Photodyn. Therapy, 2017, 20, 196–200 CrossRef PubMed.
- M. A. Nemezio, S. S. de Souza Farias, M. C. Borsatto, C. P. Aires and S. A. M. Corona, Photodiagn. Photodyn. Therapy, 2017, 20, 234–237 CrossRef CAS PubMed.
- S. de Oliveira, E. J. da Ordem Trahamane, J. Monteiro, G. P. Santos, P. Crugeira, F. Sampaio, C. Oliveira, M. B. Neto and A. Pinheiro, Lasers Med. Sci., 2017, 32, 1959–1964 CrossRef PubMed.
- E. Darabpour, N. Kashef, S. M. Amini, S. Kharrazi and G. E. Djavid, J. Drug Delivery Sci. Technol., 2017, 37, 134–140 CrossRef CAS.
- C. C. N. Sebraõ, A. G. Bezerra, P. H. C. De Francą, L. E. Ferreira and V. P. D. Westphalen, Photomed. Laser Surg., 2017, 35, 18–23 CrossRef PubMed.
- A. S. Garcez and M. R. Hamblin, Eur. Endodontic J., 2017, 2, 29 CrossRef PubMed.
- L. Černáková, S. Dižová and H. Bujdáková, Med. Mycol., 2017, 55, 748–753 Search PubMed.
- S. Khan, S. N. Khan, R. Meena, A. M. Dar, R. Pal and A. U. Khan, J. Photochem. Photobiol., B, 2017, 174, 150–161 CrossRef CAS PubMed.
- M. Pourhajibagher, A. Monzavi, N. Chiniforush, M. M. Monzavi, S. Sobhani, S. Shahabi and A. Bahador, Photodiagn. Photodyn. Therapy, 2017, 18, 78–82 CrossRef CAS PubMed.
- M. Giannelli, G. Landini, F. Materassi, F. Chellini, A. Antonelli, A. Tani, D. Nosi, S. Zecchi-Orlandini, G. M. Rossolini and D. Bani, Lasers Med. Sci., 2017, 32, 857–864 CrossRef PubMed.
- L. Silva de Figueiredo Freitas, R. Dennis Rossoni, A. Olavo Cardoso Jorge and J. Campos Junqueira, Lasers Med. Sci., 2017, 32, 549–555 CrossRef PubMed.
- F. Camacho-Alonso, E. Julián-Belmonte, F. Chiva-García and Y. Martínez-Beneyto, Photomed. Laser Surg., 2017, 35, 184–189 CrossRef CAS PubMed.
- E. M. Decker, V. Bartha and C. Von Ohle, Photomed. Laser Surg., 2017, 35, 195–205 CrossRef CAS PubMed.
- M. Shirato, K. Nakamura, T. Kanno, P. Lingström, Y. Niwano and U. Örtengren, J. Photochem. Photobiol., B, 2017, 173, 434–440 CrossRef CAS PubMed.
- H. Kariminezhad, H. Amani, R. Khanbabaie and M. Biglarnia, Appl. Biochem. Biotechnol., 2017, 182, 967–977 CrossRef CAS PubMed.
- M. Pourhajibagher, R. Ghorbanzadeh and A. Bahador, Infection Drug Resistance, 2018, 11, 717–725 CrossRef CAS PubMed.
- Z. Sun, S. Zhou, H. Qiu, Y. Gu and Y. Zhao, RSC Adv., 2018, 8, 17073–17078 RSC.
- A. Azizi, S. Mousavian, S. Taheri, S. Lawaf, E. Gonoudi and A. Rahimi, Photodiagn. Photodyn. Therapy, 2018, 21, 357–362 CrossRef CAS PubMed.
- C. C. da Silva, S. P. Chaves
Júnior, G. L. D. Pereira, K. B. F. D. C. Fontes, L. A. A. Antunes, H. C. C. Póvoa, L. S. Antunes and N. L. P. P. Iorio, Photochem. Photobiol., 2018, 94, 351–356 CrossRef CAS PubMed.
- T. Briggs, G. Blunn, S. Hislop, R. Ramalhete, C. Bagley, D. McKenna and M. Coathup, Lasers Med. Sci., 2018, 33, 523–532 CrossRef PubMed.
- Y. Nagai, A. Suzuki, H. Katsuragi and K. Shinkai, Odontology, 2018, 106, 154–161 CrossRef CAS PubMed.
- R. G. de Miranda and A. P. V. Colombo, Clin. Oral Invest., 2018, 22, 1751–1761 CrossRef PubMed.
- Y. Y. Huang, A. Wintner, P. C. Seed, T. Brauns, J. A. Gelfand and M. R. Hamblin, Sci. Rep., 2018, 8, 7257 CrossRef PubMed.
- J. A. Soares, S. M. C. S. Soares, R. R. de Jesus Tavarez, C. de Castro Rizzi, S. C. G. Vaz Rodrigues, E. M. Maia Filho, M. Brito-Júnior, R. D. Pereira, P. P. Magalhães and L. de Macêdo Farias, Photodiagn. Photodyn. Therapy, 2018, 22, 127–131 CrossRef PubMed.
- A. M. de Senna, M. M. F. Vieira, R. M. Machado-de-Sena, A. O. Bertolin, S. C. Núñez and M. S. Ribeiro, Photodiagn. Photodyn. Therapy, 2018, 22, 212–216 CrossRef PubMed.
- G. C. S. Vieira, H. S. Antunes, A. R. Pérez, L. S. Gonçalves, F. E. Antunes, J. F. Siqueira and I. N. Rôças, J. Endodontics, 2018, 44, 1593–1597 CrossRef PubMed.
- T. F. Gomes, M. M. Pedrosa, A. C. L. de Toledo, V. W. Arnoni, M. dos Santos Monteiro, D. C. Piai, S. H. Z. Sylvestre and B. Ferreira, Lasers Med. Sci., 2018, 33, 1723–1731 CrossRef PubMed.
- P. O. Ornellas, L. S. Antunes, P. C. Motta, C. Mendonça, H. Póvoa, K. Fontes, N. Iorio and L. A. A. Antunes, Photochem. Photobiol., 2018, 94, 1240–1248 CrossRef CAS PubMed.
- M. E. S. O. de Santi, R. A. Prates, C. M. França, R. G. Lopes, A. S. Sousa, L. R. Ferreira, S. K. Bussadori, A. U. Fernandes and A. M. Deana, Lasers Med. Sci., 2018, 33, 1925–1931 CrossRef PubMed.
- M. Pourhajibagher, H. Kazemian, N. Chiniforush, N. Hosseini, B. Pourakbari, A. Azizollahi, F. Rezaei and A. Bahador, Photodiagn. Photodyn. Therapy, 2018, 24, 206–211 CrossRef CAS PubMed.
- M. Terra Garcia, A. H. Correia Pereira, L. M. A. Figueiredo-Godoi, A. O. C. Jorge, J. F. Strixino and J. C. Junqueira, Photodiagn. Photodyn. Therapy, 2018, 24, 256–261 CrossRef CAS PubMed.
- N. Chiniforush, M. Pourhajibagher, S. Parker, S. Benedicenti, S. Shahabi and A. Bahador, Photodiagn. Photodyn. Therapy, 2018, 24, 311–317 CrossRef CAS PubMed.
- J. A. R. Ambrosio, B. C. D. S. Pinto, D. D. S. Godoy, J. A. Carvalho, A. D. S. Abreu, B. G. M. da Silva, L. D. C. Leonel, M. S. Costa, M. Beltrame Junior and A. R. Simioni, J. Biomater. Sci., Polym. Ed., 2019, 30, 1356–1373 CrossRef CAS PubMed.
- M. E. O. C. Vasconcelos, A. A. Cardoso, J. N. Da Silva, F. J. R. Alexandrino, R. N. Stipp, M. Nobre-Dos-Santos, L. K. A. Rodrigues and C. Steiner-Oliveira, Photobiomodulation, Photomed., Laser Surg., 2019, 37, 567–573 CrossRef CAS PubMed.
- L. H. Alvarenga, A. C. Gomes, P. Carribeiro, B. Godoy-Miranda, G. Noschese, M. S. Ribeiro, I. T. Kato, S. K. Bussadori, C. Pavani, Y. G. E. Geraldo, D. D. F. T. D. Silva, A. C. R. T. Horliana, M. Wainwright and R. A. Prates, Photodiagn. Photodyn. Therapy, 2019, 27, 132–136 CrossRef CAS PubMed.
- A. Azizi, P. Shohrati, M. Goudarzi, S. Lawaf and A. Rahimi, Photodiagn. Photodyn. Therapy, 2019, 27, 203–209 CrossRef CAS PubMed.
- S. Hendiani, M. L. Rybtke, T. Tolker-Nielsen and N. Kashef, Photodiagn. Photodyn. Therapy, 2019, 27, 467–473 CrossRef CAS PubMed.
- S. Hendiani, M. Pornour and N. Kashef, Lasers Med. Sci., 2019, 34, 1159–1165 CrossRef PubMed.
- P. Parasuraman, V. T. Anju, S. B. Sruthil Lal, A. Sharan, S. Busi, K. Kaviyarasu, M. Arshad, T. M. S. Dawoud and A. Syed, Photochem. Photobiol. Sci., 2019, 18, 563–576 CrossRef CAS PubMed.
- L. A. Valle, M. M. R. Lopes, M. S. R. Zangrando, A. C. P. Sant'Ana, S. L. A. Greghi, M. L. R. de Rezende and C. A. Damante, J. Photochem. Photobiol., B, 2019, 194, 56–60 CrossRef CAS PubMed.
- S. M. Yang, D. W. Lee, H. J. Park, M. H. Kwak, J. M. Park and M. G. Choi, Photochem. Photobiol., 2019, 95, 833–838 CrossRef CAS PubMed.
- F. Daliri, A. Azizi, M. Goudarzi, S. Lawaf and A. Rahimi, Photodiagn. Photodyn. Therapy, 2019, 26, 193–198 CrossRef CAS PubMed.
- S. Niavarzi, M. Pourhajibagher, S. Khedmat, S. Ghabraei, N. Chiniforush and A. Bahador, Photodiagn. Photodyn. Therapy, 2019, 27, 362–366 CrossRef CAS PubMed.
- I. Maliszewska, J. Wróbel, E. Wanarska, A. Podhorodecki and K. Matczyszyn, Photodiagn. Photodyn. Therapy, 2019, 27, 218–226 CrossRef CAS PubMed.
- L. M. de Freitas, E. N. Lorenzón, E. M. Cilli, K. T. de Oliveira, C. R. Fontana and T. S. Mang, Biofouling, 2019, 35, 742–757 CrossRef CAS PubMed.
- L. M. A. Figueiredo-Godoi, R. T. Menezes, J. S. Carvalho, M. T. Garcia, A. G. Segundo, A. O. C. Jorge and J. C. Junqueira, Photodiagn. Photodyn. Therapy, 2019, 27, 66–73 CrossRef CAS PubMed.
- M. L. D. M. F. Fernandes, C. A. Maia, A. M. C. Santos, C. R. Vilela, F. R. Araujo, M. D. L. Mohallen, L. B. Silveira and A. M. Fernandes, Pesqui. Bras. Odontopediatr. Clin. Integr., 2020, 20, 1–9 CAS.
- M. H. C. D. V. Catão and A. L. A. Batista, Pesqui. Bras. Odontopediatr. Clin. Integr., 2020, 20, 196–200 Search PubMed.
- C. B. Okamoto, S. K. Bussadori, R. A. Prates, A. C. C. da Mota, A. Horliana, K. P. S. Fernandes and L. J. Motta, Photodiagn. Photodyn. Therapy, 2020, 30, 101732 CrossRef CAS PubMed.
- K. Legenova, M. Kovalcikova, L. Cernakova and H. Bujdakova, Curr. Microbiol., 2020, 77, 988–996 CrossRef CAS PubMed.
- A. F. S. Barbosa, I. P. Santos, G. M. P. Santos, T. M. Bastos, V. P. C. Rocha, C. S. Meira, M. B. P. Soares, I. R. Pitta and A. L. B. Pinheiro, Lasers Med. Sci., 2020, 35, 79–85 CrossRef PubMed.
- R. B. Soares, D. H. Costa, W. Miyakawa, M. G. T. Delgado, A. S. Garcez, T. M. Yoshimura, M. S. Ribeiro and S. C. Nunez, Photochem. Photobiol., 2020, 96, 618–624 CrossRef CAS PubMed.
- G. Fonseca, D. C. Dourado, M. P. Barreto, M. Cavalcanti, M. D. Pavelski, L. B. Q. Ribeiro and L. Frigo, Photodiagn. Photodyn. Therapy, 2020, 30, 101686 CrossRef CAS PubMed.
- T. Almohareb, N. Alhamoudi, M. Al Deeb, M. S. Bin-Shuwaish, S. A. Mokeem, S. S. Shafqat, F. Vohra and T. Abduljabbar, Photodiagn. Photodyn. Therapy, 2020, 30, 101750 CrossRef CAS PubMed.
- R. J. Li, L. T. Yuan, W. Q. Jia, M. Qin and Y. G. Wang, Lasers Surg. Med., 2021, 53, 400–410 CrossRef PubMed.
- A. Tortamano, G. G. Anselmo, C. T. Kassa, B. Godoy-Miranda, C. Pavani, I. T. Kato, M. Wainwright and R. A. Prates, Photodiagn. Photodyn. Therapy, 2020, 31, 101784 CrossRef CAS PubMed.
- G. S. Furtado, M. A. B. Paschoal, L. D. S. Grenho and A. D. N. Lago, Photodiagn. Photodyn. Therapy, 2020, 31, 101884 CrossRef CAS PubMed.
- A. Alshahrani, R. A. Togoo, M. A. Kamran and I. Alshahrani, Photodiagn. Photodyn. Therapy, 2020, 31, 101978 CrossRef PubMed.
- N. K. Al Malik and O. H. Alkadhi, Photodiagn. Photodyn. Therapy, 2020, 31, 101768 CrossRef PubMed.
- X. Y. Liang, Z. H. Zou, Z. Zou, C. Y. Li, X. X. Dong, H. J. Yin and G. H. Yan, J. Innovative Opt. Health Sci., 2020, 13, 2050022 CrossRef CAS.
- O. Nadtoka, P. Virych, S. Nadtoka and N. Kutsevol, J. Chem., 2020, 2020, 6679960 Search PubMed.
- A. D. N. Lago, B. C. Fortes, G. S. Furtado, C. F. S. Menezes and L. M. Goncalves, Photodiagn. Photodyn. Therapy, 2020, 32, 102070 CrossRef CAS PubMed.
- N. D. Goncalves, V. M. Borges, J. A. A. de Arruda, E. G. dos Santos, I. M. A. Diniz, M. F. M. Madeira and A. Moreno, Photodiagn. Photodyn. Therapy, 2020, 32, 102042 CrossRef CAS PubMed.
- V. A. Svyatchenko, S. D. Nikonov, A. P. Mayorov, M. L. Gelfond and V. B. Loktev, Photodiagn. Photodyn. Therapy, 2021, 33, 102112 CrossRef CAS PubMed.
- K. M. Ramalho, S. R. Cunha, F. Goncalves, G. S. Escudeiro, C. Steiner-Oliveira, A. Horliana and C. D. Eduardo, Photodiagn. Photodyn. Therapy, 2021, 33, 102093 CrossRef CAS PubMed.
- G. R. de Sousa, L. O. Soares, B. M. Soares, R. D. Cruz, P. Uliana, T. Santiago, L. M. Farias, P. P. Magalhaes, L. B. Silveira, L. A. Lopes, M. W. Mancini, R. Huebner and M. V. L. Ferreira, Lasers Med. Sci., 2022, 37, 391–401 CrossRef PubMed.
- L. Y. Yamamoto, C. Loureiro, L. T. A. Cintra, R. D. Leonardo, H. A. Banci, A. P. F. Ribeiro, G. Sivieri-Araujo and R. D. Jacinto, Photodiagn. Photodyn. Therapy, 2021, 35, 102377 CrossRef CAS PubMed.
- P. Virych, O. Nadtoka, P. Virych and N. Kutsevol, Mol. Cryst. Liq. Cryst., 2021, 716, 123–128 CrossRef CAS.
- S. D. Moreira, J. B. Nunes, F. A. Colombo, N. D. M. Fonseca and N. V. Viola, Photodiagn. Photodyn. Therapy, 2021, 35, 102433 CrossRef CAS PubMed.
- F. Lavaee, M. Motamedifar and G. Rafiee, Lasers Med. Sci., 2022, 37, 1717–1725 CrossRef PubMed.
- F. V. Cabral, F. P. Sellera and M. S. Ribeiro, Photodiagn. Photodyn. Therapy, 2021, 36, 102602 CrossRef CAS PubMed.
- D. E. Mocuta, M. I. Miron, E. Hogea, C. Muntean and D. C. Todea, Int. J. Environ. Res. Public Health, 2021, 18, 12847 CrossRef PubMed.
- L. O. Silva, K. L. D. Souza, L. D. Beloti, W. M. R. Neto, S. C. Nunez and D. F. R. Frias, Lasers Med. Sci., 2022, 37, 2305–2310 CrossRef PubMed.
- F. G. L. Fernandes, F. B. de Moraes, J. A. De Cezare, G. R. Degasperi, C. E. Fontana, D. R. P. Grandizoli and S. L. Pinheiro, Photodiagn. Photodyn. Therapy, 2022, 37, 102718 CrossRef CAS PubMed.
- J. Benine-Warlet, A. Brenes-Alvarado and C. Steiner-Oliveira, Photodiagn. Photodyn. Therapy, 2022, 37, 102622 CrossRef CAS PubMed.
- J. Arentz and H. J. Von der Heide, Photodiagn. Photodyn. Therapy, 2022, 37, 102642 CrossRef CAS PubMed.
- I. S. Ovchinnikov, D. E. Popov, V. V. Tuchin, O. G. Shapoval and G. M. Shub, Saratov Fall Meeting 2002 Laser Physics and Photonics, Spectroscopy, and Molecular Modeling III, 2003, 5068, 437–441 Search PubMed.
- N. A. Romanova, L. Y. Brovko, L. Moore, E. Pometun, A. P. Savitsky, N. N. Ugarova and M. W. Griffiths, Appl. Environ. Microbiol., 2003, 69, 6393–6398 CrossRef CAS PubMed.
- Q. Huang, W. L. Fu, B. Chen, J. F. Huang, X. Zhang and Q. Xue, J. Photochem. Photobiol., B, 2004, 77, 39–43 CrossRef CAS PubMed.
- M. Wainwright, A. Stanforth, R. Jones, C. Loughran and K. Meegan, Lett. Appl. Microbiol., 2010, 50, 486–492 CrossRef CAS PubMed.
- C. M. Cassidy, R. F. Donnelly and M. M. Tunney, J. Photochem. Photobiol., B, 2010, 99, 62–66 CrossRef CAS PubMed.
- E. G. d O. Mima, A. C. Pavarina, L. N. Dovigo, C. E. Vergani, C. A. d S. Costa, C. Kurachi and V. S. Bagnato, Oral Surg., Oral Med., Oral Pathol., Oral Radiol. Endodontol., 2010, 109, 392–401 CrossRef PubMed.
- A. Baptista, R. Araujo Prates, I. T. Kato, M. M. Amaral, A. Zanardi de Freitas and M. S. Ribeiro, Biophotonics, 2010, 7715, 77151R Search PubMed.
- L. C. Suzuki, R. Araujo Prates, M. P. Raele, A. Zanardi di Freitas and M. S. Ribeiro, Biophotonics, 2010, 7715, 771524 Search PubMed.
- L. F. De Paula, R. O. Santos, H. D. Menezes, J. R. De Britto, J. B. Vieira, P. P. G. Filho and C. A. De Oliveira, J. Braz. Chem. Soc., 2010, 21, 694–700 CrossRef CAS.
- T. W. Wong, H. J. Huang, Y. F. Wang, Y. P. Lee, C. C. Huang and C. K. Yu, J. Antimicrob. Chemother., 2010, 65, 2176–2182 CrossRef CAS PubMed.
- I. Angelov, V. Mantareva, V. Kussovski, D. Worle, H. Kisov, M. Belcheva, T. Georgieva and S. Dimitrov, LAT 2010: International Conference on Lasers, Applications, and Technologies, 2010, 7994, 79941A Search PubMed.
- J. P. Lyon, C. M. de Pedroso e Silva Azevedo, L. M. Moreira, C. J. de Lima and M. A. de Resende, Mycopathologia, 2011, 172, 293–297 CrossRef CAS PubMed.
- L. S. Peloi, C. E. G. Biondo, E. Kimura, M. J. Politi, M. V. C. Lonardoni, S. M. A. Aristides, R. C. C. Dorea, N. Hioka and T. G. V. Silveira, Exp. Parasitol., 2011, 128, 353–356 CrossRef CAS PubMed.
- J. Chen, T. C. Cesario and P. M. Rentzepis, J. Phys. Chem. A, 2011, 115, 2702–2707 CrossRef CAS PubMed.
- A. Baptista, I. T. Kato, R. A. Prates, L. C. Suzuki, M. P. Raele, A. Z. Freitas and M. S. Ribeiro, Photochem. Photobiol., 2012, 88, 584–589 CrossRef CAS PubMed.
- J. P. M. L. Rolim, M. A. S. De-Melo, S. F. Guedes, F. B. Albuquerque-Filho, J. R. De Souza, N. A. P. Nogueira, I. C. J. Zanin and L. K. A. Rodrigues, J. Photochem. Photobiol., B, 2012, 106, 40–46 CrossRef CAS PubMed.
- H. Hasegawa, S. Sato, S. Kawauchi, D. Saitoh, N. Shinomiya, H. Ashida and M. Terakawa, Optical Methods for Tumor Treatment and Detection: Mechanisms and Techniques in Photodynamic Therapy XXI, 2012, 8210, 82100V Search PubMed.
- G. B. Rodrigues, L. K. S. Ferreira, M. Wainwright and G. U. L. Braga, J. Photochem. Photobiol., B, 2012, 116, 89–94 CrossRef CAS PubMed.
- X. Cheng, S. Guan, H. Lu, C. Zhao, X. Chen, N. Li, Q. Bai, Y. Tian and Q. Yu, Lasers Surg. Med., 2012, 44, 824–831 CrossRef PubMed.
- E. Steinmann, U. Gravemann, M. Friesland, J. Doerrbecker, T. H. Müller, T. Pietschmann and A. Seltsam, Transfusion, 2013, 53, 1010–1018 CrossRef CAS PubMed.
- A. Hasebe, I. Ishikawa, H. M. Shamsul, M. Ohtani, T. Segawa, A. Saeki, N. Tanizume, M. Oouchi, Y. Okagami, T. Okano and K. I. Shibata, Photomed. Laser Surg., 2013, 31, 125–131 CrossRef CAS PubMed.
- J. P. Lyon, L. M. Moreira, V. S. Dutra de Carvalho, F. V. dos Santos, C. J. de Lima and M. A. de Resende, Mycoses, 2013, 56, 157–161 CrossRef PubMed.
- J. P. Lyon, R. R. Rezende, M. P. Rabelo, C. J. de Lima and L. M. Moreira, Mycopathologia, 2013, 175, 159–164 CrossRef CAS PubMed.
- J. S. C. Monteiro, S. C. P. S. Oliveira, G. M. P. Santos, A. F. S. Miranda, F. J. P. Sampaio, M. F. M. Gesteira, F. A. A. Zainn, M. A. V. Santos and A. L. B. Pinheiro, Mech. Low-Light Therapy VIII, 2013, 8569, 85690R Search PubMed.
- G. B. Rodrigues, M. Dias-Baruffi, N. Holman, M. Wainwright and G. U. L. Braga, Photodiagn. Photodyn. Therapy, 2013, 10, 141–149 CrossRef CAS PubMed.
- L. Pires, S. D. M. G. Bosco, M. S. Baptista and C. Kurachi, PLoS One, 2014, 9, 85431 CrossRef PubMed.
- L. W. Figueiredo Souza, S. V. T. Souza and A. C. C. Botelho, Dermatologic Therapy, 2014, 27, 43–47 CrossRef CAS PubMed.
- K. Matthes De Freitas-Pontes, C. E. De, A. Gomes, B. M. Dias Frota De Carvalho, R. De Sousa, C. Sabóia and B. A. Garcia, J. Prosthet. Dent., 2014, 112, 632–637 CrossRef CAS PubMed.
- M. R. Sbeghen, E. M. Voltarelli, T. G. Campois, E. Kimura, S. M. A. Aristides, L. Hernandes, W. Caetano, N. Hioka, M. V. C. Lonardoni and T. G. V. Silveira, J. Lasers Med. Sci., 2015, 6, 106–111 CrossRef PubMed.
- O. Planas, R. Bresolí-Obach, J. Nos, T. Gallavardin, R. Ruiz-González, M. Agut and S. Nonell, Molecules, 2015, 20, 6284–6298 CrossRef CAS PubMed.
- S. C. Nuñez, T. M. Yoshimura, M. S. Ribeiro, H. C. Junqueira, C. Maciel, M. D. Coutinho-Neto and M. S. Baptista, J. Photochem. Photobiol., B, 2015, 150, 31–37 CrossRef PubMed.
- M. Wainwright, J. Antczak, M. Baca, C. Loughran and K. Meegan, J. Photochem. Photobiol., B, 2015, 150, 38–43 CrossRef CAS PubMed.
- J. P. Tardivo, M. Wainwright and M. Baptista, J. Photochem. Photobiol., B, 2015, 150, 66–68 CrossRef CAS PubMed.
- A. S. Sousa, R. A. Prates, M. E. S. O. de Santi, R. G. Lopes, S. K. Bussadori, L. R. Ferreira and A. M. Deana, Photodiagn. Photodyn. Therapy, 2016, 14, 111–114 CrossRef CAS PubMed.
- M. R. Ronqui, T. M. S. F. De Aguiar Coletti, L. M. De Freitas, E. T. Miranda and C. R. Fontana, J. Photochem. Photobiol., B, 2016, 158, 122–129 CrossRef CAS PubMed.
- L. Gao, S. Jiang, Y. Sun, M. Deng, Q. Wu, M. Li and T. Zeng, Front. Microbiol., 2016, 7, 617 Search PubMed.
- F. P. Sellera, C. P. Sabino, M. S. Ribeiro, R. G. Gargano, N. R. Benites, P. A. Melville and F. C. Pogliani, Photodiagn. Photodyn. Therapy, 2016, 13, 276–281 CrossRef PubMed.
- L. R. Ferreira, A. S. Sousa, L. H. Alvarenga, A. M. Deana, M. E. O. S. de Santi, I. T. Kato, C. R. L. Leal, M. S. Ribeiro and R. A. Prates, Photodiagn. Photodyn. Therapy, 2016, 15, 25–27 CrossRef CAS PubMed.
- A. Baptista, C. P. Sabino, S. C. Núñez, W. Miyakawa, A. A. Martin and M. S. Ribeiro, J. Photochem. Photobiol., B, 2017, 177, 76–84 CrossRef CAS PubMed.
- L. El-Khordagui, N. El-Sayed, S. Galal, H. El-Gowelli, H. Omar and M. Mohamed, Int. J. Pharm., 2017, 520, 139–148 CrossRef CAS PubMed.
- L. C. Suzuki, I. T. Kato, R. A. Prates, C. P. Sabino, T. M. Yoshimura, T. O. Silva and M. S. Ribeiro, Photodiagn. Photodyn. Therapy, 2017, 17, 173–179 CrossRef CAS PubMed.
- M. Michútová, V. Mrázová, M. Kúdelová, M. Smolinská, M. Šupoliková, M. Vrbová and F. Golais, Acta Virologica, 2017, 61, 308–315 CrossRef PubMed.
- C. R. L. Leal, L. H. Alvarenga, T. Oliveira-Silva, I. T. Kato, B. Godoy-Miranda, S. K. Bussadori, M. S. Ribeiro and R. A. Prates, Photodiagn. Photodyn. Therapy, 2017, 19, 1–4 CrossRef CAS PubMed.
- V. Pérez-Laguna, L. Pérez-Artiaga, V. Lampaya-Pérez, S. C. López, I. García-Luque, M. J. Revillo, S. Nonell, Y. Gilaberte and A. Rezusta, Photodiagn. Photodyn. Therapy, 2017, 19, 98–102 CrossRef PubMed.
- J. G. Pinto, J. F. d S. Martins, A. H. C. Pereira, J. Mittmann, L. J. Raniero and J. Ferreira-Strixino, Photodiagn. Photodyn. Therapy, 2017, 18, 325–330 CrossRef CAS PubMed.
- T. M. S. F. De Aguiar Coletti, L. M. De Freitas, A. M. F. Almeida and C. R. Fontana, Photomed. Laser Surg., 2017, 35, 378–385 CrossRef PubMed.
- S. Raquel De Annunzio, L. Marise De Freitas, A. Lígia Blanco, M. Martins Da Costa, C. C. Carmona-Vargas, K. Thiago De Oliveira and C. R. Fontana, J. Photochem. Photobiol., B, 2017, 178, 545–550 CrossRef PubMed.
- O. J. Oppezzo and A. F. Forte Giacobone, Photochem. Photobiol., 2018, 94, 186–189 CrossRef CAS PubMed.
- M. L. Frade, S. R. de Annunzio, G. M. F. Calixto, F. D. Victorelli, M. Chorilli and C. R. Fontana, Molecules, 2018, 23, 473 CrossRef PubMed.
- L. M. De Freitas, E. N. Lorenzón, N. A. Santos-Filho, L. H. D. P. Zago, M. P. Uliana, K. T. De Oliveira, E. M. Cilli and C. R. Fontana, Sci. Rep., 2018, 8, 43–50 CrossRef PubMed.
- D. A. C. Méndez, E. Gutierrez, E. J. Dionísio, T. M. Oliveira, M. A. R. Buzalaf, D. Rios, M. A. A. M. Machado and T. Cruvinel, Lasers Med. Sci., 2018, 33, 479–487 CrossRef PubMed.
- A. H. C. Pereira, J. G. Pinto, M. A. A. Freitas, L. C. Fontana, C. Pacheco Soares and J. Ferreira-Strixino, Photodiagn. Photodyn. Therapy, 2018, 22, 43–50 CrossRef CAS PubMed.
- L. Tonani, N. S. Morosini, H. Dantas de Menezes, M. E. Nadaletto Bonifácio da Silva, M. Wainwright, G. Ú. Leite Braga and M. Regina von Zeska Kress, Fungal Biol., 2018, 122, 436–448 CrossRef CAS PubMed.
- G. A. Da Collina, F. Freire, T. P. D. C. Santos, N. G. Sobrinho, S. Aquino, R. A. Prates, D. D. F. T. Da Silva, A. C. R. Tempestini Horliana and C. Pavani, Photochem. Photobiol. Sci., 2018, 17, 1355–1364 CrossRef CAS PubMed.
- K. Kholikov, S. Ilhom, M. Sajjad, M. E. Smith, J. D. Monroe, O. San and A. O. Er, Photodiagn. Photodyn. Therapy, 2018, 24, 7–14 CrossRef CAS PubMed.
- A. A. Foggiato, D. F. Silva and R. C. F. R. Castro, Photodiagn. Photodyn. Therapy, 2018, 24, 123–128 CrossRef CAS PubMed.
- F. V. Cabral, C. P. Sabino, J. A. Dimmer, I. P. Sauter, M. J. Cortez and M. S. Ribeiro, Photochem. Photobiol., 2019, 96, 13188 Search PubMed.
- C. P. Sabino, M. Wainwright, C. dos Anjos, F. P. Sellera, M. S. Baptista, N. Lincopan and M. S. Ribeiro, Photodiagn. Photodyn. Therapy, 2019, 28, 186–191 CrossRef CAS PubMed.
- M. H. Paziani, L. Tonani, H. D. de Menezes, L. Bachmann, M. Wainwright, G. Ú. L. Braga and M. R. von Zeska Kress, Photodiagn. Photodyn. Therapy, 2019, 25, 197–203 CrossRef CAS PubMed.
- J. Li, M. Zhu, L. An, F. Chen and X. Zhang, Eur. J. Dermatol., 2019, 29, 160–166 CAS.
- S. A. Torres-Hurtado, J. Ramírez-Ramírez, A. C. Larios-Morales, J. C. Ramírez-San-Juan, R. Ramos-García, A. P. Espinosa-Texis and T. Spezzia-Mazzocco, Photodiagn. Photodyn. Therapy, 2019, 26, 203–209 CrossRef CAS PubMed.
- B. Chen, Y. Sun, J. Zhang, R. Chen, X. Zhong, X. Wu, L. Zheng and J. Zhao, Front. Microbiol., 2019, 10, 1228 CrossRef PubMed.
- J. Tan, Z. Liu, Y. Sun, L. Yang and L. Gao, Mycopathologia, 2019, 184, 525–531 CrossRef CAS PubMed.
- T. de Oliveira-Silva, L. H. Alvarenga, C. Lima-Leal, B. Godoy-Miranda, P. Carribeiro, L. C. Suzuki, M. S. Ribeiro, I. Tiemy Kato, C. Pavani and R. A. Prates, Photodiagn. Photodyn. Therapy, 2019, 27, 54–58 CrossRef CAS PubMed.
- M. E. Santi, R. G. Lopes, R. A. Prates, A. Sousa, L. R. Ferreira, A. U. Fernandes, S. K. Bussadori and A. M. Deana, Photonic Therapeutics and Diagnostics XI, 2015, 9303, 93032H Search PubMed.
- L. S. Peloi, R. R. S. Soares, C. E. G. Biondo, V. R. Souza, N. Hioka and E. Kimura, J. Biosci., 2008, 33, 231–237 CrossRef CAS PubMed.
- E. Wanarska and I. Maliszewska, Physicochem. Probl. Miner. Process., 2020, 56, 269–279 CrossRef CAS.
- L. Yuan, P. Lyu, Y.-Y. Huang, N. Du, W. Qi, M. R. Hamblin and Y. Wang, J. Photochem. Photobiol., B, 2020, 203, 111730 CrossRef CAS PubMed.
- M. Hasenleitner and K. Plaetzer, Antibiotics, 2020, 9, 13 CrossRef CAS PubMed.
- O. Gulias, G. McKenzie, M. Bayo, M. Agut and S. Nonell, Antibiotics, 2020, 9, 98 CrossRef CAS PubMed.
- C. C. Magacho, J. G. Pinto, B. M. N. Souza, A. H. C. Pereira and J. Ferreira-Strixino, Photodiagn. Photodyn. Therapy, 2020, 30, 101691 CrossRef PubMed.
- C. P. Sabino, M. Wainwright, M. S. Ribeiro, F. P. Sellera, C. dos Anjos, M. D. Baptista and N. Lincopan, J. Photochem. Photobiol., B, 2020, 208, 111893 CrossRef CAS PubMed.
- C. dos Anjos, C. P. Sabino, F. P. Sellera, F. Esposito, F. C. Pogliani and N. Lincopan, Int. J. Antimicrob. Agents, 2020, 56, 106025 CrossRef CAS PubMed.
- L. H. D. Zago, S. R. de Annunzio, K. T. de Oliveira, P. A. Barbugli, B. R. Valdes, M. Feres and C. R. Fontana, J. Photochem. Photobiol., B, 2020, 209, 111903 CrossRef CAS PubMed.
- L. S. Sampaio, S. R. de Annunzio, L. M. de Freitas, L. O. Dantas, L. de Boni, M. C. Donatoni, K. T. de Oliveira and C. R. Fontana, Photodiagn. Photodyn. Therapy, 2020, 31, 101925 CrossRef CAS PubMed.
- M. Nie, D. M. Deng, Y. F. Wu, K. T. de Oliveira, V. S. Bagnato, W. Crielaard and A. N. D. Rastelli, Photodiagn. Photodyn. Therapy, 2020, 31, 101817 CrossRef CAS PubMed.
- C. C. Li, X. R. Jia, Y. W. Bian, D. Qi and J. B. Wu, Mycoses, 2021, 64, 48–54 CrossRef CAS PubMed.
- X.
X. Shen, L. W. Dong, X. He, C. Q. Zhao, W. Q. Zhang, X. Y. Li and Y. G. Lu, Photodiagn. Photodyn. Therapy, 2020, 32, 102051 CrossRef CAS PubMed.
- G. E. Karaoglu, Z. U. Ydin, D. Erdonmez, C. Gol and M. Durmus, Photodiagn. Photodyn. Therapy, 2020, 32, 102038 CrossRef PubMed.
- A. S. Garcez, M. Kaplan, G. J. Jensen, F. R. Scheidt, E. M. Oliveira and S. S. Suzuki, Photodiagn. Photodyn. Therapy, 2020, 32, 102029 CrossRef CAS PubMed.
- R. R. Sarker, Y. Tsunoi, Y. Haruyama, Y. Ichiki, S. Sato and I. Nishidate, Photochem. Photobiol., 2021, 97, 600–606 CrossRef CAS PubMed.
- J. J. Shen, M. C. Arendrup, G. B. E. Jemec and D. M. L. Saunte, Photodiagn. Photodyn. Therapy, 2021, 33, 102169 CrossRef CAS PubMed.
- C. H. Rodrigues, E. A. G. Araujo, R. P. Almeida, T. P. Nascimento, M. M. Silva, G. Abbas, F. D. Nunes, E. Lins, M. C. B. Lira-Nogueira, J. S. A. Falcao, A. Fontes, A. L. F. Porto, G. Pereira and B. S. Santos, Photodiagn. Photodyn. Therapy, 2021, 34, 102315 CrossRef CAS PubMed.
- A. C. C. da Mota, M. L. L. Goncalves, A. Horliana, A. M. Deana, L. A. D. Cavalcante, A. O. Gomes, M. P. A. Mayer, E. S. A. Suguimoto, K. P. S. Fernandes, R. A. Mesquita-Ferrari, R. A. Prates, L. J. Motta and S. K. Bussadori, Lasers Med. Sci., 2022, 37, 877–886 CrossRef PubMed.
- F. D. Ferreira, N. D. Tebaldi and C. A. de Oliveira, Trop. Plant Pathol., 2021, 46, 559–564 CrossRef.
- M. Perez, P. Robres, B. Moreno, R. Bolea, M. T. Verde, V. Perez-Laguna, C. Aspiroz, Y. Gilaberte and A. Rezusta, Front. Med., 2021, 8, 731 Search PubMed.
- A. M. Alhenaki, F. K. Alqarawi, S. A. Tanveer, F. A. Alshahrani, A. Alshahrani, E. M. AlHamdan, K. M. Alzahrani, N. Aldahiyan, M. Naseem, F. Vohra and T. Abduljabbar, Photodiagn. Photodyn. Therapy, 2021, 35, 102362 CrossRef CAS PubMed.
- M. P. Wylie, R. A. Craig, S. P. Gorman and C. P. McCoy, Int. J. Antimicrob. Agents, 2021, 58, 106360 CrossRef CAS PubMed.
- S. Gnat, D. Lagowski, M. Dylag, J. Zielinski and A. Nowakiewicz, J. Biophotonics, 2021, 14, e202100150 CrossRef CAS PubMed.
- V. Perez-Laguna, Y. Barrena-Lopez, Y. Gilaberte and A. Rezusta, Pharmaceutics, 2021, 13, 1176 CrossRef CAS PubMed.
- R. R. Sarker, Y. Tsunoi, Y. Haruyama, S. Sato and I. Nishidate, J. Biomed. Opt., 2022, 27, 018001 CAS.
- V. Zhukhovitsky, N. Shevlyagina, M. Zubasheva, L. Russu, V. Gushchin, G. Meerovich and M. Strakhovskaya, AMB Express, 2022, 14, 1–9 Search PubMed.
- L. M. A. Figueiredo-Godoi, M. T. Garcia, J. G. Pinto, J. Ferreira-Strixino, E. G. Faustino, L. L. C. Pedroso and J. C. Junqueira, Antibiotics, 2022, 11, 619 CrossRef CAS PubMed.
- I. G. Tiganova, G. A. Meerovich, I. D. Zulufova, E. V. Akhlyustina, R. S. Ovchinnikov, A. I. Solovyev, I. D. Romanishkin, E. I. Kozlikina, A. V. Nekhoroshev, D. A. Glechik, A. V. Parshin, V. D. Parshin, Y. M. Romanova and V. B. Loschenov, Photodiagn. Photodyn. Therapy, 2022, 38, 102753 CrossRef CAS PubMed.
- S. A. B. de Lapena, M. Terra-Garcia, R. A. D. Ward, R. D. Rossoni, V. M. M. Melo and J. C. Junqueira, Photodiagn. Photodyn. Therapy, 2022, 38, 102837 CrossRef CAS PubMed.
- P. H. G. Bariao, L. Tonani, G. T. P. Brancini, E. Nascimento, G. U. L. Braga, M. Wainwright and M. R. V. Kress, Photochem. Photobiol. Sci., 2022, 21, 1807–1818 CrossRef PubMed.
- A. M. H. P. van den Besselaar and A. C. E. Moor, J. Clin. Pathol., 2000, 53, 470–475 CrossRef CAS PubMed.
- K. E. Washburn, R. N. Streeter, J. T. Saliki, T. W. Lehenbauer and M. E. Prado, Small Ruminant Res., 2001, 42, 31–37 CrossRef.
- B. Zeina, J. Greenman, W. M. Purcell and B. Das, Br. J. Dermatol., 2001, 144, 274–278 CrossRef CAS PubMed.
- H. Mohr, J. Knuver-Hopf, U. Gravemann, A. Redecker-Klein and T. H. Muller, Transfusion, 2004, 44, 886–890 CrossRef CAS PubMed.
- S. K. Bisland, F. N. Dadani, C. Chien and B. C. Wilson, Optical Methods for Tumor Treatment and Detection: Mechanisms and Techniques in Photodynamic Therapy XVI, 2007, 6427, 64270F Search PubMed.
- S. Sabbahi, Z. Alouini, M. Jemli and A. Boudabbous, Water Sci. Technol., 2008, 58, 1047–1054 CrossRef CAS PubMed.
- C. Vieira, A. T. P. C. Gomes, M. Q. Mesquita, N. M. M. Moura, G. P. M. S. Neves, A. F. Faustino and A. Almeida, Front. Microbiol., 2018, 9, 2665 CrossRef PubMed.
- L. M. Tokubo, P. L. Rosalen, J. de Cássia Orlandi Sardi, I. A. Freires, M. Fujimaki, J. E. Umeda, P. M. Barbosa, G. O. Tecchio, N. Hioka, C. F. de Freitas and R. S. Suga Terada, Photodiagn. Photodyn. Therapy, 2018, 23, 94–98 CrossRef CAS PubMed.
- L. Huang, W. Xuan, A. Zadlo, A. Kozinska, T. Sarna and M. R. Hamblin, J. Biophotonics, 2018, 11, e201800029 CrossRef PubMed.
- S. Sabbahi, L. Ben Ayed and A. Boudabbous, J. Water Health, 2013, 11, 590–599 CrossRef CAS PubMed.
- E. J. Kim, J. H. Choi, H. J. Yang, S. S. Choi, H. K. Lee, Y. C. Cho, H. K. Kim, S. W. Kim and H. S. Chae, Photodiagn. Photodyn. Therapy, 2019, 26, 111–115 CrossRef CAS PubMed.
- R. A. Prates, M. R. Hamblin, I. T. Kato, B. Fuchs, E. Mylonakis, M. S. Ribeiro and G. Tegos, Mech. Low-Light Therapy VI, 2011, 7887, 788709 Search PubMed.
- A. Galstyan and U. Dobrindt, J. Photochem. Photobiol., B, 2019, 197, 111554 CrossRef CAS PubMed.
- T. Mito, T. Suzuki, T. Kobayashi, X. Zheng, Y. Hayashi, A. Shiraishi and Y. Ohashi, Invest. Ophthalmol. Visual Sci., 2012, 53, 6305–6313 CrossRef CAS PubMed.
- S. Mitra, C. G. Haidaris, S. B. Snell, B. R. Giesselman, S. M. Hupcher and T. H. Foster, Lasers
Surg. Med., 2011, 43, 324–332 CrossRef PubMed.
- P. Soria-Lozano, Y. Gilaberte, M. Paz-Cristobal, L. Pérez-Artiaga, V. Lampaya-Pérez, J. Aporta, V. Pérez-Laguna, I. García-Luque, M. Revillo and A. Rezusta, BMC Microbiol., 2015, 15, 187 CrossRef CAS PubMed.
- S. Sabbahi, Z. Alouini, L. B. Ayed, M. Jemli and A. Boudabbous, Desalin. Water Treat., 2010, 20, 209–219 CrossRef CAS.
- F. Nakonechny, M. A. Firer, Y. Nitzan and M. Nisnevitch, Photochem. Photobiol., 2010, 86, 1350–1355 CrossRef CAS PubMed.
- C. M. Cassidy, R. F. Donnelly and M. M. Tunney, Photodyn. Ther., 2009, 7380, 73804O Search PubMed.
- M. Tanaka, P. Mroz, T. Dai, L. Huang, Y. Morimoto, M. Kinoshita, Y. Yoshihara, N. Shinomiya, S. Seki, K. Nemoto and M. R. Hamblin, Photochem. Photobiol., 2013, 89, 679–682 CrossRef CAS PubMed.
- E. Špitalská, Z. Špitalský, Z. Markovic, K. Štefanidesová and Ľ. Škultéty, Photodiagn. Photodyn. Therapy, 2018, 24, 150–152 CrossRef PubMed.
- Z. S. Silva, Y. Y. Huang, L. F. De Freitas, C. M. França, S. B. Botta, P. A. Ana, R. A. Mesquita-Ferrari, K. P. Santos Fernandes, A. Deana, C. R. Lima Leal, R. A. Prates, M. R. Hamblin and S. K. Bussadori, Sci. Rep., 2016, 6, 33270 CrossRef PubMed.
- C. M. Cassidy, R. F. Donnelly, J. S. Elborn, N. D. Magee and M. M. Tunney, J. Photochem. Photobiol., B, 2012, 106, 95–100 CrossRef CAS PubMed.
- M. Tanaka, P. Mroz, T. Dai, M. Kinoshita, Y. Morimoto and M. R. Hamblin, Biophotonics and Immune Responses VII, 2012, 8224, 822403 Search PubMed.
- A. Shrestha and A. Kishen, Lasers Dentistry XIX, 2013, 8566, 85660G Search PubMed.
- M. Singh, K. Pakshirajan and V. Trivedi, 3 Biotechnol., 2016, 6, 176 Search PubMed.
- M. G. Tosato, P. L. Schilardi, M. F. L. de Mele, A. H. Thomas, A. Miñán and C. Lorente, Int. J. Antimicrob. Agents, 2018, 51, 255–259 CrossRef CAS PubMed.
- Y. Y. Huang, P. J. Rajda, G. Szewczyk, B. Bhayana, L. Y. Chiang, T. Sarna and M. R. Hamblin, Photochem. Photobiol. Sci., 2019, 18, 505–515 CrossRef CAS PubMed.
- S. S. Choi, H. K. Lee and H. S. Chae, Photodiagn. Photodyn. Therapy, 2014, 11, 526–532 CrossRef CAS PubMed.
- X. Dong, A. E. Bond, N. Pan, M. Coleman, Y. Tang, Y. P. Sun and L. Yang, Int. J. Nanomed., 2018, 13, 8025–8035 CrossRef CAS PubMed.
- F. Cieplik, A. Pummer, J. Regensburger, K. A. Hiller, A. Späth, L. Tabenski, W. Buchalla and T. Maisch, Front. Microbiol., 2015, 6, 706 Search PubMed.
- Y. Zhao, J. W. Ying, Q. Sun, M. R. Ke, B. Y. Zheng and J. D. Huang, Dyes Pigm., 2020, 172, 107834 CrossRef CAS.
- M. G. Tosato, P. Schilardi, M. F. L. de Mele, A. H. Thomas, C. Lorente and A. Minan, Heliyon, 2020, 6, e03522 CrossRef CAS PubMed.
- P. Dharmaratne, R. C. H. Wong, J. Wang, P. C. Lo, B. Y. Wang, B. L. Chan, K. M. Lau, C. B. S. Lau, K. P. Fung, M. Ip and D. K. P. Ng, Biomedicines, 2020, 8, 140 CrossRef CAS PubMed.
- Y. A. Anane, T. Apalata, S. Vasaikar, G. E. Okuthe and S. P. Songca, Photodiagn. Photodyn. Therapy, 2020, 30, 101752 CrossRef CAS PubMed.
- P. Dharmaratne, L. G. Yu, R. C. H. Wong, B. C. L. Chan, K. M. Lau, B. Y. Wang, C. Bik-San Lau, K. P. Fung, D. K. P. Ng and M. Ip, Curr. Med. Chem., 2021, 28, 4283–4294 CrossRef CAS PubMed.
- B. L. Chan, P. Dharmaratne, B. Y. Wang, K. M. Lau, C. C. Lee, D. W. S. Cheung, J. Y. W. Chan, G. G. L. Yue, C. B. S. Lau, C. K. Wong, K. P. Fung and M. Ip, Pharmaceutics, 2021, 13, 1399 CrossRef CAS PubMed.
- T. N. Demidova and M. R. Hamblin, Appl. Environ. Microbiol., 2005, 71, 6918–6925 CrossRef CAS PubMed.
- E. Feese and R. A. Ghiladi, J. Antimicrob. Chemother., 2009, 64, 782–785 CrossRef CAS PubMed.
- X. Ragàs, T. Dai, G. P. Tegos, M. Agut, S. Nonell and M. R. Hamblin, Lasers Surg. Med., 2010, 42, 384–390 CrossRef PubMed.
- A. Kishen, M. Upadya, G. P. Tegos and M. R. Hamblin, Photochem. Photobiol., 2010, 86, 1343–1349 CrossRef CAS PubMed.
- T. Dai, V. J. Bil De Arce, G. P. Tegos and M. R. Hamblin, Antimicrob. Agents Chemother., 2011, 55, 5710–5717 CrossRef CAS PubMed.
- J. Chibebe Junior, C. P. Sabino, X. Tan, J. C. Junqueira, Y. Wang, B. B. Fuchs, A. O. Jorge, G. P. Tegos, M. R. Hamblin and E. Mylonakis, BMC Microbiol., 2013, 13, 217 CrossRef PubMed.
- A. Gollmer, A. Felgenträger, W. Bäumler, T. Maisch and A. Späth, Photochem. Photobiol. Sci., 2015, 14, 335–351 CrossRef CAS PubMed.
- A. Rineh, N. K. Dolla, A. R. Ball, M. Magana, J. B. Bremner, M. R. Hamblin, G. P. Tegos and M. J. Kelso, ACS Infect. Dis., 2017, 3, 756–766 CrossRef CAS PubMed.
- C. Songsantiphap, J. Vanichanan, T. Chatsuwan, P. Asawanonda and E. Boontaveeyuwat, Front. Cell. Infection Microbiol., 2022, 12, 929242 CrossRef CAS PubMed.
- H. D. De Menezes, G. B. Rodrigues, S. De, P. Teixeira, N. S. Massola, L. Bachmann, M. Wainwright and G. U. L. Braga, Appl. Environ. Microbiol., 2014, 80, 1623–1632 CrossRef PubMed.
- J. C. Gonzales, G. T. P. Brancini, G. B. Rodrigues, G. J. Silva-Junior, L. Bachmann, M. Wainwright and G. Ú. L. Braga, J. Photochem. Photobiol., B, 2017, 176, 54–61 CrossRef CAS PubMed.
- J. S. Cardoso-Rurr, J. P. Paiva, I. G. Paulino-Lima, T. A. M. Alencar, C. A. S. Lage and A. C. Leitão, Photochem. Photobiol., 2019, 95, 618–626 CrossRef CAS PubMed.
- M. Nagasawa, S. Sato, S. Kawauchi, N. Shinomiya, D. Saito, H. Ashida and M. Obara, Optical Methods for Tumor Treatment and Detection: Mechanisms and Techniques in Photodynamic Therapy XIII, 2004, 5315, 61 CAS.
- S. S. Choi, H. K. Lee and H. S. Chae, J. Photochem. Photobiol., B, 2010, 101, 206–209 CrossRef CAS PubMed.
- R. De Carvalho Goulart, G. Thedei, S. L. S. Souza, A. Cláudio Tedesco and P. Ciancaglini, Photomed. Laser Surg., 2010, 28, S85–90 CrossRef PubMed.
- J. S. Guffey, W. Payne and W. Roegge, Mycoses, 2017, 60, 723–727 CrossRef CAS PubMed.
- D. Pereira De Lima Carvalho, J. Guerra Pinto, C. Di Paula Costa Sorge, F. R. Rodrigues Benedito, S. Khouri and J. Ferreira Strixino, Lasers Med. Sci., 2014, 29, 113–120 CrossRef PubMed.
-
W. M. M. Philip and H. Howard, Handbook of Physical Properties of Organic Chemicals, CRS Press, Boca Raton, USA, 1996 Search PubMed.
- C. S. Lobo, P. R. Santos, D. Pereira, J. Núñez, J. C. D. Trêpa, D. L. Sousa, J. V. Lourenço, M. F. Coelho, L. P. D. Almeida, J. S. D. Cunha and L. G. Arnaut, Photochem. Photobiol. Sci., 2022, 21, 1101–1109 CrossRef CAS PubMed.
- L. Pires, B. C. Wilson, R. Bremner, A. Lang, J. Larouche, R. McDonald, J. D. Pearson, D. Trcka, J. Wrana, J. Wu and C. M. Whyne, Sci. Rep., 2022, 12, 14438 CrossRef CAS PubMed.
|
This journal is © The Royal Society of Chemistry 2023 |
Click here to see how this site uses Cookies. View our privacy policy here.