DOI:
10.1039/D2FO01909D
(Paper)
Food Funct., 2023,
14, 206-214
Quercetin inhibits hepatotoxic effects by reducing trimethylamine-N-oxide formation in C57BL/6J mice fed with a high L-carnitine diet
Received
4th July 2022
, Accepted 17th November 2022
First published on 18th November 2022
Abstract
L-Carnitine can be metabolized to trimethylamine (TMA) by gut microbiota and further converted into trimethylamine N-oxide (TMAO) in the liver, leading to liver damage. This study aimed to investigate the protective effect of quercetin against high L-carnitine-induced liver toxicity in mice. 3% L-carnitine drinking water was used to feed mice in this study. The formation of TMAO in the blood circulation of the tested mice was down-regulated following quercetin treatment. Administration of quercetin could also effectively antagonize the liver injury caused by high L-carnitine intake, which was proved by the decreased serum AST and ALT activities and the reduced levels of inflammatory liver cytokines (IL-1, IL-6, TNF-α, and TNF-β). Moreover, quercetin exhibited a rebalancing effect on dyslipidemia (TC, TG, HDL, and LDL) and antioxidant abilities (SOD, GSH-Px, MDA, and RAHFR) in L-carnitine-treated mice. The results of hepatic H&E and Oil Red O staining further verified the liver injury of high L-carnitine-treated mice and the protective effects of quercetin. These findings suggested that quercetin could attenuate the hepatotoxic effects of the mice fed with a high L-carnitine diet via inhibiting the circulating TMAO formation.
Introduction
L-Carnitine is known as vitamin BT, which plays a crucial role in lipid and methyl-donor metabolism.1L-Carnitine is an amino-acid derivative that transports the chains of fatty acids into the mitochondrial matrix, thus allowing the cells to break down fat and get energy from the stored fat reserves, and is an essential internal nutrient for fat metabolism in the body.1 It is widely found in foods, most notably in animal source foods such as red meat. With the improvement of living quality, the acceptable daily intake of red meat has increased in recent years. L-Carnitine has gained popularity in Europe, the United States, Japan, and other countries as a dietary supplement.2,3 L.O. Dragsted's report showed that with the increase in meat consumption in recent years, the intake of L-carnitine, which is rich in meat, has significantly increased.4,5 As a result, research related to L-carnitine has gained more recognition and attention.
Numerous findings indicated that L-carnitine could be metabolized to trimethylamine (TMA) by intestinal microbiota in the colon and further converted to trimethylamine N-oxide (TMAO) in the liver. TMAO has been proven to be a risk factor for cardiovascular disease (CVD), chronic kidney disease (CKD), and metabolic syndrome.5–7 Our previous studies showed that high ingestion of L-carnitine could also induce liver injury,8 where the serum ALT and AST activities in high L-carnitine-treated mice were significantly higher than that of the chow group. At the same time, ingestion of 3% L-carnitine in drinking water induced inflammatory reactions as evidenced by a dramatic increase in the hepatic IL-1, IL-6, TNF-α, and TNF-β levels and abnormal alteration in the hepatic MDA, RAHFR, T-SOD, GSH-Px, TC, TG, LDL-C, and HDL-C levels, which was consistent with other research studies.9,10 Liver damage induced by high L-carnitine ingestion is a very serious problem, and therefore, it is necessary to further investigate the TMAO-mediated hepatotoxic mechanisms for developing safe and active ingredients that can prevent these damages.
Quercetin and its derivatives widely exist in Chinese herbs, fruits, vegetables, and other plant-based foods, which have been shown to possess anti-inflammatory ability, antioxidant activities, and antitumor effects.11–14 Moreover, quercetin can relieve liver injury induced by the ingestion of carbon tetrachloride, alcohol, and endotoxins.11–14 However, it is still unknown whether quercetin might relieve the liver injury induced by high L-carnitine ingestion. In this regard, it is necessary to investigate whether quercetin can antagonize liver injury caused by L-carnitine, and the intervention of quercetin may provide some new theoretical bases for preventing hepatic toxicity under L-carnitine consumption.
Preliminary experiments (1%, 1.5%, 2%, 2.5%, and 3% of L-carnitine drinking water) were carried out, and the results showed that 1% and 1.5% L-carnitine drinking water were safe, 2% and 2.5% L-carnitine drinking water induced mild liver injury, and 3% L-carnitine induced liver injury, which was proved by the increase in the serum AST and ALT activities, production of inflammatory liver cytokines (IL-1, IL-6, TNF-α, and TNF-β), lipid metabolism (TC, TG, HDL, and LDL) disorder, and the decline in antioxidant ability (SOD, GSH-Px, MDA and RAHFR). Based on our previous results and the reports by Wang et al.5 and Koeth et al.,6 3% L-carnitine drinking water was used to feed mice for 12 weeks. For this reason, the present animal experiments were designed to evaluate the hepatoprotective effect of quercetin by examining the liver function and the TMAO formation in the systemic circulation of L-carnitine-induced mice. These findings may help us preferably understand the protective effect of quercetin and its important implications in the treatment of liver injury caused by L-carnitine consumption.
Materials and methods
Materials and chemicals
Food grade L-carnitine was obtained from Ya-xixi Co., Ltd (Beijing, China). Quercetin (the purity ≥97%) was purchased from Shanghai Yuanye Biotechnology Co., Ltd (Shanghai, China). Haematoxylin and eosin (H&E) and oil red O were bought from Shanghai Lanji Technological Development Co. Ltd (Shanghai, China). The assay kits of alanine aminotransferase (ALT), aspartate aminotransferase (AST), total cholesterol (TC), total triglyceride (TG), high density lipoprotein-cholesterol (HDL-C), and low density lipoprotein-cholesterol (LDL-C) were purchased from Changchun Huili Biotechnology Co., Ltd (Changchun, China). The detection kits for superoxide dismutase (T-SOD), glutathione peroxidase (GSH-Px), and malonaldehyde (MDA) were the products of Nanjing Jiancheng Bioengineering Institute (Nanjing, China). ELISA kits of interleukin 1 (IL-1), interleukin 6 (IL-6), tumor necrosis factor-α (TNF-α), and tumor necrosis factor-β (TNF-β) were also purchased from Nanjing Jiancheng Bioengineering Institute (Nanjing, China). Deionized water was prepared using a Millipore Milli Q-Plus system (Millipore, Bedford, MA, USA). Acetonitrile and methanol were of chromatographic grade purchased from Kemiou Chemical Reagent Co., Ltd (Tianjin, China). All other reagents and chemicals were analytical grade.
Animal experiment and sample collection
40 healthy male C57BL/6J mice, 18–22 g weight, were purchased from the Experimental Animal Center of the Air Force Medical University (Xi'an, China). Mice were housed at a temperature range from 20 °C to 25 °C and a humidity range from 50% to 60%. They were allowed access to rodent chow (40% corn flour, 26% wheat flour, 10% fish meal, 10% bran, 10% bean cake, 2% mineral, 1% coarse, and 1% vitamin complex, Qianmin Feed Factory). All animal studies were performed under the approval of the Committee on Care and Use of Laboratory Animals of the Air Force Medical University (SYXK-007-2007), and the experimental procedure was carried out according to the Guideline of Experimental Animal Administration published by the State Committee of Science and Technology of People's Republic of China. Before the formal experiments, mice were placed in an animal laboratory for adaptive feeding for a week with free access to a chow diet and water. After one week, the mice were divided into 5 groups, with 8 mice in each group. The groups were the control group (chow diet), high L-carnitine (3% of L-carnitine diet) model group, and three quercetin intervention groups. The high L-carnitine model group and the three quercetin intervention groups were given 3% of L-carnitine in drinking water. The intervention groups were further divided into high, medium, and low dose groups. At the same time, 0.4 mL of quercetin aqueous solution with low, medium, and high dosages (150, 300, and 450 mg kg−1) were respectively given to the three groups once a day. The low, medium, and high dose groups were Que 150, Que 300, and Que 450 groups, respectively. Similarly, the mice in the chow and high L-carnitine model group were given 0.4 mL of normal saline every day. After 12 weeks of feeding, mice were fasted for 12 h and weighed, and then mice were killed as anesthetized with isoflurane. The urine, serum, and liver samples were collected, and then the blood samples were centrifuged at 3000g for 10 min. Ultimately, all of the samples from the mice were stored at −80 °C for further analysis.
Histopathological observation of liver tissues
After dissecting the mice, a square liver slice with a volume of about 0.25 cm2 was cut from the left lobe of the liver and fixed in 4% paraformaldehyde solution immediately. The liver slices were dehydrated with gradient ethanol solution and then paraffin-embedded to make 6 μm thick slices, followed by dyeing with hematoxylin and eosin (H&E).15 For Oil Red O staining, frozen liver samples were processed using a cryostat and then fixed and stained.16 Liver sections were observed, and histopathological analysis was performed by using a fully automatic microscope.
Determination of TMAO in the serum and the urine
The quantitative analysis of TMAO was achieved within 6 min by using Agilent 6460C UPLC-MS/MS with 10% methyl alcohol isocratic elution. Agilent 1260 Infinity II HILIC column was used for chromatographic separation. The flow rate was 0.2 mL min−1, and the column temperature was maintained at 30 °C. Mobile phase A was 40 mmol L−1 ammonium formate aqueous solution, and mobile phase B was methanol. The best MRM conditions, including precursor ion, product ion, retention time, collision cell energies, and fragmentation voltages used for each tested compound and internal standard, have been published in our previous study.17 76.2 > 59.2 amu (TMAO), 85.2 > 68.2 amu (d9-TMAO) were the optimum qualitative and quantitative conditions. The method correlation coefficient was 0.99978293, and the detection limit was 0.121 μg L−1. The other details of sample collection, pretreatment, and determination referred to our previous research.18
Analysis of serum and liver biochemical indexes
The serum AST and ALT activities, and the liver TG, TC, LDL-C, HDL-C, MDA, SOD, GSH-Px, RAHFR, IL-1, IL-6, TNF-α, and TNF-β levels were measured with the appropriate commercial diagnostic kits. 0.50 g of liver tissue was placed in a 5 mL centrifuge tube, and then 4.5 mL normal saline was added and homogenized with a homogenizer (F6/10-10G homogenizer, Fluke Fluid Machinery Manufacturing Co., LTD). The supernatant was centrifuged at a speed of 3000g for 10 min and stored at −80 °C for later use. The specific detection process was carried out following the kit instructions.
Statistical analysis
Prism 8.0.1 was used for statistical analysis, and data were analyzed by the one-way analysis of variance (one-way ANOVA) method, which was expressed by mean ± standard deviation (SD). The post-hoc analysis was also carried out by using the Tukey test. The p value less than 0.05 was considered as statistically significant, and the p value less than 0.01 was considered very significantly different.
Results
Effects of quercetin on body weight and liver weight of L-carnitine-treated mice
Since liver injury is closely related to the levels of body weight and liver weight, we aimed to study the impact of L-carnitine with or without quercetin on those parameters. Additionally, hepatosomatic index (HI) was calculated by dividing liver weight (g) by body weight (g) and multiplying by 100%.19 Previous studies showed that high ingestion of L-carnitine could induce an increase in liver weight, body weight, and HI.6–8 As shown in Table 1, to study the antagonistic effect of quercetin on hepatotoxicity in high L-carnitine-fed mice, the body weight, liver weight, and HI of the tested mice were observed after 12 weeks of feeding. There were no significant differences in the ingestion of food and water among all the groups before experiments, and the food intake also did not show a significant difference between these groups throughout the whole experimental period. The body weight, liver weight, and HI of the mice fed with high L-carnitine were higher than those of the normal group (chow) after 12 weeks (p < 0.05). Interestingly, the administration of quercetin improved the body weight and liver weight abnormities induced by high L-carnitine feeding. Particularly, high doses of quercetin at 450 mg per kg bw (Que 450) significantly decreased the incremental body weight, liver weight, and liver body index by comparison with L-carnitine-fed mice (p < 0.05). Administration of quercetin at a low dose (Que 150) and medium dose (Que 300) had a certain regulatory effect on these indexes, but there were no statistical differences in these parameters, respectively. These results indicated that a high dosage of flavonoid quercetin could alleviate the L-carnitine-induced body or liver overweight in C57BL/6J mice.
Table 1 Body weight, liver weight, and hepatosomatic index of mice
Relevant parameter |
Groups |
Chow |
Carnitine |
Quercetin 150 mg kg−1 |
Quercetin 300 mg kg−1 |
Quercetin 450 mg kg−1 |
Hepatosomatic index: hepatosomatic index = liver wt (g)/final body wt (g) × 100%. Data are expressed as the mean ± SD (n = 8 mice in each group), different letters indicate significant differences. |
Initial body weight (g) |
31.21 |
31.96 |
31.87 |
32.19 |
30.97 |
Final body weight (g) |
41.98 ± 2.50a |
44.72 ± 2.58b |
43.66 ± 3.79b |
42.35 ± 4.68b |
39.94 ± 2.01a |
Liver weight (g) |
1.82 ± 0.18a |
2.43 ± 0.26b |
2.06 ± 0.19c |
1.98 ± 0.20a |
1.75 ± 0.17 a |
Hepatosomatic index (%) |
4.46 ± 0.09a |
4.69 ± 0.12b |
4.68 ± 0.13b |
4.72 ± 0.08b |
4.51 ± 0.10a |
Quercetin decreased the TMAO levels in systemic blood circulation
To investigate whether the protective effect of quercetin on high L-carnitine-fed mice was related to the TMAO levels in systemic circulation, the serum and urinary TMAO levels of mice were detected by using Agilent 6460C UPLC-MS/MS in this study. As shown in Fig. 1A and B, the serum TMAO level in the high L-carnitine group was 231.1 μg L−1, which was significantly higher than the untreated control mice (84.1 μg L−1, p < 0.01). However, this abnormal enhancement of the serum TMAO was markedly reduced with the administration of quercetin at low, medium, and high dosages (150, 300, and 450 mg per kg bw), which were decreased by 18.6%, 35.5%, and 48.7%, respectively (p > 0.05, p < 0.05, p < 0.01) in comparison with high L-carnitine group of mice. Similarly, high L-carnitine intake significantly enhanced the urinary TMAO levels in the model group compared to that in the chow control mice (p < 0.01), whereas the urinary TMAO contents of the mice treated with low, medium, and high dosages of quercetin were decreased by 42.5%, 68.7%, and 85.3%, respectively (p < 0.05, p < 0.01, p < 0.01 vs. model group). These results demonstrated that quercetin intervention could dose-dependently down-regulate the serum and urinary levels of TMAO in the systemic circulation of high L-carnitine treated mice.
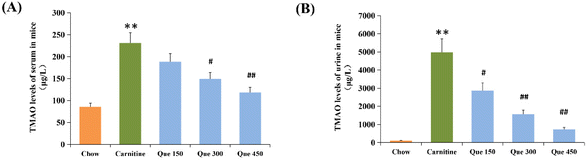 |
| Fig. 1 Quantitation of trimethylamine N-oxide (TMAO) in the serum (A) and urine (B) of C57BL/6J mice. Data are presented as the mean ± standard deviation (SD), n = 8 mice in each group. *p < 0.05, **p < 0.01 (vs. chow group), #p < 0.05, ##p < 0.01 (vs. carnitine group). | |
Effects of quercetin on liver aminotransferase activities and inflammation
Liver damage is often associated with hypohepatia and inflammatory reactions. As shown in Fig. 2A and B, the enzymatic activities of the serum AST and ALT in L-carnitine treated mice were significantly increased from 121.67 and 78.38 U L−1 to 131.75 and 87.37 U L−1 in comparison with the untreated chow mice, respectively (p < 0.01). As expected, administration of quercetin ameliorated the high L-carnitine-induced elevations in AST and ALT activities of mice, where quercetin at 300 mg per kg bw and 450 mg per kg bw dramatically inhibited the L-carnitine-caused enhancement in the serum ALT and AST activities in comparison with high L-carnitine treated mice, respectively (p < 0.01).
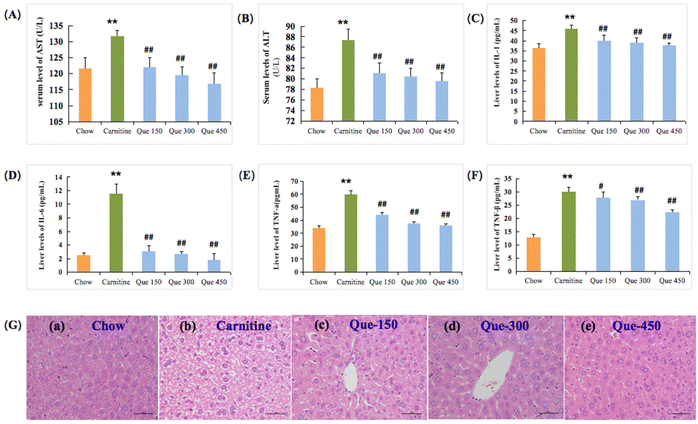 |
| Fig. 2 Effects of quercetin on the transaminase activities and inflammatory cytokines levels of the liver in high L-carnitine treated mice. (A) Serum AST activity. (B) Serum ALT activity. (C–F) Hepatic inflammatory factors IL-1, IL-6, TNF-α, and TNF-β. (G) Liver histopathological staining with H&E, and graphs were observed at 200×. Data are presented as the mean ± standard deviation (SD), n = 8 mice in each group. *p < 0.05, **p < 0.01 (vs. chow group), #p < 0.05, ##p < 0.01 (vs. carnitine group). | |
Liver injury was closely related to inflammation with the elevations of the liver IL-1, IL-6, TNF-α, and TNF-β.18,19 As shown in Fig. 2C–F, the L-carnitine-treated mice exhibited obvious elevation in the hepatic IL-1, IL-6, and TNF-α levels when compared to the chow feeding mice, respectively (p < 0.01), as well as the TNF-β increase in the liver tissues (p < 0.01). Nevertheless, this serious inflammatory response caused by high L-carnitine could be effectively prevented with quercetin treatment. The level of IL-1 in the liver was markedly decreased when the L-carnitine feeding mice were treated with 300 and 450 mg per kg bw of quercetin, respectively (p < 0.01, Fig. 2C), and the concentrations of IL-6 in intervention groups (Que 150, Que 300, and Que 450) were also down-regulated in comparison with L-carnitine treated mice, respectively (p < 0.01, Fig. 2D), suggesting that quercetin has a stronger inhibitory effect on IL-6 than IL-1. Furthermore, as compared to the mice fed with 3% L-carnitine, quercetin treatments at medium or high dosages also inhibited the liver TNF-α formation, respectively (p < 0.01, Fig. 2E). Similarly, TNF-β levels were also reduced following the treatment (p < 0.01, Fig. 2F).
To further confirm the protective effect of quercetin against the L-carnitine-caused hepatotoxicity in C57BL/6J mice, the histological observation of the liver tissues through H&E stain was performed at the termination of the treatment period (12 weeks). As displayed in Fig. 2G, the high L-carnitine intake caused histopathological changes associated with hepatic tissue degeneration, including cavitation, cell membrane, and nuclear fragmentation, as well as ballooning degeneration within the liver cells in comparison with the chow group. Whereas this status of degeneration in the liver was obviously alleviated through the treatment of quercetin in contrast to the high L-carnitine mice, especially for the Que 450 group of the tested mice showing obvious improvement of hepatic tissue pathological abnormality, and the vacuolated cytoplasm was also improved. These findings simultaneously indicated that the appropriate implementation of quercetin was effective in preventing L-carnitine-caused liver toxicity.
Effects of quercetin on antioxidant abilities in the liver of mice
Inflammatory responses are usually accompanied by oxidative stress damage in the hepatic tissues.20,21 To estimate oxidative stress injury, MDA, T-SOD, GSH-Px, and the restraining ability to hydroxyl free radical (RAHFR) of the liver tissues were evaluated. As shown in Fig. 3A–D, the MDA levels within the liver tissues of L-carnitine-treated mice were significantly higher than that of the chow group (p < 0.01), while the MDA formation was dramatically inhibited by the intervention of quercetin in which a significant decrease could be observed after treatment of mice with medium and high doses of quercetin when compared to the L-carnitine model mice, separately (p < 0.01, Fig. 3A). L-Carnitine feeding led to a marked decrease (p < 0.01) in the liver T-SOD activity in contrast with the mice fed with control chow. Interestingly, the decreased T-SOD activity was enhanced by the administration of quercetin at 150, 300, and 450 mg per kg bw, respectively (p < 0.01), suggesting that quercetin could dose-dependently increase the antioxidant ability (Fig. 3B). Herein, the levels of GSH-Px and RAHFR of the liver in high L-carnitine mice were also reduced when making a comparison between the L-carnitine model group and the chow group, respectively (p < 0.01, Fig. 3C and D). However, treatment with different doses of quercetin at 150, 300, and 450 mg per kg bw markedly enhanced the levels of both GSH-Px and RAHFR, respectively (p < 0.01), as compared to the L-carnitine model mice.
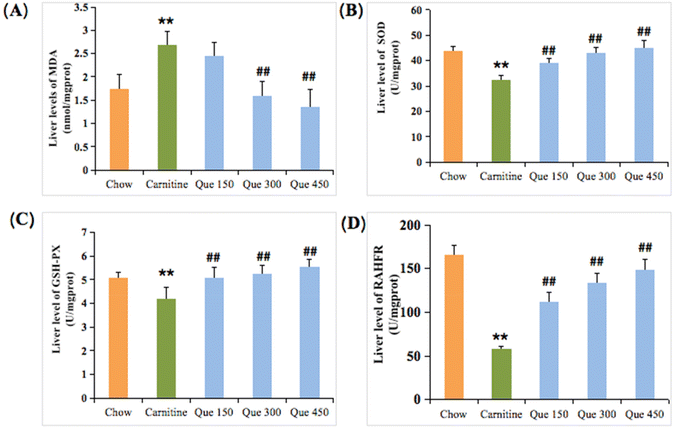 |
| Fig. 3 Effects of quercetin on the antioxidant capacity of the liver in high L-carnitine treated mice. (A) Malonaldehyde, MDA. (B) Superoxide dimutase, SOD. (C) Glutathione peroxidase, GSH-Px. (D) Restraining ability to hydroxyl free radical, RAHFR. Data are presented as the mean ± standard deviation (SD), n = 8 mice in each group. *p < 0.05, **p < 0.01 (vs. chow group), #p < 0.05, ##p < 0.01 (vs. carnitine group). | |
Quercetin improved abnormal lipid levels in liver
Hepatic lipid metabolism disorder is an important manifestation and reason for liver injury or hepatic steatosis, which can exacerbate chronic liver damage.19,22 Herein, it was found that continuous 12-weeks intake of L-carnitine in mice led to significant changes in hepatic TC, TG, and LDL-C levels, respectively (p < 0.01, Fig. 4), relative to those of the chow control group. But the HDL levels have no significant difference with the L-carnitine group (p > 0.05). Surprisingly, most lipid-related parameters were significantly ameliorated after the intervention of quercetin with appropriate dosages. The liver TC levels were significantly improved with the treatment of a high dose of quercetin, respectively (p < 0.01), while the other two interventions at lower-dose dosages did not exhibit significant differences. The liver HDL-C levels did not exhibit a significant difference (p > 0.05). Meanwhile, administration of quercetin at high dosages markedly inhibited the substantial elevation of hepatic TG and LDL-C contents, respectively (p < 0.01). The parallel phenomenon had also been revealed in liver histopathology with oil red O staining (Fig. 4E). The oil red O staining results indicated the apparent fat accumulation that emerged in the liver tissue of L-carnitine-treated mice in comparison with the chow mice. Nevertheless, this hepatic damage status of lipid accumulation in quercetin-treated mice was also decreased in a dose-dependent manner. These findings simultaneously suggested that quercetin had effective protective effects against chronic hepatic injury induced by the intake of 3% of L-carnitine drinking water in C57BL/6J mice.
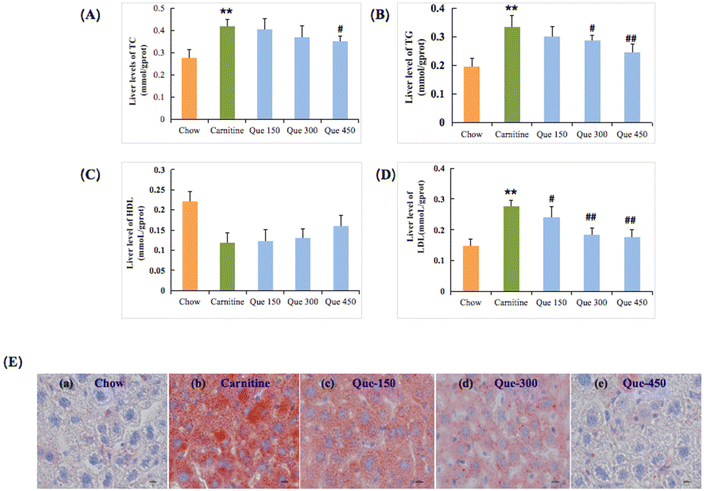 |
| Fig. 4 Effects of quercetin on the lipid homeostasis of the liver in high L-carnitine-fed mice. (A) Total cholesterol, TC. (B) Total triglyceride, TG. (C) High-density lipoprotein-cholesterol, HDL-C, and (D) low-density lipoprotein-cholesterol, LDL-C. (E) Liver histopathological staining with Oil Red, and graphs were observed at 200×. Data are presented as the mean ± standard deviation (SD), n = 8 mice in each group. *p < 0.05, **p < 0.01 (vs. chow group), #p < 0.05, (vs. carnitine group). | |
Discussion
Recent findings have specified that L-carnitine, which is a classic dietary quaternary amine nutrient mainly from red meat, can be metabolized to trimethylamine (TMA) by gut microbiota and subsequently oxidized to trimethylamine-N-oxide (TMAO) by hepatic flavin monooxygenases (FMOs).5,6 It has become clear that the nutrient L-carnitine does not itself cause damages such as atherosclerosis, but its gut microbiota-dependent metabolite TMAO is the cause of the health hazard, and TMAO has been proven to be a risk factor for cardiovascular disease (CVD), chronic kidney disease (CKD), metabolic syndrome, and some other diseases.5,6 For this reason, in the present study, the serum or urinary TMAO was assayed to reflect its liver injury and the effects of quercetin directly on TMAO concentration. Similarly, our previous study also showed that high ingestion of L-carnitine could induce liver injury.7 Accumulating research studies have reported that extensive consumption of L-carnitine can exhibit certain toxic and side effects.4–7 In the present study, the protective effect of quercetin on the liver injury of mice with high L-carnitine ingestion was investigated by analysing the levels of serum transaminase, antioxidant ability, lipid metabolization, inflammatory mediators within the liver tissues, and the potentially toxic TMAO (trimethylamine N-oxide). No significant difference in the average ingestion of food and water was found for the entire duration of the experiments, while the body weight and liver weight together with hepatosomatic index (HI) in high L-carnitine mice were significantly higher than that of the untreated chow control mice after 12 weeks feeding (Table 1). Interestingly, administration of quercetin at 450 mg per kg bw significantly decreased the body weight, liver weight, and HI index of high L-carnitine-fed mice. These results suggested that quercetin played an important role in inhibiting the L-carnitine-induced growth performance changes based on our investigation.23
The serum AST and ALT activities have been demonstrated to be significant indicators for evaluating hepatic function.24,25 Herein, the serum AST and ALT levels of high L-carnitine-fed mice were markedly elevated when compared with the normal chow group, indicating that the 3% of L-carnitine feeding for consecutive 12 weeks could induce severe liver injury in mice. However, the serum ALT and AST activities of L-carnitine model mice (Fig. 2A and B) treated with quercetin were signally declined in a dose-dependent manner, suggesting that administration of quercetin could dose-dependently alleviate the hepatotoxicity induced by high L-carnitine ingestion in mice. Besides, liver injury is often accompanied by inflammation, and thus the levels of inflammatory cytokines IL-1, IL-6, TNF-α, and TNF-β in the liver were further measured.19,22 It was found that the inflammatory cytokines IL-1, IL-6, and TNF-α together with TNF-β in high L-carnitine mice were significantly increased in comparison with the chow group,9,10 whereas these inflammatory factor levels could be down-regulated when the L-carnitine mice were treated with different doses of quercetin (Fig. 2C–F). Moreover, the observed results from the liver damage and inflammation response caused by L-carnitine application were further confirmed by the histopathological observation (Fig. 2G).
Generally speaking, inflammatory occurrence might result from oxidative stress.26,27 For this reason, the oxidative stress-related factors were also ulteriorly investigated in this study. The present results indicated that quercetin could exhibit marked effects on ameliorating the liver oxidative stress caused by high L-carnitine feeding in mice (Fig. 3A–D). The levels of the liver MDA of the high L-carnitine group were significantly higher than that of the chow group. In contrast, T-SOD activity was significantly decreased after feeding with L-carnitine, and the GSH-Px activity together with RAHFR levels were also reduced, which further proved that high L-carnitine ingestion was able to induce an oxidative stress response in mice. As shown in Fig. 3, when the high L-carnitine feeding mice were treated with 150, 300, and 450 mg per kg bw of quercetin, the MDA levels were decreased at different levels, and the T-SOD activity was increased as well, and similarly, the GSH-Px activity and the RAHFR levels were ameliorated. These findings suggested that quercetin as a natural ingredient was effective in dose-dependently regulating and ameliorating inflammatory reactions in high L-carnitine feeding mice and improved the antioxidant capacity during this process. It is well known that the antioxidant system is involved in liver lipid metabolism.21 In our study, a high intake of L-carnitine could enhance the contents of hepatic TC, TG, and LDL-C and decrease the liver HDL-C level (Fig. 4A and B), while these alterations could be improved through the treatment of quercetin in L-carnitine fed mice, and these results were also confirmed by the reduction of fat droplets in oil red O staining (Fig. 4E). It was noted that the concentration that we used in this study (150, 300, and 450 mg kg−1 d−1) was higher than that of the report by Wu et al.28 because of the different functional tests. Our previous studies have also shown that quercetin possesses a high hepatoprotective effect in mice and high anti-tumor activity in human colon cancer LoVo cells and breast cancer MCF-7 cells,29,30 providing evidence that the difference in experimental samples and animal model might result in a slight difference in the results.
TMAO was formed in the liver by flavin-containing monooxidase from TMA as the gut microbiota-dependant metabolite,31,32 which is diffused from the intestinal tract to hepatocytes through hepatic veins. TMAO present in urine or plasma has been considered a biomarker for liver injury, cardiovascular disease, metabolic syndrome, and some other diseases, which might usually produce many toxic and even pathogenic effects in vivo.7,33 Therefore, it is vital to take active and positive measures to prevent these damages. In this regard, the TMAO levels in systemic circulation, including the serum and the urine among the different tested groups of mice, were severally determined after 12 weeks of L-carnitine feeding and quercetin treatment (Fig. 1). The serum TMAO levels of mice decreased via intragastric administration of quercetin at 150, 300, and 450 mg per kg bw when compared to the L-carnitine group. The concentration of the urinary TMAO of the mice in Que150, Que 300, and Que 450 groups was down-regulated due to the quercetin treatment, indicating quercetin could dose-dependently decrease the levels of TMAO in the systemic circulation caused by high L-carnitine ingestion in mice. Interestingly, the variation tendency of circulating TMAO in mice was highly associated with the alteration of the serum transaminases, inflammatory factors, oxidative stress, and lipid metabolism. The down-regulation of TMAO formation in systemic circulation might be an important cause for ameliorating L-carnitine-induced liver injury in mice.34 It was reported that quercetin could remodel the structure of gut microbiota in HFD-induced liver injury mice35 and it could be inferred that quercetin could act with the gut microbiota to prevent TMAO formation. In the future, we will design more experiments to further study the relationship of quercetin with gut microbiota.
In conclusion, the present study ulteriorly demonstrated that quercetin treatment could dose-dependently antagonize the liver-toxic effects in high L-carnitine-fed mice. Importantly, this finding might open up the possibility for the exploitation of quercetin as a novel preventive and therapeutic ingredient to mitigate chronic L-carnitine-induced diseases and provide a scientific basis for the application of quercetin for beneficial effects on health.
Abbreviations
TMAO | Trimethylamine N-oxide |
TMA | Trimethylamine |
DMA | Dimethylamine |
d9-TMAO | d9-Trimethylamine N-oxide |
d9-TMA | d9-Trimethylamine |
d6-TMA | d6-Dimethylamine |
LC-MS | Liquid chromatography-mass spectra |
CVD | Cardiovascular disease |
CKD | Chronic kidney disease |
FMO3 | Hepatic flavin monooxygenase 3 |
ALT | Alanine aminotransferase |
AST | Aspartate aminotransferase |
IL-1 | Interleukin-1 |
IL-6 | Interleukin-6 |
TNF-α | Tumor necrosis factor-α |
TNF-β | Tumor necrosis factor-β |
TC | Total cholesterol |
TG | Total triglyceride |
HDL-C | High-density lipoprotein-cholesterol |
LDL-C | Low-density lipoprotein-cholesterol |
MDA | Malonaldehyde |
RAHFR | Restraining ability to hydroxyl free radical |
SOD | Superoxide dimutase |
GSH-Px | Glutathione peroxidase |
H&E | Hematoxylin & eosin |
Conflicts of interest
The authors declare no competing financial interest.
Acknowledgements
This study was granted by the National Natural Science Foundation of China (31871752 and 32072175), the Key Research and Development Plan in Shaanxi Province (2020ZDLSF01-07 and S2022-YD-QFY-0057), the Development Program for Innovative Research Team of Shaanxi Normal University (GK202101006), and the Sci-Tech Innovation Team of Shaanxi Province, China (2019TD-035).
References
- D. W. Foster, The role of the carnitine system in human metabolism, Ann. N. Y. Acad. Sci., 2004, 1033, 1–16 CrossRef CAS PubMed.
- J. Delgado, D. Ansorena, T. H. Van, I. Astiasarán, S. S. De and M. Estévez, Meat lipids, NaCl and carnitine: Do they unveil the conundrum of the association between red and processed meat intake and cardiovascular diseases?_Invited Review, Meat Sci., 2021, 171, 108278 CrossRef CAS PubMed.
- J. Demarquoy, B. Georges, C. Rigault, M. C. Royer, A. Clairet, M. Soty, S. Lekounoungou and F. L. Borgne, Radioisotopic determination of L-carnitine content in foods commonly eaten in Western countries, Food Chem., 2004, 86, 137–142 CrossRef CAS.
- L. O. Dragsted, Biomarkers of meat intake and the application of nutrigenomics, Meat Sci., 2010, 84(2), 301–307 CrossRef CAS PubMed.
- Z. Wang, E. Klipfell, B. J. Bennett, R. Koeth, B. S. Levison, B. Dugar, A. E. Feldstein, E. B. Britt, X. Fu, Y. M. Chung, Y. Wu, P. Schauer, J. D. Smith, H. Allayee, W. H. Tang, J. A. DiDonato, A. J. Lusis and S. L. Hazen, Gut flora metabolism of phosphatidylcholine promotes cardiovascular disease, Nature, 2011, 472, 57–63 CrossRef CAS PubMed.
- R. A. Koeth, Z. Wang, B. S. Levison, J. A. Buffa, E. Org, B. T. Sheehy, E. B. Britt, X. Fu, Y. Wu, L. Li, J. D. Smith, J. A. DiDonato, J. Chen, H. Li, G. D. Wu, J. D. Lewis, M. Warrier, J. M. Brown, R. M. Krauss, W. H. Tang, F. D. Bushman, A. J. Lusis and S. L. Hazen, Intestinal microbiota metabolism of L-carnitine, a nutrient in red meat, promotes atherosclerosis, Nat. Med., 2013, 19, 576–585 CrossRef CAS.
- Z. Wang, A. B. Roberts, J. A. Buffa, B. S. Levison, W. Zhu, E. Org, X. Gu, Y. Huang, Z. D. Maryam, M. K. Culley, A. J. DiDonato, X. Fu, J. E. Hazen, D. Krajcik, J. A. DiDonato, A. J. Lusis and S. L. Hazen, Non-lethal Inhibition of Gut Microbial Trimethylamine Production for the Treatment of Atherosclerosis, Cell, 2015, 163, 1585–1595 CrossRef CAS PubMed.
- Q. Wu, X. N. Zhang, Y. Zhao and X. B. Yang, High L-Carnitine Ingestion Impairs Liver Function by Disordering Gut Bacteria Composition in Mice, J. Agric. Food Chem., 2020, 68, 5707–5714 CrossRef CAS PubMed.
- Y. Y. Hu, Y. Zhao, L. Yuan and X. B. Yang, Protective effects of tartary buckwheat flavonoids on high TMAO diet-induced vascular dysfunction and liver injury in mice, Food Funct., 2015, 6, 3359–3372 RSC.
- J. J. Guo, Y. H. Meng, Y. Zhao, Y. Y. Hu, D. Y. Ren and X. B. Yang, Myricetin derived from Hovenia dulcis Thunb. ameliorates vascular endothelial dysfunction and liver injury in high choline-fed mice, Food Funct., 2015, 6, 1620–1634 RSC.
- M. Adachi and H. Ishii, Role of mitochondria in alcoholic liver injury, Free Radicals Biol. Med., 2002, 32, 487–491 CrossRef CAS.
- K. Nowotny, T. Jung, A. Höhn, D. Weber and T. Grune, Advanced glycation end products and oxidative stress in type 2 diabetes mellitus, Biomolecules, 2015, 5, 194–222 CrossRef CAS PubMed.
- D. Porras, E. Nistal, M. F. Susana, P. V. Sandra, J. L. Olcoz, R. Jover, G. G. Javier, V. G. M. María and S. C. Sonia, Protective effect
of quercetin on high-fat diet-induced non-alcoholic fatty liver disease in mice is mediated by modulating intestinal microbiota imbalance and related gut-liver axis activation, Free Radicals Biol. Med., 2017, 102, 188–202 CrossRef CAS PubMed.
- M. Wang, X. J. Zhang, C. Y. Yan, C. W. He, P. Li, M. W. Chen, H. X. Su and J. B. Wan, Preventive effect of α-linolenic acid-rich flaxseed oil against ethanol-induced liver injury is associated with ameliorating gut-derived endotoxin-mediated inflammation in mice, J. Funct. Foods, 2016, 23, 532–541 CrossRef CAS.
- R. J. Zhang, Y. Zhao, Y. F. Sun, X. S. Lu and X. B. Yang, Isolation, Characterization, and Hepatoprotective Effects of the Raffinose Family Oligosaccharides from Rehmannia glutinosa Libosch, J. Agric. Food Chem., 2013, 61, 7786–7793 CrossRef CAS.
- Y. M. Cui, Y. Han, X. B. Yang, Y. F. Sun and Y. Zhao, Protective Effects of Quercetin and Quercetin-5′,8-Disulfonate against Carbon Tetrachloride-Caused Oxidative Liver Injury in Mice, Molecules, 2013, 19, 291–305 CrossRef.
- Q. Wu, Y. Zhao, X. N. Zhang and X. B. Yang, A faster and simpler UPLC-MS/MS method for simultaneous determination of trimethylamine N-oxide, trimethylamine and dimethylamine in different types of biological samples, Food Funct., 2019, 10, 6484–6491 RSC.
- K. Nowotny, T. Jung, A. Höhn, D. Weber and T. Grune, Advanced glycation end products and oxidative stress in type 2 diabetes mellitus, Biomolecules, 2015, 5, 194–222 CrossRef CAS PubMed.
- N. H. Hu, C. C. Guo, X. Y. Dai, C. Wang, L. H. Gong, L. Y. Yu, C. Peng and Y. X. Li, Forsythiae Fructuse water extract attenuates liver fibrosis via TLR4/MyD88/NF-κB and TGF-β/smads signaling pathway, J. Ethnopharmacol., 2020, 262, 113275 CrossRef CAS PubMed.
- L. Ai, W. Luo, P. Yuan, L. Liu and Y. Zhou, Liver macrophages mediate effects of downhill running and caloric restriction on nonalcoholic fatty liver disease of high fat diet-fed mice, Life Sci., 2020, 256, 117978 CrossRef CAS PubMed.
- M. Ruart, L. Chavarria, G. Campreciós, N. Suárez-Herrera, C. Montironi, S. Guixé-Muntet, J. Bosch, S. L. Friedman, J. C. Garcia-Pagán and V. Hernández-Gea, Impaired endothelial autophagy promotes liver fibrosis by aggravating the oxidative stress response during acute liver injury, J. Hepatol., 2019, 70, 458–469 CrossRef CAS.
- K. Nowotny, T. Jung, A. Höhn, D. Weber and T. Grune, Advanced glycation end products and oxidative stress in type 2 diabetes mellitus, Biomolecules, 2015, 5, 194–222 CrossRef CAS PubMed.
- M. I. Yousef, S. A. Omar, M. I. El-Guendi and L. A. Abdelmegid, Potential protective effects of quercetin and curcumin on paracetamol-induced histological changes, oxidative stress, impaired liver and kidney functions and haematotoxicity in rat, Food Chem. Toxicol., 2010, 48, 3246–3261 CrossRef CAS PubMed.
- S. E. Wolverton and K. Remlinger, Suggested guidelines for patient monitoring: hepatic and hematologic toxicity attributable to systemic dermatologic drugs, Dermatol. Clin., 2007, 25, 195–205 CrossRef CAS PubMed.
- S. L. Zettervall, K. Dansey, N. J. Swerdlow, P. Soden, A. Evenson and M. L. Schermerhorn, Aspartate transaminase to platelet ratio index and Model for End-Stage Liver Disease scores are associated with morbidity and mortality after endovascular aneurysm repair among patients with liver dysfunction, J. Vasc. Surg., 2020, 72, 904–909 CrossRef PubMed.
- C. Xu, L. Chen, R. J. Wang and J. Meng, LncRNA KCNQ1OT1 knockdown inhibits ox-LDL-induced inflammatory response and oxidative stress in THP-1 macrophages through the miR-137/TNFAIP1 axis, Cytokine, 2022, 155, 155912 CrossRef CAS PubMed.
- H. Mohammadi, S. Talebi, A. Ghavami, M. Rafiei, S. Sharifi, Z. Faghihimani, G. Ranjbar, M. Miraghajani and G. Askari, Effects of zinc supplementation on inflammatory biomarkers and oxidative stress in adults: A systematic review and meta-analysis of randomized controlled trials, J. Trace Elem. Med. Biol., 2021, 68, 126857 CrossRef CAS.
- D. N. Wu, L. Guan, Y. X. Jiang, S. H. Ma, Y. N. Sun, H. T. Lei, W. F. Yang and Q. F. Wang, Microbiome and metabonomics study of quercetin for the treatment of atherosclerosis, Cardiovasc. Diagn. Ther., 2019, 9(6), 545–560 CrossRef PubMed.
- H. S. Zhang, M. Zhang, L. H. Yu, Y. Zhao, N. W. He and X. B. Yang, Antitumor activities of quercetin and quercetin-5′,8-disulfonate in human colon and breast cancer cell lines, Food Chem. Toxicol., 2012, 50, 1589–1599 CrossRef CAS PubMed.
- Y. M. Cui, Y. Han, X. B. Yang, Y. F. Sun and Y. Zhao, Protective effects of quercetin and quercetin-5′,8-disulfonate against carbon tetrachloride-caused oxidative liver injury in mice, Molecules, 2013, 19, 291–305 CrossRef PubMed.
- K. Y. Lin, X. D. Wang, J. Li, P. Zhao, X. W. Xi, Y. Feng, L. Yin, J. W. Tian, H. L. Li, X. X. Liu and B. Yu, Anti-atherosclerotic effects of geraniin through the gut microbiota-dependent trimethylamine N-oxide (TMAO) pathway in mice, Phytomedicine, 2022, 101, 154104 CrossRef CAS PubMed.
- L. Dannenberg, D. Zikeli, M. Benkhoff, S. Ahlbrecht, M. Kelm, B. Levkau and A. Polzin, Targeting the human microbiome and its metabolite TMAO in cardiovascular prevention and therapy, Pharmacol. Ther., 2020, 213, 107584 CrossRef CAS PubMed.
- W. H. W. Tang, X. S. Li, Y. P. Wu, Z. N. Wang, K. T. Khaw, N. J. Wareham, M. Nieuwdorp, S. M. Boekholdt and S. L. Hazen, Plasma trimethylamine N-oxide (TMAO) levels predict future risk of coronary artery disease in apparently healthy individuals in the EPIC-Norfolk prospective population study, Am. Heart J., 2021, 236, 80–86 CrossRef CAS.
- J. L. Flores-Guerrero, A. Post, P. R. van Dijk, M. A. Connelly, E. Garcia, G. Navis, S. J. L. Bakker and R. P. F. Dullaart, Circulating trimethylamine-N-oxide is associated with all-cause mortality in subjects with nonalcoholic fatty liver disease, Liver Int., 2021, 41, 2371–2382 CrossRef CAS PubMed.
- D. Porras-Sanabria, M. V. García-Mediavilla, S. Martínez-Flórez, E. Nistal, J. L. Olcoz and R. Jover, Modulation of Intestinal Microbiota and Gut-Liver Axis by Quercetin Improve HFD-Induced Metabolic Syndrome and Non-Alcoholic Fatty Liver Disease in Mice, J. Hepatol., 2016, 64(2), S677 CrossRef.
|
This journal is © The Royal Society of Chemistry 2023 |
Click here to see how this site uses Cookies. View our privacy policy here.