DOI:
10.1039/D2FO02659G
(Paper)
Food Funct., 2023,
14, 181-194
Bifidobacterium bifidum FJSWX19M5 alleviated 2,4,6-trinitrobenzene sulfonic acid (TNBS)-induced chronic colitis by mitigating gut barrier injury and increasing regulatory T cells†
Received
8th September 2022
, Accepted 25th November 2022
First published on 28th November 2022
Abstract
Probiotics have been evaluated as alternative approaches for preventing the relapse of Crohn's disease (CD). Previously, we observed strain-specific anti-inflammatory properties of Bifidobacterium bifidum in 2,4,6-trinitrobenzene sulfonic acid (TNBS) acute colitis models. In this study, we further assessed the effects of several B. bifidum strains on colonic damage, fibrosis, inflammatory factors, intestinal microbial and metabolic profiles, and peripheral regulatory T cells (Tregs) in the context of TNBS chronic colitis in mice. These results indicated that B. bifidum FJSWX19M5, but not FXJWS17M4, ameliorated body weight loss, reduced colonic shortening and injury, decreased markers of gut inflammation, and rebalanced colonic metabolism in TNBS-treated mice. FJSWX19M5 supplementation also promoted Treg cell differentiation and intestinal barrier restoration compared to other strains. All living B. bifidum strains (FJSWX19M5, FXJWS17M4 and FHENJZ3M6) seemed to restore the disruption of the gut microbiota caused by TNBS. The co-culture of B. bifidum strains and mesenteric lymph node cells from TNBS-treated mice showed that those strains with anti-colitis could induce higher IL-10 levels and a lower ratio of IL-22/IL-10 and IL-17/IL-10 when compared to those strains that were not protective. Furthermore, heat-killed FJSWX19M5 exhibited a relief effect on colitis-related symptoms (including body weight loss, colonic shortening and injury). These data imply that specific B. bifidum strains or their lysates may be the current therapeutic alternatives for CD.
1 Introduction
Crohn's disease (CD) is a chronic and recurrent condition of gut inflammation characterized by nausea, vomiting, diarrhea, abdominal pain, weight loss, rectal bleeding, and abdominal cramps. Since the beginning of the 21st century, CD has evolved into a global disease with high incidence in both developed and newly industrialized countries. Unlike ulcerative colitis (UC), the onset site of CD can spread throughout the gastrointestinal tract, including multiple independent inflammatory areas. Patients with CD are at high risk of cancer, anemia, nutritional deficiencies, infection, and thrombotic events. Maximizing preventive measures and maintaining remission are crucial to care for these patients.1 However, there is a lack of an established standard therapy.
The etiology of CD has not been thoroughly explained, but it is deemed to be the result of altered gut microbes and abnormal gut immunity associated with other factors (diet styles and infection) in vulnerable populations. In the gut, reduced abundance of health-associated taxa (such as Faecalibacterium prausnitzii, Roseburia intestinalis, and Bifidobacterium species) and increased abundance of opportunistic pathogens, including Bacteroides fragilis, Serratia marcescens, and Escherichia/Shigella attached to the gut epithelium, are observed in CD patients.2–5 Failure to maintain homeostasis between the host and its gut microbiota contributes to chronic conditions with potential inflammation. The mechanisms by which intestinal microorganisms induce inflammation include activation of an abnormal immune response and changes in gut permeability. Mechanistically, therapies that modulate intestinal microbiota, such as probiotics, prebiotics, dietary components, and fecal transplants, have potential therapeutic benefits in CD.
Several clinical studies have investigated the effectiveness of probiotics such as VSL#3 and Saccharomyces boulardii on the maintenance of remission and severity of inflammation in patients with CD.6,7 Probiotics also improve cognitive reactivity to sad moods in patients with CD.8Escherichia coli Nissle 1917 inhibits the adhesion and invasion of pathogenic E. coli into the intestinal epithelial cells in CD patients.9L. kefiri and L. casei treatment reduces the release of pro-inflammatory cytokines (TNF-α, IFN-γ, and IL-6) and the proportion of activated T cells in the lamina propria (LP) of CD mucosa.10,11 Furthermore, the combined use of probiotics, prebiotics, and glucocorticoids also showed good efficacy in reducing the infection rate and improving the level of intestinal microbiota in patients with CD.12,13 However, the molecular mechanisms by which probiotic therapies improve CD symptoms have not yet been studied.
It is shown that various strains of B. bifidum possess anti-inflammatory properties, limit pathogenic invasion, and maintain mucosal barrier function, and have received considerable attention as probiotics.14,15 Certain B. bifidum strains (such as MIMBb75 and CCFM16) or their inactivated bacteria relieve the symptoms of gastrointestinal diseases (including constipation, irritable bowel syndrome, UC, and colorectal cancer) and improve the gastrointestinal health of the host.16–18 The neutral polysaccharide fractions of B. bifidum PRI1 are able to induce the immunosuppressive Treg cells with the capabilities to alleviate gut inflammation.19,20 Our previous study demonstrated that treatment with B. bifidum strains reversed inflammatory responses and reduced colitis symptoms in acute 2,4,6-trinitrobenzene sulfonic acid (TNBS)-treated mice.21 We also observed that this protective effect of B. bifidum against acute colitis was strain-specific and correlated well with the immunomodulatory properties of the strains in the mesenteric lymph node (MLN) co-culture model. Based on this information, combined with our data from the in vitro discovery platform, we selected three B. bifidum strains (FJSWX19M5, FXJWS17M4 and FHENJZ3M6) and further compared the differences between those B. bifidum strains in alleviating TNBS-induced chronic colitis in mice.
In this study, when we treated chronic colitis mice with B. bifidum strains to establish associations with the potential therapeutic use of CD, only specific B. bifidum strains reduced the disease symptoms by eliciting host anti-inflammatory responses and improving the gut barrier function. Both viable and heat-killed B. bifidum FJSWX19M5 enhanced Treg cell differentiation of the MLN and reversed the metabolic disorders of the intestinal contents in mice with TNBS-induced chronic colitis.
2 Materials and methods
2.1 Strains and growth
B. bifidum strains (including FJSWX19M5, FHENJZ3M6, FXJWS17M4, M203F02M632 and FBJ1M4) were isolated from the feces of healthy Chinese individuals and obtained from the Food Biotechnology Centre of Jiangnan University. B. bifidum strains were cultivated in de Man–Rogosa–Sharpe broth (MRS) containing 0.1% cysteine hydrochloride (pH 6.4) and grown in an anaerobic chamber at 37 °C. Cultured bacterial cells were obtained and washed three times with phosphate-buffered saline (PBS). Dead bacteria were obtained by heating the strains at 95 °C for 20 min. The cell suspension was concentrated to 109 CFU per 200 μL for gavage.
2.2 Induction of colitis
A total of 60 six-week male BALB/c mice from Charles River Company (Shanghai, China) were randomly divided into six groups: control, TNBS, FJSWX19M5, heat-killed FJSWX19M5 (H-FJSWX19M5), FHENJZ3M6, and FXJWS17M4 groups, with 10 mice in each group. According to the method of Wirtz et al., with slight modifications, chronic colitis was induced in a mouse model by weekly rectal perfusion of TNBS (Sigma-Aldrich) under isoflurane anesthesia.22 Each mouse received incremental doses of TNBS in 35% ethanol for 5 weeks. At week 0, 150 μL of 1% (w/v) TNBS pre-sensitization solution was applied to the shaved skin of mice. At weeks I–IV, the mice received 50 μL of 1%, 1.5%, 2%, and 2.5% (w/v) TNBS in 35% ethanol. Mice in the control group received 5 μL of 35% ethanol. During the experiment, all animals were given 0.2 mL of PBS or a bacterial cell suspension by gavage. The mice were killed by inhalation of 100% CO2 on the third day after the last TNBS exposure. The colons were then removed for RNA extraction. The levels of IL-10, IL-17 and IL-6 in the supernatant of the colon and ileum homogenates were quantified using a mouse ELISA kit (R&D Systems). Mice were fed under SPF conditions at the animal facility of Jiangnan University (JN). This study protocol was approved by the Ethics Committee of JN, China (JN No. 20220615b1200920[229]) and was conducted following the European Community guidelines (Directive 2010/63/EU).
2.3 Evaluation of colitis
The disease activity index (DAI) was used to assess colitis severity in mice.23 The length of the colon and spleen indices were deemed as indirect indicators of disease severity. Colon tissues (0.5 cm) were fixed in 4% (w/v) formalin solution, embedded in paraffin, sectioned, and stained with hematoxylin and eosin (H&E), Masson's trichrome stain, and periodic acid-Schiff-alcian blue (AB-PAS). Furthermore, histological damage scores (ranging from 0 to 8) were calculated according to four indices: degree of crypt damage, degree of inflammation, goblet cell loss, and inflammatory cell infiltration.
2.4
In vitro co-culture model
Briefly, the MLN was freshly removed from male TNBS-treated BALB/c mice, mechanically disrupted, and cultured in RPMI 1640 medium containing 10% fetal bovine serum (FBS), 1 mM anti-CD3, and anti-CD28 (eBioscience). The cell suspensions (1 × 106) were co-cultured with viable or unviable bacterial suspension (optical density 600, 0.5) in a 96-well plate at a volume of 10
:
1 for 72 h.21 IL-10, IL-17, and IL-22 were quantified using the corresponding mouse ELISA kits (R&D Systems) and replicated three times.
2.5 Quantitative PCR
qPCR was conducted as previously described,24 and the total mRNA was extracted from the colon tissues using the TRIzol reagent and converted into cDNA using TaqMan reverse transcription kits (Applied Biosystems, USA). Primers for the targeted and reference genes were designed (Table 1).
Table 1 Test primer sequence
Primer |
Forward |
Reverse |
ZO-1 |
CTTCTCTTGCTGGCCCTAAAC |
TGGCTTCACTTGAGGTTTCTG |
Claudin-1 |
GGGGACAACATCGTGACCG |
AGGAGTCGAAGACTTTGCACT |
Claudin-2 |
CAACTGGTGGGCTACATCCTA |
CCCTTGGAAAAGCCAACCG |
MPO |
AGTTGTGCTGAGCTGTATGGA |
CGGCTGCTTGAAGTAAAACAGG |
Mucin 2 |
ATGCCCACCTCCTCAAAGAC |
GTAGTTTCCGTTGGAACAGTGAA |
Stat3 |
CAATACCATTGACCTGCCGAT |
GAGCGACTCAAACTGCCCT |
MyD88 |
TCATGTTCTCCATACCCTTGGT |
AAACTGCGAGTGGGGTCAG |
IL-17 |
TTTAACTCCCTTGGCGCAAAA |
CTTTCCCTCCGCATTGACAC |
IL-12 |
CTGTGCCTTGGTAGCATCTATG |
GCAGAGTCTCGCCATTATGATTC |
IL-6 |
CTCTGCAAGAGACTTCCATCCAGT |
GAAGTAGGGAAGGCCGTGG |
IFN-γ |
ATGAACGCTACACACTGCATC |
CCATCCTTTTGCCAGTTCCTC |
CD86 |
TGTTTCCGTGGAGACGCAAG |
TTGAGCCTTTGTAAATGGGCA |
CD80 |
ACCCCCAACATAACTGAGTCT |
TTCCAACCAAGAGAAGCGAGG |
β-Actin |
GGCTGTATTCCCCTCCATCG |
CCAGTTGGTAACAATGCCATGT |
2.6 Flow cytometry analysis
Fresh MLN and spleen cells were removed from each group of mice. Single-cell suspensions (106–107 cells per tube) were incubated with fluorescein isothiocyanate (FITC)-conjugated rat anti-mouse CD4 (0.125 μg per test) monoclonal antibody and APC-conjugated CD25 (0.06 μg per test) monoclonal antibody at 4 °C for 45 min. After three washes, the cells were permeabilized and stained with PE-conjugated rat anti-mouse Foxp3 (0.5 μg per test) monoclonal antibody for 45 min at 4 °C in darkness. IgG2a kappa isotype control-PE was used as the negative control. The final number of Tregs was calculated as the proportion of Foxp3+ CD4+ cells in the CD4+ population. Data were collected using a flow cytometer (Attune NxT; Thermo Fisher Scientific), and the results were analyzed using FlowJo software (v 10.0.7; Tree Star).
2.7 Gut microbiota analysis
16S ribosomal RNA (rRNA) gene sequencing was performed as described.25 Briefly, the total genomic DNA was extracted from the ileal contents using a QIAamp DNA Stool Mini Kit (Germany). 314F and 806R primers were utilized for the amplification of the V3–4 region of the 16S rRNA genes. QIIME2 software was utilized to select the operational taxonomic units (OTUs) and summarize the taxonomic relationships. White's non-parametric t-tests were performed using STAMP to find out the differentially enriched taxa between groups. Data are available on NCBI SRA with the accession number SRP408149.
2.8 Metabolomics analysis
The colon content (60 mg) was collected and homogenized with 800 μL of a methanol–acetonitrile solution (2
:
1, v/v) and then centrifuged at 4 °C (13
000g, 15 min). Next, 400 μL of the supernatant was concentrated, dried for 3 h, and resuspended in 200 μL of a methanol–water solution (4
:
1, v/v). After centrifugation, the samples were filtered for further analysis. Two microliters of each sample were mixed as quality control (QC) samples in the same tube. A methanol–water solution (4
:
1, v/v) was used as a blank. A Dionex Ultimate-3000 UPLC system coupled with a QE Orbitrap mass spectrometer (Thermo Fisher Scientific) in the full scan mode was used to detect fecal metabolites. An Acquity HSS T3 column (2.1 mm × 0.1 cm, 1.8 μm; Waters) was utilized for chromatographic separation.26 Metabolomics data were analyzed using Compound Discoverer 3.2 and SIMCA 14.1. Metabolic features with variable importance in projection (VIP > 1, p < 0.05) were considered as biomarkers. MetaboAnalyst 5.0 was used to conduct the pathway and enrichment analysis based on the identified metabolites.
2.9 Statistical analysis
Data analysis and visualization were performed using GraphPad 8.4.3 and R (version 4.0.4). Data are presented as the mean ± standard error of the mean. Unless otherwise noted, mean differences between groups were evaluated using one-way analysis of variance and the P values were adjusted using Tukey's correction. *p < 0.05, **p < 0.01, or ***p < 0.001 vs. the TNBS group.
3 Results
3.1
B. bifidum FJSWX19M5 and its dead strain administration alleviated chronic colitis symptoms and fibrotic stenosis in TNBS-induced mice
Since B. bifidum has strain-specific anti-inflammatory properties in TNBS acute colitis, we further investigated the potential role of B. bifidum strains against chronic colitis. Here, we compared the protective capacities of FJSWX19M5 and FHENJZ3M6 with FXJWS17M4, a strain previously shown to be non-protective in an acute colitis model. Weight changes were monitored daily after the last TNBS injection as a readout of the murine status after colitis reactivation (Fig. 1A). We observed that FJSWX19M5, H-FJSWX19M5, and FHENJZ3M6 administration significantly improved the weight loss caused by TNBS injection compared with the TNBS group (p < 0.05). Exposure to TNBS caused 50% mortality, colon shortening, spleen reduction, and an increase in the DAI in mice relative to the naive controls (p < 0.05), indicative of severe inflammation and tissue damage (Fig. 1B–E). Treatment with FJSWX19M5, H-FJSWX19M5, or FHENJZ3M6 significantly alleviated these macroscopic symptoms, and FJSWX19M5 had the best effect. These data were consistent with the H&E and Masson histological section results of the distal colon (Fig. 1F–H). Treatment with the above strains relieved the disruption of epithelial crypt architecture and alleviated massive inflammatory cell infiltration, connective tissue hyperplasia, extensive fibrosis, and collagen deposition compared with the TNBS group mice. These data indicated that inactivated FJSWX19M5 still had a protective effect on chronic colitis, but its relief effect was weaker than that of live bacteria. In contrast, the oral administration of FXJWS17M4 did not improve these symptoms, following its failure to protect against acute colitis.
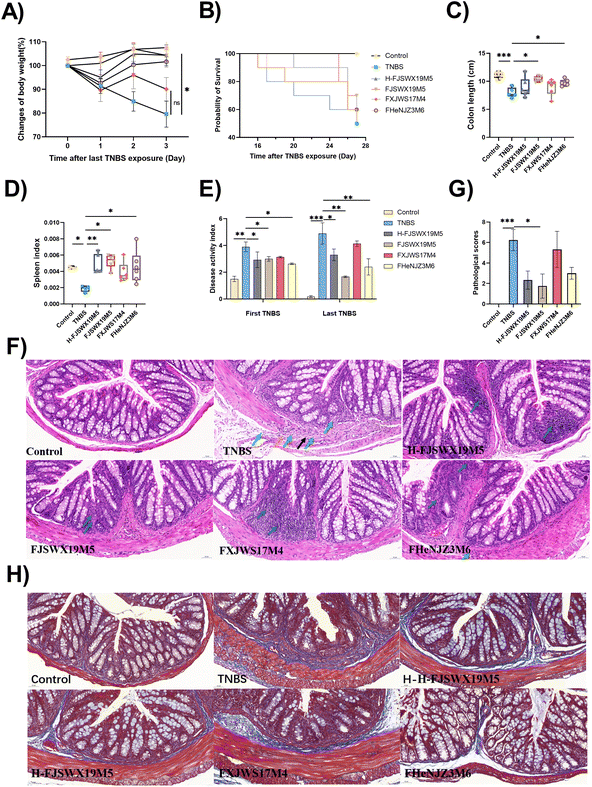 |
| Fig. 1 Live or heat-killed B. bifidum strains suppressed colonic damage and ameliorated the severity of TNBS murine chronic colitis. Chronic colitis was induced by a weekly incremental dose of TNBS in 35% ethanol. Mice were treated orally with 200 μL of live or heat-killed B. bifidum strains (109 CFU bacteria) throughout the experiment. Disease and recovery were monitored by the weight loss (A), probability of survival (B), colon length (C), spleen index (D), disease activity index (E), H&E staining of the distal colon (F), pathological score (G), and Masson's trichrome staining for fibrositis (H). Scale bar = 50 mm. The orange arrow indicates the rupture of muscularis mucosa. The black arrow indicates the proliferation of the connective tissue in the submucosa. The green arrow indicates granulocyte infiltration. Data were analyzed by ANOVA, followed by Tukey's corrected t-test. *p < 0.05, **p < 0.01, or ***p < 0.001 vs. the TNBS group. n = 5–8 mice per group. | |
3.2
B. bifidum FJSWX19M5 administration increased mucin production and improved the gut barrier of TNBS-treated mice
TNBS exposure damaged the colon mucosal physical barrier and mucus barrier, as shown by the decreased mucus layer thickness, number of goblet cells, mRNA levels of the tight junction (TJ)-associated proteins (ZO-1 and Claudin-1), and myeloperoxidase (MPO) mRNA expression in mice (p < 0.05). B. bifidum FJSWX19M5 administration significantly improved intestinal barrier damage in mice challenged with TNBS (Fig. 2A–D). Heat-killed B. bifidum FJSWX19M5 markedly increased the mRNA expressions of the pore-forming protein (Claudin-2) and MPO (p < 0.05). PAS-AB of mucus showed that B. bifidum administration increased the number of mucin-secreting goblet cells compared to TNBS-treated mice (Fig. 2E). This observation was linked to the increased mRNA expression of mucin 2 in both live and dead B. bifidum FJSWX19M5-treated mice, relative to their counterparts (p < 0.05). We next sought to determine whether mucin promotion by B. bifidum treatment was related to IL-22 secretion in vivo. Only B. bifidum FHENJZ3M6 markedly reduced the level of IL-22 in the colon tissues (p < 0.05). None of the strains significantly down-regulated the Stat3 and MyD88 mRNA levels in the colon tissues (Fig. 2F–I).
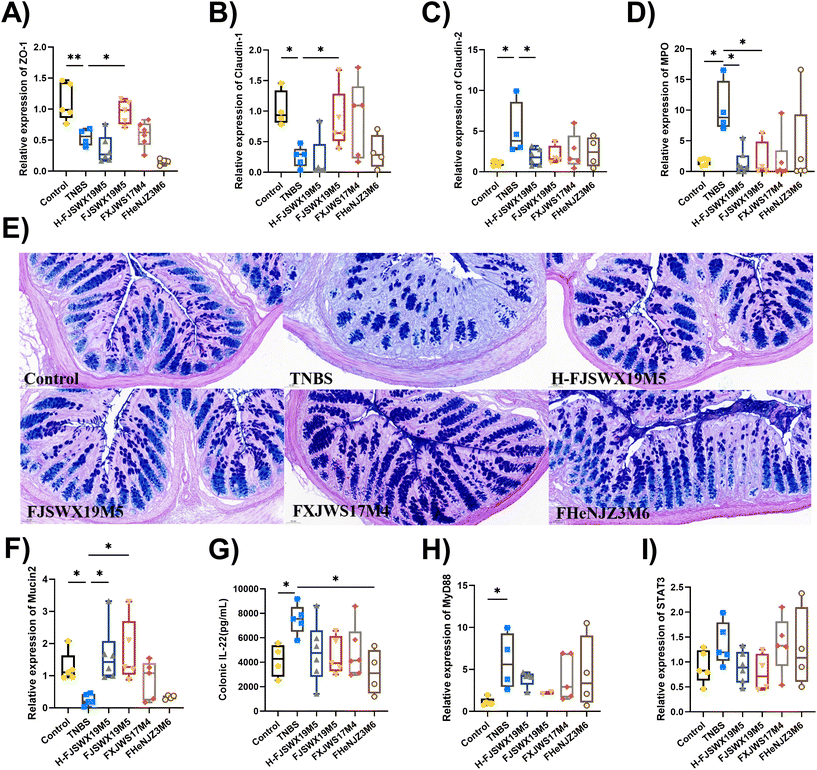 |
| Fig. 2 Live or heat-killed B. bifidum strains maintained tight junction function and mucin barrier in TNBS-treated mice. The relative mRNA expressions of ZO-1 (A), Claudin-1 (B), Claudin-2 (C), MPO (D), and mucin 2 (F) in the colon were analyzed by qPCR. PAS-AB of mucus was conducted (E). Scale bar = 50 mm. The supernatant of the colon homogenates was collected to detect the levels of the cytokine IL-22 (G). The relative mRNA expressions of MyD88 (H) and Stat3 (I) in the colon were analyzed by qPCR. n = 4–6 mice per group. Data were analyzed by ANOVA, followed by Tukey's corrected t-test. *p < 0.05 or **p < 0.01 vs. the TNBS group. | |
3.3
B. bifidum FJSWX19M5 inhibited Th1/Th17-mediated gut inflammation in mice with TNBS-induced chronic colitis
Inflammatory cytokines play pivotal roles in the development and prognosis of chronic colitis. We assessed the regulatory effects of different B. bifidum strains on the secretion of inflammatory cytokines in TNBS chronic colitis mice. Th1-cytokines (IFN-γ, IL-12, and TNF-α), Th2-cytokines (IL-6), Th17-cytokines (IL-17), and Treg-cytokines (IL-10) in the colon or ileal tissues were examined 3 d after the last TNBS injection. As shown in Fig. 3A–J, all Th1- and Th17-mediated cytokines were significantly increased following stimulation with TNBS. The levels of Th2 cytokines (IL-6) in the colon and ileum did not change in either groups (Fig. 3E–G). B. bifidum FJSWX19M5 treatment attenuated Th1- and Th17-mediated inflammatory reaction, reaching significance for most of the cytokines. Compared with mice in the TNBS group, although not significant, concentrations of the cytokine IL-10 were increased in the colonic and ileal homogenate supernatants after B. bifidum treatment (Fig. 3I and J). Furthermore, oral administration of FJSWX19M5 significantly increased the mRNA expression of CD86 and decreased the gene expression of CD80 in the colon tissues when compared with the levels in the model mice, suggesting its effect on restoring Th1/Th2 balance and inhibiting Th17 immunity (Fig. 3K and L).
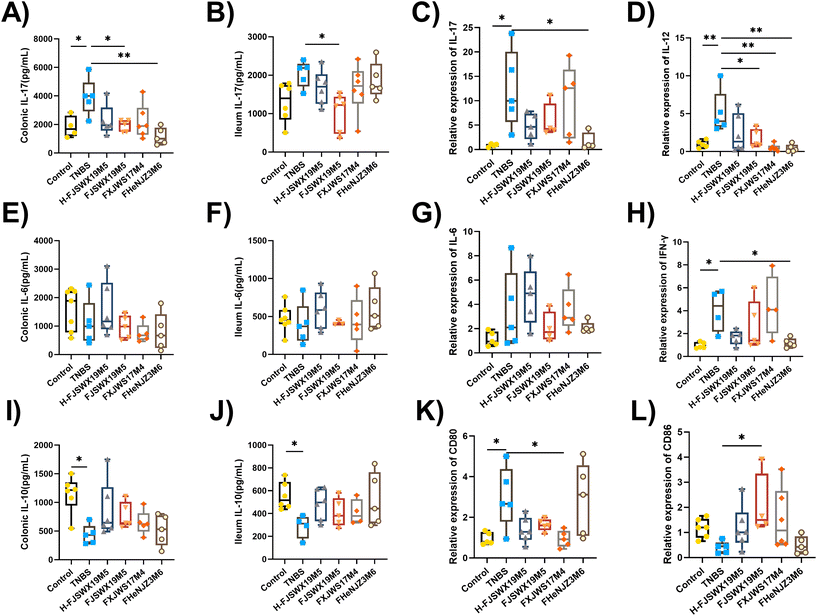 |
| Fig. 3 Live or heat-killed B. bifidum strains suppressed the production of colonic and ileal pro-inflammatory cytokines induced by TNBS exposure in mice. The supernatants of the colon (A, E, and I) and ileum (B, F, and J) homogenates were collected to detect the cytokine levels. The mRNA abundances of IL-17 (C), IL-12 (D), IL-6 (G), IFN-γ (H), CD80 (K), and CD86 (L) in the colon were analyzed by qPCR. Data were analyzed by ANOVA, followed by Tukey's corrected t-test. *p < 0.05 or **p < 0.01 vs. the TNBS group. n = 4–8 mice per group. | |
3.4
B. bifidum FJSWX19M5 and its heat-killed lysates specifically induced MLN Treg cell differentiation in a chronic model with TNBS colitis
Induced Treg (iTreg) cells have been previously reported to regulate Th1 and Th17 cell differentiation and suppress inflammatory responses in colitis mouse models. TNBS administration markedly decreased Foxp3+ Treg cells in the MLN (p < 0.05). Treatment with B. bifidum FJSWX19M5 and its dead strain alleviated this change in the MLN cells (Fig. 4A and B). Next, the MLN cells derived from the TNBS group were cultured with live or heat-killed B. bifidum strains for 72 h. The strains (including FJSWX19M5 and FHENJZ3M6) that were protective in a murine model of colitis could increase the IL-10 levels and decrease the ratio of IL-22/IL-10 and IL-17/IL-10 in the TNBS treatment MLN cells when compared with the strains (including FXJWS17M4, M203F02M632 and FBJ1M4) that were not protective (Fig. 4C–E). After the heat treatment (95 °C for 25 min), all B. bifidum strains showed a weakened ability to induce IL-10 secretion by the MLN cells (Fig. 4D).
 |
| Fig. 4 Live or heat-killed B. bifidum strains regulated the Treg function in the MLN of TNBS-treated mice. The percentage of Foxp3+ Treg cells in the CD4+ T cells was analyzed by FACS in the MLN (A) and spleen (B) cells at 3 d after the last TNBS treatment. The MLN cells derived from the TNBS group mice were co-cultured with live or heat-killed B. bifidum strains for 72 h. The levels of IL-10, IL-17, and IL-22 in the cell supernatant were detected using ELISA kits (C–E). Data were analyzed by ANOVA, followed by Tukey's corrected t-test. *p < 0.05 or **p < 0.01 vs. the TNBS group. n = 4–8 mice per group. | |
3.5
B. bifidum FJSWX19M5 significantly ameliorated the disorders of the gut microbiota in mice with TNBS-induced chronic colitis
Intestinal microbiota disorder is closely related to the pathogenesis of colitis. Chronic TNBS challenge dramatically altered intestinal microbiota, as shown by decreased α-diversity index (including shannon, evenness and faith_pd), abundance of the phylum Firmicutes, and increased abundance of the phylum Proteobacteria. All live B. bifidum strain treatments markedly increased the α-diversity and the bacterial phyla in the intestinal tracts of mice compared to the TNBS-treated mice (p < 0.05) (Fig. 5A–D). B. bifidum strains (FJSWX19M5 and FXJWS17M4) also led to the restoration of the Firmicutes and Bacteroidetes ratio, which reached 2.55 and 2.56, respectively (Fig. 5E). However, the heat-killed FJSWX19M5 failed to ameliorate the disorders of gut microbes in mice with TNBS-induced chronic colitis (Fig. 5A–E). We next investigated whether the relative abundances of specific taxa were linked with the treatment outcome of B. bifidum. Oral administration of live B. bifidum FJSWX19M5 enriched eleven bacteria taxa (such as Ruminococcaceae UCG-014, Ruminococcus 2, Romboutsia, Azospirillum sp. 47_25 and Akkermansia) and the dead FJSWX19M5 treatment only contributed to three enriched taxa (including Candidatus Gastranaerophilales bacterium Zag_111, [Eubacterium] ventriosum group and Akkermansia) in the gut of mice when compared with the levels in the model mice (p < 0.05) (Fig. 5F and G).
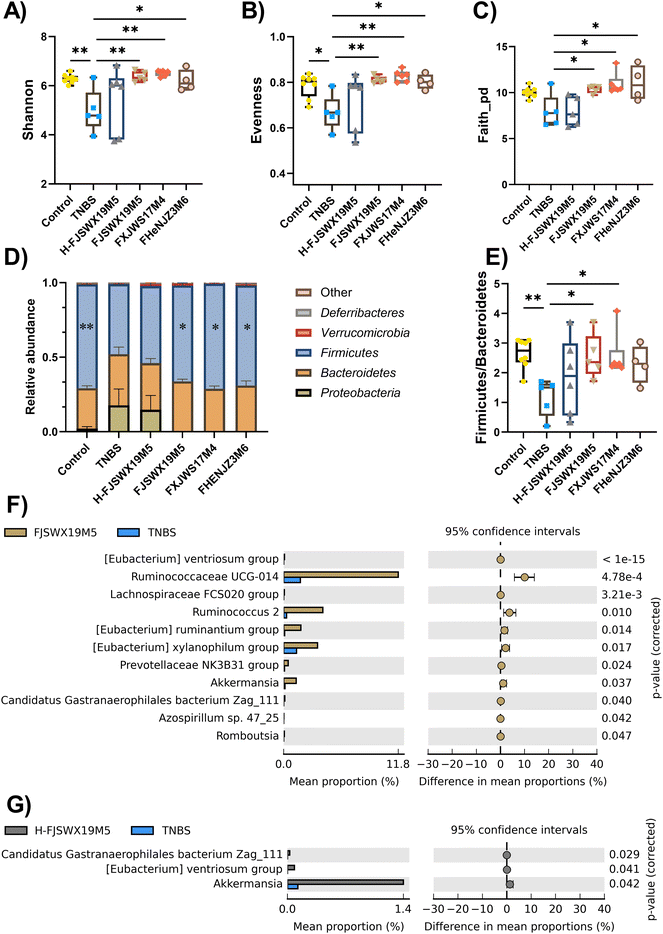 |
| Fig. 5 Live B. bifidum strain (FJSWX19M5) improved the gut microbes in TNBS-treated mice. α-Diversity of the gut microbiota (A–C). The phylogenetic composition of the gut microbiota at the phylum level (D). The ratio of Firmicutes and Bacteroidetes (E). Taxonomic markers among different groups of mice compared to TNBS mice (F and G). *p < 0.05 or **p < 0.01 vs. the TNBS group. | |
3.6
B. bifidum FJSWX19M5 and its dead strains alleviated the metabolic disorders of the colon contents in mice with TNBS-induced chronic colitis
To obtain a holistic view of the host metabolism after B. bifidum intervention, we utilized non-targeted metabolomics to identify a total of 2195 metabolites and the related pathways that were altered in the colon contents of mice. OPLS-DA analysis revealed that exposure to TNBS markedly contributed to the metabolic disorders of the colon contents in mice (ESI Fig. 1†). In total, 168 metabolites were significantly altered following TNBS treatment (p < 0.05, |log2
FC| > 1). Considering the top 100 most significant metabolites, both datasets showed a clear separation between the TNBS and the intervention groups (Fig. 6A and B). Significant clustering was observed among the control and FJSWX19M5 groups compared to the other groups, implying its full protective effects against TNBS colitis in mice. The effect of B. bifidum on the regulation of some differential metabolites (including arachidonic acid, β-muricholic acid, docosahexaenoic acid, eicosenoic acid, lauric acid ethyl ester, and deoxycholic acid) in the feces is shown in Fig. 6C (p < 0.05). The remarkable recovery of arachidonic acid (AA) and β-muricholic acid (β-MCA) was only observed after FJSWX19M5 administration (Fig. 6C). We chose the differentially expressed metabolites between the TNBS and FJSWX19M5 groups (p < 0.05, |log2
FC| > 1) for further analysis using MetaboAnalyst 5.0, and identified 14 metabolic pathways between the two groups. It is worth noting that B. bifidum FJSWX19M5 had the largest impact on the phenylalanine, tyrosine, and tryptophan biosynthesis pathways (impact = 1.0), which were extremely significant (p < 0.001) (Fig. 6D). The changed metabolites were mainly linked to the mercaptopurine (MP), thioguanine (TG) and azathioprine (AZA) action pathways (Fig. 6E).
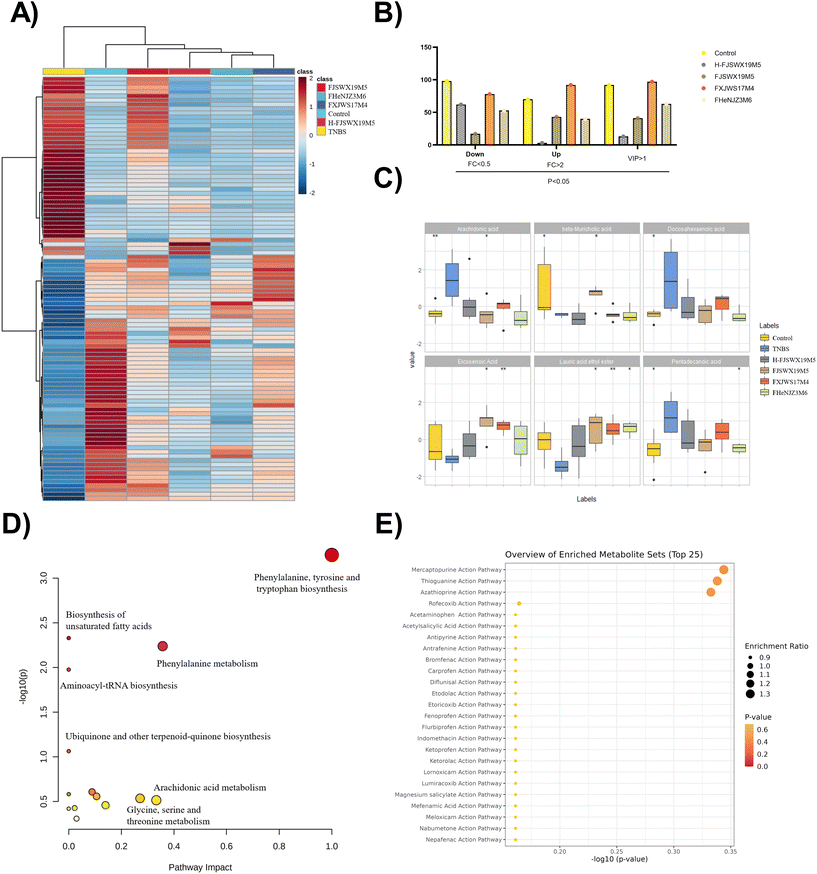 |
| Fig. 6 Live or heat-killed B. bifidum strains alleviated the metabolic disorders of the colon contents in TNBS-treated mice. The heat map compared the top 100 most important metabolites in mice that differed between the TNBS-treated and control groups (A). The bar graph shows the number of altered colonic metabolites in the different groups of mice compared to TNBS mice (B). The box-plot diagrams show several significant changes in the metabolites (C). The bubble diagram shows the classification items of the KEGG pathway in B. bifidum-treated mice (D). Enrichment analysis was conducted based on the drug pathways from SMPDB (E). *p < 0.05 or **p < 0.01 vs. the TNBS group. | |
4 Discussion
Probiotics are beneficial for human gut health. However, probiotics exhibit population- and strain-specific effects.27–29 This urged us to better characterize and compare probiotic strains under the same experimental conditions to select those with promising therapeutic properties. Herein, we demonstrated the strain-specific protective effects of B. bifidum on chronic TNBS colitis in murine models and obtained a strain (FJSWX19M5) with good performance. This functional property of the specific B. bifidum strain can be attributed to its regulatory effects on gut barrier restoration and Treg differentiation. Our data also showed that B. bifidum strains alleviated the metabolic disorders and modulated the imbalance of gut microbes in mice. In addition, non-viable bacterial strains also showed CD-ameliorating effects.
B. bifidum FJSWX19M5 and FHENJZ3M6 had protective effects on the disease symptoms of chronic colitis based on the weight changes, colon length, spleen index, DAI scores, and pathological mediators (Fig. 1). Consistent with our previous observations, these two strains significantly alleviated the primary readouts of acute colitis in the TNBS-treated mice (unpublished data). The significant effects of FJSWX19M5 treatment on colonic mucosal histopathology are of particular interest because endoscopic mucosal healing is the strongest predictor of sustained remission in the clinical field of CD treatment.6 Intestinal fibrosis is a common complication of CDs and can occur in >30% of patients with CD, but there are not enough drugs to treat this complication.30 The administration of B. bifidum FJSWX19M5 and its dead strain seemed to reduce extensive fibrin deposition in the colons of mice. Similarly, L. lactis ML2018 significantly inhibits the gene expressions of vascular endothelial growth factor and transforming growth factor 1 (TGF-β1) in the colon tissues of mice.31 A recent report showed that the lysates of multi-strain probiotics inhibited TGF-β1-induced intestinal fibrosis.32
The protective effects of the specific probiotics can be partly attributed to their regulatory effects on the intestinal barrier, including TJ function and mucin production. Herein, the specific B. bifidum strain (FJSWX19M5) increased TJ production and improved the physical barrier in the colons of TNBS-treated mice (Fig. 2A–D), consistent with the other reports.33,34 Treatment with B. pullicaecorum or a mixture of six butyrate-producing strains significantly improved the integrity of the epithelial barrier.35 Patients with CD show structural alterations at TJs, such as occludins, claudins, and zonulins. Restoration of this border reduces paracellular permeability and contributes to the regression of intestinal inflammation.36,37 Our results showed that treatment with B. bifidum FJSWX19M5 could upregulate the expression of MUC2 and increase mucin production in TNBS-treated mice (Fig. 2E and F). Bifidobacterium strains have been reported to reduce ER stress genes and modulate the unfolded protein response to induce MUC2 secretion.34 IL-22 signaling enhances STAT3 activation and promotes mucin production in goblet cells, enhancing wound repair.38,39 This might be not related to the activating effect of FJSWX19M5 on the IL-22-STAT3 pathway (Fig. 2G–I).
Gut inflammatory infiltrates in patients with CD contain Th1 and Th17 cells. B. bifidum FJSWX19M5 and its dead strains inhibited intestinal inflammation, as shown by the reduced pro-inflammatory Th1/Th17 cytokine production in the TNBS-colitis mice (Fig. 3G–I). Additionally, B. bifidum FJSWX19M5 significantly upregulated the CD86 expression in the colon tissues (Fig. 3L). Enhanced CD86 expression in the gut could mediate Th1 to Th2 skewing of cytokines and ameliorate TNBS-induced colitis.40B. bifidum, but not L. salivarius, Bacillus coagulans, and B. subtilis, induced the expression of CD86 from dendritic cells (DCs) in CD patients, and could interact with CD28, leading to the downregulation of T-cell responses.41 Chronic TNBS challenge leads to defective IL-10 production and impaired function of intestinal Treg cells, which have been reported in patients with CD.42B. bifidum FJSWX19M5 and its dead strains contributed to colonic IL-10 secretion and Treg differentiation in the MLN cells of mice with TNBS chronic colitis (Fig. 4A). Bioactive molecules derived from symbiotic microbiota critically contribute to intestinal immune maturation and regulation of IL-10 secretion.20 Inducible IL-10 production by Foxp3+ Treg cells is important for inducing tolerance to antigens on mucosal surfaces and preventing gut inflammation.43 Our observations showed that the strains with protective effects on TNBS colitis correlated well with high IL-10 inducibility in MLN cells (Fig. 4C–E). This is also consistent with the report of Im et al., who found that administration of L. helveticus HY7801 with IL-10high IL-12low phenotype in MLN cells showed both prophylactic and curative effects in slowing the course of collagen-induced rheumatoid arthritis (CIA).44 In addition, we found that the ability of B. bifidum FJSWX19M5 to induce Treg cell differentiation was partly compromised following the heat treatment (Fig. 4A). This inducibility may depend on the interactions with the gut microbiota. Microbial-derived metabolites (such as bile and short-chain fatty acids) have been reported to mitigate innate and adaptive immune responses to promote Treg function in a ligand-specific manner.45,46 Therefore, the induction of Treg differentiation by probiotics, particularly through the induction of tolerogenic DCs, may be applied as a therapeutic strategy to control intestinal inflammatory diseases.
Intestinal metabolic changes and microbiome disturbance are among the most important features of gut disease. CD patients with active disease were characterized by reduced community richness and alpha diversity, together with enrichment in the abundance of certain bacterial taxa belonging to Escherichia/Shigella, Enterococcus, Fusobacterium, Haemophilus, Megasphaera and Campylobacter.47 Clinical studies have also shown that some SCFA-producing bacterial taxa, such as the genera Faecalibacterium, Oscillospira, Akkermansia and Roseburia, were reduced in the CD patients compared to the healthy subjects.48,49 Our data demonstrated that B. bifidum strain treatment markedly increased the bacterial community richness and the abundance of some SCFA-producing bacteria, which may indicate that the disease is in remission. Both live and dead B. bifidum FJSWX19M5 administration led to an increase in the proportions of the Akkermansia genera (Fig. 5). A greater abundance of Akkermansia may contribute to the increase in neutral and acidic mucin concentrations in CD patients in remission.49 Targeting and enrichment of specific taxa (such as Akkermansia muciniphila) in the gut may be a potential approach in the recovery of CD patients.
The gut metabolic profile shifts and metabolic pathways may be altered during inflammation. Our data showed that all B. bifidum strains alleviated the metabolic disorders of the colon contents in mice (Fig. 6). Western-diet-derived ω-6 polyunsaturated fatty acids, specifically AA, fuelled epithelial ferroptosis, a CD feature.50 In contrast, hydrophilic BA and β-MCA have been reported to protect the intestinal mucosal barrier and reduce intestinal inflammation.51 The remarkable recovery of AA and β-MCA was observed with FJSWX19M5 administration, which may be the reason for its protective effects on TNBS-induced colitis. FJSWX19M5 administration also led to increased levels of C20:1 (eicosenoic acid) in the colons of mice. Similarly, Chen et al. found that increased eicosenoic acid levels were related to the anti-colitis effects of B. longum.52 Eicosenoic acid has been reported as a bioactive compound responsible for the protective activity of Ulmus macrocarpa in colitis murine models.53 At the pathway level, metabolomic analyses revealed that several pathways were enriched by FJSWX19M5 administration, such as the MP, TG, and AZA action pathways. Thiopurines (MP, TG, and AZA) are widely used in the clinical management of CD.54 This indicates the therapeutic potential of B. bifidum FJSWX19M5. Further research is needed to determine whether these compounds are directly responsible for the protective effects of B. bifidum or whether other molecules and pathways are involved.
5 Conclusion
In this study, we demonstrated that B. bifidum FJSWX19M5 had protective effects against TNBS-induced chronic colitis in mice. Both living and dead strains protected the CD mice against weight loss, pathological lesions of the colon, colonic fibrosis, and inflammatory parameters. FJSWX19M5 administration promoted the recovery of TNBS-induced metabolic disturbances in the colon tissue. Furthermore, a significant increase in the number of MLN Treg cells was observed following B. bifidum FJSWX19M5 administration. The anti-colitis effect of B. bifidum strains correlated with the upregulation of IL-10 production in the MLN cells. B. bifidum FJSWX19M5 could regulate specific bacterial genera and improve metabolic disorders in mice with TNBS-induced chronic colitis. Further studies are required to understand the strain-specific mechanisms by which B. bifidum and its bioactive components interact with the host to exert curative effects.
Author contributions
Dingwu Qu: design, methodology, data analysis, visualization, and writing – original draft. Qixiao Zhai and Leilei Yu: validation, methodology, and data curation. Fengwei Tian and Hao Zhang: investigation and supervision. Wei Chen: project administration. Qixiao Zhai and Zhennan Gu: conceptualization, supervision, funding, and resources.
Conflicts of interest
There is no conflict of interest.
Acknowledgements
This work was supported by the National Natural Science Foundation of China [No. 31820103010, 31972971 and 32021005] and the Collaborative Innovation Center of Food Safety and Quality Control in Jiangsu Province.
References
- K. Cushing and P. D. R. Higgins, Management of Crohn disease: A review, J. Am. Med. Assoc., 2021, 325, 69–80 CrossRef CAS PubMed.
- A. V. Vila, F. Imhann, V. Collij, S. A. Jankipersadsing, T. Gurry, Z. Mujagic, A. Kurilshikov, M. J. Bonder, X. Jiang and E. F. Tigchelaar, Gut microbiota composition and functional changes in inflammatory bowel disease and irritable bowel syndrome, Sci. Transl. Med., 2018, 10, eaap8914 CrossRef CAS PubMed.
- M. T. Henke, D. J. Kenny, C. D. Cassilly, H. Vlamakis, R. J. Xavier and J. Clardy,
Ruminococcus gnavus, a member of the human gut microbiome associated with Crohn's disease, produces an inflammatory polysaccharide, Proc. Natl. Acad. Sci. U. S. A., 2019, 116, 12672–12677 CrossRef CAS PubMed.
- B. Chassaing and A. Darfeuille-Michaud, The σE pathway is involved in biofilm formation by Crohn's disease-associated adherent-invasive Escherichia coli, J. Bacteriol., 2013, 195, 76–84 CrossRef CAS PubMed.
- G. Hoarau, P. Mukherjee, C. Gower-Rousseau, C. Hager, J. Chandra, M. Retuerto, C. Neut, S. Vermeire, J. Clemente and J.-F. Colombel, Bacteriome and mycobiome interactions underscore microbial dysbiosis in familial Crohn's disease, mBio, 2016, 7, e01250–e01216 CrossRef CAS PubMed.
- R. N. Fedorak, B. G. Feagan, N. Hotte, D. Leddin, L. A. Dieleman, D. M. Petrunia, R. Enns, A. Bitton, N. Chiba and P. Paré, The probiotic VSL# 3 has anti-inflammatory effects and could reduce endoscopic recurrence after surgery for Crohn's disease, Clin. Gastroenterol. Hepatol., 2015, 13, 928–935 CrossRef CAS.
- M. Guslandi, G. Mezzi, M. Sorghi and P. A. Testoni,
Saccharomyces boulardii, in maintenance treatment of Crohn's disease, Dig. Dis. Sci., 2000, 45, 1462–1464 CrossRef CAS PubMed.
- L. Wu, F. Shen, W. Wang, C. Qi, C. Wang, A. Shang and S. Xuan, The effect of multispecies probiotics on cognitive reactivity to sad mood in patients with Crohn's disease, J. Funct. Foods, 2021, 82, 104431 CrossRef.
- J. Boudeau, A. L. Glasser, S. Julien, J. F. Colombel and A. Darfeuille-Michaud, Inhibitory effect of probiotic Escherichia coli strain Nissle 1917 on adhesion to and invasion of intestinal epithelial cells by adherent–invasive E. coli strains isolated from patients with Crohn's disease, Aliment. Pharmacol. Ther., 2003, 18, 45–56 CrossRef CAS PubMed.
- M. Carol, N. Borruel, M. Antolin, M. Llopis, F. Casellas, F. Guarner and J. R. Malagelada, Modulation of apoptosis in intestinal lymphocytes by a probiotic bacteria in Crohn's disease, J. Leukocyte Biol., 2006, 79, 917–922 CrossRef CAS PubMed.
- R. Curciarello, K. E. Canziani, I. Salto, E. B. Romero, A. Rocca, I. Doldan, E. Peton, S. Brayer, A. M. Sambuelli and S. Goncalves, Probiotic lactobacilli isolated from kefir promote down-regulation of inflammatory lamina propria t cells from patients with active IBD, Front. Pharmacol., 2021, 12, 658026 CrossRef CAS PubMed.
- H. Su, Q. Kang, H. Wang, H. Yin, L. Duan, Y. Liu and R. Fan, Effects of glucocorticoids combined with probiotics in treating Crohn's disease on inflammatory factors and intestinal microflora, Exp. Ther. Med., 2018, 16, 2999–3003 Search PubMed.
- S. Fujimori, A. Tatsuguchi, K. Gudis, T. Kishida, K. Mitsui, A. Ehara, T. Kobayashi, Y. Sekita, T. Seo and C. Sakamoto, High dose probiotic and prebiotic cotherapy for remission induction of active Crohn's disease, J. Gastroenterol. Hepatol., 2007, 22, 1199–1204 CrossRef PubMed.
- F. Turroni, V. Taverniti, P. Ruas-Madiedo, S. Duranti, S. Guglielmetti, G. A. Lugli, L. Gioiosa, P. Palanza, A. Margolles and D. Van Sinderen,
Bifidobacterium bifidum PRL2010 modulates the host innate immune response, Appl. Environ. Microbiol., 2014, 80, 730–740 CrossRef CAS PubMed.
- R. Al-Sadi, V. Dharmaprakash, P. Nighot, S. Guo, M. Nighot, T. Do and T. Y. Ma, Bifidobacterium bifidum enhances the intestinal epithelial tight junction barrier and protects against intestinal inflammation by targeting the Toll-like receptor-2 pathway in an NF-kappaB-independent manner, Int. J. Mol. Sci., 2021, 22, 8070 CrossRef CAS PubMed.
- S. H. Lee, S. Y. Cho, Y. Yoon, C. Park, J. Sohn, J. J. Jeong, B. N. Jeon, M. Jang, C. An, S. Lee, Y. Y. Kim, G. Kim, S. Kim, Y. Kim, G. B. Lee, E. J. Lee, S. G. Kim, H. S. Kim, Y. Kim, H. Kim, H. S. Yang, S. Kim, S. Kim, H. Chung, M. H. Moon, M. H. Nam, J. Y. Kwon, S. Won, J. S. Park, G. M. Weinstock, C. Lee, K. W. Yoon and H. Park,
Bifidobacterium bifidum, strains synergize with immune checkpoint inhibitors to reduce tumour burden in mice, Nat. Microbiol., 2021, 6, 277–288 CrossRef CAS PubMed.
- V. Andresen, J. Gschossmann and P. Layer, Heat-inactivated Bifidobacterium bifidum MIMBb75 (SYN-HI-001) in the treatment of irritable bowel syndrome: a multicentre, randomised, double-blind, placebo-controlled clinical trial, Lancet Gastroenterol., 2020, 5, 658–666 Search PubMed.
- L. Wang, L. Wang, P. Tian, B. Wang, S. Cui, J. Zhao, H. Zhang, L. Qian, Q. Wang, W. Chen and G. Wang, A randomised, double-blind, placebo-controlled trial of Bifidobacterium bifidum CCFM16 for manipulation of the gut microbiota and relief from chronic constipation, Food Funct., 2022, 13, 1628–1640 RSC.
- I. Speciale, R. Verma, F. Di Lorenzo, A. Molinaro, S.-H. Im and C. De Castro,
Bifidobacterium bifidum, presents on the cell surface a complex mixture of glucans and galactans with different immunological properties, Carbohydr. Polym., 2019, 218, 269–278 CrossRef CAS PubMed.
- R. Verma, C. Lee, E.-J. Jeun, J. Yi, K. S. Kim, A. Ghosh, S. Byun, C.-G. Lee, H.-J. Kang, C.-D. Jun, G. Jan, C.-H. Suh, Ju.-Y. Jung, J. Sprent and D. Rudra, Cristina De Castro,Antonio Molinaro, C. D. Surh and S.-H. Im, Cell surface polysaccharides of Bifidobacterium bifidum induce the generation of Foxp3+ regulatory T cells, Sci. Immunol., 2018, 3, eaat6975 CrossRef PubMed.
- S. Feng, J. Li, D. Qu, F. Tian, L. Yu, H. Zhang, W. Chen, J. Zhao and Q. Zhai, Characteristics of an in vitro mesenteric lymph node cell suspension model and its possible association with in vivo functional evaluation, Int. J. Mol. Sci., 2022, 23, 1003 CrossRef CAS PubMed.
- S. Wirtz, V. Popp, M. Kindermann, K. Gerlach, B. Weigmann, S. Fichtner-Feigl and M. F. Neurath, Chemically induced mouse models of acute and chronic intestinal inflammation, Nat. Protoc., 2017, 12, 1295–1309 CrossRef CAS PubMed.
- L. Wang, Z. Yu, S. Wan, F. Wu, W. Chen, B. Zhang, D. Lin, J. Liu, H. Xie and X. Sun, Exosomes derived from dendritic cells treated with Schistosoma japonicum soluble egg antigen attenuate DSS-induced colitis, Front. Pharmacol., 2017, 8, 651 CrossRef PubMed.
- Q. Zhai, S. Cen, P. Li, F. Tian, J. Zhao, H. Zhang and W. Chen, Effects of dietary selenium supplementation on intestinal barrier and immune responses associated with its modulation of gut microbiota, Environ. Sci. Technol. Lett., 2018, 5, 724–730 CrossRef CAS.
- Q. X. Zhai, T. Q. Li, L. L. Yu, Y. Xiao, S. S. Feng, J. P. Wu, J. X. Zhao, H. Zhang and W. Chen, Effects of subchronic oral toxic metal exposure on the intestinal microbiota of mice, Sci. Bull., 2017, 62, 831–840 CrossRef CAS.
- Y. Qi, L. Yu, F. Tian, J. Zhao, H. Zhang, W. Chen and Q. Zhai, A. muciniphila supplementation in mice during pregnancy and lactation affects the maternal intestinal microenvironment, Nutrients, 2022, 14, 390 CrossRef CAS PubMed.
- R. Zhai, X. Xue, L. Zhang, X. Yang, L. Zhao and C. Zhang, Strain-specific anti-inflammatory properties of two Akkermansia muciniphila strains on chronic colitis in mice, Front. Cell. Infect. Microbiol., 2019, 9, 239 CrossRef CAS PubMed.
- D. Srutkova, M. Schwarzer, T. Hudcovic, Z. Zakostelska, V. Drab, A. Spanova, B. Rittich, H. Kozakova and I. Schabussova, Bifidobacterium longum, CCM 7952 promotes epithelial barrier function and prevents acute DSS-induced colitis in strictly strain-specific manner, PLoS One, 2015, 10, e0134050 CrossRef PubMed.
- L. Wang, L. Hu, Q. Xu, B. Yin, D. Fang, G. Wang, J. Zhao, H. Zhang and W. Chen, Bifidobacterium adolescentis exerts strain-specific effects on constipation induced by loperamide in BALB/c mice, Int. J. Mol. Sci., 2017, 18, 318 CrossRef PubMed.
- G. Latella, J. Di Gregorio, V. Flati, F. Rieder and I. C. Lawrance, Mechanisms of initiation and progression of intestinal fibrosis in IBD, Scand. J. Gastroenterol., 2015, 50, 53–65 CrossRef CAS PubMed.
- M. Liu, X. Zhang, Y. Hao, J. Ding, J. Shen, Z. Xue, W. Qi, Z. Li, Y. Song and T. Zhang, Protective effects of a novel probiotic strain, Lactococcus lactis ML2018, in colitis: in vivo and in vitro evidence, Food Funct., 2019, 10, 1132–1145 RSC.
- F. Lombardi, F. R. Augello, P. Palumbo, E. Mollsi, M. Giuliani, A. M. Cimini, M. G. Cifone and B. Cinque, Soluble fraction from lysate of a high concentration multi-strain probiotic formulation inhibits TGF-β1-induced intestinal fibrosis on CCD-18Co cells, Nutrients, 2021, 13, 882 CrossRef CAS PubMed.
- X. Chen, Y. Fu, L. Wang, W. Qian, F. Zheng and X. Hou,
Bifidobacterium longum and VSL# 3® amelioration of TNBS-induced colitis associated with reduced HMGB1 and epithelial barrier impairment, Dev. Comp. Immunol., 2019, 92, 77–86 CrossRef CAS PubMed.
- M. A. Engevik, B. Herrmann, W. Ruan, A. C. Engevik, K. A. Engevik, F. Ihekweazu, Z. Shi, B. Luck, A. L. Chang-Graham and M. Esparza, Bifidobacterium dentium-derived y-glutamylcysteine suppresses ER-mediated goblet cell stress and reduces TNBS-driven colonic inflammation, Gut Microbes, 2021, 13, 1–21 CrossRef PubMed.
- A. Geirnaert, M. Calatayud, C. Grootaert, D. Laukens, S. Devriese, G. Smagghe, M. De Vos, N. Boon and T. Van de Wiele, Butyrate-producing bacteria supplemented in vitro to Crohn's disease patient microbiota increased butyrate production and enhanced intestinal epithelial barrier integrity, Sci. Rep., 2017, 7, 1–14 CrossRef CAS PubMed.
- H.-X. Shang, A.-Q. Wang, C.-H. Bao, H.-G. Wu, W.-F. Chen, L.-Y. Wu, R. Ji, J.-M. Zhao and Y. Shi, Moxibustion combined with acupuncture increases tight junction protein expression in Crohn's disease patients, World J. Gastroenterol., 2015, 21, 4986 CrossRef PubMed.
- W.-T. Kuo, L. Zuo, M. A. Odenwald, S. Madha, G. Singh, C. B. Gurniak, C. Abraham and J. R. Turner, The tight junction protein ZO-1 is dispensable for barrier function but critical for effective mucosal repair, Gastroenterology, 2021, 161, 1924–1939 CrossRef CAS PubMed.
- S. Tiwari, S. Begum, F. Moreau, H. Gorman and K. Chadee, Autophagy is required during high MUC2 mucin biosynthesis in colonic goblet cells to contend metabolic stress, Am. J. Physiol.: Gastrointest. Liver Physiol., 2021, 321, G489–G499 CrossRef CAS PubMed.
- L. Patnaude, M. Mayo, R. Mario, X. Wu, H. Knight, K. Creamer, S. Wilson, V. Pivorunas, J. Karman and L. Phillips, Mechanisms and regulation of IL-22-mediated intestinal epithelial homeostasis and repair, Life Sci., 2021, 271, 119195 CrossRef CAS PubMed.
- Y. Zhou, Z. Ruan, X. Zhou, X. Huang, H. Li, L. Wang, C. Zhang, Z. Deng, G. Wu and Y. Yin, Lactosucrose attenuates intestinal inflammation by promoting Th2 cytokine production and enhancing CD86 expression in colitic rats, Biosci., Biotechnol., Biochem., 2015, 79, 643–651 CrossRef CAS PubMed.
- S. B. Ghavami, A. Yadegar, H. A. Aghdaei, D. Sorrentino, M. Farmani, A. S. Mir, M. Azimirad, H. Balaii, S. Shahrokh and M. R. Zali, Immunomodulation and generation of tolerogenic dendritic cells by probiotic bacteria in patients with inflammatory bowel disease, Int. J. Mol. Sci., 2020, 21, 6266 CrossRef CAS PubMed.
- M. Saruta, Q. T. Yu, P. R. Fleshner, P.-Y. Mantel, C. B. Schmidt-Weber, A. H. Banham and K. A. Papadakis, Characterization of FOXP3+CD4+ regulatory T cells in Crohn's disease, Clin. Immunol., 2007, 125, 281–290 CrossRef CAS PubMed.
- M. P. Kuczma, E. A. Szurek, A. Cebula, B. Chassaing, Y.-J. Jung, S.-M. Kang, J. G. Fox, B. Stecher and L. Ignatowicz, Commensal epitopes drive differentiation of colonic Tregs, Sci. Adv., 2020, 6, eaaz3186 CrossRef CAS PubMed.
-
J.-E. Kim, C. S. Chae, G.-C. Kim, W. Hwang, J.-s. Hwang, S.-M. Hwang, Y. Kim, Y.-T. Ahn, S.-G. Park, C.-D. Jun, D. Rudra and S.-H. Im, Lactobacillus helveticus suppresses experimental rheumatoid arthritis by reducing inflammatory T cell responses, J. Funct. Foods, 2015, 13, 350–362 Search PubMed.
- W. Li, S. Hang, Y. Fang, S. Bae, Y. Zhang, M. Zhang, G. Wang, M. D. McCurry, M. Bae and D. Paik, A bacterial bile acid metabolite modulates Treg activity through the nuclear hormone receptor NR4A1, Cell Host Microbe, 2021, 29, 1366–1377 CrossRef CAS PubMed.
- C. H. Kim, Control of lymphocyte functions by gut microbiota-derived short-chain fatty acids, Cell. Mol. Immunol., 2021, 18, 1161–1171 CrossRef CAS PubMed.
- A. Metwaly, A. Dunkel, N. Waldschmitt, A. C. D. Raj, I. Lagkouvardos, A. M. Corraliza, A. Mayorgas, M. Martinez-Medina, S. Reiter, M. Schloter, T. Hofmann, M. Allez, J. Panes, A. Salas and D. Haller, Integrated microbiota and metabolite profiles link Crohn's disease to sulfur metabolism, Nat. Commun., 2020, 11, 4322 CrossRef PubMed.
- Y. Wang, X. Gao, X. Zhang, F. Xiao, H. Hu, X. Li, F. Dong, M. Sun, Y. Xiao and T. Ge, Microbial and metabolic features associated with outcome of infliximab therapy in pediatric Crohn's disease, Gut Microbes, 2021, 13, 1865708 CrossRef PubMed.
- D. O. Magro, A. Santos, D. Guadagnini, F. M. de Godoy, S. H. M. Silva, W. J. F. Lemos, N. Vitulo, S. Torriani, L. V. Pinheiro and C. A. R. Martinez, Remission in Crohn's disease is accompanied by alterations in the gut microbiota and mucins production, Sci. Rep., 2019, 9, 1–10 CrossRef CAS PubMed.
- L. Mayr, F. Grabherr, J. Schwärzler, I. Reitmeier, F. Sommer, T. Gehmacher, L. Niederreiter, G.-W. He, B. Ruder and K. T. Kunz, Dietary lipids fuel GPX4-restricted enteritis resembling Crohn's disease, Nat. Commun., 2020, 11, 1–15 CrossRef PubMed.
- L. K. Stenman, R. Holma, A. Eggert and R. Korpela, A novel mechanism for gut barrier dysfunction by dietary fat: epithelial disruption by hydrophobic bile acids, Am. J. Physiol.: Gastrointest. Liver Physiol., 2013, 304, G227–G234 CrossRef CAS PubMed.
- Y. Chen, H. Chen, J. Ding, C. Stanton, R. P. Ross, J. Zhao, H. Zhang, B. Yang and W. Chen,
Bifidobacterium longum ameliorates dextran sulfate sodium-induced colitis by producing conjugated linoleic acid, protecting intestinal mechanical barrier, restoring unbalanced gut microbiota, and regulating the Toll-Like receptor-4/nuclear factor-κB signaling pathway, J. Agric. Food Chem., 2021, 69, 14593–14608 CrossRef CAS PubMed.
- J.-W. Park, Y. Bu, J. Bae, B. Lee, S. Ko, J. Kim and B. Ryu, Protective effects of Ulmus macrocarpa on experimental colitis mice models, Orient. Pharm. Exp. Med., 2011, 11, 107–112 CrossRef.
- S. B. Hanauer, W. J. Sandborn and G. R. Lichtenstein, Evolving considerations for thiopurine therapy for inflammatory bowel diseases—a clinical practice update: commentary, Gastroenterology, 2019, 156, 36–42 CrossRef PubMed.
|
This journal is © The Royal Society of Chemistry 2023 |
Click here to see how this site uses Cookies. View our privacy policy here.