DOI:
10.1039/D2FO02910C
(Paper)
Food Funct., 2023,
14, 160-170
Identification, molecular docking, and protective effects on H2O2-induced HEK-293 cell oxidative damage of antioxidant peptides from Pacific saury (Cololabis saira)†
Received
29th September 2022
, Accepted 28th November 2022
First published on 7th December 2022
Abstract
We identified novel antioxidant peptides from Pacific saury (Cololabis saira). Enzymatic hydrolysates were isolated, purified, and identified by ultrafiltration, gel chromatography, reverse phase high-performance liquid chromatography (RP-HPLC), and matrix-assisted laser desorption/ionization-time of flight (MALDI-TOF) mass spectrometry (MS). Twenty putative peptides were identified from five components of HPLC, among which sixteen peptides were predicted to have good water solubility and non-toxicity by online tools. Fifteen peptides were successfully docked with myeloperoxidase, and we observed that Arg31, Arg323, and Lys505 played a key role in the antioxidant mechanism, with van der Waals forces and conventional hydrogen bonds as important interaction forces. Six identified peptides with lower CDOCKER energy values were synthesized to verify the antioxidant activity, and the results showed that the synthetic peptide QQAAGDKIMK displayed the strongest 2,2-diphenyl-1-picrylhydrazyl (DPPH) radical scavenging rate (31.05 ± 0.27%) and reducing power (0.29 ± 0.01). The synthetic peptide KDEPDQASSK at a concentration of 300 μg mL−1 exhibited the strongest protective effects on H2O2-induced oxidative damage of HEK-293 cells, with cell viability and ROS level of 0.38 ± 0.03 and 0.08 ± 0.01, respectively.
1. Introduction
Antioxidant peptides exert beneficial effects on health by inhibiting oxidative stress, which is known to promote certain chronic diseases.1 Marine fish protein-derived antioxidant peptides are currently the topic of interest in research due to their complex structure, variety, high activity, and fewer side effects. The peptides separated and purified from the enzymatic hydrolysate of fish skin gelatin exhibited high antioxidant activity and significantly improved the clinical symptoms of dextran sodium sulfate (DSS)-induced colitis in mice.2 Three peptides (WGGPK, GPP, and GVPLT) from the enzymatic hydrolysate of bluefin tuna head exhibited high antioxidant activity.3 Similarly, three peptides (YLMSR, VLYEE, and MILMR) were purified from the protein hydrolysate of croceine croaker (Pseudosciaena crocea) muscle and they exhibited strong antioxidant activity.4 However, to date, only a few marine protein-derived antioxidant peptides have been reported, and the discovery of new antioxidant peptides from seafood has attracted increasing attention.
Studies have demonstrated that the composition and order of amino acids, as well as the conformation of peptides, significantly affect the antioxidant capacity.5 Numerous studies have been conducted to separate and purify antioxidant peptides to obtain a single peptide fraction with improved antioxidant activity.6 The separation and purification techniques can be divided into ultrafiltration,7 electrophoresis,8 gel chromatography,9 and reversed-phase high-performance liquid chromatography (RP-HPLC)10 depending on the molecular weight, polarity, and isoelectric point of peptides. A combination of multiple separation and purification techniques not only results in higher purity bioactive peptides but also saves time, reduces costs, and achieves complementary advantages.11 Mass spectrometry is a commonly used method for identifying the amino acid sequences of bioactive peptides. In addition, the molecular weight and primary structures of the peptide can be obtained through mass spectrometry.12 The commonly used mass spectrometry techniques include LC-MS/MS,13 ESI-MS,14 and MALDI-TOF-MS.11
Although the exact antioxidant mechanism of peptides remains unclear, studies have shown that the amino acid type, sequence, and spatial structure of peptides significantly affect the interactions between peptides and receptors. Prediction of such interactions can be achieved by molecular docking. Molecular docking is a technique to model the intermolecular interactions and predict the mode of receptor–ligand binding, location of binding, and type of force, using chemometric methods based on the known steric structure of ligands and receptors.15 Molecular docking is usually used to explain the interactions between bioactive peptides and the receptor to better understand the interaction mechanisms.16 In recent years, myeloperoxidase (MPO) has often been used in studies of antioxidant activity of peptides as a molecular docking receptor.13 MPO is a peroxidase abundantly expressed and secreted by activated polymorphonuclear leukocytes (neutrophils). Because the extracellular MPO catalyzes the peroxidation of lipoproteins and other molecules in the blood, MPO is considered the major driving factor of lipid peroxidation in vivo.17
The antioxidant activity of peptides has been widely evaluated by using physiological experiments in vitro and cell models due to their low cost, high speed, and short cycle. HEK-293 cells are commonly used cell models. HEK-293 cells are a cell line derived from human embryonic kidney cells, with high transfection efficiency and ease of culture. Compared to tumor cells, the metabolic conditions are closer to those of normal human cells and therefore more realistically mimic oxidative stress in humans.18 Thus, HEK-293 cells are used to evaluate the antioxidant activity of peptides as a predictive in vitro model.
Pacific saury (Cololabis saira), one of the major economic marine fishes largely captured from the North Pacific Ocean, is favored by consumers due to its high nutritional value, which is attributed to the presence of essential amino acids and unsaturated fatty acids. However, to date, little information is available on its antioxidant peptides. Thus, we aimed to identify novel antioxidant peptides from the enzymatic hydrolysate of Pacific saury using multiple separation and purification techniques. The fractions were screened by measuring the 2,2-diphenyl-1-picrylhydrazyl (DPPH) radical scavenging rate and reducing power. The amino acid sequences of peptides were identified by matrix-assisted laser desorption/ionization-time of flight (MALDI-TOF) mass spectrometry (MS). Afterward, the physicochemical characteristics (water solubility and toxicity) of the identified peptides were predicted using online tools. Furthermore, the interaction between the identified peptides and the receptor MPO was investigated by molecular docking. Finally, putative antioxidant peptides were synthesized to verify their activities using the HEK-293 cell line. We believe the results of this study will fill the knowledge gap by discovering new antioxidant peptides from marine fish enzymatic extracts.
2. Materials and methods
2.1 Materials
2.1.1 Sample preparation.
Pacific saury was purchased from an aquatic market (Qingdao, China). The head, tail, gut, and bones were removed. The muscles were cleaned, ground into minced meat, and stored at −80 °C until use.
HEK-293 cells were purchased from the Center for Excellence and Innovation in Molecular and Cell Science, Chinese Academy of Sciences (Shanghai, China).
2.1.2 Chemicals.
DPPH was purchased from Feijing Biotechnology Co., Ltd (Fuzhou, China). Trichloroacetic acid and FeCl3 were purchased from Shanghai Aladdin Biochemical Technology Co., Ltd. The reagents used for RP-HPLC were of HPLC grade and purchased from Merck Drugs and Biotechnology Ltd (Beijing, China). All other reagents were of analytical grade and commercially available.
2.2 Preparation of the enzymatic hydrolysate from Pacific saury
The Pacific saury meat was thawed and hydrolyzed using neutral protease under the following conditions: material-to-liquid ratio of 1
:
2.67 (m/v, g mL−1); enzyme addition amount of 0.6% (enzyme/substrate, g g−1); enzymatic hydrolysis time of 4 h at 50 °C and pH of 7.0. The enzymatic hydrolysate was boiled for 20 min to inactivate the enzyme, and the filtrate was centrifuged for 20 min at 4 °C (Biofuge Stratos; Heraeus Instruments, Hanau, Germany) at 8000 rpm. The supernatant was collected, lyophilized (Labconco freeze-dry system; Labconco Corp., MO, USA), and stored at −80 °C until use.
2.3 Separation and purification of antioxidant peptides by ultrafiltration
The lyophilized samples were dissolved in ultrapure water and fractionated through an ultrafiltration membrane (Sartorius Corporation, Gottingen, Germany) with molecular weight cutoff sizes of 10
000 and 3000 Da. The fractions were concentrated and lyophilized for measuring the DPPH radical scavenging rate and reducing power (the sample concentration was 15 mg mL−1 and the enzymatic hydrolysate was used as the control). The fraction with the highest antioxidant activity was selected for further separation and purification by gel chromatography.
2.4 Separation and purification of antioxidant peptides by gel chromatography
The sample was dissolved in ultrapure water and separated and purified using a Sephadex G-25 gel chromatography column (1.6 × 70 cm; Solarbio Corp., Beijing, China). The separation was performed at 25 °C at a flow rate of 1 mL min−1, with ultrapure water as the eluent. The sample loading volume was 2 mL, and the sample concentration was 20 mg mL−1. The detection wavelength was 214 nm. The component collector collected one tube of 4 mL per tube every 3 min.19 Different fractions were collected, concentrated, and lyophilized to measure the DPPH radical scavenging rate and reducing power (the sample concentration was 10 mg mL−1, and the enzymatic hydrolysate with a molecular weight less than 3000 Da was used as the control). The fraction with the highest antioxidant activity was selected for further separation and purification by RP-HPLC.
2.5 Separation and purification of antioxidant peptides by RP-HPLC
The chromatographic conditions were as follows: YMC-Pack ODS-AQ C18 column (10 × 250 mm, 5 μm; YMC Corporation, Kyoto, Japan). Mobile phase A consisted of acetonitrile, and mobile phase B consisted of ultrapure water with 0.1% (v/v) trifluoroacetic acid. The flow rate was 1 mL min−1. The elution time was 50 min. The elution peak was detected at 214 nm. The sample loading volume was 100 μL. Isocratic elution was performed (10% A, 90% B). All fractions were collected, concentrated, lyophilized, and stored at −80 °C until use.
2.6 Determination of the DPPH radical scavenging rate
The DPPH radical scavenging rate was determined using the following method which was performed in triplicate.20 The DPPH radical scavenging rate was calculated using eqn (1): |
 | (1) |
where ODsample, ODblank, and ODcontrol are absorbance values of the sample group, blank group, and control group, respectively.
2.7 Determination of the reducing power
The reducing power was determined using the following method which was performed in triplicate.21
The experimental group: 2 mL PBS (0.2 mol L−1 pH 6.6), 2 mL 1% K3[Fe(CN)6], and 2 mL sample (20 mg mL−1) were mixed evenly, then bathed at 50 °C for 20 min, 2 mL 10% TCA was added, centrifuged (5000 rpm, 4 °C), and 2 mL supernatant was taken. 2 mL distilled water and 0.4 mL 0.1% FeCl3 were added and the absorbance values at 700 nm after 10 min were measured.
Control group: An equal amount of distilled water was used instead of the sample.
2.8 Identification of antioxidant peptides by MALDI-TOF/TOF MS
The amino acid sequences of peptides were identified by MADIL-TOF/TOF MS. The mass spectrometry acquisition parameters were as follows: positive ion reflection mode, molecular weight range of 700–3500 Da for primary mass spectra and 40–1050 Da for secondary mass spectra.22
2.9 Physicochemical characteristic prediction of identified peptides
Prot Param (https://web.expasy.org/protparam/) was used to predict the water solubility of identified peptides based on the grand average of hydropathicity (GRAVY). Toxin Pred (https://webs.iiitd.edu.in/raghava/toxinpred/multi_submit.php) was used to predict the toxicity of identified peptides.
2.10 Molecular docking of antioxidant peptides with the receptor MPO
The CDOCKER module in the Discovery Studio 2017 R2 (Accelrys, CA, USA) software was used to perform the molecular docking of 16 identified peptides with good water solubility and non-toxicity and the receptor MPO. Docking allowed to explore the interaction mechanism between the identified peptides and the receptor MPO. The crystal complex structure of the receptor MPO (PDB ID: 3F9P) at a resolution of 2.93 Å was downloaded from the RCSB Protein Data Bank.23 The performance parameters were set as per the method.13
2.11 Synthesis of identified peptides and verification of activities
The six antioxidant peptides screened by molecular docking were synthesized using the Fmoc solid-phase method by Qiang Yao Biotechnology Co., Ltd (Hubei, China) with a purity of over 98%. Antioxidant activities of synthetic peptides were verified by measuring the DPPH radical scavenging rate, reducing power, protective effects on H2O2-induced oxidative damage to HEK-293 cells, and ROS levels. The enzymatic hydrolysate from Pacific saury with a molecular weight less than 3000 Da was used as the control.
The experiment model for H2O2-induced oxidative damage in HEK-293 cells was established according to the method.24 The protective effects of synthetic peptides on H2O2-induced oxidative damage to HEK-293 cells were determined according to the method.25 Cell viability was measured using the CCK-8 kit. The ROS levels were determined using a DCFH-DA fluorescence probe.26
2.12 Statistical analysis
The significance of data was analyzed using analysis of variance with IBM SPSS Statistics 25.0 (SPSS Inc., IL, USA) and tested by Duncan's method with a 95% confidence level (P < 0.05), (A, B, C, …) and (a, b, c, …) represent significant differences among different components, the same as below. The results are expressed as mean ± standard deviation. Figures were drawn using Origin lab Origin 2021b (Origin Lab Co., MA, USA).
3. Results and discussion
3.1 Separation, purification, and in vitro antioxidant activities of separation fractions
Ultrafiltration is a membrane separation and purification technology that is driven by a pressure difference and depends on the size of molecules to be retained.16 Ultrafiltration is widely used for the preliminary separation and purification of antioxidant peptides under mild conditions and high separation volumes.27 DPPH radical scavenging rates and reducing powers of different ultrafiltrate fractions are shown in Fig. 1A. The DPPH radical scavenging rates of fractions with a molecular weight less than 3000 Da (61.08 ± 2.99%) and greater than 10
000 Da (60.23 ± 2.91%) were significantly higher than that of the control group (52.71 ± 1.07%), whereas DPPH radical scavenging rates of the fraction with molecular weights ranging from 3000 to 10
000 Da (38.92 ± 3.07%) were significantly lower than that of the control group. The reducing powers of the fraction with a molecular weight less than 3000 Da was 0.33 ± 0.01, which was significantly higher than that of the control group (0.28 ± 0.01) and the other two fractions with a molecular weight ranging from 3000 to 10
000 Da (0.25 ± 0.01) and greater than 10
000 Da (0.29 ± 0.01). Therefore, the fraction with a molecular weight less than 3000 Da was selected for further separation and purification by gel chromatography. The fractions with a molecular weight less than 3000 Da displayed higher antioxidant activity compared with other fractions. This could be due to the fact that small-molecule peptides are exposed to more active groups and more contact sites with free radicals and metal ions.3
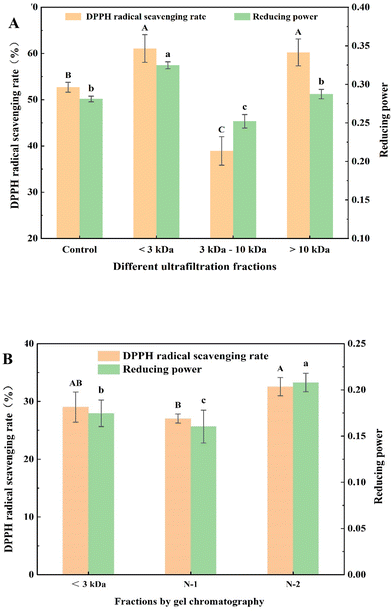 |
| Fig. 1 (A) DPPH radical scavenging rates and reducing powers of ultrafiltration fractions. Three fractions (<3 kDa, 3 kDa–10 kDa, >10 kDa) were separated from the enzymatic hydrolysate of Pacific saury by ultrafiltration. The enzymatic hydrolysate was used as control. The DPPH radical scavenging rates and reducing powers of four components (control, <3 kDa, 3 kDa–10 kDa, >10 kDa, the concentration of sample was 20 mg mL−1) were determined. Different capital letters represent significant differences in DPPH radical scavenging rate of each component (P < 0.05); different lower letters represent significant differences in reducing power of each component (P < 0.05). (B) DPPH radical scavenging rates and reducing powers of gel chromatography fractions. The ultrafiltration components (components with a molecular weight less than 3 kDa) were further separated and purified by gel chromatography. Two components were obtained, named N-1 and N-2, respectively. The DPPH radical scavenging rates and reducing powers of three components (<3 kDa, N-1, and N-2, the concentration of sample was 10 mg mL−1) were determined. Different capital letters represent significant difference in DPPH radical scavenging rate of each component (P < 0.05); different lower letters represent significant differences in reducing power of each component (P < 0.05). | |
Gel chromatography uses the special network structure of gels to separate and purify substances of different molecular weights. Components with higher molecular weights are associated with shorter permeation paths in the packing and shorter residence time in the column, resulting in separation and purification.16Fig. 2A shows the gel chromatography separation and purification results of the fraction with a molecular weight less than 3000 Da. Two chromatographic peaks were obtained, and the two fractions, namely, N-1 and N-2 were collected for assessing the in vitro antioxidant activities. The results (Fig. 1B) showed that the DPPH radical scavenging rates and reducing powers of the N-2 fraction (32.56 ± 1.59% and 0.21 ± 0.01, respectively) were significantly higher than those of N-1 (27.04 ± 0.79% and 0.16 ± 0.02, respectively) and the control group (29.02 ± 2.62% and 0.18 ± 0.01, respectively). Therefore, fraction N-2 was selected for further separation and purification by RP-HPLC.
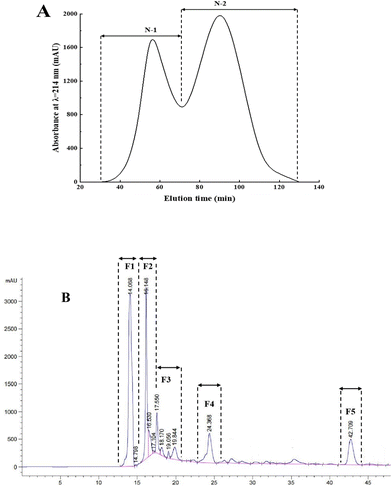 |
| Fig. 2 (A) Gel chromatography separation profile. The ultrafiltration component (components with a molecular weight less than 3 kDa) was dissolved in ultrapure water and separated and purified using a Sephadex G-25 gel chromatography column. The conditions of gel chromatography were as follows: the sample loading volume was 2 mL, the sample concentration was 20 mg mL−1, the flow rate was 1 mL min−1, the detection wavelength was 214 nm, and the component collector collected one tube of 4 mL per tube every 3 min. Two components were obtained, named N-1 and N-2, respectively. (B) RP-HPLC separation profile. The gel chromatography component (N-2) was dissolved in ultrapure water and separated and purified using an YMC-Pack ODS-AQ C18 column. The chromatographic conditions were as follows: mobile phase A consisted of acetonitrile, and mobile phase B consisted of ultrapure water with 0.1% (v/v) trifluoroacetic acid. The flow rate was 1 mL min−1. The elution time was 50 min. The elution peak was detected at 214 nm. The sample loading volume was 100 μL. Isocratic elution was performed (10% A, 90% B). Five components were obtained, named F1, F2, F3, F4, and F5, respectively. | |
Fig. 2B shows that the five antioxidant fractions separated by RP-HPLC were F1, F2, F3, F4, and F5, with retention times of 14.068, 16.148, 17.550, 24.368, and 42.709 min, respectively. To obtain the maximum number of novel peptides with high antioxidant activity, the five main peak components were collected and lyophilized. Next, the amino acid sequences of peptides were identified by MALDI-TOF/TOF MS.
3.2 Identification of antioxidant peptides
MS/MS spectra of identified peptides are shown in ESI Fig. S1.† A total of 20 novel peptides were obtained from F1, F2, F3, and F4 by matching the sequence of the Pacific saury protein in the database. The MS/MS spectra of F5 did not match the corresponding amino acid sequences, which could be attributed to the low purity or contents of peptides, resulting in low ion abundance, and thus, a database search could not be performed.28Table 1 shows amino acid sequences and molecular weights of identified peptides, including 2 octapeptides, 6 ninth peptides, 10 tenth peptides, and 2 eleventh peptides. Several studies have reported that the molecular weight exerts a significant influence on the antioxidant activity of peptides, and peptides with molecular weights between 500 and 1800 Da usually had a higher antioxidant activity.29 The molecular weights of identified peptides in this study were between 900 and 1150 Da, indicating high antioxidant activity.
Table 1 Sequences, physicochemical property prediction, and molecular docking energy of identified peptides
Group |
Sequences |
Length |
Molecular weight |
Water solubility (GRAVY) |
Toxicity |
Energy (kcal mol−1) |
Interaction energy (kcal mol−1) |
F1-1 |
VPAMQRTMR |
9 |
1089.34 |
Good (−0.556) |
Non-toxicity |
−68.788 |
−78.575 |
F1-2 |
VNLTDVSQTK |
10 |
1104.21 |
Good (−0.440) |
Non-toxicity |
−131.086 |
−98.621 |
F1-3 |
ADPDAAHRPR |
10 |
1105.17 |
Good (−1.700) |
Non-toxicity |
— |
— |
F1-4 |
VALSNCNGLR |
10 |
1046.21 |
Poor (0.340) |
Non-toxicity |
— |
— |
F1-5 |
VLVLDEFNR |
9 |
1104.26 |
Poor (0.422) |
Non-toxicity |
— |
— |
F2-1 |
VLVQPSNR |
8 |
912.05 |
Good (−0.212) |
Non-toxicity |
−95.314 |
−88.397 |
F2-2 |
RNFNDAPDR |
8 |
912.13 |
Good (−2.222) |
Non-toxicity |
−113.212 |
−104.093 |
F2-3 |
KQASAQTGVSK |
9 |
1104.13 |
Good (−0.882) |
Non-toxicity |
−116.246 |
−103.107 |
F2-4 |
SSSHSFTPKK |
11 |
1104.22 |
Good (−1.370) |
Non-toxicity |
−131.256 |
−57.114 |
F2-5 |
VIVKNALR |
10 |
1105.20 |
Poor (0.825) |
Non-toxicity |
— |
— |
F3-1 |
NGLNRTFVGK |
10 |
1105.25 |
Good (−0.610) |
Non-toxicity |
−145.742 |
−110.862 |
F3-2 |
VDALEKSNTK |
10 |
1104.21 |
Good (−1.000) |
Non-toxicity |
−139.408 |
−113.225 |
F3-3 |
NPMIEKNNK |
9 |
1087.25 |
Good (−1.889) |
Non-toxicity |
−134.689 |
−98.431 |
|
F3-4 |
QQAAGDKIMK |
10 |
1089.27 |
Good (−0.870) |
Non-toxicity |
−145.573 |
−105.228 |
F3-5 |
KMLIEVNDK |
9 |
1089.31 |
Good (−0.433) |
Non-toxicity |
−142.248 |
−117.244 |
F4-1 |
QQLLIGAYAK |
10 |
1104.30 |
Poor (0.310) |
Non-toxicity |
— |
— |
F4-2 |
QLEEENKSK |
9 |
1104.17 |
Good (−2.478) |
Non-toxicity |
−190.113 |
−120.953 |
F4-3 |
DAPVDQMGAGK |
11 |
1088.19 |
Good (−0.645) |
Non-toxicity |
−175.828 |
−126.084 |
F4-4 |
KDEPDQASSK |
10 |
1104.13 |
Good (−2.320) |
Non-toxicity |
−149.875 |
−104.062 |
F4-5 |
IGVLDEGKMK |
10 |
1089.31 |
Good (−0.120) |
Non-toxicity |
−121.360 |
−98.064 |
3.3 Physicochemical property prediction of the identified peptides
The length of peptide chains, composition, and hydrophobic/hydrophilic properties of amino acids affect the physiochemical characteristics and antioxidant activity of the peptides. Physiochemical property prediction of peptides through an online tool enables preliminary screening of peptides.30Table 1 shows the physiochemical property prediction results of the identified peptides. Different amino acids have different hydrophobic values, in which the GRAVY value can reflect the water solubility of peptides. A GRAVY score of less than 0 indicates good water solubility of peptides. In contrast, water solubility may be poor.31 Peptide GRRRRSVQWCA, identified and screened from human lactoferrin, displayed a remarkable ability to inhibit the receptor MPO activity. In addition, the reference peptide had a GRAVY score of −1.373, indicating good water solubility.32 The GRAVY score of identified peptides VALSNCNGLR, VLVLDEFNR, VIVKNALR, and QQLLIGAYAK was greater than 0; therefore, the four identified peptides with poor water solubility were discarded. Prediction results of toxicity showed that the identified peptides were non-toxic to organisms. Finally, 16 identified peptides with a GRAVY score lower than 0 were selected for molecular docking to investigate their potential antioxidant activity and interactions with the receptor MPO.
3.4 Molecular docking of identified peptides with MPO
Molecular docking results and a two-dimensional (2D) graph of interactions are shown in ESI Fig. S2.† Fifteen identified peptides were successfully docked with receptor MPO. The key amino acid residues around the active sites in MPO during molecular docking are shown in Table 2. Twenty-four amino acid residues were involved in molecular docking. The binding sites of identified peptides with receptor MPO primarily included Arg31, Arg323, Cys153, Ile160, Lys505, Phe439, Pro34, Thr159, and Trp32. The top three amino acid residues in the binding sites were Arg31, Arg323, and Lys505, indicating them to be the most predominant binding amino acid residues that played an important role in the interaction of identified peptides with receptor MPO. Study have investigated the molecular docking sites of abalone antioxidant peptides with receptor MPO and reported that amino acid residues Ala152, Ala28, Arg31, Arg323, Cys153, Ile160, Lys505, Phe29, Thr159, Trp32, and Val30 generated interaction forces on receptor MPO.13 The same amino acid residues were reported between their findings and ours in our study, namely, Arg31, Arg323, Cys153, Ile160, Lys505, Thr159, and Trp32, indicating that these amino acid residues played a key role in the binding between antioxidant peptides and receptor MPO.
Table 2 The key amino acid residues around active site in MPO during molecular docking
Binding site |
F1-1 |
F1-2 |
F2-1 |
F2-2 |
F2-3 |
F2-4 |
F3-1 |
F3-2 |
F3-3 |
F3-4 |
F3-5 |
F4-2 |
F4-3 |
F4-4 |
F4-5 |
“+” indicates the number of interactions between the ligand and the receptor. |
Ala152 |
|
|
|
+ |
|
+ |
|
+ |
|
+ |
|
|
|
|
|
Ala28 |
|
|
|
+ |
|
|
|
|
|
|
|
|
|
|
|
Ala35 |
|
+ |
|
+ |
|
|
|
|
|
|
|
|
|
|
|
Arg161 |
|
|
|
|
|
|
+ |
|
|
|
|
|
|
|
++ |
Arg31 |
++++++ |
+++++ |
++++ |
++++++ |
++++ |
+++++ |
+++++ |
+++++ |
++ |
+++++ |
+++++ |
++++ |
+++++ |
+++++++ |
+++++ |
Arg323 |
+ |
+ |
++ |
+ |
+ |
+++++ |
+++ |
++ |
+++ |
+++ |
++++ |
++++ |
+ |
++++++ |
+ |
Arg438 |
|
|
|
+ |
++ |
|
|
|
|
|
+ |
|
|
|
|
Asn162 |
+ |
|
|
|
|
++ |
+ |
+++ |
+ |
|
++ |
+ |
|
+ |
++ |
Asp321 |
|
|
|
+++ |
+ |
|
|
|
|
|
|
+ |
+ |
|
|
Cys153 |
+ |
|
+ |
++ |
+ |
++ |
++ |
+ |
+++ |
+ |
|
|
|
|
++ |
Gly155 |
|
|
|
|
|
|
+ |
|
|
|
|
|
|
|
|
Ile160 |
+ |
++ |
+ |
++ |
++++ |
+ |
++ |
++ |
++ |
|
+ |
|
+ |
+ |
+ |
Leu33 |
|
|
++ |
+ |
+ |
|
|
+ |
+ |
++ |
|
|
|
|
+ |
|
Lys505 |
+ |
|
+ |
++ |
|
+++ |
++ |
++ |
|
++ |
|
++++ |
++ |
+++ |
++++ |
Phe29 |
+ |
+++ |
|
|
|
++ |
++ |
++ |
|
++ |
|
|
+ |
|
|
Phe41 |
|
|
|
|
|
|
+ |
|
|
|
|
|
|
|
+ |
Phe439 |
|
+ |
++++ |
++ |
|
|
+ |
+ |
+ |
+ |
+ |
+ |
+++++ |
+ |
+ |
Pro34 |
++ |
+ |
++ |
|
+ |
|
++ |
+ |
+ |
++ |
++ |
+ |
+ |
+ |
+ |
Ser156 |
++ |
|
|
|
|
|
++ |
|
|
|
|
|
|
|
+ |
Ser319 |
|
+ |
|
|
|
|
|
|
|
++ |
+ |
|
|
|
|
Ser42 |
|
|
|
|
|
|
+ |
|
|
|
|
|
|
|
|
Thr159 |
+ |
++ |
++ |
+ |
+++ |
+ |
++ |
+ |
++ |
+ |
|
+ |
|
|
+ |
Trp32 |
|
+ |
|
+ |
+++ |
+++ |
++ |
+ |
++ |
++ |
|
+++ |
+++ |
++ |
|
Val30 |
|
|
|
+ |
|
|
|
|
+ |
|
++ |
|
|
+ |
|
Val320 |
|
|
|
|
|
|
|
+ |
|
|
|
|
|
|
|
The docking energy values of identified peptides and MPO are shown in Table 1. The results showed that the identified peptides NGLNRTFVGK (−145.742 kcal mol−1), QQAAGDKIMK (−145.573 kcal mol−1), KMLIEVNDK (−142.248 kcal mol−1), QLEEENKSK (−190.113 kcal mol−1), DAPVDQMGAGK (−175.828 kcal mol−1), and KDEPDQASSK (−149.875 kcal mol−1) had lower CDOCKER energy values compared with other identified peptides, indicating that the six identified peptides docked more tightly with the receptor MPO. In addition, molecular docking results showed that the interaction energy value of reference peptide GRRRRSVQWCA with receptor MPO was −10.202 kcal mol−1.33 The CDOCKER energy of the synthetic peptide and receptor MPO in molecular docking was lower than −10.202 kcal mol−1. The lower the CDOCKER energy, the closer the binding, the higher the potential activity. Therefore, the six identified peptides showed a higher capacity to inhibit the production of oxidative stress in the body.
We further explored the docking mechanism of antioxidant peptides by analyzing the interaction forces and the corresponding binding amino acid residues (Fig. S2†). The results showed that 28 van der Waals forces (Arg323, Asn162, Cys153, etc.), 2 attractive charge interactions (Arg323 and Lys505), 14 conventional hydrogen bonds (Asn162, Arg31, Arg323, etc.), 7 carbon–hydrogen bonds (Arg31, Ile160, Phe29, etc.), and 7 alkyl/pi-alkyl interactions (Arg31, Ile160, Phe439, etc.) were involved in the docking of identified peptides and MPO. Study has demonstrated that interactions between sea cucumber antioxidant peptides and receptor MPO and reported interactions involving 27 van der Waals forces (Arg323, Asn162, Cys153, etc.), 4 attractive charge interactions (Asp321, Arg323, Lys308, and Lys505), 7 hydrogen bonds (Arg31, Arg323, Asn162, etc.), 5 carbon–hydrogen bonds (Cys153, Leu33, Pro34, etc.), and 11 alkyl/pi-alkyl interactions (Arg31, Ile160, Phe439, etc.).32 Another study has demonstrated that the combination between false abalone peptides and receptor MPO and found that the interaction forces included two attractive charge interactions (Arg323 and Lys505), two hydrogen bonds (Arg31 and Ile160), four carbon–hydrogen bonds (Phe29, Thr159, Trp32, and Val30), and three alkyl/pi-alkyl interactions (Ala28, Ala152, and Cys153).13 The interaction forces and corresponding binding amino acid residues between the identified peptides from Pacific saury and receptor MPO were similar to the previous findings, indicating that the six identified peptides had potential antioxidant activity.
3.5
In vitro antioxidant activity of synthetic peptides
Fig. 3A shows the DPPH radical scavenging rate and reducing power of six synthetic peptides at 2 mg mL−1. The results showed that the synthetic peptide QQAAGDKIMK had the strongest DPPH radical scavenging rate (31.05 ± 0.27%) and reducing power (0.29 ± 0.01). Furthermore, synthetic peptides QLEEENKSK and DAPVDQMGAGK showed stronger DPPH radical scavenging rates than the control group, the values were 19.93 ± 1.94% and 24.65 ± 0.48%, respectively. Study has demonstrated that glutamine, a precursor of reduced glutathione, contributes to the synthesis of glutathione, and thus has high antioxidant activities in vitro, and glutamine peptides had similar properties as glutathione-stabilized alternatives.34 The above three peptides contained glutamine, which contributes to their strong antioxidant activities in vitro.
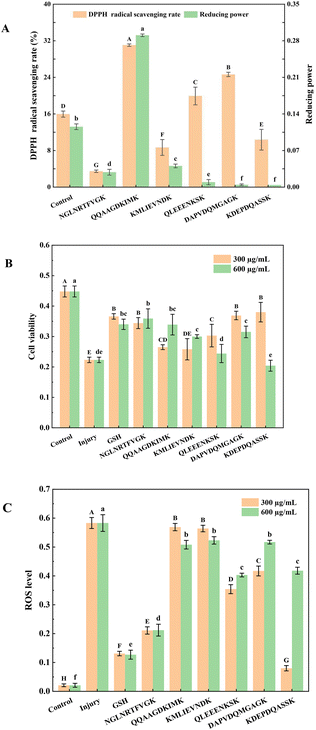 |
| Fig. 3 (A)In vitro antioxidant activity of synthetic peptides. DPPH radical scavenging rates and reducing powers of the enzymatic hydrolysate (components with a molecular weight less than 3 kDa) and six synthetic peptides were determined at 2 mg mL−1. Different capital letters represent significant differences in DPPH radical scavenging rate of each component (P < 0.05); different lower letters represent significant differences in reducing power of each component (P < 0.05). (B) Protective effects of synthetic peptides on H2O2-induced damage of HEK-293 cells. HEK-293 cells were cultured by using the DMEM medium. The cell model was constructed using H2O2. Cell viability of the enzymatic hydrolysate (components with a molecular weight less than 3 kDa), H2O2, GSH, and six synthetic peptides were determined at 300 μg mL−1 and 600 μg mL−1 by using the CCK-8 kit. Different capital and lower letters represent significant difference in cell viability of each component at 300 μg mL−1 and 600 μg mL−1, respectively (P < 0.05). (C) Inhibitory effects of synthetic peptides on H2O2-induced ROS levels in HEK-293 cells. HEK-293 cells were cultured by using the DMEM medium. The cell model was constructed by using H2O2. ROS levels of the enzymatic hydrolysate (components with a molecular weight less than 3 kDa), H2O2, GSH, and six synthetic peptides were determined at 300 μg mL−1 and 600 μg mL−1 by using a flow cytometer. Different capital and lower letters represent significant difference in ROS levels of each component at 300 μg mL−1 and 600 μg mL−1, respectively (P < 0.05). | |
The synthetic peptides QLEEENKSK and KMLIEVNDK displayed moderate DPPH radical scavenging rates and reducing powers, which could be related to the sequences of LEEE and LIE. Ile (I) and Leu (L) are hydrophobic amino acids with a certain antioxidant capacity, whereas Glu (E) is an acidic amino acid that can bind metal ions through the amino group or carbonyl group on the side chain group to exert antioxidant effects.
Hydrophobic amino acids, such as Val (V), Pro (P), and His (R), and aromatic amino acids, including Phe (F), Gly (G), Asp (D), and Asn (N), have strong radical scavenging rates. The proportion of these amino acids in the synthetic peptide NGLNRTFVGK was 70%; however, the DPPH radical scavenging rate and reducing power were weak. Peptides rich in amino acids with antioxidant activity exhibit a higher antioxidant activity; however, this feature is not absolute. For instance, study has demonstrated that peptides ARK and AR had strong antioxidant activity; however, peptides RK and RKA did not exhibit any antioxidant activity.35 Therefore, the arrangement of amino acids in peptides is another key factor affecting their antioxidant activity.
The DPPH radical scavenging rate and reducing power do not reflect the whole antioxidant activity of peptides due to the diversity of antioxidant mechanisms. To comprehensively explore the antioxidant activity of the synthetic peptides, further experiments were conducted using a H2O2-induced HEK-293 cell oxidative damage model.
3.6 Protective effects of synthetic peptides on H2O2-induced HEK-293 cell damage
In the current research, we selected HEK-293 cells to establish a model of oxidative damage with glutathione (GSH) as the positive control. The results (Fig. 3B) showed that GSH effectively protected HEK-293 cells from oxidative damage by H2O2, with a cell viability of 0.37 ± 0.01. The synthetic peptides NGLNRTFVGK, DAPVDQMGAGK, and KDEPDQASSK showed similar protective effects to GSH at a concentration of 300 μg mL−1, with cell viabilities of 0.35 ± 0.02, 0.37 ± 0.01, and 0.38 ± 0.03, respectively. Moreover, synthetic peptides NGLNRTFVGK and QQAAGDKIMK exhibited similar protective effects to GSH at a concentration of 600 μg mL−1, with cell viabilities of 0.36 ± 0.03 and 0.34 ± 0.03, respectively. The results demonstrated that synthetic peptides effectively prevented oxidative damage to HEK-293 cells. In addition, the protective effects of synthetic peptides QLEEENKSK, DAPVDQMGAGK, and KDEPDQASSK on HEK-293 cells did not increase in a concentration-dependent manner, which could be attributed to the toxic effects of high concentrations of synthetic peptides on HEK-293 cells.36 The synthetic peptides exerted stronger protective effects on H2O2-induced oxidative damage in HEK-293 cells at appropriate concentrations; however, the antioxidant mechanism needs to be investigated further.
3.7 Inhibitive effects of synthetic peptides on cellular ROS levels
ROS can oxidize intracellular macromolecules and disrupt their normal functions, leading to cell damage and even apoptosis.37 The ROS flow cytograms are shown in Fig. S3† and the inhibitory effects of synthetic peptides on H2O2-induced ROS levels in HEK-293 cells are shown in Fig. 3C. The ROS level in the injury group (0.58 ± 0.02) was significantly higher than that in the control group (0.02 ± 0.01) (P < 0.05), indicating that HEK-293 cells were severely injured by H2O2 oxidation. The ROS level in the GSH group was 0.13 ± 0.01, indicating that GSH effectively protected HEK-293 cells from oxidative damage.38 In addition, the synthetic peptide NGLNRTFVGK effectively reduced H2O2-induced ROS levels in HEK-293 cells, with a ROS level of 0.21 ± 0.01. The synthetic peptide KDEPDQASSK exhibited the lowest ROS level among other groups at 300 μg mL−1, with a ROS level of 0.08 ± 0.01, indicating that the antioxidant mechanism of synthetic peptides NGLNRTFVGK and KDEPDQASSK reduced the cellular ROS levels. Studies mentioned that amino acid sequence ED improved the antioxidant activity of peptides; however, independent amino acid sequence ED had no antioxidant activity.39 Therefore, the antioxidant activity of the synthetic peptide KDEPDQASSK could be due to the acidic amino acid ED in the sequence. Similarly, with an increase in the concentration of the synthetic peptide KDEPDQASSK, the cellular ROS level significantly reduced, which could be due to the cytotoxicity of the peptide at high concentrations.
Although synthetic peptides NGLNRTFVGK and KDEPDQASSK significantly inhibited oxidative damage by reducing the cellular ROS levels, DPPH radical scavenging rates, and reducing powers were lower than those of other peptides. This inconsistency could be attributed to different antioxidant mechanisms. Similarly, synthetic peptides QQAAGDKIMK and DAPVDQMGAGK exerted strong protective effects against H2O2-induced oxidative damage; however, the ROS levels did not decrease significantly, indicating that they protected HEK-293 cells from H2O2 oxidative damage through scavenging radicals rather than by reducing cellular ROS levels. Study has demonstrated that the peptide VYLPR exhibited stronger protective effects on H2O2-induced HEK-293 cell damage and higher ORAC values than other peptides; however, its ABTS radical scavenging activity was lower than peptides VEVYLPR and VVEVYLPR.40
The intracellular environment is highly complex and dynamic, and the protective effects of peptides on cell oxidative damage are multifaceted. From the current study, it could be speculated that the synthetic peptides attenuated oxidative damage by scavenging radicals and reducing ROS levels. For the role of other mechanisms that can attenuate oxidative damage, such as SOD, CAT, and LDH, further research is warranted in the future.
4. Conclusions
The enzymatic hydrolysate from Pacific saury was separated and purified using ultrafiltration, gel chromatography, and RP-HPLC. A total of 20 novel peptides were identified by MALDI-TOF/TOF MS, of which 16 peptides were predicted to have good water solubility and non-toxicity, and 15 were successfully docked with receptor MPO. Molecular docking results showed that the key binding amino acid residues primarily included Arg31, Arg323, and Lys505, and the major interaction forces involved were van der Waals forces and conventional hydrogen bonds. The identified peptides NGLNRTFVGK, QQAAGDKIMK, KMLIEVNDK, QLEEENKSK, DAPVDQMGAGK, and KDEPDQASSK displayed lower CDOCKER energy values. The synthetic peptide QQAAGDKIMK exhibited the strongest antioxidant activity in vitro, and synthetic peptides NGLNRTFVGK, QQAAGDKIMK, DAPVDQMGAGK, and KDEPDQASSK exerted significant protective effects on H2O2-induced oxidative damage in HEK-293 cells. After that, western blotting will be used to study the expression of antioxidant peptides in cells and specific cellular pathways.
Author contributions
Xuepeng Li and Jianrong Li designed the study and revised the manuscript. Junjia Luan and Lei Wang performed all the research including the data analysis and wrote the manuscript. Junnan Yang and Jinxiang Wang partly performed the research experiments of isolation and identification. Fangchao Cui and Hongbo Mi partly performed the research experiments of molecular docking. Yongxia Xu and Shumin Yi partly performed the research experiments of cell viability and ROS production.
Conflicts of interest
There are no conflicts of interest to declare.
Acknowledgements
This study was supported by the National Key Research and Development Program of China (2019YFD0901605).
References
- S. K. Kim and I. Wijesekara, Development and biological activities of marine-derived bioactive peptides: A review, J. Funct. Foods, 2010, 2(1), 1–9, DOI:10.1016/j.jff.2010.01.003.
- Z. Deng, C. Cui, Y. Wang, J. Ni, L. Zheng, H. K. Wei and J. Peng, FSGHF3 and peptides, prepared from fish skin gelatin, exert a protective effect on DSS-induced colitis via the Nrf2 pathway, Food Funct., 2019, 11(1), 414–423, 10.1039/C9FO02165E.
- C. F. Chi, B. Wang, Y. M. Wang, B. Zhang and S. G. Deng, Isolation and characterization of three antioxidant peptides from protein hydrolysate of bluefin leatherjacket (Navodon septentrionalis) heads, J. Funct. Foods, 2015, 12, 1–10, DOI:10.1016/j.jff.2014.10.027.
- C. F. Chi, F. Y. Hu, B. Wang, X. J. Ren, S. G. Deng and C. F. Wu, Purification and characterization of three antioxidant peptides from protein hydrolysate of croceine croaker (Pseudosciaena crocea) muscle, Food Chem., 2015, 168, 662–667, DOI:10.1016/j.foodchem.2014.07.117.
- E. Malle, P. G. Furtmüller, W. Sattler and C. Obinger, Myeloperoxidase: a target for new drug development, Br. J. Pharmacol., 2007, 152(6), 838–854, DOI:10.1016/s1040-8428(09)70003-4.
- W. H. Zhao, Q. B. Luo, X. Pan, C. F. Chi and K. L. Sun, Preparation, identification, and activity evaluation of ten antioxidant peptides from protein hydrolysate of swim bladders of miiuy croaker (Miichthys miiuy), J. Funct. Foods, 2018, 47, 503–511, DOI:10.1016/j.jff.2018.06.014.
- C. F. Chi, F. Y. Hu, Z. R. Li, B. Wang and H. Y. Luo, Influence of different hydrolysis processes by trypsin on the physicochemical, antioxidant, and functional properties of collagen hydrolysates from sphyrna lewini, dasyatis akjei, and raja porosa, J. Aquat. Food Prod. Technol., 2016, 25(5), 616–632, DOI:10.1080/10498850.2014.898004.
- X. R. Yang, Y. Q. Zhao, Y. T. Qiu, C. F. Chi and B. Wang, Preparation and characterization of gelatin and antioxidant peptides from gelatin hydrolysate of skipjack tuna (Katsuwonus pelamis) bone stimulated by in vitro gastrointestinal digestion, Mar. Drugs, 2019, 17(2), 78, DOI:10.3390/md17020078.
- K. M. Young, J. G. Yeong, L. Y. Jeong, W. K. Sik, H. B. Yeon, L. Junsoo and J. H. Sang, Identification of anti-inflammatory active peptide from black soybean treated by high hydrostatic pressure after germination, Phytochem. Lett., 2018, 27, 167–173, DOI:10.1016/j.phytol.2018.07.008.
- K. Sato, S. Miyasaka, A. Tsuji and H. Tachi, Isolation and characterization of peptides with dipeptidyl peptidase IV (DPPIV) inhibitory activity from natto using DPPIV from aspergillus oryzae, Food Chem., 2018, 261, 51–56, DOI:10.1016/j.foodchem.2018.04.029.
- X. Kong, L. Zhang, W. Song, C. Zhang and X. Li, Separation, identification and molecular binding mechanism of dipeptidyl peptidase iv inhibitory peptides derived from walnut (Juglans regia L.) protein, Food Chem., 2021, 347(2), 129062, DOI:10.1016/J.FOODCHEM.2021.129062.
- A. G. Garzón, R. E. Cian, M. E. Aquino and S. R. Drago, Isolation and identification of cholesterol esterase and pancreatic lipase inhibitory peptides from brewer's spent grain by consecutive chromatography and mass spectrometry, Food Funct., 2020, 11(6), 4994–5003, 10.1039/d0fo00880j.
- S. D. He, Y. Zhang, H. J. Sun, M. Du, J. L. Qiu, M. M. Tang, X. B. Sun and B. W. Zhu, Antioxidative peptides from proteolytic hydrolysates of false abalone (Volutharpa ampullacea perryi): characterization, identification, and molecular docking, Mar. Drugs, 2019, 17(2), 116, DOI:10.3390/md17020116.
- X. Zhang, Q. Jia, M. Li, H. Liu and Z. Liu, Isolation of a novel calcium-binding peptide from phosvitin hydrolysates and the study of its calcium chelation mechanism, Food Res. Int., 2021, 141(3), 110169, DOI:10.1016/J.FOODRES.2021.110169.
- T. Valarmathi, R. Premkumar and A. M. F. Benial, Spectroscopic and molecular docking studies on 1-Hydroxyanthraquinone: A potent ovarian cancer drug, J. Mol. Struct., 2020, 12(13), 128–163, DOI:10.1016/j.molstruc.2020.128163.
- X. P. Li, X. X. Xie, J. X. Wang, Y. X. Xu, S. M. Yi, W. H. Zhu, H. B. Mi, T. T. Li and J. R. Li, Identification, taste characteristics and molecular docking study of novel umami peptides derived from the aqueous extract of the clam meretrix meretrix linnaeus, Food Chem., 2019, 312, 126053, DOI:10.1016/j.foodchem.2019.126053.
- A. M. van der Does, P. J. Hensbergen, S. J. Bogaards, M. Cansoy, A. M. Deelder, H. C. van Leeuwen, J. W. Drijfhout, J. T. van Dissel and P. H. Nibbering, The human lactoferrin-derived peptide hLF1–11 exerts immunomodulatory effects by specific inhibition of myeloperoxidase activity, J. Immunol., 2012, 188(10), 5012–5019, DOI:10.4049/jimmunol.1102777.
- D. M. Liu, X. Chen, J. C. Huang, M. Huang and G. H. Zhou, Generation of bioactive peptides from duck meat during post-mortem aging, Food Chem., 2017, 237, 408–415, DOI:10.1016/j.foodchem.2017.05.094.
- J. Y. Ko, J. H. Lee, K. Samarakoon, J. S. Kim and Y. J. Jeon, Purification and determination of two novel antioxidant peptides from flounder fish (Paralichthys olivaceus) using digestive proteases, Food Chem. Toxicol., 2013, 52, 113–120, DOI:10.1016/j.fct.2012.10.058.
- H. Cao, S. Ma, H. Guo, X. W. Cui, S. S. Wang, X. F. Zhong, Y. M. Wu, W. X. Zheng, H. N. Wang, J. Y. Yu, L. Y. Ma and C. C. Han, Comparative study on the monosaccharide compositions, antioxidant and hypoglycemic activities in vitro of intracellular and extracellular polysaccharides of liquid fermented Coprinus comatus, Int. J. Biol. Macromol., 2019, 139, 543–549, DOI:10.1016/j.ijbiomac.2019.08.017.
- W. Li, X. Wang, J. Zhang, X. Zhao, Y. Wu, S. Tan, Q. Zheng and X. Gao, Multivariate analysis illuminates the effects of vacuum drying on the extractable and nonextractable polyphenols profile of loquat fruit, J. Food Sci., 2019, 84, 726–737, DOI:10.1111/1750-3841.14500.
- A. L. Yergey, J. R. Coorssen, P. S. Backlund, P. S. Blank and M. L. Vestal, De novo sequencing of peptides using MALDI/TOF-TOF, J. Am. Soc. Mass Spectrom., 2002, 13(7), 784–791, DOI:10.1016/S1044-0305(02)00393-8.
- X. Carpena, P. Vidossich, K. Schroettner, B. M. Calisto, S. Banerjee, J. Stampler, M. Soudi, P. G. Furtmuller, C. Rovira, I. Fita and C. Obinger, Essential role of proximal histidine-asparagine interaction in mammalian peroxidases, J. Biol. Chem., 2009, 284(38), 25929–25937, DOI:10.1074/jbc.M109.002154.
- J. B. Liu, Z. F. Chen, J. He, Y. Zhang, T. Zhang and Y. Q. Jiang, Anti-oxidative and anti-apoptosis effects of egg white peptide, Trp-Asn-Trp-Ala-Asp, against H2O2-induced oxidative stress in human embryonic kidney 293 cells, Food Funct., 2014, 5(12), 3179–3188, 10.1039/c4fo00665h.
- Q. Z. Zhang, X. H. Tong, Y. Li, H. Wang, Z. J. Wang, B. K. Qi, X. N. Sui and L. Z. Jiang, Purification and characterization of antioxidant peptides from alcalase-hydrolyzed soybean (Glycine max L.) hydrolysate and their cytoprotective effects in human intestinal Caco-2 cells, J. Agric. Food Chem., 2019, 67(20), 5772–5781, DOI:10.1021/acs.jafc.9b01235.
- D. Young, M. Z. Fan and Y. Mine, Egg yolk peptides up-regulate glutathione synthesis and antioxidant enzyme activities in a porcine model of intestinal oxidative stress, J. Agric. Food Chem., 2010, 58(13), 7624–7633, DOI:10.1021/jf1011598.
- A. A. Famuwagun, A. M. Alashi, O. S. Gbadamosi, K. A. Taiwo and R. E. Aluko, Antioxidant and enzymes inhibitory properties of Amaranth leaf protein hydrolyzates and ultrafiltration peptide fractions, J. Food Biochem., 2020, 45(3), e13396, DOI:10.1111/jfbc.13396.
- W. H. Zhu, H. W. Luan, Y. Bu, X. P. Li and Y. Y. Zhang, Identification, taste characterization and molecular docking study of novel umami peptides from the Chinese Anchovy Sauce, J. Sci. Food Agric., 2020, 101(8), 3140–3155, DOI:10.1002/jsfa.10943.
- A. G. P. Samaranayaka and E. C. Y. Li-Chan, Food-derived peptidic antioxidants: a review of their production, assessment, and potential applications, J. Funct. Foods, 2011, 3(4), 229–254, DOI:10.1016/j.jff.2011.05.006.
- J. R. Ruiz, D. B. Ancona and M. S. Campos, Bioactive vegetable proteins and peptides in lipid-lowering; nutraceutical potential, Nutr. Hosp., 2014, 29(4), 776–784, DOI:10.3305/nh.2014.29.4.7208.
- A. Gholami, S. Shahin, M. Mohkam, N. Nezafat and Y. Ghasemi, Cloning, characterization and bioinformatics analysis of novel cytosine deaminase from escherichia coli AGH09, Int. J. Pept. Res. Ther., 2015, 21(3), 365–374, DOI:10.1007/s10989-015-9465-9.
- Y. Zhang, S. D. He, E. Bonneil and B. K. Simpsonet, Generation of antioxidative peptides from Atlantic sea cucumber using alcalase versus trypsin: In vitro activity, de novo sequencing, and in silico docking for in vivo function prediction - ScienceDirect, Food Chem., 2019, 306, 125581, DOI:10.1016/j.foodchem.2019.125581.
- D. D. A. M. Van, P. J. Hensbergen, S. J. Bogaards, M. Cansoy, A. M. Deelder, H. C. Van Leeuwen, J. W. Drijfhout, J. T. van Dissel and P. H. Nibbering, The human lactoferrin-derived peptide hLF1–11 exerts immunomodulatory effects by specific inhibition of myeloperoxidase activity, J. Immunol., 2012, 188(10), 5012–5019, DOI:10.4049/jimmunol.1102777.
- V. F. Cruzat and J. Tirapegui, Effects of oral supplementation with glutamine and alanyl-glutamine on glutamine, glutamate, and glutathione status in trained rats and subjected to long-duration exercise, Nutrition, 2008, 25(4), 428–435, DOI:10.1016/j.nut.2008.09.014.
- N. Tsuge, Y. Eikawa, Y. Nomura, M. Yamamoto and K. Sugisawa, Antioxidative activity of peptides prepared by enzymatic-hydrolysis of egg-white albumin, Nippon Nōgeikagaku Kaishi, 1991, 65(11), 1635–1641, DOI:10.1271/nogeikagaku1924.65.1635.
- Y. I. Sahar and R. Carmichael, Are synthetic peptides real toxic allergens? a comprehensive literature review and update on therapeutic and adverse toxic immunogenic potentials of peptides-truth or dare, Int. J. Pharmacol. Toxicol. Sci., 2020, 8(1), 36–40, DOI:10.14419/ijpt.v8i1.30275.
- K. Soghra, S. S. Sima, M. H. Hamideh, G. Mehdi, V. Shirin, K. Shohreh, A. Soheila, E. Ali, A. Jafar, A. I. F. Abbas and R. A. Mehdi, Effects of the antifungal peptide Skh-AMP1 derived from Satureja khuzistanica on cell membrane permeability, ROS production, and cell morphology of conidia and hyphae of Aspergillus fumigatus, Peptides, 2020, 123(C), 170195, DOI:10.1016/j.peptides.2019.170195.
- H. S. Tehrani and A. A. Moosavi-Movahedi, Catalase and its mysteries, Prog. Biophys. Mol. Biol., 2018, 140, 5–12, DOI:10.1016/j.pbiomolbio.2018.03.001.
- Y. Jie, H. F. Zhao and B. L. Zhang, The role of an acidic peptide on controlling the oxidation process of walnut oil, Foods, 2019, 8(10), 499, DOI:10.1111/jfbc.13396.
- B. Y. Zhang, H. Y. Wang, Y. Wang, Y. D. Yu, J. B. Liu, B. Q. Liu and T. Zhang, Identification of antioxidant peptides derived from egg–white protein and its protective effects on H2O2−induced cell damage, Int. J. Food Sci. Technol., 2019, 54(6), 2219–2227, DOI:10.1111/ijfs.14133.
|
This journal is © The Royal Society of Chemistry 2023 |
Click here to see how this site uses Cookies. View our privacy policy here.