DOI:
10.1039/D2GC03710F
(Communication)
Green Chem., 2023,
25, 153-160
Photo-induced spirocyclization of biaryl ynones with ammonium thiocyanate: access to thiocyanate-featured spiro[5,5]trienones†
Received
4th October 2022
, Accepted 22nd November 2022
First published on 25th November 2022
Abstract
Photo-promoted mild tandem 6-exo-trig spirocyclization of biaryl ynones with ammonium thiocyanate has been developed to access thiocyanate-containing spiro[5,5]trienones with good yields and regioselectivity. Meanwhile, other thiocyanate-substituted cycles also could be produced under similar conditions. The merits and reaction process of this radical system were examined by a variety of scaled-up operations, functional group transformations, intermediate capture study, and on/off light experiments.
Organic thiocyanates commonly exhibit important and wide-ranging biological activities and drug properties (Fig. 1).1 They also can be used as organic scaffolds to produce a variety of thiols,2 thioethers,3 thiocarbamates,4 and alkynyl thioethers,5etc., in synthetic chemistry. To date, various efficient protocols for the construction of aryl, alkyl, or vinyl thiocyanates have been developed by many chemists.6–9 Among them, as valuable organic substrates, vinyl thiocyanates are commonly produced from alkenes, alkynes, or their derivatives via coupling/addition/cascade reactions (Scheme 1a).8,9 Recently, our group reported a photo-promoted tandem cyclization of ammonium thiocyanate (NH4SCN) with aryl acetylenes to access seven-membered exocyclic vinyl thiocyanates (Scheme 1b).10 Despite this advanced work, diverse mild strategies for the synthesis of spirocycle-containing vinyl thiocyanates with good regioselectivity/yields are still rarely reported (Scheme 1c).
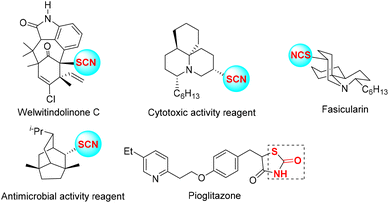 |
| Fig. 1 Representative bioactive thiocyanates and derivative drugs. | |
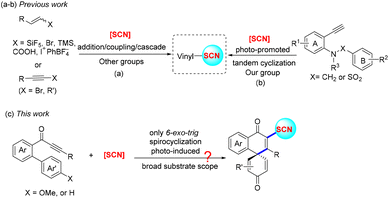 |
| Scheme 1 Methods of preparation of vinyl thiocyanates. | |
On the other hand, spirocycles, representing an important and prevalent organic skeleton, are extensively found in natural products, pharmaceuticals, agrochemicals, as well as in organic chemistry (such as the ligands utilized in metal-catalyzed systems).11 Given that, many efficient protocols for the synthesis of spirocycles have been developed, which involve a variety of transition-metal-promoted dearomatization, nucleophilic/electrophilic dearomatization, oxidative dearomatization, or radical-induced dearomatization processes.12–15 Among them, diverse spiro compounds were obtained via the radical tandem 5-exo-trig or 6-exo-trig spirocyclization of alkynes with common radical species.15c–e,16,17 For instance, efficient cascade 6-exo-trig spirocyclization of biaryl ynones to access spiro[5,5]trienones has been developed by Zhang/Ackermann, Wang, Zhou, Duan, Zhu, Reddy, Perin, Liu, and other groups recently.16c,g,18 Despite these critical achievements, some challenges still need to be solved by using biaryl ynones as starting materials: (1) more mild and simple reaction conditions need to be found; (2) regioselectivity for the 5-exo-trig, 6-exo-trig, or 7-exo-trig reaction process needs to be controlled; (3) a broader substrate scope and more radical species need to be explored. The above problems are being studied by our group. Based on our previous radical study,19 we finally developed a mild, solely 6-exo-trig spirocyclization of biaryl ynones with NH4SCN, which resulted in SCN-modified spiro[5,5]trienones with good regioselectivity and a broad substrate scope. Meanwhile, other SCN-substituted cycles also could be obtained under similar radical conditions.
Initially, 1-(4′-methoxy-[1,1′-biphenyl]-2-yl)-3-phenylprop-2-yn-1-one and NH4SCN were selected as model substrates to screen the reaction conditions, and the results are described in Table 1. First, a variety of photocatalysts were tested in this spirocyclization reaction, which indicated that fluorescein was better than rhodamine 6G, rhodamine B, eosin Y, and 4CZIPN (entries 1–8). Next, varying the kinds and equivalents of bases resulted in worse outcomes (entries 9–13). Meanwhile, the yield of product 1 was not improved after further optimizations of thiocyanates and the reaction solvent (entries 14–21). Finally, product 1 was gained in 74% yield under the following optimum conditions: 1-(4′-methoxy-[1,1′-biphenyl]-2-yl)-3-phenylprop-2-yn-1-one (1 equiv., 0.1 mmol), NH4SCN (3 equiv., 0.3 mmol), fluorescein (0.05 equiv., 0.005 mmol), DMAP (0.2 equiv., 0.02 mmol), CH3CN (3.5 mL), 18 W blue LEDs, rt.
Table 1 Optimization of reaction conditionsa
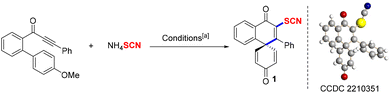
|
Entry |
Photocatalyst (mol %) |
Thiocyanate (equiv.) |
Base (equiv.) |
Solvent (mL) |
Yieldb (%) |
Reaction conditions: 1-(4′-methoxy-[1,1′-biphenyl]-2-yl)-3-phenylprop-2-yn-1-one (1 equiv., 0.1 mmol), NH4SCN (3 equiv., 0.3 mmol), fluorescein (0.05 equiv., 0.005 mmol), DMAP (0.2 equiv., 0.02 mmol), CH3CN (3.5 mL), 18 W blue LEDs, rt.
Isolated yields.
|
1 |
Rhodamine 6G (1) |
NH4SCN (3) |
DMAP (0.2) |
CH3CN (3.5) |
59 |
2 |
Rhodamine B (1) |
NH4SCN (3) |
DMAP (0.2) |
CH3CN (3.5) |
34 |
3 |
Eosin Y (1) |
NH4SCN (3) |
DMAP (0.2) |
CH3CN (3.5) |
62 |
4 |
4CZIPN (1) |
NH4SCN (3) |
DMAP (0.2) |
CH3CN (3.5) |
46 |
5 |
Fluorescein (1) |
NH4SCN (3) |
DMAP (0.2) |
CH3CN (3.5) |
66 |
6 |
Fluorescein (2) |
NH4SCN (3) |
DMAP (0.2) |
CH3CN (3.5) |
68 |
7 |
Fluorescein (3) |
NH4SCN (3) |
DMAP (0.2) |
CH3CN (3.5) |
68 |
8
|
Fluorescein (5)
|
NH
4
SCN (3)
|
DMAP (0.2)
|
CH
3
CN (3.5)
|
74
|
9 |
Fluorescein (5) |
NH4SCN (3) |
–– |
CH3CN (3.5) |
70 |
10 |
Fluorescein (5) |
NH4SCN (3) |
DMAP (0.1) |
CH3CN (3.5) |
72 |
11 |
Fluorescein (5) |
NH4SCN (3) |
DMAP (0.3) |
CH3CN (3.5) |
62 |
12 |
Fluorescein (5) |
NH4SCN (3) |
Et3N (0.2) |
CH3CN (3.5) |
69 |
13 |
Fluorescein (5) |
NH4SCN (3) |
K2HPO4 (0.2) |
CH3CN (3.5) |
60 |
14 |
Fluorescein (5) |
NH4SCN (2) |
DMAP (0.2) |
CH3CN (3.5) |
59 |
15 |
Fluorescein (5) |
NH4SCN (4) |
DMAP (0.2) |
CH3CN (3.5) |
51 |
16 |
Fluorescein (5) |
NaSCN (3) |
DMAP (0.2) |
CH3CN (3.5) |
70 |
17 |
Fluorescein (5) |
KSCN (3) |
DMAP (0.2) |
CH3CN (3.5) |
64 |
18 |
Fluorescein (5) |
NH4SCN (3) |
DMAP (0.2) |
CH3CN (2.5) |
38 |
19 |
Fluorescein (5) |
NH4SCN (3) |
DMAP (0.2) |
CH3CN (4.5) |
48 |
20 |
Fluorescein (5) |
NH4SCN (3) |
DMAP (0.2) |
CH3OH (3.5) |
66 |
21 |
Fluorescein (5) |
NH4SCN (3) |
DMAP (0.2) |
DCE(3.5) |
72 |
Next, under the optimum conditions, the substrate scope of the spirocyclization reactions was studied as depicted in Table 2. First, the R group on the alkyne moiety of the substrates modified with electron-donating or electron-withdrawing substituents could react well with NH4SCN in the reaction system, which produced the corresponding SCN-containing spiro[5,5]trienones in 53–88% yields (1–7). In addition, the R group of biaryl ynones bearing halogen, heterocycle, and alkyl groups also were compatible with this radical system, and products 8–13 were isolated in 58–72% yields, respectively. Subsequently, various substituents (such as Me, NO2, F, and Cl) modifying the aromatic rings adjacent to the ketones of the starting materials afforded the desired products 14–17 in moderate to good yields. Finally, naphthyl and thiophenyl group-containing biaryl ynones also could smoothly proceed with the radical spirocyclization reaction (18–19).
Table 2 Substrate scope of biaryl ynonesa
Reaction conditions: biaryl ynones (1 equiv., 0.1 mmol), NH4SCN (3 equiv., 0.3 mmol), fluorescein (0.05 equiv., 0.005 mmol), DMAP (0.2 equiv., 0.02 mmol), CH3CN (3.5 mL), 18 W blue LEDs, rt, isolated yields.
|
|
Afterward, the functional group tolerance of the dearomative aryl rings of the substrates also was explored under typical conditions (Table 3). First, the dearomative aryl ring-bearing unit with the combination of 3-Me/4-MeO, 3,5-di-Me/4-MeO, 2,4-di-MeO, 3,4-di-MeO, and even 2,3,4-tri-MeO groups all could well undergo the 6-exo-trig spirocyclization process, which gave the corresponding final products in 52–84% yields (20–24). Meanwhile, halogen-modified substrates (such as 2-F/4-MeO, 3-F/4-MeO, and 3-Cl/4-MeO groups) resulted in products 25–27 in 52–75% yields. Notably, the OH or CHO group-containing biaryl ynones also could exist well in this radical system (28–29).
Table 3 Substrate scope of dearomative aryl ringsa
Reaction conditions: biaryl ynones (1 equiv., 0.1 mmol), NH4SCN (3 equiv., 0.3 mmol), fluorescein (0.05 equiv., 0.005 mmol), DMAP (0.2 equiv., 0.02 mmol), CH3CN (3.5 mL), 18 W blue LEDs, rt, isolated yields.
|
|
A variety of application research methods were performed to test the merit and synthetic value of the reaction system (Scheme 2). First, no p-MeO-containing dearomative aryl ring moiety of the substrates could also undergo spirocyclization reaction with good regioselectivity (Scheme 2a and b). Next, product 1 was isolated in a 53% yield with the reaction scaled up to 1 mmol (Scheme 2c). Meanwhile, other unsaturated alkenes or alkynes also were compatible with this radical system, which produced SCN-substituted 5- or 6-membered cycles in moderate yields (Scheme 2d–f). Finally, two functional group transformations have been operated to access the corresponding derivatives 36–37 in 69–75% yields (Scheme 2g and h).
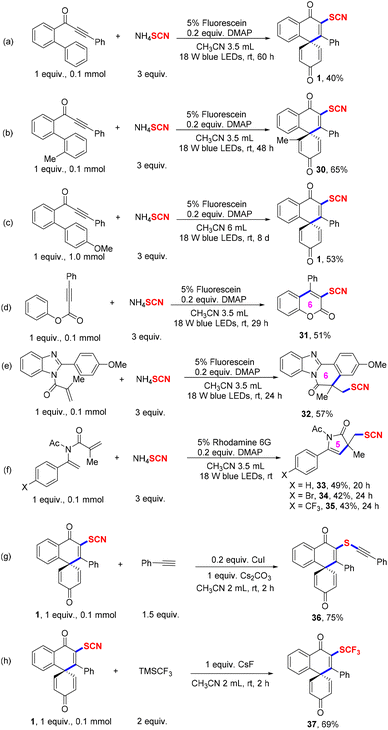 |
| Scheme 2 Scaled-up experiments and further transformations. | |
Various mechanistic studies were operated to verify the radical process (Scheme 3). First, with the addition of 2,6-di-tert-butyl-4-methylphenol (BHT) or 2,2,6,6-tetramethyl-1-piperidyloxy (TEMPO) into the reaction system, respectively, product 1 was not found by TLC. Excitingly, radical intermediates 38, 39, 40, and 41 all could successfully be detected by HRMS (Scheme 3a and b, also see ESI for details†). The yield of product 1 was very poor under the N2 atmosphere, which indicated that dioxygen was important for the reaction system (Scheme 3c). Finally, to test the importance of blue light, a variety of on/off light experiments were performed under typical conditions (Scheme 4).
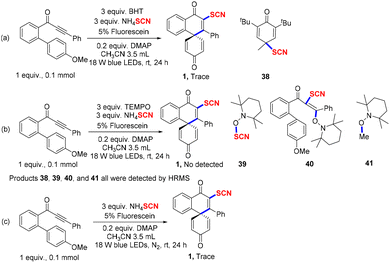 |
| Scheme 3 Mechanistic study. | |
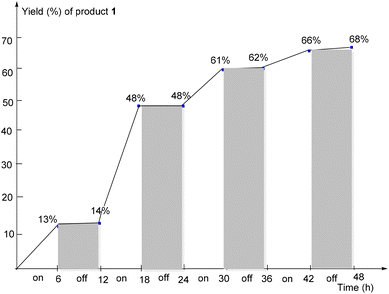 |
| Scheme 4 On/off light experiments of product 1. | |
According to the experimental results as well as previous studies,20 a plausible reaction pathway was described as shown in Scheme 5. First, with the assistance of fluorescein/blue light/oxygen, NH4SCN could smoothly produce the SCN radical. With the formation of the SCN radical, DMAP could stabilize the ammonium ion. Next, vinyl radical A was gained via the SCN radical attacking the triple bond of biaryl ynones, which then underwent spirocyclization and resulted in radical B. Finally, intermediate B proceeded with β-fragmentation to access the final product and methyl radical, which was successfully captured by TEMPO.
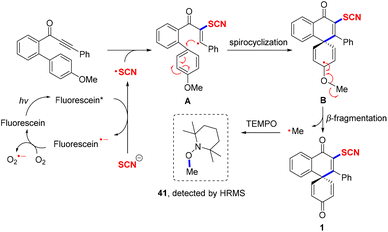 |
| Scheme 5 Plausible mechanism. | |
Conclusions
In conclusion, a mild and available radical spirocyclization of NH4SCN with biaryl ynones was accomplished, which could efficiently produce various SCN-modified spiro[5,5]trienones. Other unsaturated substrates also were compatible with this reaction system to access SCN-containing cycles. This radical system gave a good green matrix (such as atom economy and E-factor, respectively calculated as 91% and 64%).21 Meanwhile, a variety of applied research and mechanistic studies were also completed in this work. Additional studies on the construction of other spirocycles or fused cycles are ongoing in our laboratory.
Author contributions
S. Chen and Q. Yan studied the reaction conditions, substrate scopes, further transformations, and plausible mechanisms. J. Fan and C. Guo prepared a variety of biaryl ynones. Finally, L.-J. L., Z.-Q. Liu, and Z.-J. Li all conducted the corresponding experiments and wrote the manuscript.
Conflicts of interest
There are no conflicts to declare.
Acknowledgements
This project is supported by the National Natural Science Foundation of China (21702044, 21971116), the Natural Science Foundation of Hebei Province (B2020201014, B2022201059), Science and Technology Project of Hebei Education Department (QN2019063), and Research Innovation Team of College of Chemistry and Environmental Science of Hebei University (hxkytd-py2102).
References
- For representative references, see:
(a) A. K. Mukerjee and R. Ashare, Isothiocyanates in the chemistry of heterocycles, Chem. Rev., 1991, 91, 1–24 CrossRef CAS;
(b)
R. J. Lukens, Chemistry of Fungicidal Action, Chapman and Hall, London, 1971 CrossRef;
(c) Y. Yasman, R. A. Edrada, V. Wray and P. Proksch, New 9-Thiocyanatopupukeanane Sesquiterpenes from the Nudibranch Phyllidia varicosa and Its Sponge-Prey Axinyssa aculeata, J. Nat. Prod., 2003, 66, 1512–1514 CrossRef PubMed;
(d) J. R. Falck, S. H. Gao, R. N. Prasad and S. R. Koduru, Electrophilic α-thiocyanation of chiral and achiral N-acyl imides. A convenient route to 5-substituted and 5,5-disubstituted 2,4-thiazolidinediones, Bioorg. Med. Chem. Lett., 2008, 18, 1768–1771 CrossRef CAS PubMed;
(e) E. Elhalem, B. N. Bailey, R. Docampo, I. Ujvary, S. H. Szajnman and J. B. Rodriguez, Design, Synthesis, and Biological Evaluation of Aryloxyethyl Thiocyanate Derivatives against Trypanosoma cruzi, J. Med. Chem., 2002, 45, 3984–3999 CrossRef CAS;
(f) V. A. Kokorekin, A. O. Terent'ev, G. V. Ramenskaya, N. E. Grammatikova, G. M. Rodionova and A. I. Ilovaiskii, Synthesis and Antifungal Activity of Arylthiocyanates, Pharm. Chem. J., 2013, 47, 422–425 CrossRef CAS;
(g) Z. An, Y. Liu, P. Zhao and R. L. Yan, I2-Promoted [3 + 2] Cyclization of 1,3-Diketones with Potassium Thiocyanate: a Route to Thiazol-2(3H)-One Derivatives, Adv. Synth. Catal., 2021, 363, 3240–3244 CrossRef CAS;
(h) K. M. Werner and J. M. de los Santos, Weinreb SM, Shang MY. A Convergent Stereoselective Synthesis of the Putative Structure of the Marine Alkaloid Lepadiformine via an Intramolecular Nitrone/1,3-Diene Dipolar Cycloaddition, J. Org. Chem., 1999, 64, 686–687 CrossRef CAS;
(i) R. P. Austin, P. Barton, R. V. Bonnert, R. C. Brown, P. A. Cage, D. R. Cheshire, A. M. Davis, I. G. Dougall, F. Ince and G. Pairaudeau, QSAR and the Rational Design of Long-Acting Dual D2-Receptor/β2-Adrenoceptor Agonists, J. Med. Chem., 2003, 46, 3210–3220 CrossRef CAS;
(j) S. P. Tanis, T. T. Parker, J. R. Colca, R. M. Fisher and R. F. Kletzein, Synthesis and Biological Activity of Metabolites of the Antidiabetic, Antihyperglycemic Agent Pioglitazone, J. Med. Chem., 1996, 39, 5053–5063 CrossRef CAS;
(k) S. A. Mosure, J. Shang, J. Eberhardt, R. Brust, J. Zheng, P. R. Griffin, S. Forli and D. J. Kojetin, Structural Basis of Altered Potency and Efficacy Displayed by a Major in Vivo Metabolite of the Antidiabetic PPARY Drug Pioglitazone, J. Med. Chem., 2019, 62, 2008–2023 CrossRef CAS PubMed;
(l) S. Dutta, H. Abe, S. Aoyagi, C. Kibayashi and K. S. Gates, DNA Damage by Fasicularin, J. Am. Chem. Soc., 2005, 127, 15004–15005 CrossRef CAS;
(m) I. C. Piña, J. T. Gautschi, G. Y. S. Wang, M. L. Sanders, F. J. Schmitz, D. France, S. Cornell-Kennon, L. C. Sambucetti, S. W. Remiszewski, L. B. Perez, K. W. Bair and P. Crews, Psammaplins from the Sponge Pseudoceratina purpurea: Inhibition of Both Histone Deacetylase and DNA Methyltransferase, J. Org. Chem., 2003, 68, 3866–3873 CrossRef PubMed.
- For selected references, see:
(a) G. Segalovich-Gerendash, I. Rozenberg, N. Alassad, N. B. Nechmad, I. Goldberg, S. Kozuch and N. G. Lemcoff, Imposing Latency in Ruthenium Sulfoxide-Chelated Benzylidenes: Expanding Opportunities for Thermal and Photoactivation in Olefin Metathesis, ACS Catal., 2020, 10, 4827–4834 CrossRef CAS;
(b) C. Maurya, A. Mazumder and P. K. Gupta, Phosphorus pentasulfide mediated conversion of organic thiocyanates to thiols, Beilstein J. Org. Chem., 2017, 13, 1184–1188 CrossRef CAS PubMed;
(c) J. V. N. V. Prasad, Synthesis of Heterocyclic Thiosulfonates, Org. Lett., 2000, 2, 1069–1072 CrossRef CAS;
(d) B. Robillard, L. Hughes, M. Slaby, D. A. Lindsay and K. U. Ingold, Synthesis of 2-substituted 5,7,8-trimethyl-6-hydroxythiochromans and purported syntheses of sulfur-containing analogs of vitamin E, J. Org. Chem., 1986, 51, 1700–1704 CrossRef CAS.
- For selected references, see:
(a) A. R. Hajipour, R. Pourkaveha and H. Karimia, Synthesis of diaryl thioethers from aryl halides and potassium thiocyanate, Appl. Organomet. Chem., 2014, 28, 879–883 CrossRef CAS;
(b) F. Ke, Y. Qu, Z. Jiang, Z. Li, D. Wu and X. Zhou, An Efficient Copper-Catalyzed Carbon−Sulfur Bond Formation Protocol in Water, Org. Lett., 2011, 13, 454–457 CrossRef CAS PubMed;
(c) M. Gulea, F. Hammerschmidt, P. Marchand, S. Masson, V. Pisljagic and F. Wuggenig, Synthesis of chiral, nonracemic α-sulfanylphosphonates and derivatives, Tetrahedron: Asymmetry, 2003, 14, 1829–1836 CrossRef CAS;
(d) I. W. J. Still, Toste D, Reduction of Aryl Thiocyanates with SmI2 and Pd-Catalyzed Coupling with Aryl Halides as a Route to Mixed Aryl Sulfides, J. Org. Chem., 1996, 61, 7677–7680 CrossRef CAS PubMed.
- For selected references, see:
(a) Y. G. Kim, H. N. Lim and K. J. Lee, A new route to allyl thiols and allyl thiocarbamates from Baylis-Hillman adducts, J. Heterocycl. Chem., 2009, 46, 23–27 CrossRef CAS;
(b) J. R. Falck, S. Gao, R. N. Prasad and S. R. Koduru, Electrophilic α-thiocyanation of chiral and achiral N-acyl imides. A convenient route to 5-substituted and 5,5-disubstituted 2,4-thiazolidinediones, Bioorg. Med. Chem. Lett., 2008, 18, 1768–1771 CrossRef CAS;
(c) A. Klásek, V. Mrkvička, A. Pevec and J. Košmrlj, Novel Tandem Hydration/Cyclodehydration of α-Thiocyanatoketones to 2-Oxo-3-thiazolines. Application to Thiazolo[5,4-c]quinoline-2,4(3aH,5H)-dione Synthesis, J. Org. Chem., 2004, 69, 5646–5651 CrossRef PubMed;
(d) A. K. Shiryaev and I. K. Moiseev, Adamantylation of Thiocyanatoacetamide and Thiocyanatoacetylurea, Russ. J. Org. Chem., 2001, 37, 746–747 CrossRef CAS.
- For selected references, see:
(a) R. Chandran, A. Pise, S. K. Shah, D. Rahul, V. Suman and K. Tiwari, Copper-Catalyzed Thiolation of Terminal Alkynes Employing Thiocyanate as the Sulfur Source Leading to Enaminone-Based Alkynyl Sulfides under Ambient Conditions, Org. Lett., 2020, 22, 6557–6561 CrossRef;
(b) L. Yang, Z. Y. Tian and C. P. Zhang, Transition-Metal-Free Selective Synthesis of (Z,)-1,2-Diarylthio-1-arylalkenes, (2-Arylethene-1,1,2-triyl)tris(arylsulfane)s and Alkynyl Sulfides from Thiocyanates and Terminal Arylalkynes, ChemistrySelect, 2019, 4, 311–315 CrossRef CAS.
- For selected references, see:
(a) J. Yadav, B. S. Reddy, S. Shubashree and K. Sadashiv, Iodine/MeOH: a novel and efficient reagent system for thiocyanation of aromatics and heteroaromatics, Tetrahedron Lett., 2004, 45, 2951–2954 CrossRef CAS;
(b) X. Q. Pan, M. Y. Lei, J. P. Zou and W. Zhang, Mn(OAc) 3-p romoted regioselective free radical thiocyanation of indoles and anilines, Tetrahedron Lett., 2009, 50, 347–349 CrossRef CAS;
(c) W. Fan, Q. Yang, F. Xu and P. Li, A Visible-Light-Promoted Aerobic Metal-Free C-3 Thiocyanation of Indoles, J. Org. Chem., 2014, 79, 10588–10592 CrossRef CAS PubMed;
(d) D. Yang, K. Yan, W. Wei, G. Li, S. Lu, C. Zhao, L. Tian and H. Wang, Catalyst-Free Regioselective C-3 Thiocyanation of Imidazopyridines, J. Org. Chem., 2015, 80, 11073–11079 CrossRef CAS PubMed;
(e) M. Barbero, I. Degani, N. Diulgheroff, S. Dughera and R. Fochi, Improved Procedure to Aryl Thiocyanates: A New Synthetic Application of Dry Arenediazonium o-Benzenedisulfonimides, Synthesis, 2001, 585–590 CrossRef CAS;
(f) Y. F. Wang, Y. Zhou, J. R. Wang, L. Liu and Q. X. Guo, CuI-catalyzed Synthesis of Aryl Thiocyanates from Aryl Iodides, Chin. Chem. Lett., 2006, 17, 1283–1386 CAS;
(g) N. Sun, L. Che, W. Mo, B. Hu, Z. Shen and X. Hu, A mild copper-catalyzed aerobic oxidative thiocyanation of arylboronic acids with TMSNCS, Org. Biomol. Chem., 2015, 13, 691–696 RSC;
(h) H. F. Jiang, W. Yu, X. Tang, J. Li and W. Q. Wu, Copper-Catalyzed Aerobic Oxidative Regioselective Thiocyanation of Aromatics and Heteroaromatics, J. Org. Chem., 2017, 82, 9312–9320 CrossRef CAS.
- For selected references, see:
(a) Y. Ju, D. Kumar and R. S. Varma, Revisiting Nucleophilic Substitution Reactions: Microwave-Assisted Synthesis of Azides, Thiocyanates, and Sulfones in an Aqueous Medium, J. Org. Chem., 2006, 71, 6697–6700 CrossRef CAS;
(b) J. S. Qiu, D. Wu, P. G. Karmaker, H. Q. Yin and F. X. Chen, Enantioselective Organocatalyzed Direct α-Thiocyanation of Cyclic β-Ketoesters by N-Thiocyanatophthalimide, Org. Lett., 2018, 20, 1600–1603 CrossRef CAS;
(c) D. Wu, J. S. Qiu, P. G. Karmaker, H. Q. Yin and F. X. Chen,
N-Thiocyanatosaccharin: A “Sweet” Electrophilic Thiocyanation Reagent and the Synthetic Applications, J. Org. Chem., 2018, 83, 1576–1583 CrossRef CAS PubMed;
(d) J. Jiao, L. X. Nguyen, D. R. Patterson and R. A. Flowers, An Efficient and General Approach to β-Functionalized Ketones, Org. Lett., 2007, 9, 1323–1326 CrossRef CAS PubMed;
(e) Y. T. Chen, S. F. Wang, Q. W. Jiang, C. G. Cheng, X. H. Xiao and G. G. Zhu, Palladium-Catalyzed Site-Selective sp3 C–H Bond Thiocyanation of 2-Aminofurans, J. Org. Chem., 2018, 83, 716–722 CrossRef CAS PubMed;
(f) C. Xu, Z. He, X. Kang and Q. L. Zeng, Highly selective radical isothiocyano-chalcogenization of alkenes with NH4SCN in water, Green Chem., 2021, 23, 7544–7548 RSC;
(g) P. Natarajan, Priya and D. Chuskit, Peroxodisulfate-assisted three-component benzylation of coumarins with styrenes and KSCN: a transition-metal-free approach for the synthesis of 3-(1-aryl-2-thiocyanatoethyl)-2H-chromen-2-one in ethyl lactate, Green Chem., 2021, 23, 4873–4881 RSC.
- For selected references, see:
(a) K. Tamao, T. Kakui and M. Kumada, (E)-alkenyl thiocyanates from (E)-alkenylpentafluorosilicates by the oxidative cleavage with copper(II) thiocyanate, Tetrahedron Lett., 1980, 21, 111–114 CrossRef CAS;
(b) T. Kitamura, S. Kobayashi and H. Taniguchi, Photolysis of vinyl halides. Reaction of photogenerated vinyl cations with cyanate and thiocyanate ions, J. Org. Chem., 1990, 55, 1801–1805 CrossRef CAS;
(c) J. Yan, H. W. Jin and Z. C. Chen, Study on the Stereoselective Reactions of Vinyl(phenyl)Iodonium Salts with Sodium Selenide, Sodium Sulfide, Sodium Azide and Potassium Thiocyanate, J. Chem. Res., 2007, 233–235 CrossRef CAS;
(d) Y. Tanabe, K. Mori and N. Kawabata, Phosphorus, Sulfur Silicon Relat. Elem., 1997, 120, 385 Search PubMed;
(e) S. Yang, T. He, D. Lin and J. M. Huang, Electrosynthesis of (E)-Vinyl Thiocyanates from Cinnamic Acids via Decarboxylative Coupling Reaction, Org. Lett., 2019, 21, 1958–1962 CrossRef CAS PubMed.
- For selected references, see:
(a) O. Prakash, V. Sharma, H. Batra and R. M. Moriarty, (Dichloroiodo)benzene and lead(II) thiocyanate as an efficient reagent combination for stereoselective 1,2-dithiocyanation of alkynes, Tetrahedron Lett., 2001, 42, 553–555 CrossRef CAS;
(b) G. B. Jiang, C. L. Zhu, J. X. Li, W. Q. Wu and H. F. Jiang, Silver-Catalyzed Regio- and Stereoselective Thiocyanation of Haloalkynes: Access to (Z)-Vinyl Thiocyanates, Adv. Synth. Catal., 2017, 359, 1208–1212 CrossRef CAS;
(c) C. Wu, L. H. Lu, A. Z. Peng, G. K. Jia, C. Peng, Z. Cao, Z. L. Tang, W. M. He and X. H. Xu, Ultrasound-promoted Brønsted acid ionic liquid-catalyzed hydrothiocyanation of activated alkynes under minimal solvent conditions, Green Chem., 2018, 20, 3683–3688 RSC;
(d) X. H. Zeng and L. Chen, Iodine-mediated regio- and stereoselective iodothiocyanation of alkynes in aqueous ethanol, Org. Biomol. Chem., 2018, 16, 7557–7560 RSC.
- S. Chen, Q. Yan, J. Fan, Y. Gao, X. Yang, L. J. Li, Z. Q. Liu and Z. J. Li, Photoinduced cascade cyclization of alkynes with NH4SCN: access to SCN-containing dibenzazepines or dioxodibenzothiazepines, Green Chem., 2022, 24, 4742–4747 RSC.
- For representative references, see:
(a) A. R. Díaz-Marrero, G. Porras, Z. Aragón and J. M. de la Rosa, Dorta E, Cueto M, D'Croz L, Maté J, Darias J. Carijodienone from the Octocoral Carijoa multiflora. A Spiropregnane-Based Steroid, J. Nat. Prod., 2011, 74, 292–295 CrossRef;
(b) S. Kotha, A. C. Deb, K. Lahiri and E. Manivannan, Selected Synthetic Strategies to Spirocyclics, Synthesis, 2009, 165–193 CrossRef CAS;
(c) J. F. Hu, H. Fan, J. Xiong and S. B. Wu, Discorhabdins and Pyrroloiminoquinone-Related Alkaloids, Chem. Rev., 2011, 111, 5465–5491 CrossRef CAS PubMed;
(d) W. T. Wu, L. Zhang and S. L. You, Catalytic asymmetric dearomatization (CADA) reactions of phenol and aniline derivatives, Chem. Soc. Rev., 2016, 45, 1570–1580 RSC.
- For representative references, see:
(a) A. R. Pape, K. P. Kaliappan and E. P. Kündig, Transition-Metal-Mediated Dearomatization Reactions, Chem. Rev., 2000, 100, 2917–2940 CrossRef CAS PubMed;
(b) A. H. Bansode, S. R. Shaikh, R. G. Gonnade and N. T. Patil, Intramolecular ipso-arylative cyclization of aryl-alkynoates and N-arylpropiolamides with aryldiazonium salts through merged gold/visible light photoredox catalysis, Chem. Commun., 2017, 53, 9081–9084 RSC;
(c) L. Yang, H. Zheng, L. Luo, J. Nan, J. Liu, Y. Wang and X. Luan, Palladium-Catalyzed Dynamic Kinetic Asymmetric Transformation of Racemic Biaryls: Axial-to-Central Chirality Transfer, J. Am. Chem. Soc., 2015, 137, 4876–4879 CrossRef CAS PubMed;
(d) J. Nan, Z. Zuo, L. Luo, L. Bai, H. Zheng, Y. Yuan, J. Liu, X. Luan and Y. Wang, RuII-Catalyzed Vinylative Dearomatization of Naphthols via a C(sp2)–H Bond Activation Approach, J. Am. Chem. Soc., 2013, 135, 17306–17309 CrossRef CAS PubMed;
(e) L. Ding, W. T. Wu, L. Zhang and S. L. You, Construction of Spironaphthalenones via Gold-Catalyzed Intramolecular Dearomatization Reaction of β-Naphthol Derivatives, Org. Lett., 2020, 22, 5861–5865 CrossRef CAS PubMed.
- For representative references, see:
(a) J. J. Farndon, X. Ma and J. F. Bower, Transition Metal Free C–N Bond Forming Dearomatizations and Aryl C–H Aminations by in Situ Release of a Hydroxylamine-Based Aminating Agent, J. Am. Chem. Soc., 2017, 139, 14005–14008 CrossRef CAS PubMed;
(b) Y. Zhou, X. Zhang, Y. Zhang, L. Ruan, J. Zhang, D. Zhang-Negrerie and Y. Du, Iodocyclization of N-Arylpropynamides Mediated by Hypervalent Iodine Reagent: Divergent Synthesis of Iodinated Quinolin-2-ones and Spiro[4,5]trienones, Org. Lett., 2017, 19, 150–153 CrossRef CAS PubMed;
(c) R. P. Singh, J. Das, M. Yousufuddin, D. Gout and C. J. Lovely, Tandem Oxidative Dearomatizing Spirocyclizations of Propargyl Guanidines and Ureas, Org. Lett., 2017, 19, 4110–4113 CrossRef CAS PubMed;
(d) P. Fedoseev, G. Coppola, G. M. Ojeda and E. V. Van der Eycken, Synthesis of spiroindolenines by intramolecular ipso-iodocyclization of indol ynones, Chem. Commun., 2018, 54, 3625–3628 RSC;
(e) P. Fedoseev and E. V. Van der Eycken, Temperature switchable Brønsted acid-promoted selective syntheses of spiro-indolenines and quinolines, Chem. Commun., 2017, 53, 7732–7735 RSC.
- For representative references, see:
(a) C. Y. Jin, J. Y. Du, C. Zeng, X. H. Zhao, Y. X. Cao, X. Z. Zhang, X. Y. Lu and C. A. Fan, Hypervalent Iodine(III)-Mediated Oxidative Dearomatizing Cyclization of Arylamines, Adv. Synth. Catal., 2014, 356, 2437–2444 CrossRef CAS;
(b) L. Pouységu, D. Deffieux and S. Quideau, Hypervalent iodine-mediated phenol dearomatization in natural product synthesis, Tetrahedron, 2010, 66, 2235–2261 CrossRef;
(c) A. Rudolph, P. H. Bos, A. Meetsma, A. J. Minnaard and B. L. Feringa, Catalytic Asymmetric Conjugate Addition/Oxidative Dearomatization Towards Multifunctional Spirocyclic Compounds, Angew. Chem., Int. Ed., 2011, 50, 5834–5838 CrossRef CAS PubMed.
- For representative references, see:
(a) D. Ryzhakov, M. Jarret, J. P. Baltaze, R. Guillot, C. Kouklovsky and G. Vincent, Synthesis of 3,3-Spirocyclic 2-Phosphonoindolines via a Dearomative Addition of Phosphonyl Radicals to Indoles, Org. Lett., 2019, 21, 4986–4990 CrossRef CAS PubMed;
(b) D. Ryzhakov, M. Jarret, R. Guillot, C. Kouklovsky and G. Vincent, Radical-Mediated Dearomatization of Indoles with Sulfinate Reagents for the Synthesis of Fluorinated Spirocyclic Indolines, Org. Lett., 2017, 19, 6336–6339 CrossRef CAS PubMed;
(c) W. C. Yang, M. M. Zhang and J. G. Feng, Recent Advances in the Construction of Spiro Compounds via Radical Dearomatization, Adv. Synth. Catal., 2020, 362, 4446–4461 CrossRef CAS;
(d) H. Zhang, Z. Gu, P. Xu, H. Hu, Y. Cheng and C. Zhu, Metal-free tandem oxidative C(sp3)–H bond functionalization of alkanes and dearomatization of N-phenyl-cinnamamides: access to alkylated 1-azaspiro[4.5]decanes, Chem. Commun., 2016, 52, 477–480 RSC;
(e) X. H. Ouyang, R. J. Song, B. Liu and J. H. Li, Synthesis of 3-alkyl spiro[4,5]trienones by copper-catalyzed oxidative ipso-annulation of activated alkynes with unactivated alkanes, Chem. Commun., 2016, 52, 2573–2576 RSC.
- For representative references, see:
(a) Y. Zhang, J. Zhang, B. Hu, M. Ji, S. Ye and G. Zhu, Synthesis of Difluoromethylated and Phosphorated Spiro[5.5]trienones via Dearomative Spirocyclization of Biaryl Ynones, Org. Lett., 2018, 20, 2988–2992 CrossRef CAS PubMed;
(b) W. Dong, Y. Yuan, X. Xie and Z. Zhang, Visible-Light-Driven Dearomatization Reaction toward the Formation of Spiro[4.5]deca-1,6,9-trien-8-ones, Org. Lett., 2020, 22, 528–532 CrossRef CAS PubMed;
(c) D. Xia and X. F. Duan, Alkylative Dearomatization by Using an Unactivated Aryl Nitro Group as a Leaving Group: Access to Diversified Alkylated Spiro[5.5]trienones, Org. Lett., 2021, 23, 2548–2552 CrossRef PubMed;
(d) H. E. Ho, A. Pagano, J. A. Rossi-Ashton, J. R. Donald, R. G. Epton, J. C. Churchill and M. J. James, O'Brien P, Taylor RJK, Unsworth WP. Visible-light-induced intramolecular charge transfer in the radical spirocyclisation of indole-tethered ynones, Chem. Sci., 2020, 11, 1353–1360 RSC;
(e) C. Li, L. Xue, J. Zhou, Y. Zhao, G. Han, J. Hou, Y. Song and Y. Liu, Copper-Catalyzed Trifluoromethylation of Ynones Coupled with Dearomatizing Spirocyclization of Indoles: Access to CF3-Containing Spiro[cyclopentane-1,3′-indole, Org. Lett., 2020, 22, 3291–3296 CrossRef CAS PubMed;
(f) D. P. Jin, P. Gao, D. Q. Chen, S. Chen, J. Wang, X. Y. Liu and Y. M. Liang, AgSCF3-Mediated Oxidative Trifluoromethythiolation of Alkynes with Dearomatization to Synthesize SCF3-Substituted Spiro[4,5]trienones, Org. Lett., 2016, 18, 3486–3489 CrossRef CAS PubMed;
(g) L. Zhou, Y. Xia, Y. Z. Wang, J. D. Fang and X. Y. Liu, Mn(III)-promoted synthesis of spiroannular tricyclic scaffolds via sulfonylation/dearomatization of biaryl ynones, Tetrahedron, 2019, 75, 1267–1274 CrossRef CAS.
- For representative references, see:
(a) X. H. Yang, X. H. Ouyang, W. T. Wei, R. J. Song and J. H. Li, Nitrative Spirocyclization Mediated by TEMPO: Synthesis of Nitrated Spirocycles from N-Arylpropiolamides, tert-Butyl Nitrite and Water, Adv. Synth. Catal., 2015, 357, 1161–1166 CrossRef CAS;
(b) B. Tang, Q. Yin, R. Tang and J. H. Li, Electrophilic ipso-Cyclization of N-(p-Methoxyaryl)propiolamides Involving an Electrophile-Exchange Process, J. Org. Chem., 2008, 73, 9008–9011 CrossRef CAS PubMed;
(c) C. R. Reddy, U. Ajaykumar and D. H. Kolgave, Expeditious Access to Spiro-Fused 2,5-Cyclohexadienones via Thio(seleno)cyanative ipso-Cyclization, J. Org. Chem., 2020, 85, 15521–15531 CrossRef PubMed;
(d) P. Chen, J. Fan, W. Yu, B. Xiong, Y. Liu, K. Tang and J. Xie, Alkylation/Ipso-cyclization of Active Alkynes Leading to 3-Alkylated Aza- and Oxa-spiro[4,5]-trienones, J. Org. Chem., 2022, 87, 5643–5659 CrossRef CAS PubMed.
- For representative references, see:
(a) Y. Zhang, C. Ma, J. Struwe, J. Feng, G. G. Zhu and L. Ackermann, Electrooxidative dearomatization of biaryls: synthesis of tri- and difluoromethylated spiro[5.5]trienones, Chem. Sci., 2021, 12, 10092–10096 RSC;
(b) W. C. Yang, M. Zhang, Y. Sun, C. Chen and L. Wang, Electrochemical Trifluoromethylthiolation and Spirocyclization of Alkynes with AgSCF3: Access to SCF3-Containing Spiro[5,5]trienones, Org. Lett., 2021, 23, 6691–6696 CrossRef CAS PubMed;
(c) F. Chen, Y. Zheng, H. Yang, Q. Yang, L. Wu and N. N. Zhou, Iron-Catalyzed Silylation and Spirocyclization of Biaryl-Ynones, A Radical Cascade Process toward Silylated Spiro[5.5]trienones, Adv. Synth. Catal., 2022, 364, 1537–1542 CrossRef CAS;
(d) D. Xia and X. F. Duan, Iron-Catalyzed Dearomatization of Biaryl Ynones with Aldehydes via Double C–H Functionalization in Eco-Benign Solvents: Highly Atom-Economical Synthesis of Acylated Spiro[5.5]trienones, J. Org. Chem., 2021, 86, 15263–15275 CrossRef CAS PubMed;
(e) C. R. Reddy and D. H. Kolgave, Electrochemical Selenylative Carbannulation of Biaryl Ynones to Seleno-Dibenzocycloheptenones/Spiro[5.5]Trienones, J Org. Chem., 2021, 86, 17071–17081 CrossRef PubMed;
(f) H. Goulart, R. Bartz, T. Peglow, A. Barcellos, R. Cervo, R. Cargnelutti, R. Jacob, E. Lenardão and G. Perin, J. Org. Chem., 2022, 87, 4273–4283 CrossRef CAS PubMed;
(g) Z. Chen, X. Zheng, S. F. Zhou and X. L. Cui, Visible light-induced selenylative spirocyclization of biaryl ynones toward the formation of selenated spiro[5.5]trienones, Org. Biomol. Chem., 2022, 20, 5779–5783 RSC;
(h) J. Li, Z. Li, L. Shen, P. Li, Y. Zhang and W. C. Yang, Synthesis of polychloromethylated and halogenated spiro[5,5]trienones via dearomative spirocyclization of biaryl ynones, Org. Biomol. Chem., 2022, 20, 6659–6666 RSC;
(i) L. Shen, Y. Sun, Y. Wang, B. Li, W. C. Yang and P. Dai, K2S2O8-promoted radical trifluoromethylthiolation/spirocyclization for the synthesis of SCF3-featured spiro[5,5]trienones, Tetrahedron, 2022, 106, 132649–132652 CrossRef.
-
(a) Z. J. Li, X. Cui, L. Niu, Y. Ren, M. Bian, X. Yang, B. Yang, Q. Yan and J. Zhao, An Iron(II) Chloride-Promoted Radical Cascade Methylation or α-Chloro-β-methylation of N-Arylacrylamides with Dimethyl Sulfoxide, Adv. Synth. Catal., 2017, 359, 246–249 CrossRef CAS;
(b) R. Zhang, H. Yu, Z. J. Li, Q. Yan, P. Li, J. Wu, J. Qi, M. Jiang and L. Sun, Iron-Mediated Azidomethylation or Azidotrideuteromethylation of Active Alkenes with Azidotrimethylsilane and Dimethyl Sulfoxide, Adv. Synth. Catal., 2018, 360, 1384–1388 CrossRef CAS;
(c) Y. Ge, Y. Tian, J. Wu, Q. Yan, L. Zheng, Y. Ren, J. Zhao and Z. J. Li, Iron-promoted free radical cascade difunctionalization of unsaturated benzamides with silanes, Chem. Commun., 2020, 56, 12656–12659 RSC;
(d) Y. Ren, Y. Ge, Q. Yan, S. Chen, Y. Li, L. Li, Z. Q. Liu and Z. J. Li, Free Radical-Promoted Monochloroalkylarylation of Alkenes with Chloralkanes, J. Org. Chem., 2021, 86, 12460–12466 CrossRef CAS PubMed;
(e) Y. Ren, Q. Yan, Y. Li, Y. Gao, J. Zhao, L. J. Li, Z. Q. Liu and Z. J. Li, Free Radical Promoted Trifluoromethylthiolation of Alkynes to Access SCF3-Containing Dibenzazepines or Dioxodibenzothiazepines, J. Org. Chem., 2022, 87, 8773–8781 CrossRef CAS PubMed.
- For selected references, see:
(a) Y. Ban, J. Dai, X. Jin, Q. Zhang and Q. Liu, Thiocyanate radical mediated dehydration of aldoximes with visible light and air, Chem. Commun., 2019, 55, 9701–9704 RSC;
(b) P. Yuan, Q. Zhang, X. Jin, W. Lei, L. Z. Wu and Q. Liu, Visible-light-promoted aerobic metal-free aminothiocyanation of activated ketones, Green Chem., 2018, 20, 5464–5468 RSC;
(c) J. Zhou, P. Zhou, T. Zhao, Q. Ren and J. Li, (Thio)etherification of Quinoxalinones under Visible-Light Photoredox Catalysis, Adv. Synth. Catal., 2019, 361, 5371–5382 CrossRef CAS;
(d) L. Li, J. Li, Y. Sun, C. Luo, H. Qiu, K. Q. Tang, H. X. Liu and W. T. Wei, Visible-Light-Catalyzed Tandem Radical Addition/1,5-Hydrogen Atom Transfer/Cyclization of 2-Alkynylarylethers with Sulfonyl Chlorides, Org. Lett., 2022, 24, 4704–4709 CrossRef CAS PubMed;
(e) X. Yu, C. Zhang, L. Wang, J. Li, T. Li and W. T. Wei, The synthesis of seven- and eight-membered rings by radical strategies, Org. Chem. Front., 2022, 9, 4757–4781 RSC;
(f) D. Wang, L. Li, F. Liu, H. Qiu, J. Li, J. F. Zhang, C. Deng and W. T. Wei, Fe-Catalyzed Selective Formal Insertion of Diazo Compounds into C(sp)–C(sp3) Bonds of Propargyl Alcohols: Access to Alkyne-Substituted All-Carbon Quaternary Centers, ACS Cent. Sci., 2022, 8, 1028–1034 CrossRef CAS PubMed.
- For selected references, see:
(a) B. M. Trost, The Atom Economy – A Search for Synthetic Efficiency, Science, 1991, 254, 1471–1477 CrossRef CAS PubMed;
(b) R. A. Sheldon, The E Factor: fifteen years on, Green Chem., 2007, 9, 1273–1283 RSC.
|
This journal is © The Royal Society of Chemistry 2023 |
Click here to see how this site uses Cookies. View our privacy policy here.