DOI:
10.1039/D3MA00661A
(Paper)
Mater. Adv., 2023,
4, 4954-4964
Synthesis, characterization and cell selectivity of poly(quaternary ammonium chlorides): effect of the degree of quaternization and copolymer composition†
Received
4th September 2023
, Accepted 14th September 2023
First published on 15th September 2023
Abstract
Antimicrobial resistance is a global problem due to widespread evolution of resistant pathogens in both health care and community. Polymeric quaternary ammonium and imidazolium chlorides are well known for their antibacterial activity and have the potential to address this problem. In spite of the widespread reports on quaternary ammonium polymers, there is no facile, common synthetic route available for the preparation of these cationic polymers by which the cationic species can be varied in a facile manner. Particularly, such a common synthetic route should enable changing the cationic source conveniently, altering the charge density of the polymer, and tuning the structure of the cationic polymer to influence various characteristics like the hydrophilic–hydrophobic balance, H-bonding, π–π interactions and donor–acceptor interactions. Importantly, the influence of systematically changing the charge density of the cationic polymer on antibacterial activity as well as its effect on toxicity is not well studied. Moreover, since the properties of polymers can be enhanced through copolymerization, the roles of the other constituents of the cationic polymer introduced through copolymerization on the aforementioned characteristics of the cationic polymer are worth investigating. In this work, we report the synthesis of a series of homo- and copolymers containing various quaternary ammonium groups. We also investigated their selectivity in discriminating microbial and mammalian cells. Combining the antimicrobial and hemolytic activities of the polymers allowed us to obtain structure–activity relationships and identify polymers with good selectivity for further refinement.
Introduction
The increasing prevalence of antibiotic-resistant pathogens is considered as a clinical superchallenge of the 21st century.1 Excessive use of antibiotics, subtherapeutic dosage or too short duration contribute significantly to the evolution of antibiotic-resistant pathogens.2 A number of strategies have been developed with potential to combat drug-resistant pathogens.3 One of the most promising strategies is the design of antimicrobial systems that target multiple metabolic pathways of microbial cells. In this regard, microbial membrane disrupting antimicrobial polymers are attractive due to the increased fitness cost associated with entire membrane formation.4–6 Thus, cationic polymers that are considered as synthetic mimics of naturally occurring biomacromolecules such as antimicrobial peptides/proteins are attractive as a new class of antimicrobial agents.7–9 Polymers have the advantage of diversity of chemical structures, produced and processed on a large scale, as well as excellent biocompatibility and biodegradability.10 The antibacterial activity of polymers arises from their amphiphilicity similar to antimicrobial peptides (AMP) which exhibit their bactericidal properties by a combination of electrostatic and hydrophobic interactions with the cytoplasmic membrane of bacteria.11 In principle, the designed membrane active antimicrobial polymers contain cationic, hydrophobic and hydrophilic co-monomer units with diverse chemical backbones. Polymers bearing permanent positive charges in the form of quaternary ammonium, pyridinium and imidazolium units are well known to possess antibacterial properties.12,13 The major driving force for their activity originates from the interaction of the cationic groups with the negatively charged lipophilic bacterial cell walls/components.14,15 Subsequent insertion of hydrophobic segments of positively charged polymers into the negatively charged lipids of the bacterial cytoplasmic membrane leads to the leakage of vital intracellular components and concomitant cell death.16,17
In principle, there are two general synthetic pathways possible for preparing polymeric quaternary ammonium chlorides. One pathway (Method A, tertiary amine route; Scheme 1) involves preparing tertiary amine containing polymers and subsequently converting these polymers into quaternary ammonium chlorides by reacting with alkyl halides. The second method (Method B, alkyl chloride route; Scheme 1) involves preparing a polymer bearing chloroalkyl groups and in a post-polymer analogous reaction the alkyl chloride is converted to quaternary ammonium chloride by reacting with tertiary amines.
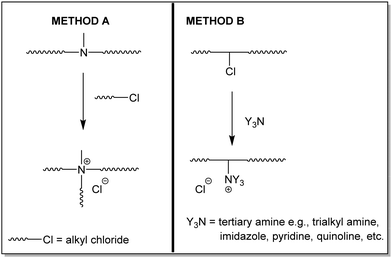 |
| Scheme 1 Synthetic pathways for preparing polymeric quaternary ammonium chlorides by a polymer analogous reaction: (a) Method A – tertiary amine route and (b) Method B – alkyl chloride route. | |
The third possibility of directly using quaternary ammonium monomers to prepare quaternary ammonium polymers does not offer the possibility of tuning quaternary ammonium species in a facile manner because the quaternary ammonium species is predetermined by the choice of monomer. Another possibility that is similar to Method B could involve pendant epoxy groups instead of alkyl chloride. However, there are issues such as high toxicity of the epoxy group bearing monomer, incomplete reaction and unwanted ring opening by nucleophiles that will not result in quaternary ammonium groups.18 Between these two methods, the latter method (Method B) of converting polymeric alkyl chlorides to quaternary ammonium polymers is attractive for many reasons. Firstly, in the method involving the transformation of tertiary amines to ammonium chloride (Method A), the possibility to tune the antimicrobial properties is restricted to varying the alkyl chain length of alkyl chloride alone. In this method, the cationic species remains the same. In contrast, in the latter method (Method B), any kind of tertiary amine, e.g., trialkyl amine, imidazole, pyridine, quinoline, etc., can be used, thereby offering an immense diversity in cationic species. This is important in terms of antimicrobial performance because of the diversity of microorganisms and the associated variation in their cell wall/membrane composition. It may be noted here that the charge density of quaternary ammonium polymers plays a vital role in antimicrobial properties.19,20 The charge density is predominantly determined by the nature and concentration of the tertiary amine. The high charge density is also the source of the hemolytic and cytotoxic nature of these polymers.21,22 Thus, an intricate balance between hydrophobicity and cationic charge is essential for the design of antimicrobial polymers that are more selective towards microbial cells than mammalian cells. Copolymerization is an often employed strategy to modify and impart desired characteristics to polymers.23 To balance the inherently conflicting characteristics between antibacterial nature and cytotoxicity to human cells and to broaden the applications of these polymers, it is useful to modify these cationic polymers. Attempts have been made in this regard by tuning factors like the charge density, molecular weight, active moiety, alkyl chain length, etc. and in general the amphiphilicity of these polymers.24–33
Some of the essential requirements for implementing Method B are the ease of availability of raw materials, favorable reactivity ratios between comonomers,34 and facile reaction with tertiary amines in the polymer analogous reaction to achieve quantitative transformation to quaternary ammonium units. One such vinyl monomer that fulfills all these requirements is vinyl benzyl chloride, such as 3- or 4-vinyl benzyl chloride. This is due to the separation of the chloroalkyl moiety from neighboring groups which helps to avoid any steric hindrance in the polymer analogous reaction, leading to the formation of quaternary ammonium salts. Polymers or copolymers of vinyl benzyl chloride have predominantly been used as anion exchange membranes for various industrial applications.35–38 Historically, (co)polymers of vinyl benzyl chloride have been used in Merrifield resins that are part of solid phase synthesis, in gas chromatography, phase transfer catalysis and many other applications.39–46 To the best of our knowledge, there is no report detailing the facile preparation of poly(vinyl quaternary ammonium chloride)s with varying quaternary ammonium species starting from 4-vinyl benzyl chloride, except for two reports that contain specific derivatives such as poly(vinyl benzyl trimethylammonium chloride) and 4-vinyl benzyl dimethylhexadecylammonium chloride.47–49 Considering that benzalkyl ammonium chlorides are widely used50 as disinfectants, ophthalmic preservatives and antiseptics, quaternary ammonium polymers obtained by the quaternization of homo- and copoly(4-vinyl benzyl chloride) can be potentially used as antimicrobial agents. Thus, in spite of the usefulness of this approach for preparing a diverse range of homo- and copoly(vinyl quaternary ammonium and imidazolium chloride)s, a facile route for preparing these polymers conveniently from readily available starting materials has not been reported. The overall objectives of this work are to determine the antimicrobial properties and hemolytic activity of homo- and copolymers of PVBC bearing various quaternary ammonium groups and correlate their structure–activity relationship. We anticipate that this would deepen our understanding and rational design of cell selective antimicrobial polymers.
Results and discussion
Preparation of homopoly(quaternary ammonium chloride)s
The homopolymers HP 1 to 5 were prepared by a two-step process as shown in Scheme 2. 4-Vinylbenzyl chloride was polymerized by free radical polymerization to obtain poly(4-vinyl benzyl chloride) (PVBC). PVBC was soluble in common organic solvents like chloroform and tetrahydrofuran and insoluble in methanol. PVBC was subsequently converted to quaternary ammonium polymers with tertiary amines, tertiary amines bearing hydroxyl group(s) and with 1-methyl imidazole (MIz). A mixed quaternary ammonium chloride homopolymer, HP 4, was prepared by the quaternization of PVBC with triethyl amine (TEA) and N,N-dimethyl aminoethanol (DMAE). The tertiary amines were chosen in such a way to vary the nature of the cation as well as to study the effect of polar functional groups on the antimicrobial properties and hemolytic behavior of cationic homopolymers.
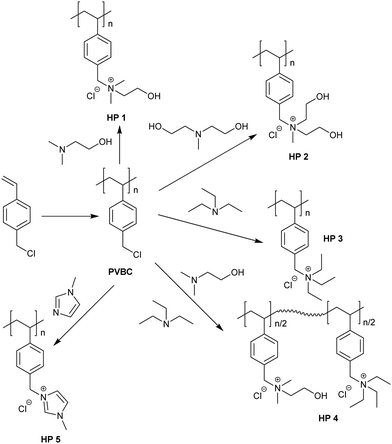 |
| Scheme 2 Preparation of PVBC by free radical polymerization and homopoly(quaternary ammonium chloride)s through polymer analogous reactions. | |
All the polymers were thoroughly characterized by spectroscopic techniques. Due to the insolubility in common organic solvents like tetrahydrofuran as well as the strong adsorption of quaternary ammonium polymers in gel permeation chromatographic columns, the molecular weight of HP 1 to 5 was calculated from that of PVBC by following a literature procedure.51 PVBC was soluble in THF and its molecular weight was determined by GPC analysis. Fig. S1 (ESI†) shows the GPC of PVBC. PVBC was converted to the corresponding polymeric quaternary ammonium chloride quantitatively in a facile manner, by refluxing with tertiary amine in methanol. Predominantly, the originally insoluble PVBC formed a clear solution in methanol upon converting to the quaternary ammonium polymer. Table 1 provides the molecular weight details of homopoly(quaternary ammonium chloride)s.
Table 1 Composition and molecular weight of homopoly(quaternary ammonium chloride)s
Polymer code |
Tertiary amine |
Molecular weight (g mol−1) |
PVBC |
Nil |
12 040 |
HP 1 |
N,N-Dimethyl aminoethanol (DMAE) |
19 100 |
HP 2 |
N-Methyl diethanolamine (NMDEA) |
21 470 |
HP 3 |
Triethylamine (TEA) |
20 050 |
HP 4 |
DMAE and TEA |
19 580 |
HP 5 |
1-Methylimidazole (MIz) |
18 540 |
Preparation of copolymers of VBC with styrene (PVBC-co-Sty)
Scheme 3 shows the copolymerization of VBC with styrene. VBC was copolymerized with styrene in different molar ratios, viz., 0.25
:
0.75, 0.5
:
0.5 and 0.75
:
0.25, respectively. The molar ratio of VBC was varied to study the effect of changing the concentration of cations on antimicrobial properties and the hemolytic behavior.
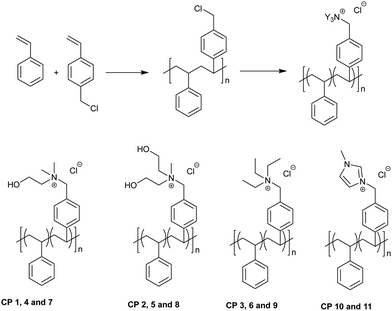 |
| Scheme 3 Preparation of copolymers of VBC-co-Sty and the copolymeric quaternary ammonium chlorides. | |
The molecular weight details of intermediate copolymers as determined by gel permeation chromatography using THF as the eluent are given in Table 2. Fig. S2 to S7 (ESI†) show the GPC of precursor copolymers. A detailed explanation is given in the Experimental section for determining the degree of polymerization (DP) of respective monomers (VBC and Sty or MMA) in the copolymer as well as the molecular weight of copolymers after quaternization. GPC analysis showed that the molecular weights of copolymers, PVBC-co-Sty, were comparable and so also the polydispersity. Since the molecular weight of 4-VBC (152.62) is higher than that of styrene (104.15), the increasing concentration of VBC in copolymers would cause an increase in the molecular weight of the copolymers as well. As compared to the molecular weight of homopolymers, PVBC, the molecular weight of copolymers was lower with the degree of polymerization being reduced by about 8 units in the copolymerization with styrene. This is likely due to the lower propagation rate of styrene radicals.52 In the case of copolymerization of VBC with MMA, the molecular weight of copolymers increased with the concentration of MMA. This is due to the higher propagation rate of MMA radicals.52 Because of this reason, the molecular weights of VBC-co-MMA copolymers were higher than that of VBC-co-Sty.
Table 2 Molecular weights of copolymers of VBC-co-Sty and VBC-co-MMA
Copolymer composition |
DP of VBC in the copolymer |
DP of comonomer in the copolymer |
M
n
|
M
w
|
Đ
|
VBC0.25–Sty0.75 |
16 |
72 |
9940 |
16 150 |
1.6 |
VBC0.5–Sty0.5 |
32 |
48 |
9930 |
16 820 |
1.7 |
VBC0.75–Sty0.25 |
50 |
25 |
10 230 |
17 780 |
1.7 |
VBC0.25–MMA0.75 |
28 |
127 |
16 940 |
37 040 |
2.2 |
VBC0.5–MMA0.5 |
49 |
75 |
14 980 |
31 960 |
2.1 |
VBC0.75–MMA0.25 |
58 |
29 |
11 790 |
33 920 |
2.9 |
The composition and molecular weight details of quaternary ammonium chlorides derived from copolymers of VBC-co-Sty are given in Table 3.
Table 3 Compositions and molecular weights of copolymeric quaternary ammonium chlorides of VBC-co-Sty
Polymer code |
Copolymer composition |
Molecular weight (g mol−1) |
CP 1 |
(DMAE–VBC)0.25–Sty0.75 |
11 370 |
CP 2 |
(NMDEA–VBC)0.25–Sty0.75 |
11 850 |
CP 3 |
(TEA–VBC)0.25–Sty0.75 |
11 560 |
CP 4 |
(DMAE–VBC)0.5–Sty0.5 |
12 820 |
CP 5 |
(NMDEA–VBC)0.5–Sty0.5 |
13 790 |
CP 6 |
(TEA–VBC)0.5–Sty0.5 |
13 210 |
CP 7 |
(DMAE–VBC)0.75–Sty0.25 |
14 760 |
CP 8 |
(NMDEA–VBC)0.75–Sty0.25 |
16 260 |
CP 9 |
(TEA–VBC)0.75–Sty0.25 |
15 360 |
CP 10 |
(MIz–VBC)0.25–Sty0.75 |
Insoluble |
CP 11 |
(MIz–VBC)0.75–Sty0.25 |
14 400 |
The copolymers VBCa–Styb were insoluble in methanol. However, similar to the homopoly(quaternary ammonium chloride)s, the quaternary ammonium chlorides of copolymers were also soluble in methanol with the exception of CP 10. CP 10 possibly due to the lower degree of quaternization was insoluble in methanol and deionized water and hence was not evaluated further. For this reason, the 50–50 copolymer (MIz–VBC)0.5–Sty0.5 was also not prepared. However, the 75
:
25 copolymer, CP 11, (MIz–VBC)0.75–Sty0.25 was prepared and found to be soluble in methanol although the solution was slightly translucent unlike the transparent solution of other quaternized polymers in methanol.
To study the effect of the constituents of copolymers on their antimicrobial and hemolytic behaviors, another series of copolymers were prepared. In these copolymers, styrene was replaced by methyl methacrylate (MMA). As discussed in subsequent sections, since the MIC increased with lowering concentration of quaternary ammonium units in copolymers, only selected copolymers with higher concentrations of cationic groups were prepared and studied. However, to facilitate comparison cationic functionalities with 50% concentration were also prepared, for example, CP 14 and 15, which contained cationic charges at 50 and 75%, respectively. TEA was chosen for this purpose since the TEA derivative of the homopolymer showed a low MIC in the homopolymer series (HP 3). Scheme 4 shows the copolymerization of VBC with MMA.
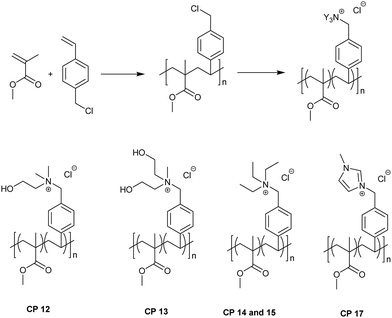 |
| Scheme 4 Preparation of copolymers of VBC-co-MMA and the copolymeric quaternary ammonium chlorides. | |
In addition to the copolymeric quaternary ammonium chlorides, similar to HP 4, copolymers with mixed quaternary ammonium groups were also prepared. The chemical structure of the copolymers with mixed quaternary ammonium groups is shown in Fig. 1.
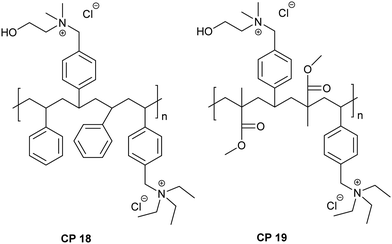 |
| Fig. 1 Chemical structure of mixed copoly(quaternary ammonium chloride)s. | |
The composition and molecular weight details of quaternary ammonium chlorides derived from copolymers of VBC-co-MMA and those of mixed copoly(quaternary ammonium chloride)s are given in Table 4.
Table 4 Compositions and molecular weights of copolymeric quaternary ammonium chlorides of VBC-co-MMA and mixed copoly(quaternary ammonium chloride)s
Polymer code |
Copolymer composition |
Molecular weight (g mol−1) |
CP 12 |
(DMAE–VBC)0.75–MMA0.25 |
16 970 |
CP 13 |
(NMDEA–VBC)0.75–MMA0.25 |
18 710 |
CP 14 |
(TEA–VBC)0.5–MMA0.5 |
19 970 |
CP 15 |
(DMAE–VBC)0.75–MMA0.25 |
17 670 |
CP 16 |
(MIz–VBC)0.5–MMA0.5 |
19 030 |
CP 17 |
(MIz–VBC)0.75–MMA0.25 |
16 560 |
CP 18 |
(DMAE–VBC)0.375–(TEA–VBC)0.375–Sty0.25 |
15 060 |
CP 19 |
(DMAE–VBC)0.375–(TEA–VBC)0.375–Sty0.25 |
17 320 |
The composition of copolymers and degree of quaternization can be conveniently established by 1H-NMR spectroscopic analysis of polymers. 1H-NMR spectroscopic analysis indicated that the quaternization was quantitative. Fig. 2 shows the 1H-NMR spectrum of CP 4. The 1H-NMR spectra of all other polymers are shown in Fig. S8 to S37 (ESI†).
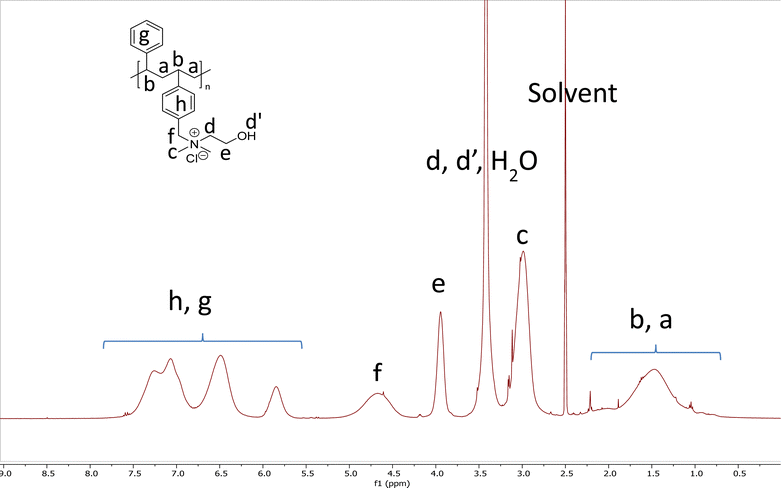 |
| Fig. 2
1H-NMR spectrum of CP 4 (solvent: DMSO-d6). | |
Determination of the minimum inhibitory concentration (MIC) and hemolytic activity of homopolymers
Antimicrobial polymers elicit bactericidal properties by disrupting the cytoplasmic membrane of the bacteria. To achieve optimum antimicrobial properties, the polymer must encompass cationic charges and hydrophobic and hydrophilic ratios. Therefore, the antibacterial properties of homopolymers were evaluated against American Type Culture Collection (ATCC) strains of P. aeruginosa, S. aureus and C. albicans. The MIC values are given in Table 5. S. aureus and P. aeruginosa strains were susceptible to HP 1–HP 4. HP 4 which contained mixed quaternary ammonium ions had the lowest MIC against Pseudomonas bacterial strains. These results suggest that the polymers could target both Gram-positive and Gram-negative bacteria despite the differences in their cell wall structure. However, none of the polymers displayed potent antimicrobial activity against C. albicans strains, suggesting the protective role of the chitin cell wall present in the microorganisms. Homopolymeric quaternary ammonium chlorides derived from imidazolium ions displayed the poorest antibacterial properties as no observable inhibitory effect was detected at the maximum polymer concentration (25 μM) tested.
Table 5 Antimicrobial and hemolytic activities of homopoly(quaternary ammonium chloride)s against bacterial and fungal pathogens
Polymer |
MIC, μM |
Hemolytic activity (%) at MIC |
P. aeruginosa
|
S. aureus
|
C. albicans
|
HP 1 |
6.5 |
6.5 |
>25 |
8.7 |
HP 2 |
11.6 |
11.6 |
>25 |
12.3 |
HP 3 |
6.2 |
6.2 |
>25 |
31.9 |
HP 4 |
3.2 |
6.3 |
>25 |
70.5 |
HP 5 |
>27 |
>27 |
>27 |
98.8 |
To infer if the synthesized polymers discriminate between microbial and mammalian cells, we determined the hemolytic activity of the polymers for rabbit erythrocytes. Fig. 3 compares the concentration-dependent hemolytic activity of various polymers. For comparison, hemolytic activity at their MICs is provided in Table 5. As shown in Table 5, DMEA and NMDEA displayed the lowest hemolytic activity. Both the polymers caused <20% hemolysis even at the highest concentration tested. The polymer containing TEA (HP 3), however, lysed 31.9% of rabbit erythrocytes, whereas HP 4 which contained a mixture of DMAE and TEA caused 70.5% hemolysis at their MIC values. HP 5 which contains imidazolium salt displayed pronounced hemolytic activity (86.6) at 25 mM, despite the lack of antibacterial activity. These results suggest superior membrane selectivity of homopolymers containing DMEA/NMDEA cationic charges. Based on the overall performance, the selectivity of homopolymeric quaternary ammonium chlorides follows the order: HP 1 > HP 2 ≥ HP 3 > HP 4 > HP 5. The high MIC shown by HP 5 is possibly due to the reduction of hydrophobicity. It may be noted that the antibacterial activity of imidazolium ions is dependent on factors like the alkyl chain length of the N-substituted imidazolium moiety.9
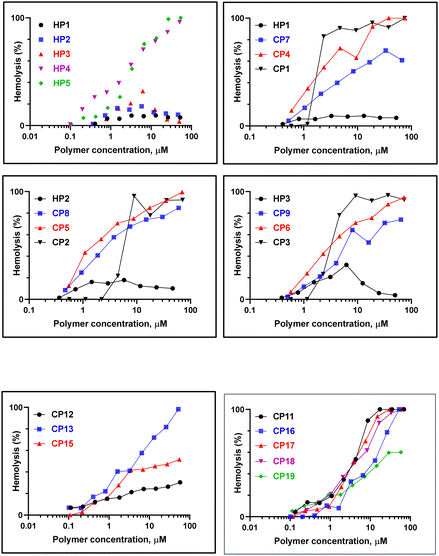 |
| Fig. 3 Concentration-dependent hemolytic activity of homo- and copolymers for rabbit erythrocytes. | |
Cell selectivity of quaternary ammonium chlorides of copolymers VBC-co-Sty and VBC-co-MMA
To probe the effect of hydrophobicity and the degree of quaternization, we synthesized a series of copolymers containing varying concentrations of polyvinyl units such as those derived from styrene (Sty) and methyl methacrylate (MMA). Unlike the homopolymers where the quaternization alters the polarity to a large extent, in copolymers the presence of polymerized vinyl units helps to retain part of the hydrophobic character even after quaternization. It may be noted that the polarity change after quaternization will also be decreased due to the lower concentration of VBC units in the copolymers of VBC-co-Sty and VBC-co-MMA. Therefore, we investigated the effect of varying the VBC to vinyl ratio on the antimicrobial activity and hemolytic activity. Table 6 shows the MICs of copolymeric quaternary ammonium chlorides containing various ratios of VBC to styrene and MMA. The results suggest that increasing the number of styrene units decreases the MIC value against both P. aeruginosa and S. aureus strains initially for the copolymer containing DMAE but increases when the ratio is increased further. At their MIC values, CP 7 caused 40% hemolysis, whereas CP 4 and CP 1 caused complete lysis of rabbit erythrocytes, suggesting lack of cell selectivity upon increasing the styrene content (Fig. 3).
Table 6 MICs of copoly(quaternary ammonium chloride)s of VBC-co-Sty and VBC-co-MMA
Polymer |
MIC, μM |
Hemolytic activity (%) at MIC |
P. aeruginosa
|
S. aureus
|
CP 1 |
>44.0 |
>44.0 |
91.4 |
CP 4 |
39 |
>39 |
100 |
CP 7 |
4.2 |
4.2 |
40.0 |
CP 2 |
>42.2 |
>42.2 |
92.0 |
CP 5 |
36.3 |
>36.3 |
91.5 |
CP 8 |
15.4 |
15.4 |
74.0 |
CP 3 |
>43.3 |
>43.3 |
96.6 |
CP 6 |
>37.9 |
>37.9 |
88.2 |
CP 9 |
16.3 |
16.3 |
52.7 |
CP 10 |
Insoluble |
Insoluble |
N.D. |
CP 11 |
34.7 |
34.7 |
100 |
CP 12 |
14.7 |
14.7 |
24.5 |
CP 13 |
26.7 |
6.7 |
81.7 |
CP 15 |
7.0 |
7.0 |
42.3 |
CP 14 |
12.5 |
6.3 |
83.8 |
CP 11 |
34.7 |
34.7 |
100 |
CP 16 |
26.3 |
26.3 |
78.7 |
CP 17 |
30.2 |
30.2 |
100 |
CP 18 |
33.2 |
>33.2 |
96.7 |
CP 19 |
7.2 |
14.4 |
47.4 |
For the homopolymers containing NMDEA and TEA, addition of styrene blocks increased the MIC values. As was observed for DMAE copolymers, increasing the polystyrene content resulted in heightened hemolytic activity when compared to homopolymers. These results suggest that, for the two copolymers containing NMDEA and TEA, increasing the number of styrene units and decreasing the degree of quaternization render the polymer more hemolytic than the homopolymers. It has been observed that, for a number of quaternized polymers, the balance between the charge and hydrophobicity of polymers is a key determinant of cell selectivity. Unlike the quaternized VBC homopolymers, the introduction of hydrophobic units resulted in loss of cell selectivity in copolymers.
To determine if replacement of polystyrene units with poly(methyl methacrylate) would enhance the cell selectivity, we examined the antibacterial and hemolytic properties of VBC–methyl methacrylate copolymers. Since the MIC at lower degrees of quaternization (25 and 50%) was appreciably higher in the case of copolymers of VBC-co-Sty, in VBC-co-MMA we compared the properties of polymers that had 75% quaternization (Table 6). The results suggest only a moderate improvement in the selectivity for the polymers containing NMDEA (cf. CP 8 and CP 13), whereas a marked improvement in selectivity was observed for the polymer containing DMAE (cf. CP 7 and CP 12) and TEA (cf. CP 9 and CP 15), the latter being more selective than copolymers containing DMAE alone. In addition, copolymers containing imidazolium groups displayed poor selectivity than the homopolymer (cf. HP 5, CP 11, CP 16 and CP 17). We further investigated the effect of copolymers that contained a mixture of quaternized ammonium groups while maintaining the concentration of ionic units at 75% (Table 6). Both the mixed quaternary ammonium copolymers (CP 18 and CP 19) displayed substantial hemolytic activity at their MIC values, suggesting poor selectivity of these polymers.
Cell selectivity of homo- and copolymers
Since low MIC values and low hemolytic activity are the hallmarks of an ideal antimicrobial agent, we combined the two properties to identify homo/copolymers with optimum therapeutic value (Fig. 4). For polymers wherein no MIC was observed, 2× the highest concentration tested was taken as the MIC. The resultant graph was divided into 4 quadrants in terms of their antimicrobial and hemolytic activities. Based on this, we identified that HP 1 and HP 2 displayed greater selectivity than the other polymers, while HP 5, CP 1–3 and CP 6 were highly hemolytic with poor antimicrobial properties. The other polymers were highly hemolytic with appreciable antimicrobial properties. Interestingly, all the imidazolium containing homo- and copolymers displayed low antimicrobial and high hemolytic activities, suggesting their lack of cell selectivity.
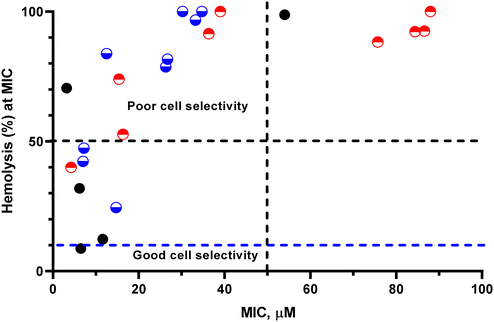 |
| Fig. 4 Cell selectivity of homo- and copolymers. The vertical and horizontal dotted lines are shown to indicate 4 quadrants in terms of antimicrobial and hemolytic activities. The blue dotted line indicates 10% hemolytic activity at the MIC. Quaternary VBC homopolymers and VBC-co-Sty and VBC-co-MMA copolymers are indicated in black, red and blue half-filled circles, respectively. | |
Conclusion
A general synthetic strategy was designed to prepare homo- and copoly(quaternary ammonium chloride)s. Named as the alkyl chloride route, this offers immense possibilities for varying the type and nature of tertiary amines that are the source of cationic charges in quaternary ammonium polymers. Through the alkyl chloride route, both homo- and copoly(4-vinyl benzyl chloride)s were converted to the respective homo- and copoly(quaternary ammonium chloride)s with hydroxyl group bearing tertiary amines, tertiary amines free from functional groups and imidazole in a facile manner. The antibacterial and hemolytic properties of homo- and copoly(quaternary ammonium chloride)s were investigated to identify polymers with good cell selectivity in discriminating between microbial and mammalian cells. We determined that cell selectivity was dependent on the overall percentage of quaternary ammonium ions and copolymer composition. The results from this study would allow us to refine the polymer properties further and design cell selective polymers for combating antibiotic-resistant pathogens.
Experimental
Materials and methods
1-Vinylimidazole (≥99%), styrene (St, ≥99%), methyl methacrylate (MMA, 99%), 1,3,5-trioxane (≥99%), benzyl chloride (99%), 4-vinyl benzyl chloride (≥90%) (4VBC), triethylamine (≥99.5%), N-methyl diethanolamine (99%), N,N-dimethylethanolamine (≥99.5%), and 1-methyl imidazole (99%) were obtained from Sigma-Aldrich and used without further purification. 2,2′-Azobis(isobutyronitrile) (AIBN) (Wako Chemicals) was recrystallized from methanol prior to use. N,N-dimethylformamide (DMF) (≥98%) and toluene (≥98%) were obtained from VWR and used as received. All other reagents were obtained from commercial sources and used as received.
Instrumental analysis
Liquid state nuclear magnetic resonance (NMR) spectroscopy was performed using a Bruker 400 MHz NMR spectrometer. 1H-NMR spectra were obtained with 64 scans. The molecular weights of uncharged precursor polymers were determined by gel permeation chromatography (GPC) using a Waters GPC system consisting of integrated solvent and the sample delivery module. Tetrahydrofuran (THF) was used as the eluent at a flow rate of 1 mL min−1 at 40 °C and molecular weight values were determined against polystyrene standards.
Determination of the degree of quaternization
The degree of quaternization was determined by comparing the intensity of proton signals corresponding to the benzylic proton with that of the signals corresponding to the alkyl groups or preferably methylene units attached with the tertiary amine used for the quaternization reaction. For example, as shown in Fig. 2, the intensity of benzylic protons appearing as a broad signal in the range 4.25–5.25 ppm and that of the methylene protons α- to the hydroxyl group (–CH2–CH2–OH) at 3.75–4.1 ppm of CP 4 are the same, indicating that quaternization is quantitative. The following formula was used to determine the degree of quaternization:
Degree of quaternization = [(intensity of benzylic protons)/(intensity of selected methylene protons attached to tertiary amine)] × 100
The conversion is 1 if the quaternization is quantitative and is lower than 1 for lower degrees of quaternization.
Determination of the molecular weights of quaternized homopolymers
The molecular weight (MW) was calculated using a reported procedure40 according to the following equation:
M
W of quaternized homopolymer = {DP × [(MW of VBC + MW of tertiary amine) × conversion]}
DP = [(Mn of PVBC as determined by GPC/MW of VBC)
Thus, the MW of HP 1 was calculated as follows:
DP = 12
042/152.62 = 79
M
W = {79 × [(152.62 + 89.14) × 1} = 19
099 ≈ 19
100.
Determination of the molecular weights of quaternized copolymers
To determine the molecular weights of copolymeric quaternary ammonium chlorides, the degree of polymerization (DP) of precursor copolymers (VBC-co-M) were calculated as follows:
DP of VBC in copolymer = [(Mn as determined by GPC/MW of VBC) × concentration of VBC in copolymer]
DP of comonomer = [(Mn as determined by GPC/MW of comonomer) × concentration of comonomer in copolymer]
DP of copolymer = DP of VBC + DP of comonomer
After determining the degree of polymerization of VBC and comonomers, the molecular weight of copolymeric quaternary ammonium chlorides was determined by following a literature procedure40 as explained below.
An example is shown below for VBC0.25–Sty0.75:
DP of Sty0.75–VBC0.25 = [(9937/104.15) × 0.75] + [(9937/152.62) × 0.25] = 72 + 16
Molecular weight = [(72 × 104.15) + (16 × 152.65)] = 9940 (this compared well with the value of 9937 g mol−1 determined by GPC analysis).
Using the above DP, the MW of CP 1 was determined as follows:
M
W of CP 1 = {(DP of Sty in copolymer × MW of Sty) + [DP of VBC in copolymer × (MW of VBC + MW of DMAE) × 1]}
M
W of CP 1 = {(72 × 104.15) + [16 × (152.62 + 89.14) × 1]} = 11
367 ≈ 11
370.
Copolymerization of 4-vinylbenzyl chloride and St/MMA
St or MMA (0.05 mol), 4-vinylbenzyl chloride (0.05 mol), trioxane (3 mmol), AIBN (1 mmol) and toluene (50 g) were charged into a 4-neck European flask (Chemglass) with 4 baffles. A magnetic stirrer coupling (Premex-Glenfiz) and overhead stirrer (Heidolph) were used for continuous stirring with the help of a half-moon blade. The monomer mixture was purged with argon for at least 30 min prior to polymerization and maintained under an argon atmosphere during the polymerization for 18–20 h at 72 °C (temperature of oil-bath) and stirred at 400–500 rpm. The polymerization solution was precipitated in methanol, dried under vacuum at 50–60 °C and analyzed by GPC and 1H-NMR.
VBC0.5–MMA0.5: GPC (THF eluent): av. Mn = 14
983 av. Mw = 31
957 Đ = 2.1; 1H-NMR (400 MHz, DMSO-d6, δ): 0.25–1.1 (b, –CH3), 1.25–2 (b, –CH2), 2–2.5 (b, –CH), 3.25–3.5 (b, –COOCH3), 4.5–4.8 (b, Ar-CH2), 6.3–7.1 (b, ArH), 7.1–7.5 (b, ArH).
VBC0.5–Sty0.5: GPC (THF eluent): av. Mn = 9933 av. Mw = 16
823 Đ = 1.69; 1H-NMR (400 MHz, DMSO-d6, δ): 1.2–2.25 (b, –CH2, –CH), 4.5–4.8 (b, Ar-CH2), 6.1–6.75 (b, ArH), 6.75–7.3 (b, ArH).
General procedure for quaternization of copolymers VBC0.5–Sty0.5
The copolymer VBC-co-Sty (0.5615 g, 0.0022 mol) was dispersed in methanol (10 mL). Triethylamine (0.2178 g, 0.0022 mol) was added and then the mixture was refluxed. The reaction mixture became a clear solution after 2 h of reflux. The reaction mixture was refluxed for 24 h and cooled. The solution was concentrated and washed thoroughly with diethyl ether. The copolymer after quaternization was soluble in water. The copolymers were characterized by 1H-NMR spectroscopic analysis. Fig. 2 shows the 1H-NMR spectra of CP 4 along with assignment of signals. The 1H-NMR spectra of other homo- and copolymeric quaternary ammonium chlorides are shown in the ESI.†
Antimicrobial assay
The minimum inhibitory concentration (MIC) was determined according to Wikler et al.53 The MIC was defined as the lowest concentration that prevented the visible growth of the bacteria and was determined using the broth microdilution method in 96-well plates. The MIC is the lowest concentration of tested polymers that completely inhibits the growth, disregarding a single colony or a faint haze caused by the inoculum. The antimicrobial properties of the polymers were assessed in terms of their ability to inhibit the bacterial growth in full strength Mueller–Hinton broth (MHB) for bacterial isolates and Sabouraud dextrose broth (SDB) for C. albicans. Bacterial cultures were grown overnight on tryptic soy agar (TSA) and Candida cultures were cultured on Sabouraud dextrose agar (SDA) plates, respectively. The cell density was adjusted to achieve the turbidity equivalent to a 0.5 McFarland standard. This results in a suspension containing approximately 1–2 × 108 colony-forming units (CFU) mL−1. Optimally, within 15 minutes of preparation, the adjusted inoculum suspension was diluted in broth to achieve a cell density of approximately 5 × 105 CFU mL−1. Hundred mL of inoculum was mixed with equal volumes of polymers at 2× their concentration in 96-well plates. Positive and negative controls contained 200 mL of inoculum without any polymers and broth alone, respectively. The 96-well plates were then incubated at 37 °C for 18–24 h. All the experiments were performed in duplicate and the MICs were determined as the lowest concentration in which no visible growth was observed. The presence or absence of visible growth was monitored at 20–24 h (at 35 ± 2 °C) upon incubation. MIC experiments were conducted as described by the clinical and laboratory standards institute (CLSI) guidelines and the MIC results were expressed in μM.
Hemolytic assay
The blood compatibility of each polymer sample was evaluated with the hemolysis assay. Fresh rabbit blood was extracted through the ear using a yellow Neoflon (24 G) needle. The rabbit was anesthetized prior to the procedure and blood samples were collected using EDTA anticoagulant tubes (3.8%, 3 mL; Greiner Bio-One). Polymers were weighed exactly (10 mg mL−1) and the diluent buffer HEPES (50 mM, 10 mM NaCl; pH 7.4) was prepared accordingly. Polymer samples were diluted and were 100% soluble in phosphate-buffered saline (PBS). Testing the concentration of each polymer started with 1 mg mL−1. Eighty microliters of polymers were aliquoted into 320 μL of HEPES to yield 400 μL of the final concentration and double dilution was performed (the assay concentration ranged from 1 mg mL−1 to 1.95 μg mL−1). Red blood cells (RBCs) were washed with HEPES two times and centrifuged (3000 rpm; 4 °C; 10 min). The supernatant was removed by aspiration and erythrocytes were washed by adding 2 mL of HEPES. The washing step was repeated at least three times or until the supernatant was completely removed. After discarding the supernatant, the erythrocyte pellet was diluted to obtain 8% erythrocyte suspension by adding HEPES. Two hundred microliters were aliquoted to all the tubes, yielding a final concentration of 4%. Meanwhile, 200 μL of HEPES were added into 200 μL of 8% erythrocyte suspension for negative control; 200 μL of triton 4% was added to 200 μL of 8% erythrocyte suspension for positive control. The samples were then incubated at 37 °C in a shaker incubator for 1 h at 300 rpm. Subsequently, the samples were centrifuged at 3000 rpm for 3 minutes at 4 °C. Finally, 100 μL samples were transferred into 96-well plates. The absorbance of the hemolytic assay was measured at 576 nm using an Infinite M200 microplate reader (Tecan Group Ltd, Switzerland). The experiment contained at least two technical replicates (i.e., two wells per sample). The hemolytic degree was expressed in terms of the hemolytic ratio using the following formula:
Author contributions
WR contributed to investigation and methodology. PMH contributed to the investigation and methodology. LXJ contributed to conceptualization, funding acquisition, and writing – review and editing. RL contributed to methodology, validation, funding acquisition, resources, review and editing. AP contributed to conceptualization, investigation, formal analysis, funding acquisition, methodology, project administration, supervision and writing the original draft, review and editing.
Conflicts of interest
There are no conflicts to declare.
Acknowledgements
The financial support from the Agency for Science, Technology and Research (A*STAR), Singapore is gratefully acknowledged. This work was funded in part by the Agency for Science, Technology and Research (A*STAR), Singapore under Advanced Manufacturing and Engineering – Industry Alignment Fund (Pre-Positioning) (AME IAF-PP/A1786a0030). The authors gratefully acknowledge Ms Thi Bich Van Nguyen of Singapore Institute of Food and Biotechnology Innovation (SIFBI), Agency for Science, Technology and Research (A*STAR), Singapore for her help in preliminary screening of the antibacterial activity of some of the copolymers. RL acknowledges the funding support from the Singapore Ministry of Health's National Medical Research Council under its Centre Grant Program (MOH-001001-00) and Open Funding—Independent Research Grant (MOH-000963-00).
Notes and references
- C. A. Arias and B. E. Murray, New Engl. J. Med., 2009, 360, 439 CrossRef CAS PubMed
.
- D. I. Andersson and D. Hughes, Nat. Rev. Microbiol., 2014, 12, 465 CrossRef CAS PubMed
.
- U. Theuretzbacher, K. Outterson, A. Engel and A. Karlén, Nat. Rev. Microbiol., 2020, 18, 275 CrossRef PubMed
.
- B. P. Lazzaro, M. Zasloff and J. Rolff, Science, 2020, 368, eaau5480 CrossRef CAS PubMed
.
- R. E. Hancock and H. G. Sahl, Nat. Biotechnol., 2006, 24, 1551 CrossRef CAS PubMed
.
- N. Mookherjee, M. A. Anderson, H. P. Haagsman and D. J. Davidson, Nat. Rev. Drug Discov., 2020, 19, 311 CrossRef CAS PubMed
.
- B. Mishra, S. Reiling, D. Zarena and G. Wang, Curr. Opin. Chem. Biol., 2017, 38, 87 CrossRef CAS PubMed
.
- L. A. T. W. Asri, M. Crismaru, S. Roest, Y. Chen, O. Ivashenko, P. Rudolf, J. C. Tiller, H. C. van der Mei, T. J. A. Loontjens and H. J. Busscher, Adv. Funct. Mater., 2014, 24, 346 CrossRef CAS
.
- N. Duan, Z. Sun, Y. Ren, Z. Liu, L. Liu and F. Yan, Polym. Chem., 2020, 11, 867 RSC
.
- P. Pham, S. Oliver and C. Boyer, Macromol. Chem. Phys., 2023, 224, 2200226 CrossRef CAS
.
- M. Haktaniyan and M. Bradley, Chem. Soc. Rev., 2022, 51, 8584 RSC
.
- H. Qiu, Z. Si, Y. Luo, P. Feng, X. Wu, W. Hou, Y. Zhu and M. B. Chan-Park, Front. Bioeng. Biotechnol., 2020, 8, 910 CrossRef PubMed
.
- Y. Ma, S. Xu, P. Yue, H. Cao, Y. Zou, L. Wang, H. Long, S. Wu and Q. Ye, Carbohyd. Polym., 2023, 306, 120575 CrossRef CAS PubMed
.
- S. N. Riduan and Y. Zhang, Chem. Soc. Rev., 2013, 42, 9055 RSC
; S. N. Riduan and Y. Zhang, Chem. Soc. Rev., 2013, 42, 9055 RSC
.
- K. Littunen, J. S. De Castro, A. Samoylenko, Q. Xu, S. Quaggin, S. Vainio and J. Seppälä, Eur. Polym. J., 2016, 75, 116 CrossRef CAS
.
- J. Qin, J. Guo, Q. Xu, Z. Zheng, H. Mao and F. Yan, ACS Appl. Mater. Interfaces, 2017, 9, 10504 CrossRef CAS PubMed
.
- X. Ding, C. Yang, T. P. Lim, L. Y. Hsu, A. C. Engler, J. L. Hedrick and Y.-Y. Yang, Biomaterials, 2012, 33, 6593 CrossRef CAS PubMed
.
- R. F. Dsouza and A. Parthiban, J. Polym. Sci. Part A: Polym. Chem., 2018, 56, 626 CrossRef CAS
.
- J. Chen, C. Bao, R. Han, G.-Z. Li, Z. Zheng, Y. Wang and Q. Zhang, Polym. Chem., 2022, 13, 2285 RSC
.
- T. J. Cuthbert, B. Hisey, T. D. Harrison, J. F. Trant, E. R. Gillies and P. J. Ragogna, Angew. Chem., Int. Ed., 2018, 57, 12707 CrossRef CAS PubMed
.
- K. Kuroda, G. A. Caputo and W. F. DeGrado, Chem. – Eur. J., 2009, 15, 1123 CrossRef CAS PubMed
.
- J. Guo, J. Qin, H. Mao, B. Wang and F. Yan, ACS Appl. Mater. Interfaces, 2016, 8, 12684 CrossRef PubMed
.
-
A. Parthiban, Synthesis and Applications of copolymers, Wiley, Hoboken, NJ, USA, 2014 Search PubMed
.
- Z. M. Al-Badri, A. Som, S. Lyon, C. F. Nelson, K. Nüsslein and G. N. Tew, Biomacromolecules, 2008, 9, 2805 CrossRef CAS PubMed
.
- K. Lienkamp, A. E. Madkour, K.-N. Kumar, K. Nüsslein and G. N. Tew, Chem. – Eur. J., 2009, 15, 11715 CrossRef CAS PubMed
.
- Y. Qiao, C. Yang, D. J. Coady, Z. Y. Ong, J. L. Hedrick and Y.-Y. Yang, Biomaterials, 2012, 33, 1146 CrossRef CAS PubMed
.
- A. C. Engler, A. Shukla, S. Puranam, H. G. Buss, N. Jreige and P. T. Hammond, Biomacromolecules, 2011, 12, 1666 CrossRef CAS PubMed
.
- E. F. Palermo and K. Kuroda, Biomacromolecules, 2009, 10, 141 Search PubMed
.
- K. Kuroda and W. F. DeGrado, J. Am. Chem. Soc., 2005, 127, 4128 CrossRef CAS PubMed
.
- L. Mi and S. Y. Jiang, Angew. Chem., Int. Ed., 2014, 53, 1746 CrossRef CAS PubMed
.
- Y. Zheng, Y. Luo, K. Feng, W. Zhang and G. Chen, ACS Macro Lett., 2019, 8, 326 CrossRef CAS PubMed
.
- J. Wang, D. Wang, Y. Zhang and J. Dong, ACS Biomater. Sci. Eng., 2021, 7, 963 CrossRef CAS PubMed
.
- E. H. H. Wong, M. M. Khin, V. Ravikumar, Z. Y. Si, S. A. Rice and M. B. Chan-Park, Biomacromolecules, 2016, 17, 1170 CrossRef CAS PubMed
.
- W. Rusli, S. W. B. Tan, A. Parthiban and A. M. van Herk, Polymer, 2022, 247, 124774 CrossRef CAS
.
- J. S. Koo, N.-S. Kwak and T. S. Hwang, J. Membr. Sci., 2012, 423–424, 293 CAS
.
- R. E. Coppola, D. Herranz, R. E.-Cid, N. Ming, N. B. D’Accorso, P. Ocón and G. C. Abuin, Renew. Energy, 2020, 157, 71 CrossRef CAS
.
- Y.-C. Cao, C. Xu, L. Zou, K. Scott and J. Liu, J. Power Sources, 2015, 294, 691 CrossRef CAS
.
- K. F. Handle, R. Bakrya and G. K. Bonn, J. Chromatogr. A, 2023, 1700, 464049 CrossRef CAS PubMed
.
- N. Kesharwani and C. Haldar, Polyhedron, 2022, 219, 115787 CrossRef CAS
.
- D. Das, A. Sivaramakrishna, C. V. S. B. Rao, N. Sivaraman and K. Vijayakrishna, Polym. Int., 2018, 67, 374 CrossRef CAS
.
- D. Pauli and S. Bienz, Tetrahedron, 2014, 70, 1348 CrossRef CAS
.
- W. Shen, L.-M. Wang and H. Tian, J. Fluor. Chem., 2008, 129, 267 CrossRef CAS
.
- J. M. J. Frechet, M. D. Smet and M. J. Farrall, J. Org. Chem., 1979, 44, 1774 CrossRef CAS
.
- C. Racles, E. Avram, M. Marcu, V. Cozan and M. Cazacu, Polymer, 2000, 41, 8205 CrossRef CAS
.
- M. C. P.-Blanco, A. P.-Franco, S. M.-Lorenzo and M. J. G.-Castro, Talanta, 2023, 255, 124228 CrossRef PubMed
.
- N. S. Abdelwahab, N. F. Farid, M. Elagawany and E. H. Abdelmomen, Biomed. Chromatogr., 2018, 32, e4346 CrossRef PubMed
.
- B. Lou, N. Beztsinna, G. Mountrichas, J. B. V. D. Dikkenberg, S. Pispas and W. E. Hennink, Int. J. Pharmaceut., 2017, 525, 388 CrossRef CAS PubMed
.
- D. Druvari, N. D. Koromilas, G. C. Lainioti, G. Bokias, G. Vasilopoulos, A. Vantarakis, I. Baras, N. Dourala and J. K. Kallitsis, ACS Appl. Mater. Interfaces, 2016, 8, 35593 CrossRef CAS PubMed
.
- N. D. Koromilas, G. Ch Lainioti, G. Vasilopoulos, A. Vantarakis and J. K. Kallitsis, Polym. Chem., 2016, 7, 3562 RSC
.
- J. M. Boyce, Antimicrobial Resistance & Infection Control, 2023, 12, 32 Search PubMed
.
- R. F. Dsouza and A. Parthiban, Langmuir, 2019, 35, 13942 CrossRef CAS PubMed
.
- T. Cheikhalard, V. Massardier, L. Tighzert and J. P. Pascault, J. Appl. Polym. Sci., 1998, 70, 613 CrossRef CAS
.
-
M. A. Wikler, et al. Methods for dilution antimicrobial susceptibilty tests for bacteria that grow aerobically; Approved standard-Ninth Edition CLSI document M07-A9 2012, 29(2).
Footnotes |
† Electronic supplementary information (ESI) available: GPC analysis and 1H-NMR spectra of polymers. See DOI: https://doi.org/10.1039/d3ma00661a |
‡ These authors have contributed equally to this manuscript. |
|
This journal is © The Royal Society of Chemistry 2023 |
Click here to see how this site uses Cookies. View our privacy policy here.