DOI:
10.1039/D3NA00210A
(Paper)
Nanoscale Adv., 2023,
5, 3985-3993
A polyoxometalate-based heterojunction nanozyme with peroxidase-mimic catalytic activity for sensitive biomolecule detection
Received
2nd April 2023
, Accepted 15th June 2023
First published on 13th July 2023
Abstract
Nanozymes are a class of nanomaterials that can specifically mimic the structures and catalytic activities as well as overcome limitations of natural enzymes and have hence been considered as a competitive alternative to natural enzymes. At present, plenty of nanozymes, especially those with peroxidase (POD)-like catalytic activity, have been extensively explored for biosensing. In this work, we proposed polyoxometalate-based heterojunction GdP5W30O110@WS2 nanoclusters (NCs) to exert intrinsic POD-like catalytic activity even under harsh catalytic conditions. Detailedly, GdP5W30O110@WS2 NCs possessing conducive POD-like catalytic activity can oxidize chromogenic substrates into colored substances in the presence of H2O2. On the strength of the POD-like catalytic activity of GdP5W30O110@WS2 NCs, a reliable analytical platform is then constructed after the optimization of catalytic conditions for the detection of H2O2, glutathione (GSH) and glucose via a simple TMB colorimetric strategy. This work advances the utilization of versatile polyoxometalate-based nanomaterials for biosensing, dramatically broadening the potential applications of other nanozyme-based biosensors.
Introduction
Biomolecules play crucial roles in diverse physiological and pathological processes, and their levels in the human body can serve as important indicators for monitoring physical status.1,2 Therefore, highly selective and sensitive quantitative detection of biomolecules not only helps to reveal their functions in various physiological processes but also is a prerequisite and basis for monitoring the physical status of the human body.3,4 Of note, benefiting from the specificity of active enzymes to substrates, enzymatic methods can achieve rapid and accurate biomolecule detection, which is one of the most mature and commonly applied typical strategies.5,6 Natural enzymes are a class of proteins possessing high substrate specificity and efficacious catalytic activity and are implicated in almost all biocatalytic reactions in organisms.7,8 However, their practical applications are severely limited by intrinsic shortcomings such as high cost, susceptibility to environmental factors, and difficult purification.9,10 To overcome these drawbacks, it is of great importance to develop novel artificial enzyme mimics that have remarkable enzyme-like activities by facile synthesis. Since the first demonstration of the peroxidase (POD)-like property of Fe3O4 nanoparticles by Gao in 2007, many nanomaterials with multiple enzymatic catalytic activities, namely nanozymes, have been considered as strong substitutes for nature enzymes and have been widely used in biological, medical and industrial catalysis fields.11 So far, a surge of artificial nanozymes with POD-like catalytic activity, such as precious metals, metal oxides, transition metal dichalcogenides (TMDCs), carbon-based nanomaterials, single-atom nanozymes and metal–organic frameworks (MOFs), have been extensively utilized for biosensing.12–18 However, their enzymatic catalytic efficiency is still far less than that of natural enzymes. Thus, investigations on nanozymes with efficient catalytic activity, minimal cytotoxicity and high affinity to substrates are still imperative and pivotal.
In recent years, emerging kinds of metal-based nanomaterials with shifting or mixed redox states have been found to exert intrinsic POD-like catalytic activity.19,20 However, the insufficient enzymatic performance of these nanomaterials is inadequate for their applications in the fields of biosensing. Since most of the reactions take place on the surface of nanozymes, we speculate that surface modification or increasing the specific surface area of nanozymes enables higher catalytic activity for a more sensitive enzymatic reaction.21 Interestingly, constructing a heterojunction structure can also further enhance the enzymatic activity of these nanozymes via accelerating the electron transfer on the heterogeneous interface and reducing the required energy for the binding between nanozymes and H2O2.22–24 Based on the aforementioned findings, we assume that polyoxometalate-based heterojunctions can act as prospective POD-like nanozymes for biosensing.
Herein, polyoxometalate-based heterostructure GdP5W30O110@WS2 NCs were constructed (Scheme 1A). Benefiting from the specific heterostructure and high specific surface area inherited from WS2 nanosheets, the prepared GdP5W30O110@WS2 exhibited a remarkable POD-like catalytic performance and superior tolerance to stringent conditions. Furthermore, GdP5W30O110@WS2 was implicated in a facile colorimetric platform to realize the quantitative detection of H2O2, glutathione (GSH) and glucose. The excellent analytical performance manifested that our work opens up broad prospects for the elaborate design and development of polyoxometalate-based nanozymes and dramatically expands the potential application of nanozyme-based biosensors.
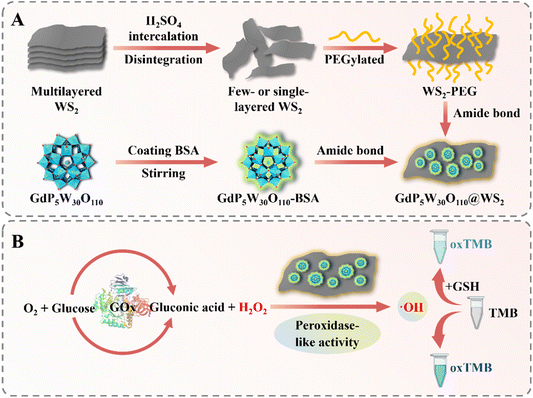 |
| Scheme 1 (A) Schematic illustration of the general synthetic procedures of the GdP5W30O110@WS2 nanozymes; (B) Mechanism description of GdP5W30O110@WS2 nanozymes with peroxidase-like activity for the colorimetric detection of biomolecules, including H2O2, GSH and glucose. | |
Experimental section
Chemicals
Sodium tungstate dihydrate (Na2WO4·2H2O), bovine serum albumin (BSA), 1-(3-dimethylaminopropyl)-3-ethylcarbodiimide (EDC) and N-hydroxysuccinimide (NHS) were obtained from Aladdin Industrial Corporation (Shanghai, China). Gadolinium(III) chloride hexahydrate (GdCl3·6H2O) and tungsten sulfide (WS2, 99.8%) were purchased from Alfa Aesar Reagent Company (Shanghai, China). Sodium acetate (NaAc), acetic acid (HAc), citric acid monohydrate, disodium hydrogen phosphate anhydrous (Na2HPO4), methanol (CH3OH, 99.5%) and sulfuric acid (H2SO4, 95–98%) were bought from Chron Chemicals Co., Ltd (Shanghai, China). Polyethylene glycol 400 (PEG-400) was supplied by Kermel (Tianjin, China). Ethanol, hydrogen peroxide (H2O2, 30%) and phosphoric acid (H3PO4, 85%) were purchased from Jinshan Chemical Reagent Co., Ltd (Chengdu, China). Potassium acetate (CH3COOK) and potassium chloride (KCl) were from Tianjin Zhiyuan Chemical Reagent Co., Ltd (Tianjin, China). 3,3′,5,5′-Tetramethylbenzidine (TMB) was obtained from Adamas Reagent Co., Ltd. Acetic acid buffer (HAc–NaAc, 0.2 M) and Na2HPO4–citric acid buffer (0.2 M) solutions were prepared in the laboratory. All reagents and solvents were of commercially analytical grade and used as received without any previous purification.
Preparation of GdP5W30O110 nanoclusters
The GdP5W30O110 nanoclusters were synthesized by a two-step hydrothermal approach according to a previously reported procedure with an adjusted dose.25 In a typical synthetic procedure, 16.5 g Na2WO4·2H2O was first completely dissolved in 15 mL ultrapure water with ultrasound assistance and then 85% H3PO4 (13.25 mL) was slowly added into the solution. Afterwards, the above mixture was placed into a sample preparation bomb and was heated up to 120 °C for 12 h. After the bomb was spontaneously cooled down to room temperature, ultrapure water (7.5 mL) and KCl (5 g) were added into the reaction system and some white precipitate was immediately formed. Then, the precipitate was filtered and washed with potassium acetate solution (2 M) and methanol. When the precipitate naturally dried, it was dissolved in 15 mL hot ultrapure water (around 65 °C) and the intermediate product Kl2.5Nal.5[NaP5W30O110]·15H2O was subsequently formed by cooling and recrystallization.
Then, Kl2.5Nal.5[NaP5W30O110]·15H2O (1 g) was dissolved in 12 mL ultrapure water and heated to 65 °C. Afterwards, GdCl3·6H2O aqueous solution (0.08 mmol mL−1, 3 mL) was dropwise added into the above solution under heating with magnetic stirring. After reaction, the mixture was poured into a sample preparation bomb and heated at 160 °C for 12 h. After the bomb was spontaneously cooled down to room temperature, KCl (4 g) was introduced into the reaction system and the precipitate was immediately formed. Then, the precipitate was filtered and washed with potassium acetate solution (2 M) and methanol. Finally, the as-synthesized GdP5W30O110 nanoclusters were air-dried and ready for use.
Surface functionalization of GdP5W30O110 nanoclusters with BSA
In brief, 400 mg BSA and 0.118 g GdP5W30O110 nanoclusters were dispersed into 20 mL ultrapure water and the mixture was stirred for 6 h. The carboxy-functionalized GdP5W30O110 nanoclusters (GdP5W30O110-BSA) were kept at room temperature for later use.
Preparation of WS2 nanosheets
The WS2 nanosheets were synthesized by ultrasound-assisted liquid exfoliation using H2SO4 as an intercalation agent by referring to a previous report.26 First, commercial WS2 powder was ground in a ball miller for 6 h. Next, the preconditioned WS2 (60 mg) was dispersed into 60 mL concentrated sulfuric acid (H2SO4, 95–98%) and the reaction mixture was kept at 90 °C in an oil bath for 24 h under stirring. Afterwards, the H2SO4-intercalated WS2 was centrifugally collected at 6000 rpm (10 min) and washed with ultrapure water to remove supernatant H2SO4, repeating four times. Then, the purified H2SO4-intercalated WS2 was re-dispersed into ultrapure water (about 30 mL) and was ultrasonically peeled off in an ice-cold water bath for 2 h. Finally, the layered WS2 nanosheets were harvested by centrifugation (12
000 rpm, 20 min).
Surface functionalization of WS2 nanosheets with PEG-400
To synthesize amino-functionalized WS2 nanosheets, PEG-400 ethanol solution (50 mg mL−1, 1 mL) was added into layered WS2 solution (1 mg mL−1, 10 mL), and the mixture was stirred for 12 h at room temperature. Then, the WS2-PEG was obtained by centrifugation (6000 rpm, 10 min) and washed with ultrapure water to remove residual PEG-400.
Preparation of GdP5W30O110@WS2
In a typical preparation process, 5 mg 1-(3-dimethylaminopropyl)-3-ethylcarbodiimide (EDC) was first introduced into WS2-PEG aqueous solution (1 mg mL−1, 10 mL) and some white floccules formed immediately. Then, 5 mg N-hydroxysuccinimide (NHS) was added and the mixture was set under magnetic stirring until the white floccules disappeared. Afterwards, GdP5W30O110-BSA (2 mL) was added into the above solution, followed by stirring at room temperature for 12 h. Finally, the as-prepared GdP5W30O110@WS2 was collected by centrifugation (6000 rpm, 10 min) and washed with ultrapure water.
Peroxidase-like catalytic activity of GdP5W30O110@WS2
Peroxidase-like catalytic activity assay.
The representative colorimetric analysis assay using 3,3′,5,5′-tetramethylbenzidine (TMB), 2,2′-azino-bis(3-ethylbenzothiazoline-6-sulfonic acid) (ABTS), and o-phenylenediamine (OPD) as peroxidase colorimetric substrates in the presence of H2O2 was performed to preliminarily evaluate the peroxidase-like catalytic activity of GdP5W30O110@WS2. The hydroxyl radical (˙OH) that was generated by a Fenton-like reaction between H2O2 and W5+ of GdP5W30O110@WS2 can oxidize colorimetric substrates into colored substances with their respective characteristic peaks. Therefore, the peroxidase-like catalytic activity of GdP5W30O110@WS2 can be readily determined via monitoring the absorption intensity at characteristic peaks of the reaction system. In general, GdP5W30O110@WS2 (final concentration 100 μg mL−1) and colorimetric substrates (TMB, ABTS or OPD, final concentration 0.5 mM) were first added into Na2HPO4–citric acid buffer (0.2 M). Subsequently, H2O2 (final concentration 50 mM) was introduced into the reacting system to launch the chromogenic reaction. After 30 min incubation, the supernatant was collected by centrifugation (6000 rpm, 10 min) and the absorbance spectrum was recorded with UV-vis spectroscopy (UV-6100, MAPADA).
H2O2 and GSH detection by GdP5W30O110@WS2
Briefly, GdP5W30O110@WS2 (final concentration 100 μg mL−1), TMB ethanol solution (final concentration 0.5 mM) as well as varied concentrations of H2O2 (0–50 mM) or different concentrations of GSH (0–25 μM) with 50 mM H2O2 were mixed into Hac–NaAc buffer (0.2 M, pH 3.0) and the solution was incubated for 30 min at 50 °C in the dark. Finally, the UV-vis spectrum was recorded by UV-vis spectroscopy.
Glucose detection by GdP5W30O110@WS2
In detail, GOx (final concentration 1 mg mL−1) and various concentrations of glucose aqueous solution (0–1.0 mM) were first mixed together and incubated at 37 °C for 1 h. Afterwards, GdP5W30O110@WS2 (final concentration 100 μg mL−1) and TMB ethanol solution (final concentration 0.5 mM) were then introduced and the UV-vis spectrum was recorded after 30 min reaction at 50 °C.
Results and discussion
Preparation and characterization of GdP5W30O110@WS2
The concise fabrication process of the heterostructure GdP5W30O110@WS2 NCs is illustrated in Scheme 1A. Here, the easy-to-obtain heterostructure GdP5W30O110@WS2 NCs were synthesized according to the previously reported strategies by a facile amidation reaction activated by 1-(3-dimethylaminopropyl)-3-ethylcarbodiimide (EDC)/N-hydroxysuccinimide (NHS) (Scheme 1A).27 As visualized by the transmission electron microscopy (TEM) image and the average hydrodynamic diameter recorded by dynamic light scattering (DLS) measurement, the as-synthesized GdP5W30O110@WS2 NCs showed a uniform and well-dispersed nanostructure and the size of GdP5W30O110@WS2 NCs was approximately 60 nm (Fig. 1A and B). Additionally, as presented in Fig. 1C, the changes of zeta potential for the samples could verify the successful surface loading of GdP5W30O110 nanoclusters onto WS2 nanosheets.27 Furthermore, the Fourier transform infrared (FTIR) spectra and Raman spectra of the different components also affirmed that the as-prepared GdP5W30O110@WS2 NCs had specific absorption peaks corresponding to GdP5W30O110 nanoclusters and WS2 nanosheets (Fig. 1D and E), which is consistent with previously reported work. The strong characteristic peaks at 1164, 1170, 917 and 780 cm−1 in GdP5W30O110 nanoclusters are assigned to the stretching vibration of the P–O band, W–O asymmetric vibration and W–O–W asymmetric vibration, respectively. In addition, the chemical composition of GdP5W30O110@WS2 NCs was further verified by UV-vis absorption spectra, indicating the successful fabrication (Fig. 1F). Taken together, the heterojunction GdP5W30O110@WS2 NCs were successfully fabricated as designed and have the potential for subsequent application in sensitive biomolecule detection.
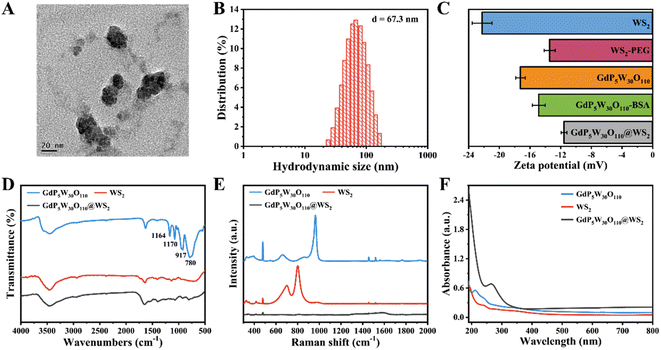 |
| Fig. 1 Preparation and characterization of GdP5W30O110@WS2 nanoclusters. (A) TEM image of GdP5W30O110@WS2 nanoclusters. (B) Hydrodynamic size distribution of GdP5W30O110@WS2 nanoclusters measured by dynamic light scattering (DLS). (C) Zeta potential of GdP5W30O110@WS2 and its components. (D) FT-IR spectra and (E) Raman spectra of GdP5W30O110, WS2 and GdP5W30O110@WS2 nanoclusters. (F) UV-vis-NIR adsorption spectra of GdP5W30O110, WS2 and GdP5W30O110@WS2 aqueous solutions (100 μg mL−1). | |
Peroxidase-like catalytic activity of GdP5W30O110@WS2
Peroxidases are a class of enzymes that can catalyze the production of reactive oxygen species (ROS) by applying peroxides (e.g., H2O2) as substrates, and have been widely used in biological catalysis, biosensing, disease treatment and regulating physiological activities.28,29 Until now, a large number of nanomaterials have been shown to possess intrinsic POD-like catalytic activity, the most representative one of which is metal-based nanomaterials with mixed redox states. Inspired by the recent advances, the heterojunction GdP5W30O110@WS2 NCs with mixed redox states thus exhibit great potential to exert considerable peroxidase-like catalytic activity. We next investigated the POD-like catalytic activity of GdP5W30O110@WS2 NCs in the presence of H2O2 through the oxidation of a series of colorimetric substrates (e.g., 3,3′,5,5′-tetramethylbenzidine (TMB), 2,2′-azino-bis(3-ethylbenzothiazoline-6-sulfonic acid) (ABTS), and o-phenylenediamine (OPD)). As shown in Fig. 2A, due to the intrinsic peroxidase-like catalytic activity of GdP5W30O110@WS2 NCs, H2O2 could be catalyzed into hydroxyl radicals (˙OH) and the reaction solution turned into the corresponding color of the oxidized colorimetric substrates (blue for oxTMB, green for oxABTS, yellow for oxOPD). As indicated in Fig. 2B, no significant characteristic absorbance and color change of TMB alone or TMB + GdP5W30O110@WS2 could be found. Meanwhile, the distinct characteristic absorption peaks were observed in the mixture of GdP5W30O110@WS2 NCs, H2O2 and TMB (green curve), reflecting the efficacious peroxidase-like catalytic activity. Analogously, the maximum absorption peaks of oxABTS and oxOPD in the ABTS/OPD + H2O2 + GdP5W30O110@WS2 group also implied that GdP5W30O110@WS2 NCs exhibited a superior peroxidase-like catalytic performance. Consequently, the prominent peroxidase-like catalytic activity of GdP5W30O110@WS2 NCs ensures their further controllable utilization for further application.
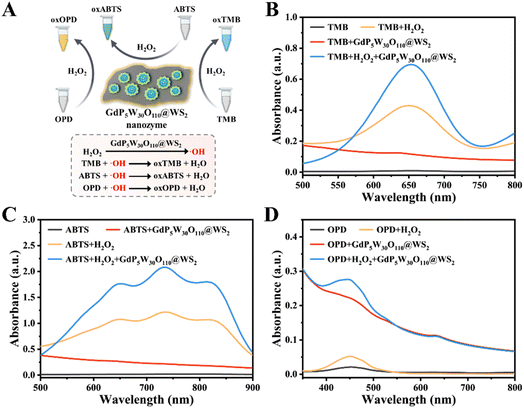 |
| Fig. 2 (A) Schematic illustration of the mechanism for the peroxidase-like activity evaluation of GdP5W30O110@WS2 applying TMB, OPD and ABTS as substrates. UV-vis-NIR adsorption spectra of the catalyzed oxidation of (B) TMB, (C) ABTS and (D) OPD after 15 min of incubation with PBS, GdP5W30O110@WS2, H2O2 and H2O2 + GdP5W30O110@WS2 at pH 4.0. | |
Similar to natural enzymes, the catalytic activity of nanozymes is also largely influenced by catalytic conditions, such as concentration, pH and temperature.30 In order to enable GdP5W30O110@WS2 to realize a maximal catalytic performance, we subsequently explored the optimal catalytic conditions in the classical TMB colorimetric system. As indicated in Fig. 3A and E, along with the increasing concentration of GdP5W30O110@WS2, the characteristic absorbance of oxTMB at 652 nm gradually increased, which is mainly attributed to the concentration-dependent peroxidase-like catalytic performance of GdP5W30O110@WS2. Meanwhile, GdP5W30O110@WS2 exhibited a stable peroxidase-like catalytic activity under acidic experimental conditions (pH at 3.0) and higher temperature than most natural enzymes (Fig. 3B, C, F and G). Besides, as shown in Fig. 3D and H, maximum catalytic activity was reached with 0.5 mM TMB.
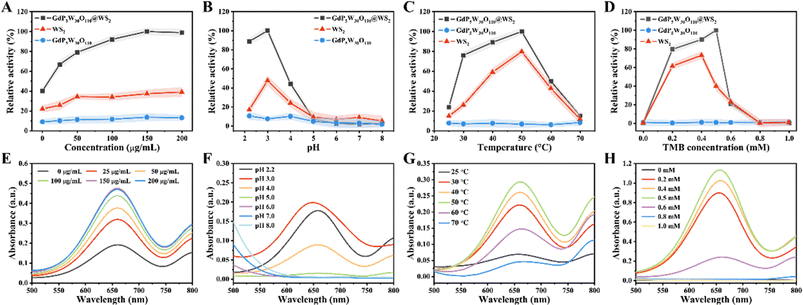 |
| Fig. 3 The optimization of (A) concentration, (B) pH, (C) temperature and (D) TMB concentration for the peroxidase-like activity of GdP5W30O110@WS2, GdP5W30O110 and WS2 calculated from the corresponding absorbance values (E–H). The maximum point in every curve was set as 100%. Error bars represent the standard error derived from three independent measurements. | |
Biomolecule detection by GdP5W30O110@WS2
Inspired by the outstanding peroxidase-like catalytic activity of GdP5W30O110@WS2, a GdP5W30O110@WS2-based analytical platform was established for biomolecule detection, including H2O2, GSH and glucose detection.
During the peroxidase-like catalytic process of GdP5W30O110@WS2, the absorbance intensity of oxidized substrates could vary according to the experimental conditions, thereby offering reliable evidence for quantifying biomolecules related to H2O2. In detail, ˙OH was generated under the POD-like catalytic action of GdP5W30O110@WS2 in the presence of H2O2. Subsequently, the formed ˙OH would catalyze the oxidation of TMB into oxTMB, which can be easily quantified by monitoring the characteristic absorbance intensity at 652 nm. Conceivably, a higher level of H2O2 would lead to higher absorbance intensity (Fig. 4A). For H2O2 detection, the characteristic absorbance intensity at 652 nm gradually increased with the increasing addition of H2O2 on a low concentration scale. As demonstrated in Fig. 4B and C, H2O2 concentration was linearly positively related to the absorbance intensity within the range from 0 to 50 μM.
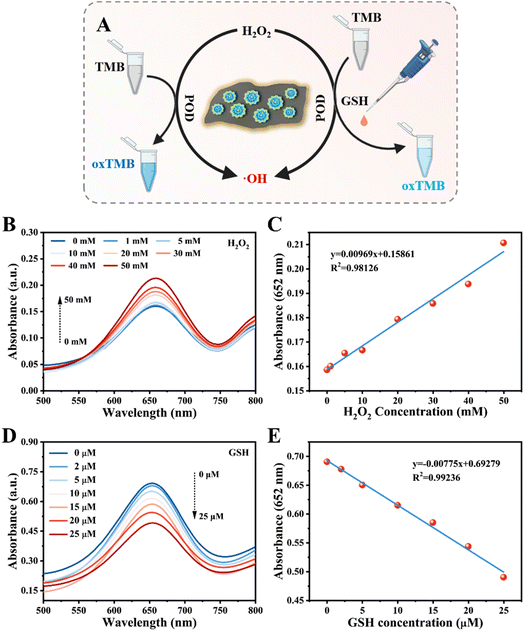 |
| Fig. 4 GdP5W30O110@WS2 nanoclusters-based sensing platform for H2O2 and GSH detection. (A) Mechanism description of GdP5W30O110@WS2 nanozymes with peroxidase-like activity for H2O2 and GSH detection. (B) UV-vis-NIR adsorption spectra of GdP5W30O110@WS2 (100 μg mL−1) incubated with TMB (0.5 mM) in the presence of different concentration of H2O2 at pH 4.0. (C) The linear calibration plot for H2O2 detection. (D) UV-vis-NIR adsorption spectra of GdP5W30O110@WS2 (100 μg mL−1) incubated with TMB (0.5 mM) and H2O2 (50 mM) in the presence of different concentrations of GSH at pH 4.0. (E) The linear calibration plot for GSH detection. | |
As one of the most representative endogenous antioxidants in cells, GSH plays a vital part in modulating the balance between free radicals and the antioxidant defense system.31–34 The disorders of GSH homeostasis are closely associated with such diseases as cancer, aging, neurodegenerative diseases and inflammations.33 Therefore, investigations on convenient and rational methods for GSH detection have aroused widespread concern in biochemical analysis and clinical diagnosis. As a radical scavenger, GSH can quench ˙OH released by the POD-like catalytic reaction of GdP5W30O110@WS2 with H2O2, giving rise to inhibition of the TMB oxidation process. As shown in Fig. 4A, the generation of ˙OH from H2O2 catalyzed by GdP5W30O110@WS2 could gradually oxidize the substrate TMB into oxTMB. After the introduction of GSH, the released ˙OH was depleted and weaker absorbance intensity at 652 nm could be detected. According to the considerable scavenging effect of GSH on ˙OH, a reliable analytical platform was then constructed for GSH detection. As shown in Fig. 4D, with the increasing concentration of GSH, the absorbance signal at 652 nm responded with a descending trend. Meanwhile, there was a satisfactory linear correlation between the absorbance intensity and GSH concentration ranging from 0 to 25 μM.
Glucose is the impetus of cell metabolism and is a universally available energy source for ATP production.33 In the presence of glucose oxidase (GOx), glucose can be effectively translated into gluconic acid and H2O2. In this regard, assisted by GOx-triggered oxidation, the generated H2O2 can act as a mediate substrate for glucose detection. After linear fitting, it was found that the GdP5W30O110@WS2-based analytical platform was able to detect glucose in a range from 0.1 to 1 mM (Fig. 5).
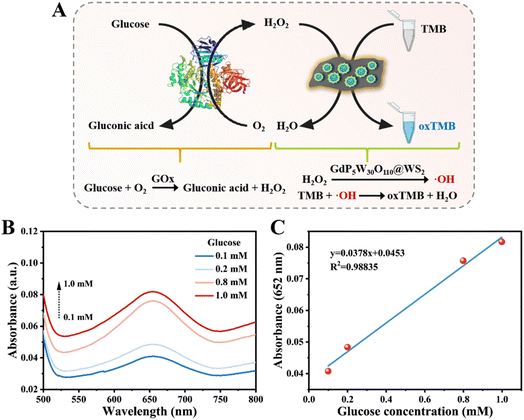 |
| Fig. 5 GdP5W30O110@WS2 nanoclusters-based sensing platform for glucose detection. (A) Mechanism description of GdP5W30O110@WS2 nanozymes with peroxidase-like activity for glucose detection. (B) UV-vis-NIR adsorption spectra of GdP5W30O110@WS2 (100 μg mL−1) incubated with TMB (0.5 mM) and GOx (1 mg mL−1) in the presence of different concentrations of glucose at pH 4.0. (C) The linear calibration plot for glucose detection. | |
These content results indicated the potential application value of the proposed GdP5W30O110@WS2-based analytical platform for biomolecule detection.
Conclusion
In conclusion, heterojunction GdP5W30O110@WS2 possessing intrinsic POD-like activity was prepared by a simple approach. As proved by our primary experiments, the proposed heterojunction GdP5W30O110@WS2 can generally oxidize several colorimetric substrates such as TMB, ABTS, and OPD in the presence of H2O2. Meanwhile, assisted by the excellent POD-like catalytic activity, the fabricated GdP5W30O110@WS2 was introduced as a probe for the sensitive detection of H2O2, GSH and glucose. All of the above favorable results highlighted that this work provided more possibilities for the construction of multifunctional polyoxometalate-based nanomaterials for biosensing, greatly expanding the potential value of other nanozyme-based biosensors.
Author contributions
Guobo Du and Mingzhu Lv contributed equally to this work. Yuan Yong and Guobo Du conceived and supervised this project. Mingzhu Lv, Chenghui Liu, Jiajie Liu, Zhu Yang, Huan Wang and Qiqi Xu performed the experiments and analysed the data. Yuan Yong, Guobo Du and Mingzhu Lv revised the paper. All authors have reviewed and approved the final version of this manuscript.
Conflicts of interest
The authors declare no conflict of interest.
Acknowledgements
This work was financially supported by the National Natural Science Foundation of China (31901005 and 52273304), the Natural Science Foundation of Sichuan Province (2022NSFSC0796), the Southwest Minzu University Talent Supporting Funds (RQD2021008), Scientific research and development funds of Affiliated Hospital of North Sichuan Medical College (2021ZD013), Nanchong City-School Cooperative Scientific Research Funds (20SXZRKX0006), Young Elite Scientists Sponsorship Program by CAST (YESS) (2022-2024QNRC001), the Open Project of Central Nervous System Drug Key Laboratory of Sichuan Province (230011–01SZ) and the Fundamental Research Funds for the Central Universities, Southwest Minzu University (2021NYYXS30).
References
- G. Maduraiveeran, M. Sasidharan and V. Ganesan, Electrochemical sensor and biosensor platforms based on advanced nanomaterials for biological and biomedical applications, Biosens. Bioelectron., 2018, 103, 113–129 CrossRef CAS PubMed.
- S. K. Krishnan, E. Singh, P. Singh, M. Meyyappan and H. S. Nalwa, A review on graphene-based nanocomposites for electrochemical and fluorescent biosensors, RSC Adv., 2019, 9, 8778–8881 RSC.
- W. Q. Xu, L. Jiao, Y. Wu, L. Y. Hu, W. L. Gu and C. Z. Zhu, Metal-Organic Frameworks Enhance Biomimetic Cascade Catalysis for Biosensing, Adv. Mater., 2021, 33, 2005172 CrossRef CAS PubMed.
- C. Song, W. Ding, W. W. Zhao, H. B. Liu, J. Wang, Y. W. Yao and C. Yao, High peroxidase-like activity realized by facile synthesis of FeS2 nanoparticles for sensitive colorimetric detection of H2O2 and glutathione, Biosens. Bioelectron., 2020, 151, 111983 CrossRef CAS PubMed.
- B. F. Wang, Y. Y. Luo, L. Gao, B. Liu and G. T. Duan, High-performance field-effect transistor glucose biosensors based on bimetallic Ni/Cu metal-organic frameworks, Biosens. Bioelectron., 2021, 171, 112736 CrossRef CAS PubMed.
- Z. H. Zhao, Y. J. Huang, W. R. Liu, F. G. Ye and S. L. Zhao, Immobilized Glucose Oxidase on Boronic Acid-Functionalized Hierarchically Porous MOF as an Integrated Nanozyme for One-Step Glucose Detection, ACS Sustainable Chem. Eng., 2020, 8, 4481–4488 CrossRef CAS.
- Y. Chong, Q. Liu and C. C. Ge, Advances in oxidase-mimicking nanozymes: Classification, activity regulation and biomedical applications, Nano Today, 2021, 37, 101076 CrossRef CAS.
- Z. R. Wang, R. F. Zhang, X. Y. Yan and K. L. Fan, Structure and activity of nanozymes: Inspirations for de novo design of nanozymes, Mater. Today, 2020, 41, 81–119 CrossRef CAS.
- N. F. Zhu, C. B. Liu, R. Liu, X. H. Niu, D. H. Xiong, K. Wang, D. Q. Yin and Z. Zhang, Biomimic Nanozymes with Tunable Peroxidase-like Activity Based on the Confinement Effect of Metal-Organic Frameworks (MOFs) for Biosensing, Anal. Chem., 2022, 94, 4821–4830 CrossRef CAS PubMed.
- L. Huang, Y. Zhou, Y. X. Zhu, H. Y. Su, S. Z. Yang, L. Feng, L. Zhao, S. R. Liu and K. Qian, Dual-modal nanoplatform integrated with smartphone for hierarchical diabetic detection, Biosens. Bioelectron., 2022, 210, 114254 CrossRef CAS PubMed.
- L. Z. Gao, J. Zhuang, L. Nie, J. B. Zhang, Y. Zhang, N. Gu, T. H. Wang, J. Feng, D. L. Yang, S. Perrett and X. Y. Yan, Intrinsic peroxidase-like activity of ferromagnetic nanoparticles, Nat. Nanotechnol., 2007, 2, 577–583 CrossRef CAS PubMed.
- M. Wang, M. Y. Chang, Q. Chen, D. M. Wang, C. X. Li, Z. Y. Hou, J. Lin, D. Y. Jin and B. G. Xing, Au2Pt-PEG-Ce6 nanoformulation with dual nanozyme activities for synergistic chemodynamic therapy/phototherapy, Biomaterials, 2020, 252, 120093 CrossRef CAS PubMed.
- W. C. Hu, M. R. Younis, Y. Zhou, C. Wang and X. H. Xia, In Situ Fabrication of Ultrasmall Gold Nanoparticles/2D MOFs Hybrid as Nanozyme for Antibacterial Therapy, Small, 2020, 16, 2000553 CrossRef CAS PubMed.
- X. Q. Meng, D. D. Li, L. Chen, H. L. He, Q. Wang, C. Y. Hong, J. Y. He, X. F. Gao, Y. L. Yang, B. Jiang, G. H. Nie, X. Y. Yan, L. Z. Gao and K. L. Fan, High-Performance Self-Cascade Pyrite Nanozymes for Apoptosis-Ferroptosis Synergistic Tumor Therapy, ACS Nano, 2021, 15, 5735–5751 CrossRef CAS PubMed.
- F. Wei, X. Y. Cui, Z. Wang, C. C. Dong, J. D. Li and X. J. Han, Recoverable peroxidase-like Fe3O4@MoS2-Ag nanozyme with enhanced antibacterial ability, Chem. Eng. J., 2021, 408, 127240 CrossRef CAS PubMed.
- Z. W. Liu, X. Y. Zhao, B. R. Yu, N. N. Zhao, C. Zhang and F. J. Xu, Rough Carbon-Iron Oxide Nanohybrids for Near-Infrared-II Light-Responsive Synergistic Antibacterial Therapy, ACS Nano, 2021, 15, 7482–7490 CrossRef CAS PubMed.
- M. Y. Chang, Z. Y. Hou, M. Wang, C. Z. Yang, R. F. Wang, F. Li, D. L. Liu, T. L. Peng, C. X. Li and J. Lin, Single-Atom Pd Nanozyme for Ferroptosis-Boosted Mild-Temperature Photothermal Therapy, Angew. Chem., Int. Ed., 2021, 60, 12971–12979 CrossRef CAS PubMed.
- J. Kong, J. Zheng, Z. M. Li, J. B. Huang, F. H. Cao, Q. Zeng and F. Li, One-pot synthesis of AuAgPd trimetallic nanoparticles with peroxidase-like activity for colorimetric assays, Anal. Bioanal. Chem., 2021, 413, 5383–5393 CrossRef CAS PubMed.
- B. J. Ma, S. Wang, F. Liu, S. Zhang, J. Z. Duan, Z. Li, Y. Kong, Y. H. Sang, H. Liu, W. B. Bu and L. L. Li, Self-Assembled Copper Amino Acid Nanoparticles for in Situ Glutathione “AND” H2O2 Sequentially Triggered Chemodynamic Therapy, J. Am. Chem. Soc., 2019, 141, 849–857 CrossRef CAS PubMed.
- Z. L. Dong, L. Z. Feng, Y. Chao, Y. Hao, M. C. Chen, F. Gong, X. Han, R. Zhang, L. Cheng and Z. Liu, Amplification of Tumor Oxidative Stresses with Liposomal Fenton Catalyst and Glutathione Inhibitor for Enhanced Cancer Chemotherapy and Radiotherapy, Nano Lett., 2019, 19, 805–815 CrossRef CAS PubMed.
- L. W. Wang, B. Li, Z. You, A. Z. Wang, X. Y. Chen, G. J. Song, L. Yang, D. Chen, X. Yu, J. Liu and C. Y. Chen, Heterojunction of Vertically Arrayed MoS2 Nanosheet/N-Doped Reduced Graphene Oxide Enabling a Nanozyme for Sensitive Biomolecule Monitoring, Anal. Chem., 2021, 93, 11123–11132 CrossRef CAS PubMed.
- G. T. Wang, T. Wang, Y. Dang, Z. W. Lu, G. H. Su, B. Feng, Y. Zhuo, X. M. Jiang, Q. B. Ye, C. Wu, X. Pu, Y. Zhao, X. Q. Zhao, S. Cai, S. Y. Du, S. S. Jia, Y. Y. Wang, D. Wu, H. B. Rao and M. M. Sun, Insights into the antibacterial mechanism of MoS2/CoS2 heterostructure nanozymes with double enzyme-like activities for MRSA-infected wound therapy, Chem. Eng. J., 2023, 461, 141959 CrossRef CAS.
- L. W. Wang, Z. W. Yang, G. X. Song, Z. You, X. Y. Zhang, L. Liu, J. Zhang, L. H. Ding, N. Ren, A. Z. Wang, J. Liu, L. Liu and X. Yu, Construction of S-N-C bond for boosting bacteria-killing by synergistic effect of photocatalysis and nanozyme, Appl. Catal., B, 2023, 325, 122345 CrossRef CAS.
- L. W. Wang, F. N. Gao, A. Z. Wang, X. Y. Chen, H. Li, X. Zhang, H. Zheng, R. Ji, B. Li, X. Yu, J. Liu, Z. J. Gu, F. L. Chen and C. Y. Chen, Defect-Rich Adhesive Molybdenum Disulfide/rGO Vertical Heterostructures with Enhanced Nanozyme Activity for Smart Bacterial Killing Application, Adv. Mater., 2020, 32, 2005423 CrossRef CAS PubMed.
- S. Cardona-Serra, J. M. Clemente-Juan, E. Coronado, A. Gaita-Arino, A. Camon, M. Evangelisti, F. Luis, M. J. Martinez-Perez and J. Sese, Lanthanoid Single-Ion Magnets Based on Polyoxometalates with a 5-fold Symmetry: The Series [LnP5W30O110]12- (Ln3+ = Tb, Dy, Ho, Er, Tm, and Yb), J. Am. Chem. Soc., 2012, 134, 14982–14990 CrossRef CAS PubMed.
- Y. Yong, L. J. Zhou, Z. J. Gu, L. Yan, G. Tian, X. P. Zheng, X. D. Liu, X. Zhang, J. X. Shi, W. S. Cong, W. Y. Yin and Y. L. Zhao, WS2 nanosheet as a new photosensitizer carrier for combined photodynamic and photothermal therapy of cancer cells, Nanoscale, 2014, 6, 10394–10403 RSC.
- L. Y. Zong, H. X. Wu, H. Lin and Y. Chen, A polyoxometalate-functionalized two-dimensional titanium carbide composite MXene for effective cancer theranostics, Nano Res., 2018, 11, 4149–4168 CrossRef CAS.
- B. W. Yang, Y. Chen and J. L. Shi, Reactive Oxygen Species (ROS)-Based Nanomedicine, Chem. Rev., 2019, 119, 4881–4985 CrossRef CAS PubMed.
- M. Y. Chang, M. Wang, M. F. Wang, M. M. Shu, B. B. Ding, C. X. Li, M. L. Pang, S. Z. Cui, Z. Y. Hou and J. Lin, A Multifunctional Cascade Bioreactor Based on Hollow-Structured Cu2MoS4 for Synergetic Cancer Chemo-Dynamic Therapy/Starvation Therapy/Phototherapy/Immunotherapy with Remarkably Enhanced Efficacy, Adv. Mater., 2019, 31, 1905271 CrossRef CAS PubMed.
- Y. Y. Huang, J. S. Ren and X. G. Qu, Nanozymes: Classification, Catalytic Mechanisms, Activity Regulation, and Applications, Chem. Rev., 2019, 119, 4357–4412 CrossRef CAS PubMed.
- G. X. Yin, T. T. Niu, T. Yu, Y. B. Gan, X. Y. Sun, P. Yin, H. M. Chen, Y. Y. Zhang, H. T. Li and S. Z. Yao, Simultaneous Visualization of Endogenous Homocysteine, Cysteine, Glutathione, and their Transformation through Different Fluorescence Channels, Angew. Chem., Int. Ed., 2019, 58, 4557–4561 CrossRef CAS PubMed.
- L. S. Lin, J. B. Song, L. Song, K. M. Ke, Y. J. Liu, Z. J. Zhou, Z. Y. Shen, J. Li, Z. Yang, W. Tang, G. Niu, H. H. Yang and X. Y. Chen, Simultaneous Fenton-like Ion Delivery and Glutathione Depletion by MnO2-Based Nanoagent to Enhance Chemodynamic Therapy, Angew. Chem., Int. Ed., 2018, 57, 4902–4906 CrossRef CAS PubMed.
- N. Ballatori, S. M. Krance, S. Notenboom, S. J. Shi, K. Tieu and C. L. Hammond, Glutathione dysregulation and the etiology and progression of human diseases, Biol. Chem., 2009, 390, 191–214 CrossRef CAS PubMed.
- J. S. Huang, Y. C. Li, L. Zhang, J. Wang, Z. G. Xu, Y. J. Kang and P. Xue, A platinum nanourchin-based multi-enzymatic platform to disrupt mitochondrial function assisted by modulating the intracellular H2O2 homeostasis, Biomaterials, 2022, 286, 121572 CrossRef CAS PubMed.
Footnote |
† These authors contributed equally. |
|
This journal is © The Royal Society of Chemistry 2023 |
Click here to see how this site uses Cookies. View our privacy policy here.