Spirocyclization and Michael addition of 3-benzylidene succinimides: route to spirocyclopentapyrrolidine-tetraones and benzylidene N-arylpyrrolidine-diones†
Received
6th October 2023
, Accepted 31st October 2023
First published on 9th November 2023
Abstract
Reactions of 3-benzylidene succinimides with 2-substituted 2-hydroxy-indane-1,3-diones and unsaturated pyrazolones are carried out under basic conditions to afford spirocyclized derivatives and Michael adducts, respectively, with high regio- and stereo-selectivities. The most notable aspect of the reaction is the ability of highly reactive benzylidene succinimide to act as both an electrophile and a nucleophile causing spirocyclization. The reaction proceeded under mild and metal-free conditions and products were isolated in good to high yields. The current strategy utilizes simple and easily accessible precursors, and provides functionally rich products of medicinal interest with two to four contiguous stereogenic centres and complete regioselectivity with excellent diastereoselectivity.
Introduction
The synthesis of biologically active motifs is an important concern. Among these, cyclic β-diketones have tremendous synthetic utility; for example, 1,3-indanedione is a key component of many natural product structures like fredericamycin A, indacrinone (MK-196), and meroindenon (Fig. 1).1,2 In addition, it is a key component of marine alkaloids like ningalin and lamillarin.3 On the other hand, the alkylidene or arylidene indanedione system has enormous synthetic utility and is found in many biologically active compounds possessing anti-inflammatory, antibacterial, β-amyloid inhibitory and anticancer properties and that act as therapeutics for hypertension.4–6 Similarly, some compounds like indanostatin were found to have potential neuroprotective activity against glutamate toxicity,7 and fredericamycin8 acts as an anticancer agent. Likewise, the alkylidene succinimide moiety, introduced in many natural products, has great cytotoxic activity.9 These are also used as reactive functional components in many asymmetric synthetic protocols,10,11 and act as nucleophiles in enantioselective addition to several electrophiles from their allylic site.12,13 Furthermore, they undergo various cycloaddition reactions, important synthetic techniques for the production of spiro compounds having therapeutic values.14 Alkylidene succinimides are employed as efficient exocyclic enones to undergo [3 + 2] cycloaddition with various electrophiles resulting in the formation of spiro compounds.15,16 These are used as important synthons providing robust access to spiropyrrolidines and pyrones,17–19 and are also used for the preparation of new succinimide scaffolds.20 Similarly, nitrogen containing heterocycles were found to be incorporated in many natural products.21 For example, the pyrazoline ring, known as the master key in heterocyclic chemistry, is indispensable in endowing bioactive drugs with anticancer,22 neuroprotective,23 antidepressive,24 antibacterial,25 anticonvulsive,26 and antimalarial properties.27 Herein, we report the reactions of 3-ylidene-indane-1,3-diones with 3-benzylidene succinimides, resulting in the formation of spirocyclopentane compounds, which are potential intermediates in the synthesis of natural products and probable lead compounds in the development of new drugs.28–30 In addition, we also studied reactions between 3-benzylidene succinimides and alkylidene pyrazolones, which interestingly formed Michael adducts with functionally active units.
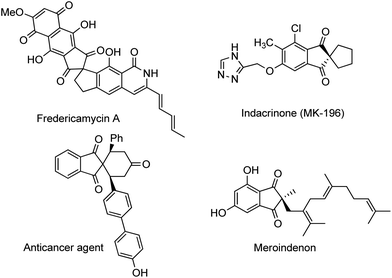 |
| Fig. 1 Indanedione-based structures of some biologically active natural products. | |
Results and discussion
We commenced our work by synthesizing precursors such as 2-substituted-2-hydroxy-indane-1,3-diones,31 benzylidene succinimides,32 and (E)-4-arylidene-5-methyl-2-phenyl-2,4-dihydro-3H-pyrazol-3-ones33 using literature procedures.
With the substrates 2-substituted-2-hydroxy-indane-1,3-dione 1a and benzylidene succinimide (2a) in hand, the reaction was initially set as a template to investigate the optimum reaction conditions. Firstly, preliminary investigation was done for selecting a suitable base. No reaction ensued when Et3N and pyridine were used as the base (Table 1, entries 1 and 2). Encouragingly, we observed the formation of product 3a, albeit in a low yield, with DIPEA and DABCO (15% and 20% in entries 3 and 4, respectively). Interestingly, the yield was further improved to 38% with DBU as a base (entry 5). Importantly, the efficiency of the reaction was nullified by the use of the Brønsted acid PTSA and Lewis acids BF3·OEt2 and I2 (entries 6–8). Next, we continued with DBU for reaction optimization to find out the best conditions.
Table 1 Optimization of reaction conditionsa
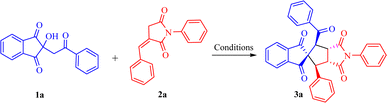
|
Entry |
Reagent |
Solvent |
Temp. |
Time (h) |
Yieldb (%) |
Reaction conditions: all reactions were carried out by using 0.25 mmol of each reactant and 0.25 mmol of reagent in 3 mL of solvent.
Isolated yield after column chromatography.
60 mol% of DBU was used.
30 mol% of DBU was used.
20 mol% of DBU was used.
|
1 |
Et3N |
DCE |
rt |
24 |
Nr |
2 |
Pyridine |
DCE |
rt |
24 |
Nr |
3 |
DIPEA |
DCE |
rt |
24 |
15 |
4 |
DABCO |
DCE |
rt |
24 |
20 |
5 |
DBU |
DCE |
rt |
24 |
38 |
6 |
PTSA |
DCE |
rt |
24 |
Nr |
7 |
BF3·OEt2 |
DCE |
rt |
24 |
Nr |
8 |
I2 |
DCE |
rt |
24 |
Nr |
9 |
DBU |
H2O |
rt |
24 |
Nr |
10 |
DBU |
MeOH |
rt |
24 |
Nr |
11 |
DBU |
EtOH |
rt |
24 |
Nr |
12 |
DBU |
1,4-Dioxane |
rt |
24 |
Nr |
13 |
DBU |
CHCl3 |
rt |
24 |
40 |
14 |
DBU |
ACN |
rt |
24 |
47 |
15 |
DBU |
ACN |
60 °C |
6 |
64 |
16 |
DBU |
ACN |
70 °C |
6 |
71 |
17 |
DBU |
ACN |
90 °C |
4 |
73 |
18c |
DBU |
ACN |
90 °C |
4 |
75 |
19d |
DBU |
ACN |
90 °C |
4 |
79 |
20e |
DBU |
ACN |
90 °C |
4 |
81 |
Among various solvents examined, such as water, methanol, ethanol and 1,4-dioxane, no conversion of the reactants was observed by TLC (entries 9–12). Presumably, polar protic solvents and acids might be reducing the efficiency of the base by causing its protonation, thereby making it inactive. Substituting with CHCl3 gave a similar yield to that of DCE (comparison of entries 5 and 13). However, the use of acetonitrile seemed fruitful, producing 47% 3a (entry 14). Fortified by the above results, we then explored the influence of temperature on the reaction outcome. In an effort to increase the reaction yield, the temperature of the reaction was raised further. The efficacy of the reaction was gradually increased from 60 °C to 90 °C (entries 15–17). Taking the above factors into consideration, base loading of the catalyst was also evaluated which revealed that the reaction with 20% DBU was more effective and produced product 3a in 81% yield (entry 20). The NMR spectrum of the crude sample of 3a revealed the formation of a single diastereomer.
To widen the scope of the reaction, we envisioned the substrates with various substituents. For this motive, we chose substrates 1a–1d, 2a–2c with substituents of diverse electronic features, such as electron-releasing groups (ERG; CH3 and OCH3) and electron-withdrawing groups (EWG; F and Br), on different positions of the arene moieties for this synthetic protocol (Scheme 1). The reactions of parent unsubstituted 1a and p-fluoro, p-methyl and p-bromo substituted indenones 1b–1d with unsubstituted (2a) and p-methoxy substituted (2b and 2c) 3-benzylidene succinimides afforded the corresponding products 3a–3j in 63–81% yields. While products 3a–3f and 3i were obtained in good yields (71–81%), the spirocyclized derivatives 3g, 3h and 3j were isolated in moderate yields of 63–67%. This may be attributed to the substituent effect which is well tolerated from 3a–3f and 3i, while in 3g, 3h and 3j, the electron-withdrawing inductive effect of bromine on the indanedione moiety reduces the rate of spirocyclization. Nevertheless, product yields were improved as observed in 3i and 3j where the methoxy group was introduced at the para position of both the phenyl rings i.e., the benzylidene phenyl group and phenyl ring attached to the nitrogen of the succinimide ring.
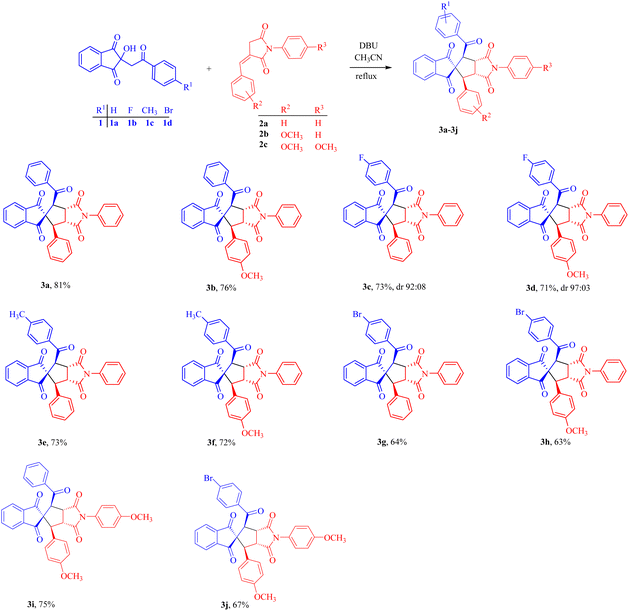 |
| Scheme 1 Reactions of indanediones with benzylidene succinimides leading to spirocyclopentane derivatives. | |
Encouraged by the broad substrate scope, the effect of the introduction of five-membered furyl and thienyl rings in place of the phenyl ring of the alkylidene succinimide moiety was also tested, which improved the efficiency of the reaction and the corresponding products 3k–3r were obtained in 73–82% yields (Scheme 2).
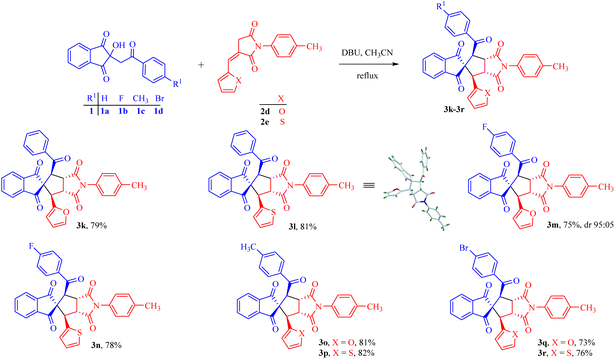 |
| Scheme 2 Reactions of indanediones with furylidene and thienylidene succinimides to access spirocyclopentane derivatives. | |
The structures of spiro compounds 3a–3r were established by rigorous NMR and HRMS spectral analysis. Furthermore, to gain a deeper understanding, the structure of the product was corroborated by 2D NMR studies such as COSY, NOESY, HMBC, and HSQC. From 2D experiments, the stereochemical correlations between Ha, Hb, Hc and Hd were established.
The heteronuclear single quantum correlation (HSQC) experiment of 3c revealed connectivity between various protons (Ha–Hd) with their corresponding carbons (Ca–Cd) (Fig. 2).
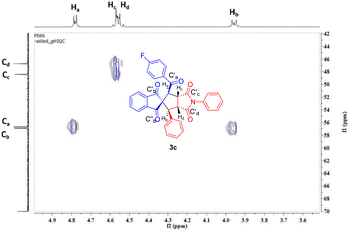 |
| Fig. 2
1H–13C HSQC spectrum of 3c. | |
The NOESY experiment of 3c revealed that Ha at 4.77 ppm is connected weakly with Hb at 3.96 ppm, while Hc at 4.58 ppm is connected very weakly with Hd at 4.56 ppm. The heteronuclear multiple-bond correlation (HMBC) experiment confirmed the correlation of Ha at 4.77 ppm with four carbonyl groups at 176.7, 174.6, 195.2 and 199.6 ppm (Fig. 3). Thus, the Hb at 3.96 ppm shows correlation with four carbonyl groups at 176.7, 174.6, 195.2 and 199.6 ppm, the Hc at 4.58 ppm shows correlation with three carbonyl groups at 174.6, 176.7, and 195.2 ppm while the Hd at 4.56 ppm shows correlation with three carbonyl groups at 174.6, 176.7 and 195.2 ppm. The presence of the fluorine atom was revealed from the fluorine NMR spectra of 3c, 3d and 3m. The DEPT-90 and DEPT-135 of 3h and 3p provided information about the methyl, methylene and methine carbons.
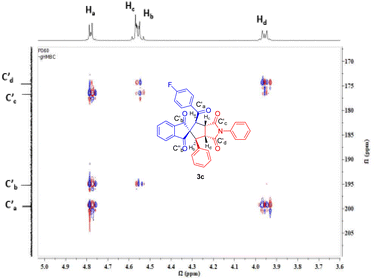 |
| Fig. 3
1H–13C HMBC spectrum of 3c. | |
The assigned stereochemical structure of compound 3l was further confirmed from its single crystal X-ray analysis (CCDC 2227184†).34 The reaction was found to be diastereoselective affording particularly one diastereomer in most of the cases except 3c (92
:
08), 3d (97
:
03), and 3o (95
:
05). The stereochemical outcome of the reaction can be rationalized based on steric factors. In the obtained structures, bulky groups are in opposite phases like bulky benzoyl and phenyl groups on the cyclopentane ring are on the opposite side of the succinimide ring.21
Inspired by the synthetic importance of N-aryl succinimide derivatives, we also explored the reactions of 2a with alkylidene pyrazolone 4a as an electrophile; however, Michael adduct 5a formation was observed instead of spirocyclization (Table 2). This reaction was optimized under the same set of reaction conditions as mentioned in Table 1.
Table 2 Optimization of reaction conditionsa
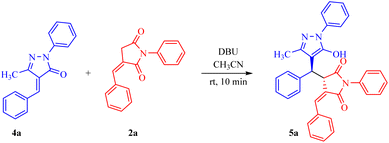
|
Entry |
Reagent |
Solvent |
Temp. |
Time |
Yieldb (%) |
Reaction conditions: all the reactions were carried out with 0.25 mmol each of 1a and 2a in the presence of 1 equiv. of reagent in 3 mL of solvent at room temperature, unless otherwise mentioned.
Isolated yield of 5a after column chromatography.
30 mol% of DBU was used.
20 mol% of DBU was used.
|
1 |
Et3N |
DCE |
rt |
10 h |
70 |
2 |
DABCO |
DCE |
rt |
10 h |
48 |
3 |
DBU |
DCE |
rt |
10 h |
72 |
4 |
DIPEA |
DCE |
rt |
10 h |
25 |
5 |
Pyridine |
DCE |
rt |
10 h |
Nr |
6 |
PTSA |
DCE |
rt |
10 h |
Nr |
7 |
BF3·OEt2 |
DCE |
rt |
10 h |
Nr |
8 |
I2 |
DCE |
rt |
10 h |
Nr |
9 |
DBU |
H2O |
rt |
10 h |
Nr |
10 |
DBU |
MeOH |
rt |
10 h |
52 |
11 |
DBU |
EtOH |
rt |
10 h |
58 |
12 |
DBU |
1,4-Dioxane |
rt |
10 h |
Nr |
13 |
DBU |
CHCl3 |
rt |
10 h |
72 |
14 |
DBU |
ACN |
rt |
1 h |
76 |
15 |
DBU |
ACN |
rt |
10 min |
76 |
16c |
DBU |
ACN |
rt |
10 min |
82 |
17d |
DBU |
ACN |
rt |
10 min |
80 |
Adduct 5a was isolated in a good yield within 10 minutes from the reaction carried out in the presence of 30 mol% DBU in acetonitrile solvent at room temperature. Furthermore, the substrate scope of the reaction was tested, and we found that most substituents, whether ERG or EWG, on both substrates were well tolerated, giving moderate to good yields of 69–86% for 5a–5g (Scheme 3). In addition, products 5h–5q were formed in good yields (72–86%) when we introduced a heterocyclic ring in N-phenyl benzylidene succinimide without altering the other substitutions in both the substrates (Scheme 4). In contrast, when the heterocyclic ring was introduced in both the substrates, the heterocycle 5r was isolated in a moderate yield of 65%.
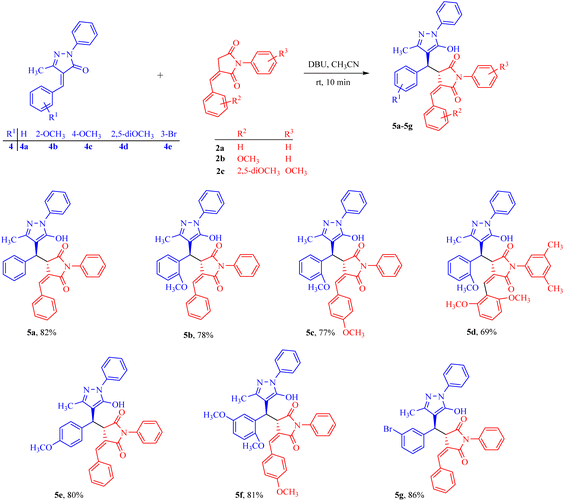 |
| Scheme 3 Reactions of pyrazolidines with benzylidene succinimides resulting in the formation of Michael adducts. | |
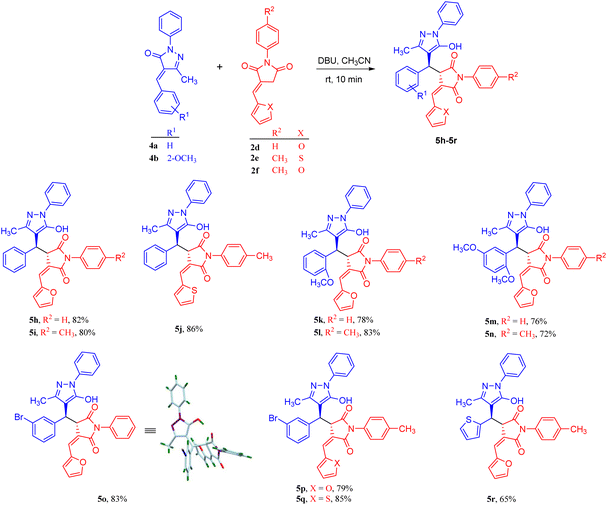 |
| Scheme 4 Reactions of pyrazolidines with furylidene succinimides resulting in the formation of Michael adducts. | |
Furthermore, the assigned structures of the products were corroborated by NMR and HRMS spectral analysis. Moreover, the structures of compounds 3c and 5o were confirmed unambiguously by 2D NMR experiments and by single crystal X-ray analysis (CCDC 2227184 and 2266707†).34 The enolic OH of 5j resonates at a chemical shift of 11.66 ppm as observed by the deuterium exchange experiment. The HSQC spectrum of 5o showed connectivity between the proton Ha at 4.73 ppm and the carbon Ca, bound directly to it, at 49.3 ppm (Fig. 4). Additionally, a correlation between Hb at 4.64 ppm and Cb at 43.4 ppm was observed. The HMBC spectroscopy studies of 5o showed the two bond and three bond correlations of Ha (δ = 4.73 ppm) with two carbonyls C′a (δ = 179.9 ppm) and C′b (δ = 168.4 ppm), respectively (Fig. 5). The NOESY spectrum of 5o indicated that Ha and Hb are on the opposite sides, i.e., anti, because the Ha proton is showing its correlation with the OH proton and the Hb proton is showing its correlation with the Hc proton.
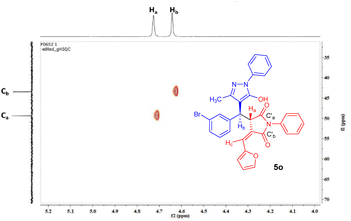 |
| Fig. 4
1H–13C HSQC spectrum of 5o. | |
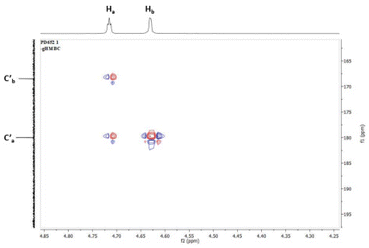 |
| Fig. 5
1H–13C HMBC spectrum of 5o. | |
Considering the reactive sites on both the reactants, we propose that there is a possibility of formation of two different regioisomers 3a and 3a′via [3 + 2] cycloaddition. However, the spiro compound 3a was obtained as a sole product and its isomer 3a′ was not observed.
On the basis of the above results and literature reports,21 a mechanistic route for the current protocol is outlined in Scheme 5. Apparently, the base DBU displays dual functionality in this reaction. Firstly, it facilitates dehydration from 1a to generate species A with a more reactive olefinic centre. Secondly, it activates nucleophile 2a to undergo a Michael attack on the β-position of the olefinic carbon of A, resulting in the formation of B, which ultimately undergoes [3 + 2] cyclization through intermediate C to yield spirocyclized tetracyclic compound 3a. The formation of 3a′ requires the attack of a nucleophile on the α-position of the electrophile. The steric hindrance at this position disfavours the formation of 3a′.
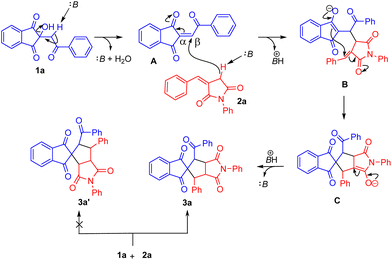 |
| Scheme 5 Plausible mechanism for the formation of 3a. | |
Similarly, we have shown the mechanistic route for the formation of 5a (Scheme 6). Here, the base abstracts the acidic proton from the nucleophile, which in turn attacks the β-position of the electrophile. This is followed by migration of the bond towards the keto group transforming it into the enol form leading to the formation of the Michael adduct.
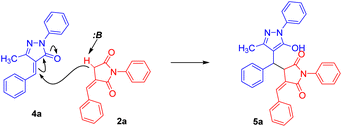 |
| Scheme 6 Plausible mechanism for the formation of 5a. | |
Conclusions
In summary, we have demonstrated the synthesis of spirocyclopentane derivatives by profound implication of reactive starting compounds, and Michael adducts by DBU catalysis under mild and metal-free conditions with high regio- and stereoselectivities. The simplicity of the experimental procedures and the ready accessibility of the raw materials thus render this an experimentally attractive method for the preparation of spirocyclopentanes and pyrazole pyrrolidinediones. The current protocol facilitates the formation of products with many contiguous stereocentres. Another adventitious aspect of the reaction is the broad substrate scope and good functional group tolerance.
Experimental
General procedure for the synthesis of 3a–3r
Indanedione 1 (0.25 mmol) and benzylidene succinimide 2 (0.25 mmol) were taken in 4 mL of acetonitrile and DBU (0.05 mmol, 20 mol%) was added, followed by refluxing for 4 h. Completion of the reaction was checked by TLC, and then the reaction mixture was diluted with DCM (15 mL). The organic layer was washed with H2O (2 × 15 mL), dried over anhydrous Na2SO4, filtered, and concentrated in vacuo. Thus, the obtained crude material was purified by column chromatography on silica gel (15% EtOAc/hexanes) to obtain pure product 3.
General procedure for the synthesis of 5a–5r
Arylidene pyrazolidinones 4 (0.25 mmol) and benzylidene succinimide 2 (0.25 mmol) were taken in 3 mL of acetonitrile and DBU (0.075 mmol, 30 mol%) was added. The reaction mixture was stirred at room temperature until the completion of reaction (10 min) as checked by TLC. After completion of the reaction, the reaction mixture was diluted with DCM (15 mL). The organic layer was washed with H2O (2 × 15 mL), dried over anhydrous Na2SO4, filtered, and concentrated in vacuo. The crude product was subjected to silica gel column chromatography (15% EtOAc/hexanes) to obtain pure product 5.
Author contributions
RKP supervised the project. PD carried out the experiments. PD and AY collected the data and wrote the original draft. The manuscript was reviewed by RKP. The fund was raised by RKP.
Conflicts of interest
There are no conflicts to declare.
Acknowledgements
We gratefully acknowledge financial support from the Science and Engineering Research Board (SERB, Grant No. CRG/2021/007619) and DST-FIST, New Delhi for providing fund (SR/FST/CS-II/2018/72 (C)) to the department for 500 MHz facility. PD thanks CSIR and AY thanks UGC, New Delhi for research fellowships.
References
-
(a) S. Das, RSC Adv., 2020, 10, 18875 RSC;
(b) P. Das, S. Maity, P. Ghosh, A. Dutta and S. Das, J. Mol. Struct., 2020, 1202, 127260 CrossRef CAS;
(c) S. Wang and G. A. Kraus, Synlett, 2019, 353 CAS.
-
(a) V. Bhajammanavar, S. Mallik, V. S. K. Choutipalli, V. Subramanian and M. Baidya, Chem. Commun., 2022, 58, 2188 RSC;
(b) M.-J. Ryu, S. Hwang, S. Kim, I. Yang, D.-C. Oh, S.-J. Nam and W. Fenical, Org. Lett., 2019, 21, 5779 CrossRef CAS PubMed;
(c) S. Asadi and G. M. Ziarani, Mol. Divers., 2016, 20, 111 CrossRef CAS PubMed.
- P. Dahiya, A. Yadav, R. Budhwan and R. K. Peddinti, Eur. J. Org. Chem., 2023, e202300251 CrossRef CAS.
-
(a) D. Giles, K. Roopa, F. R. Sheeba, P. M. Gurubasavarajaswamy, G. Divakar and T. Vidya, Eur. J. Med. Chem., 2012, 58, 478 CrossRef CAS PubMed;
(b) M. Catto, R. Aliano, A. Cellamare, F. Palluotto, R. Purgatoroi, A. D. Stradis and F. Campagna, Eur. J. Med. Chem., 2010, 45, 1359 CrossRef CAS PubMed.
- G. Le Baut, L. Sparfel, M.-H. Creuzet, C. Feniou, H. Pontagnier and G. Prat, US Pat., 4758561A, 1988 Search PubMed.
-
(a) Q. Zhang, J. P. Shrestha and C.-W. T. Chang, Tetrahedron Lett., 2014, 55, 1839 CrossRef CAS;
(b) S. Mokesch, M. S. Novak, A. Roller, M. A. Jakupec, W. Kandioller and B. K. Keppler, Organometallics, 2015, 34, 848 CrossRef CAS.
-
(a) Y. Hayakawa, T. Kobayashi and M. Izawa, J. Antibiot., 2013, 66, 731 CrossRef CAS PubMed;
(b) A. Thangamani, Eur. J. Med. Chem., 2010, 45, 6120 CrossRef CAS PubMed;
(c) C. E. Cook, M. C. Wani, J. M. Jump, Y.-W. Lee, P. A. Fail, S. A. Anderson, Y.-Q. Gu and V. Petrow, J. Med. Chem., 1995, 38, 753 CrossRef CAS PubMed.
-
(a) S. Kotha, S. R. Cheekatla and A. Fatma, ACS Omega, 2019, 4, 17109 CrossRef CAS PubMed;
(b) G. Bhaskar, Y. Arun, C. Balachandran, C. Saikumar and P. T. Perusmal, Eur. J. Med. Chem., 2012, 51, 79 CrossRef CAS PubMed.
-
(a) K. Luo, Y. Bao, F. Liu, C. Xiao, K. Li, C. Zhang, R. Huang, J. Lin, J. Zhang and Y. Jin, Eur. J. Med. Chem., 2019, 179, 805 CrossRef CAS PubMed.
-
(a) X.-Y. Zhang, Y. You, Z.-H. Wang, J.-Q. Zhao and W.-C. Yuan, Chem. Commun., 2020, 56, 13449 RSC;
(b) J. Wang, H. Liu, Y. Fan, Y. Yang, Z. Jiang and C.-H. Tan, Chem. – Eur. J., 2010, 16, 12534 CrossRef CAS PubMed.
- W. Yang, D. Tan, L. Liu, Z. Han, L. Yan, K.-W. Huang, C.-H. Tan and Z. Jiang, J. Org. Chem., 2012, 77, 6600 CrossRef CAS PubMed.
- C. Yuan, X. Ning, T. Gao, Z. Zeng, K. Lee, Y. Xing, S. Sun and G. Wang, Asian J. Org. Chem., 2022, 11, e202100699 CrossRef CAS.
-
(a) L. Liu and D.-M. Du, Org. Biomol. Chem., 2016, 14, 6337 RSC;
(b) S. G. Hegde, L. Koodlur and M. Narayanarao, Synth. Commun., 2019, 49, 3453 CrossRef;
(c) W.-L. Yang, Y.-Z. Liu, S. Luo, X. Yu, J. S. Fossey and W.-P. Deng, Chem. Commun., 2015, 51, 9212 RSC.
-
(a) S. Hadded, S. Boudriga, F. Porzio, A. Soldera, M. Askri, D. Sriram, P. Yogeeswari, M. Knorr, Y. Rousseline and M. M. Kubicki, RSC Adv., 2014, 4, 59462 RSC;
(b) T. A. King, H. L. Stewart, K. T. Mortensen, A. J. P. North, H. F. Sore and D. R. Spring, Eur. J. Org. Chem., 2019, 5219 CrossRef CAS PubMed.
-
(a) S. Boudriga, S. Hadded, M. Askri, A. Soldera, M. Knorr, C. Strohmannd and C. Golz, RSC Adv., 2019, 9, 11082 RSC;
(b) X. Wang, P. Yang, Y. Zhang, C.-Z. Tang, F. Tian, L. Peng and L.-X. Wang, Org. Lett., 2017, 19, 646 CrossRef CAS PubMed.
- S. Haddad, S. Boudriga, F. Porzio, A. Soldera, M. Askri, D. Sriram, P. Yogeeswari, M. Knorr, Y. Rousselin and M. M. Kubicki, RSC Adv., 2014, 4, 59462 RSC.
-
(a) C.-Y. Li, M. Xiang, X.-J. Song, Y. Zou, Z.-C. Huang, X. Li, F. Tian and L.-X. Wang, Org. Biomol. Chem., 2020, 18, 951 Search PubMed;
(b) W.-L. Yang, Y.-Z. Liu, S. Luo, X. Yu, J. S. Fossey and W.-P. Deng, Chem. Commun., 2015, 51, 9212 RSC.
- W. Luo, H. Hu, S. Nian, L. Qi, F. Ling and W. Zhong, Org. Biomol. Chem., 2017, 15, 7523 RSC.
-
(a) S. Boudriga, S. Haddad, M. Askri, A. Soldera, M. Knorr, C. Strohmann and C. Golz, RSC Adv., 2019, 9, 11082 RSC;
(b) M.-X. Zhao, Q. Liu, K.-M. Yu, X.-L. Zhao and M. Shi, Org. Chem. Front., 2019, 6, 3879 RSC.
-
(a) S. Haddad, S. Boudriga, T. N. Akhaja, J. P. Raval, F. Porzio, A. Soldera, M. Askri, M. Knorr, Y. Rousselin, M. M. Kubicki and D. Rajani, New J. Chem., 2015, 39, 520 RSC;
(b) W.-J. Luo, H.-W. Hu, S.-F. Nian, L. Qi, F. Ling and W.-H. Zhong, Org. Biomol. Chem., 2017, 15, 7523 RSC;
(c) S. Boudriga, S. Haddad, M. Askri, A. Soldera, M. Knorr, C. Strohmann and C. Golz, RSC Adv., 2019, 9, 11082 RSC;
(d) S. Lin, D. Leow, K.-W. Huang and C.-H. Tan, Chem. – Asian J., 2009, 4, 1741 CrossRef CAS PubMed.
-
(a) P. Dahiya, A. Yadav, M. Rawat and R. K. Peddinti, Asian J. Org. Chem., 2022, 11, e202200571 CrossRef CAS;
(b) P. Tehri and R. K. Peddinti, Org. Biomol. Chem., 2019, 17, 3964 RSC;
(c) Y. Liu and W. Zhang, Angew. Chem., Int. Ed., 2013, 52, 2203 CrossRef CAS PubMed;
(d) Z. Gan, M. Zhi, R. Han, E.-Q. Li, Z. Duan and F. Mathey, Org. Lett., 2019, 21, 2782 CrossRef CAS PubMed;
(e) S. Laru, S. Bhattacharjee, M. Singsardar, S. Samanta and A. Hajra, J. Org. Chem., 2021, 86, 2784 CrossRef CAS PubMed.
-
(a) B.-L. Zhao and D.-M. Du, Chem. Commun., 2016, 52, 6162 RSC;
(b) W.-L. Yang, Y.-Z. Liu, S. Luo, X. Yu, J. S. Fossey and W.-P. Deng, Chem. Commun., 2015, 51, 9212 RSC.
-
(a) V. Kumar, K. Kaur, G. K. Gupta and A. K. Sharma, Eur. J. Med. Chem., 2013, 69, 735 CrossRef CAS PubMed;
(b) H. X. Dai, A. F. Stepan, M. S. Plummer, Y. H. Zhang and J. Q. Yu, J. Am. Chem. Soc., 2011, 133, 7222 CrossRef CAS PubMed;
(c) L. H. Jones, G. Allan, R. Corbau, D. S. Middleton, C. E. Mowbray, S. D. Newman, C. Phillips, R. Webster and M. Westby, Chem. Biol. Drug Des., 2011, 77, 393 CrossRef CAS PubMed;
(d) W. J. Lominac, M. L. D. Angelo, M. D. Smith, D. A. Ollison and J. M. Hanna, Tetrahedron Lett., 2012, 53, 906 CrossRef CAS PubMed;
(e) M. Tang, Y. Kong, B. Chu and D. Feng, Adv. Synth. Catal., 2016, 358, 926 CrossRef CAS;
(f) J. Pang, Z. G. Xi, M. Chen, J. Wang and Q. Zhu, Org. Lett., 2014, 16, 4702 CrossRef PubMed;
(g) S. Fustero, M. Sanchez-Rosello, P. Barrio and A. Simon-Fuentes, Chem. Rev., 2011, 111, 6984 CrossRef CAS PubMed.
-
(a) M. Shaharyar, M. M. Abdullah, M. A. Bakht and J. Majeed, Eur. J. Med. Chem., 2010, 45, 114 CrossRef CAS PubMed;
(b) A. H. Bandey, B. P. Mir, I. H. Lone, K. A. Suri and H. M. Kumar, Steroids, 2010, 75, 805 CrossRef PubMed;
(c) M. Johnson, B. Younglove, L. Lee, R. LeBlanc, H. Holt Jr., P. Hills, H. Mackay, T. Brown, S. L. Mooberry and M. Lee, Bioorg. Med. Chem. Lett., 2007, 17, 5897 CrossRef CAS PubMed.
-
(a) M. E. Camacho, J. Leon, A. Entrena, G. Velasco, M. D. Carrion, G. Escames, A. Vivo, D. Acuna-Castroviejo, M. A. Gallo and A. Espinosa, J. Med. Chem., 2004, 47, 5641 CrossRef CAS PubMed;
(b) L. Tamborine, Y. Chen, C. A. Foss, A. Pinto and A. G. Horty,
et al.
, J. Med. Chem., 2016, 59, 11110 CrossRef PubMed.
-
(a) Z. Ozdemir, H. B. Kandilci, B. Gumusel, U. Calis and A. A. Bilgin, Eur. J. Med. Chem., 2007, 42, 373 CrossRef PubMed;
(b) Y. R. Prasad, A. L. Rao, L. Prasoona, K. Murali and P. R. Kumar, Bioorg. Med. Chem. Lett., 2005, 15, 5030 CrossRef CAS PubMed.
-
(a) A. Ahmad, A. Husain, S. A. Khan, M. Mujeeb and A. Bhandari, J. Saudi Chem. Soc., 2016, 20, 577 CrossRef CAS;
(b) A. Burmudzija and J. Muskinja,
et al.
, Inorg. Chim. Acta, 2018, 471, 570 CrossRef CAS.
-
(a) N. Beyhan, B. Kocyigit-Kaymakcioglu, S. Gumru and F. Aricioglu, Arabian J. Chem., 2017, 10, S2073 CrossRef CAS.
-
(a) G. Kumar, O. Tanwar, J. Kumar, M. Akhter and S. Sharma, Eur. J. Med. Chem., 2018, 149, 139 CrossRef CAS PubMed;
(b) A. K. Pndey, S. Sharma, M. Pandey, M. M. Alam, M. Shaquiquzzaman and M. Akhter, Eur. J. Med. Chem., 2016, 123, 476 CrossRef PubMed;
(c) B. N. Acharya, D. Saraswat, M. Tiwari, A. K. Shrivastava, R. Ghorpade, S. Bapna and M. P. Kaushik, Eur. J. Med. Chem., 2010, 45, 430 CrossRef CAS PubMed.
-
(a) H. P. A. Khan, D. Das and T. K. Chakraborty, J. Org. Chem., 2018, 83, 6086 CrossRef CAS PubMed;
(b) K. Morisaki, Y. Sasano, T. Koseki, T. Shibuta, N. Kanoh, W.-H. Chiou and Y. Iwabuchi, Org. Lett., 2017, 19, 5142 CrossRef CAS PubMed;
(c) A. Goto, S. Yoshimura, Y. Nakao, M. Inai, T. Asakawa, M. Egi, Y. Hamashima and M. Kondo, Org. Lett., 2017, 19, 3358 CrossRef CAS PubMed;
(d) B. M. Trost and T. M. Lam, J. Am. Chem. Soc., 2012, 134, 11319 CrossRef CAS PubMed;
(e) Z. Lu, M. Shen and T. P. Yoon, J. Am. Chem. Soc., 2011, 133, 1162 CrossRef CAS PubMed.
-
(a) P. Verma, A. Mishra, S. Chauhan, S. Singh and V. Srivastava, ChemistrySelect, 2019, 4, 5394 CrossRef CAS.
-
(a) P. Bhatt, M. Kumar and A. Jha, Mol. Diversity, 2018, 22, 827 CrossRef CAS PubMed;
(b) E. Hedaya and S. Theodoropulos, Tetrahedron, 1968, 24, 2241 CrossRef CAS;
(c) K. P. Haval and N. P. Argade, J. Org. Chem., 2008, 73, 6936 CrossRef CAS PubMed.
-
(a) H. Sheibani and M. Babaie, Synth. Commun., 2010, 40, 257 CrossRef CAS.
- Crystallographic data (excluding structure factors) for 3l (CCDC 2227184) and 5o (CCDC 2266707) have been deposited with the Cambridge Crystallographic Data Centre.†.
|
This journal is © The Royal Society of Chemistry 2023 |
Click here to see how this site uses Cookies. View our privacy policy here.