DOI:
10.1039/D2QO01562E
(Research Article)
Org. Chem. Front., 2023,
10, 109-114
Palladium-catalyzed intramolecular C–H amination using aluminum nitrate as the oxidant†
Received
5th October 2022
, Accepted 12th November 2022
First published on 15th November 2022
Abstract
A palladium catalyzed intramolecular C(sp2)–H amination using a readily available aluminum nitrate (Al(NO3)3·9H2O) as the oxidant is reported. The C–H amination is promoted by in situ nitration of the quinoline directing group (DG). The oxindole products can be efficiently converted into novel polycyclic compounds (up to 7 fused rings) via a sequential reduction/cyclization involving the DG. Mechanistic studies indicate that nitration of the quinoline DG favors the following activation of γ-aryl C(sp2)–H bonds over β-methyl C(sp3)–H bonds.
Introduction
Intramolecular C–N bond formation via the activation of the C–H bond1 has become a general and practical tool for the synthesis of N-based molecules including important heterocycles such as lactams,2 indoles,3 indolines,4 oxindoles,5 carbozoles,6etc. The straightforward approach could simplify substrate preparation and facilitate novel retrosynthetic planning in view of atom economy and synthetic simplicity. Oxindoles have attracted a lot of interest as important structural motifs present in natural products and pharmacologically active compounds (Scheme 1a).7 The synthesis of oxindoles via C–H amination was first reported by Yu in 2008 through the palladium-catalyzed intramolecular amination of ortho C(sp2)–H bonds of N-methoxyhydroxamic acids using a combination of CuCl2/AgOAc as the oxidant (Scheme 1b).5a In 2009, Murakami and co-workers reported a C–H amination of N-tosylphenylacetamide derivatives with Cu(OAc)2.5b Since then, a few examples have been reported where oxindoles have been synthesized through C(sp2)–H amination using palladium,2a,h copper2b and cobalt catalysts2c with PhI(OAc)2 or copper/silver based oxidants. In addition, a ligand-assisted enantioselective amination of C(sp2)–H bonds was reported recently by Wang using Ag2O as the oxidant.5c Nevertheless, the selectivity between the methyl sp3 C–H bonds and the aryl sp2 C–H bonds has always caused an issue in these methods. Herein, we report a simple and efficient reaction for the synthesis of oxindoles promoted by in situ directing group (DG) modification using Al(NO3)3·9H2O as a readily available oxidant8 (Scheme 1c). The oxindole products can be efficiently converted into novel polycyclic compounds involving the DG.
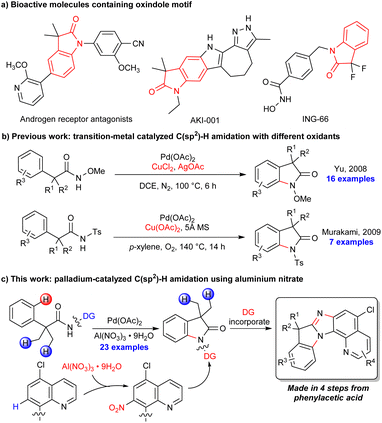 |
| Scheme 1 Oxindole containing molecules and transition-metal catalyzed C(sp2)–H amination. | |
The 8-aminoquinoline (AQ) group, first introduced by Daugulis et al.,9 has shown great ability and success in a variety of C–H functionalization reactions.10 A number of reactions on AQ itself were discovered during these studies,11 and the use of modified/substituted AQ was also reported to avoid AQ reactions or to enhance its directing ability for C–H functionalization reactions.12 In our study, the 5-chloro AQ auxiliary on the substrate was nitrated13in situ at its C7 position prior to the C–H amination reaction, and a reduction/cyclization with the nitro group could efficiently convert the oxindole products to novel polycyclic compounds involving the DG (Scheme 1c). In the past few decades, DGs have played an important role in transition metal-catalyzed site-selective C–H activations, and a variety of mono- or bidentate chelating groups have been identified as powerful DGs.14 However, the installation and removal of these DGs add additional steps, compromising the step-economical nature of the overall C–H activation strategy.15 Our work demonstrated an in situ DG modification strategy to enhance its directing ability for C–H functionalization and to incorporate DGs into the targeted products16 for an efficient synthesis of complex molecules.
Results and discussion
We began our research with α,α-dimethyl amide 1a with the 5-Cl AQ auxiliary as the model substrate. After a careful evaluation of various reaction parameters (see the ESI†), the C(sp2)–H amination product 2a was isolated in 80% yield under simple conditions with Pd(OAc)2 as the catalyst, Al(NO3)3·9H2O as the oxidant, and CH3CN as the solvent (Table 1). No C(sp3)–H amination on the α-methyl group was observed in the reaction. Then, control experiments were conducted to understand the role of each reactant. The use of the 5-Cl AQ auxiliary was crucial for this transformation. Directing groups such as AQ (R1 = H) or AQs with different substituents (R1 = OMe or NO2) all led to no desired product formation (entries 2–4). A range of other nitrates or nitrite-based oxidants were examined, and only the nitration intermediate 1a′ was observed (entries 5–8). As expected, the palladium catalyst was essential for this transformation, while the nitration of AQ could be promoted by the nitrate itself (entry 9). The yield decreased when the reaction was conducted at a lower temperature, while a higher temperature led to no improvement (entries 10 and 11). The addition of H2O as the cosolvent was detrimental to the reaction and no desired product was observed in DCE (entries 12 and 13). Notably, the reaction gave a comparable yield under an argon atmosphere (entry 14).
Table 1 Selected optimization and control experimentsa
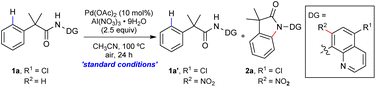
|
Entry |
Variations from the ‘standard conditions’ |
1a′/2a b [%] |
Standard conditions: substrate 1 (0.2 mmol, 1.0 equiv.), Pd(OAc)2 (10 mol%), Al(NO3)3·9H2O (2.5 equiv.), CH3CN (2 mL), at 100 °C, 24 h, under air.
Yields determined by 1H NMR spectroscopy using 1,3,5-trimethoxbenzene as the internal standard.
Isolated yield.
|
1 |
None |
Trace/80c |
2 |
1aa, R1 = H |
Trace/0 |
3 |
1ab, R1 = OMe |
Trace/0 |
4 |
1ac, R1 = NO2 |
<10/0 |
5 |
Fe(NO3)3·9H2O instead of Al(NO3)3·9H2O |
75c/trace |
6 |
Cu(NO3)3·3H2O instead of Al(NO3)3·9H2O |
42/0 |
7 |
Co(NO3)2·6H2O instead of Al(NO3)3·9H2O |
45/0 |
8 |
t-BuONO instead of Al(NO3)3·9H2O |
70/trace |
9 |
Without Pd(OAc)2 |
55/0 |
10 |
At 90 °C |
20/55 |
11 |
At 110 °C |
Trace/78c |
12 |
CH3CN/H2O (2 : 1) as solvent |
0/trace |
13 |
DCE as solvent |
70/0 |
14 |
Under Ar |
Trace/77c |
The scope of the C(sp2)–H amination reaction was then examined under the optimized conditions (Scheme 2). The structure of 2a was confirmed by X-ray crystallography. Substrates bearing diverse substituents at the para position on the phenyl ring furnished the desired oxindoles (2b–2h) in good yields. The electronic density of the phenyl ring did not affect the yields, and halogen or triflate groups were tolerated in the reaction (2b–2d). Biaryl substrates also reacted smoothly under these conditions (2f–2h). With meta substituents, the amination occurred exclusively at the less hindered 6-position (2i, 2j). For amides with different alkyl (ethyl, cyclopentyl, benzyl or butyl) or aryl substituents at the α-position, the desired oxindoles could be obtained in modest to good yields (2k–2p). Remarkably, α-cyclic substrates containing three- to six-membered rings all worked well to give the spiro-oxindole products (2q–2v). Further modification of the DG at the C3 position with a bromo substituent did not affect the reaction (2w). Unfortunately, α-nonsubstituted substrates failed to afford the C(sp2)–H amination product (2x), due to the gem-dialkyl effect, which was commonly observed in such cyclization reactions.17
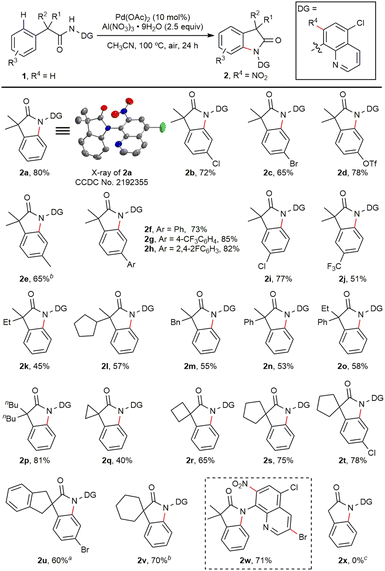 |
| Scheme 2 Substrate scope of the C(sp2)–H amination. Conditions: substrate 1 (0.1 mmol, 1.0 equiv.), Pd(OAc)2 (10 mol%), Al(NO3)3·9H2O (2.5 equiv.), CH3CN (2 mL), at 100 °C, 24 h, under air, isolated yields. a Al(NO3)3·9H2O (3.5 equiv.) was used. b 90 °C, 48 h. c Only the nitrated intermediate was formed. | |
The practical transformation could be scaled up to the gram scale using substrate 1a as an example; the auxiliary group in the oxindole products could be removed through nitro reduction with Fe/AcOH (2aa) followed by oxidation with ceric ammonium nitrate (CAN) to afford the free amide 4 (Scheme 3, see the ESI†).18 Cyclization of 2aa with TsOH efficiently led to a novel polycyclic compound 3a. Derivatization of the 5-chloro-7-nitro AQ auxiliary could be achieved through the nucleophilic substitution of the nitro group to obtain sulphide 519 and Suzuki coupling with arylboric acid to obtain the biaryl compound 6.20
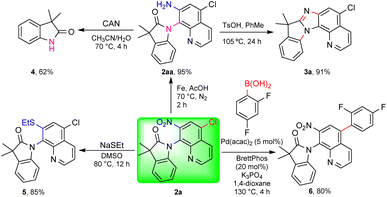 |
| Scheme 3 Auxiliary group removal and derivatization. | |
The synthesis of 3a demonstrated the power of C–H activation and the strategy of embedding the DG in the targeted product. 3a could be produced in just 4 steps starting from α,α-dimethylphenylacetic acid. Then we studied the scope of this reduction/cyclization sequence with more oxindole products (Scheme 4). With different substituents on the phenyl (3b, 3e) and quinolinyl (3g) rings, at the α-position (3h), and α-cyclic substrates (3c–3f), the reaction gave good to excellent yields of the polycyclic products. The structure of 3a was confirmed by X-ray crystallography.
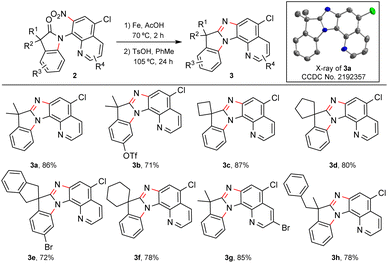 |
| Scheme 4 Synthesis of novel polycyclic compounds. Isolated yields are given. | |
To understand the mechanism of the C(sp2)–H amination reaction, especially the selectivity of the C–H activation of different C–H bonds, some control experiments, a kinetic isotope effect (KIE) study and the trapping of palladacycle intermediates were conducted (Scheme 5). A stepwise control of the reaction showed that the nitration intermediate 1a′ could be obtained in 84% yield with 1.0 equivalent of Al(NO3)3·9H2O and the palladium catalyst at 90 °C for 8 hours. When 1a′ was isolated and subjected again to 1.5 equivalents of Al(NO3)3·9H2O with the palladium catalyst at 100 °C for 12 hours, the targeted product 2a was obtained in 77% yield. The results indicated that the nitration of the quinolinyl ring might occur prior to the amination step. When the nitro group in 1a′ was replaced with chlorine (1ad), no desired amination product was observed, which showed the importance of the nitro group in enhancing the directing ability of the DG and improving the reactivity of the C–H amination. The intramolecular competitive KIE study was carried out with 1a-d1 to obtain a value of 1.33, which suggested that the cleavage of the C(sp2)–H bond was probably not involved in the rate-determining step. This result inspired us to characterize the C–H insertion palladium complex using the strategy of ligand stabilization to gain more insights into the selectivity of C–H activation between the γ-aryl sp2 C–H bonds and the β-methyl sp3 C–H bonds. Gratifyingly, the sp3 C–H insertion palladium complex 7 was isolated by treating 1a with Pd(OAc)2 and Bu3P in CH3CN at room temperature, while the sp2 C–H insertion palladium intermediate 8 was detected when 1a′ was used under the same conditions. The structures of both complexes were confirmed by X-ray crystallography. These intermediates provided direct evidence for the role of the nitro group on the DG in favoring the sp2 C–H bond cleavage over the sp3 C–H bond cleavage. We anticipated that the oxidation of 8 by the nitrate8a would afford the high-valence Pd(IV) intermediate, which would undergo reductive elimination to form a C–N bond and release the oxindole product. The reductive elimination might be the rate-determining step and the high-valence Pd(IV) intermediate generated from 7 would be unfavorable for delivering the β-lactam product.
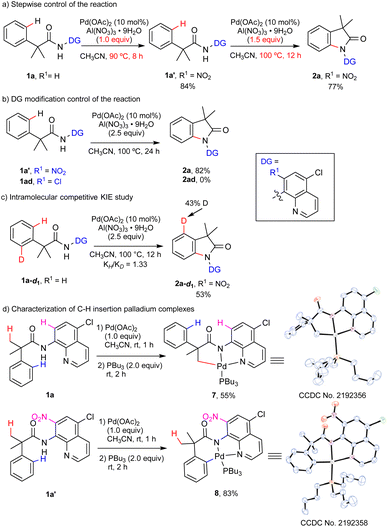 |
| Scheme 5 Control experiments, KIE study and characterization of C–H insertion palladium complexes. | |
Conclusions
In summary, a palladium-catalyzed intramolecular C(sp2)–H amination reaction utilizing aluminum nitrate as the oxidant is reported. The in situ modification of the DG and the incorporation of the DG into the final product might provide some insights into the development and application of new C–H functionalization reactions.
Conflicts of interest
There are no conflicts to declare.
Acknowledgements
This work was supported by the National Natural Science Foundation of China (NSFC) (grant no. 21772092 and 22075144).
References
- For selected reviews, see:
(a) P. J. Borpatra, B. Deka, M. L. Deb and P. K. Baruah, Recent advances in intramolecular C–O/C–N/C–S bond formation via C–H functionalization, Org. Chem. Front., 2019, 6, 3445 RSC;
(b) M. Zhang, Q. Wang, Y. Peng, Z. Chen, C. Wan, J. Chen, Y. Zhao, R. Zhang and A. Q. Zhang, Transition metal-catalyzed sp3 C–H activation and intramolecular C–N coupling to construct nitrogen heterocyclic scaffolds, Chem. Commun., 2019, 55, 13048 RSC;
(c) Y. N. Timsina, B. F. Gupton and K. C. Ellis, Palladium-catalyzed C–H amination of C(sp2) and C(sp3)–H bonds: Mechanism and scope for N-based molecule synthesis, ACS Catal., 2018, 8, 5732 CrossRef CAS;
(d) Y. Park, Y. Kim and S. Chang, Transition metal-catalyzed C–H amination: Scope, mechanism, and applications, Chem. Rev., 2017, 117, 9247 CrossRef CAS PubMed;
(e) J. Jiao, K. Murakami and K. Itami, Catalytic methods for aromatic C–H amination: An ideal strategy for nitrogen-based functional molecules, ACS Catal., 2015, 6, 610 CrossRef;
(f) B. Desai, M. Patel, B. Z. Dholakiya, S. Rana and T. Naveen, Recent advances in directed sp2 C–H functionalization towards the synthesis of N-heterocycles and O-heterocycles, Chem. Commun., 2021, 57, 8699 RSC.
-
(a) G. He, S. Y. Zhang, W. A. Nack, Q. Li and G. Chen, Use of a readily removable auxiliary group for the synthesis of pyrrolidones by the palladium-catalyzed intramolecular amination of unactivated γ C(sp3)–H bonds, Angew. Chem., Int. Ed., 2013, 52, 11124 CrossRef CAS;
(b) Z. Wang, J. Ni, Y. Kuninobu and M. Kanai, Copper-catalyzed intramolecular C(sp3)–H and C(sp2)–H amidation by oxidative cyclization, Angew. Chem., Int. Ed., 2014, 53, 3496 CrossRef CAS;
(c) X. Wu, K. Yang, Y. Zhao, H. Sun, G. Li and H. Ge, Cobalt-catalysed site-selective intra- and intermolecular dehydrogenative amination of unactivated sp3 carbons, Nat. Commun., 2015, 6, 6462 CrossRef CAS PubMed;
(d) X. Wu, Y. Zhao, G. Zhang and H. Ge, Copper-catalyzed site-selective intramolecular amidation of unactivated C(sp3)–H bonds, Angew. Chem., Int. Ed., 2014, 53, 3706 CrossRef CAS PubMed;
(e) P. Roy, J. R. Bour, J. W. Kampf and M. S. Sanford, Catalytically relevant intermediates in the Ni-catalyzed C(sp2)–H and C(sp3)–H functionalization of aminoquinoline substrates, J. Am. Chem. Soc., 2019, 141, 17382 CrossRef CAS;
(f) J. Kweon and S. Chang, Highly robust iron catalyst system for intramolecular C(sp3)–H amidation leading to γ-lactams, Angew. Chem., Int. Ed., 2021, 60, 2909 CrossRef CAS;
(g) P. X. Ling, S. L. Fang, X. S. Yin, Q. Zhang, K. Chen and B. F. Shi, Palladium-catalyzed sequential monoarylation/amidation of C(sp3)–H bonds: Stereoselective synthesis of α-amino-β-lactams and anti-α,β-diamino acid, Chem. Commun., 2017, 53, 6351 RSC;
(h) S. Liu, Z. Zhuang, J. X. Qiao, K.-S. Yeung and S. Su, Ligand enabled Pd(II)-catalyzed γ-C(sp3)–H lactamization of native amides, J. Am. Chem. Soc., 2021, 143, 21657 CrossRef CAS PubMed;
(i) K. Nozawa-Kumada, S. Saga, Y. Matsuzawa, M. Hayashi, M. Shigeno and Y. Kondo, Copper-catalyzed oxidative benzylic C(sp3)–H cyclization for the synthesis of β-lactams, Chem. – Eur. J., 2020, 26, 4496 CrossRef CAS PubMed;
(j) J. Long, L. Le, T. Iwasaki, R. Qiu and N. Kambe, Copper-catalyzed amination of C(sp3)–H bonds: From anilides to indolines, J. Org. Chem., 2020, 85, 482 CrossRef CAS PubMed.
-
(a) D. I. Bugaenko, A. A. Dubrovina, M. A. Yurovskaya and A. V. Karchava, Synthesis of indoles via electron-catalyzed intramolecular
C–N bond formation, Org. Lett., 2018, 20, 7358 CrossRef CAS PubMed;
(b) M. Matsumoto, K. Wada, K. Urakawa and H. Ishikawa, Diaryliodonium salt-mediated intramolecular C–N bond formation using boron-masking N-hydroxyamides, Org. Lett., 2020, 22, 781 CrossRef CAS;
(c) B. S. Zhang, F. Wang, Y. H. Yang, X. Y. Gou, Y. F. Qiu, X. C. Wang, Y. M. Liang, Y. Li and Z. J. Quan, Synthesis of C4-substituted indoles via a Catellani and C–N bond activation strategy, Org. Lett., 2020, 22, 8267 CrossRef CAS PubMed;
(d) J. Li, J. Chen, L. Wang and Y. Shi, Palladium-catalyzed sequential C–H activation/amination with diaziridinone: An approach to indoles, Org. Lett., 2021, 23, 3646 CrossRef CAS;
(e) H. Zhang, D. Yang, X.-F. Zhao, J.-L. Niu and M.-P. Song, Cobalt-catalyzed C(sp3)–H bond functionalization to access indole derivatives, Org. Chem. Front., 2022, 9, 3723 RSC.
-
(a) G. He, C. Lu, Y. Zhao, W. A. Nack and G. Chen, Improved protocol for indoline synthesis via palladium-catalyzed intramolecular C(sp2)–H amination, Org. Lett., 2012, 14, 2944 CrossRef CAS;
(b) G. He, Y. Zhao, S. Zhang, C. Lu and G. Chen, Highly efficient syntheses of azetidines, pyrrolidines, and indolines via palladium catalyzed intramolecular amination of C(sp3)–H and C(sp2)–H bonds at γ and δ positions, J. Am. Chem. Soc., 2012, 134, 3 CrossRef CAS PubMed;
(c) E. T. Nadres and O. Daugulis, Heterocycle synthesis via direct C–H/N–H coupling, J. Am. Chem. Soc., 2012, 134, 7 CrossRef CAS;
(d) H. Xu, X. Zhang, Z. Ke and C. A. Zhao, A theoretical study of dirhodium-catalyzed intramolecular aliphatic C–H bond amination of aryl azides, RSC Adv., 2016, 6, 29045 RSC;
(e) T.-S. Mei, D. Leow, H. Xiao, B. N. Laforteza and J.-Q. Yu, Synthesis of indolines via Pd(II)-catalyzed amination of C–H bonds using PhI(OAc)2 as the bystanding oxidant, Org. Lett., 2013, 15, 3058 CrossRef CAS;
(f) X. Sun, Z. Wu, W. Qi, X. Ji, C. Cheng and Y. Zhang, Synthesis of indolines by palladium-catalyzed intermolecular amination of unactivated C(sp3)–H bonds, Org. Lett., 2019, 21, 6508 CrossRef CAS.
-
(a) M. Wasa and J. Q. Yu, Synthesis of β-, γ-, and δ-Lactams via Pd(II)-catalyzed C–H activation reactions, J. Am. Chem. Soc., 2008, 130, 14058 CrossRef CAS PubMed;
(b) T. Miura, Y. Ito and M. Murakami, Synthesis of oxindoles by palladium-catalyzed C–H bond amidation, Chem. Lett., 2009, 38, 328 CrossRef CAS;
(c) X.-F. Cheng, F. Fei, Y. Li, Y.-M. Hou, X. Zhou and X.-S. Wang, Ligand-accelerated palladium(II)-catalyzed enantioselective amination of C(sp2)–H bonds, Org. Lett., 2020, 22, 6394 CrossRef CAS PubMed.
-
(a) S. Zhang, H. Ma, H. E. Ho, Y. Yamamoto, M. Bao and T. Jin, Pd-catalyzed cascade cyclization of o-alkynylanilines via C−H/C−N bond cleavage leading to dibenzo[a,c]carbazoles, Org. Biomol. Chem., 2018, 16, 5236 RSC;
(b) H. R. Bjørsvik and V. Elumalai, Synthesis of the carbazole scaffold directly from 2-aminobiphenyl by means of tandem C–H activation and C–N bond formation, Eur. J. Org. Chem., 2016, 5474 CrossRef;
(c) S. V. Kumar, S. Banerjee and T. Punniyamurthy, Rh-catalyzed C–C/C–N bond formation via C–H activation: Synthesis of 2H-indazol-2-yl-benzo[a]carbazoles, Org. Chem. Front., 2019, 6, 3885 RSC.
-
(a) I. N. Gaisina, S. H. Lee, N. A. Kaidery, M. Ben Aissa, M. Ahuja, N. N. Smirnova, S. Wakade, A. Gaisin, M. W. Bourassa, R. R. Ratan, S. V. Nikulin, A. A. Poloznikov, B. Thomas, G. R. J. Thatcher and I. G. Gazaryan, Activation of Nrf2 and hypoxic adaptive response contribute to neuroprotection elicited by phenylhydroxamic acid selective HDAC6 inhibitors, ACS Chem. Neurosci., 2018, 9, 894 CrossRef CAS;
(b) A. D. Marchese, E. M. Larin, B. Mirabi and M. Lautens, Metal-catalyzed approaches toward the oxindole core, Acc. Chem. Res., 2020, 53, 1605 CrossRef CAS PubMed;
(c) W. Shi, Z. Jiang, H. He, F. Xiao, F. Lin, Y. Sun, L. Hou, L. Shen, L. Han, M. Zeng, K. Lai, Z. Gu, X. Chen, T. Zhao, L. Guo, C. Yang, J. Li and S. Chen, Discovery of 3,3′-spiro[azetidine]-2-oxo-indoline derivatives as fusion inhibitors for treatment of RSV infection, ACS Med. Chem. Lett., 2018, 9, 94 CrossRef CAS PubMed;
(d) C. Guo, M. Pairish, A. Linton, S. Kephart, M. Ornelas, A. Nagata, B. Burke, L. Dong, J. Engebretsen and A. N. Fanjul, Design of oxobenzimidazoles and oxindoles as novel androgen receptor antagonists, Bioorg. Med. Chem. Lett., 2012, 22, 2572 CrossRef CAS;
(e) F. Ran, H. Xing, Y. Liu, D. Zhang, P. Li and G. Zhao, Recent developments in androgen receptor antagonists, Arch. Pharm., 2015, 348, 757 CrossRef CAS PubMed;
(f) T. E. Rawson, M. Rüth, E. Blackwood, D. Burdick, L. Corson, J. Dotson, J. Drummond, C. Fields, G. J. Georges, B. Goller, J. Halladay, T. Hunsaker, T. Kleinheinz, H.-W. Krell, J. Li, J. Liang, A. Limberg, A. McNutt, J. Moffat, G. Phillips, Y. Ran, B. Safina, M. Ultsch, L. Walker, C. Wiesmann, B. Zhang, A. Zhou, B.-Y. Zhu, P. Rüger and A. G. Cochran, A Pentacyclic aurora kinase inhibitor (AKI-001) with high in vivo potency and oral bioavailability, J. Med. Chem., 2008, 51, 4465 CrossRef CAS PubMed.
-
(a) M. N. Wenzel, P. K. Owens, J. T. Bray, J. M. Lynam, P. M. Aguiar, C. Reed, J. D. Lee, J. F. Hamilton, A. C. Whitwood and I. J. Fairlamb, Redox couple involving NOx in aerobic Pd-catalyzed oxidation of sp3–C–H bonds: Direct evidence for Pd–NO3−/NO2− interactions involved in oxidation and reductive elimination, J. Am. Chem. Soc., 2017, 139, 1177 CrossRef CAS PubMed;
(b) Y. Xue, H. S. Park, C. Jiang and J.-Q. Yu, Palladium-catalyzed β-C(sp3)–H nitrooxylation of ketones and amides using practical oxidants, ACS Catal., 2021, 11, 14188 CrossRef CAS.
- V. G. Zaitsev, D. Shabashov and O. Daugulis, Highly regioselective arylation of sp3 C−H bonds catalyzed by palladium acetate, J. Am. Chem. Soc., 2005, 127, 13154 CrossRef CAS PubMed.
- For selected reviews, see:
(a) S. Rej, Y. Ano and N. Chatani, Bidentate directing groups: An efficient tool in C–H bond functionalization chemistry for the expedient construction of C–C bonds, Chem. Rev., 2020, 120, 1788 CrossRef CAS;
(b) J. P. Wan, Y. Li and Y. Liu, Annulation based on 8-aminoquinoline assisted C–H activation: an emerging tool in N-heterocycle construction, Org. Chem. Front., 2016, 3, 768 RSC.
- For recent works, see:
(a) W. Y. Shi, Y. N. Ding, C. Liu, N. Zheng, X. Y. Gou, M. Li, Z. Zhang, H. C. Liu, Z. J. Niu and Y. M. Liang, Three-component ruthenium-catalyzed remote C–H
functionalization of 8-aminoquinoline amides, Chem. Commun., 2020, 56, 12729 RSC;
(b) X. Lin, C. Zeng, C. Liu, Z. Fang and K. Guo, C-5 selective chlorination of 8-aminoquinoline amides using dichloromethane, Org. Biomol. Chem., 2021, 19, 1352 RSC;
(c) F. Zhan, W. Zhang and H. Zhao, Copper/silver cocatalyzed regioselective C5–H functionalization of 8-aminoquinoline amides with 1,3-dicarbonyl compounds, Synthesis, 2020, 52, 1007 CrossRef CAS. For selected review, see:
(d) Z. Xu, X. Yang, S. F. Yin and R. Qiu, Remote C−H functionalization of 8-aminoquinoline ring, Top. Curr. Chem., 2020, 378, 42 CrossRef CAS PubMed.
- For selected examples, see:
(a) Y. B. Kim, J. Won, J. Lee, J. Kim, B. Zhou, J. W. Park, M. H. Baik and S. Chang, Ni-catalyzed intermolecular C(sp3)–H amidation tuned by bidentate directing groups, ACS Catal., 2021, 11, 3067 CrossRef CAS;
(b) K. Sano, N. Kimura, T. Kochi and F. Kakiuchi, Palladium-catalyzed C–H iodination of N,-(8-quinolinyl)benzamide derivatives under electrochemical and non-electrochemical conditions, Asian J. Org. Chem., 2018, 7, 1311 CrossRef CAS;
(c) Y. Aihara and N. Chatani, Nickel-catalyzed reaction of C–H bonds in amides with I2: ortho-Iodination via the cleavage of C(sp2)–H bonds and oxidative cyclization to β-lactams via the cleavage of C(sp3)–H bonds, ACS Catal., 2016, 6, 4323 CrossRef CAS.
-
(a) C. J. Whiteoak, O. Planas, A. Company and X. Ribas, A first example of cobalt-catalyzed remote C–H functionalization of 8-aminoquinolines operating through a single electron transfer mechanism, Adv. Synth. Catal., 2016, 358, 1679 CrossRef CAS;
(b) B. Khan, A. A. Khan, D. Bora, D. Verma and D. Koley, Copper-catalyzed remote C–H nitration of 8-amidoquinolines, ChemistrySelect, 2017, 2, 260 CrossRef CAS;
(c) S. Mondal, S. Samanta and A. Hajra, Regioselective C-7 nitration of 8-aminoquinoline amides using tert-butyl nitrite, Adv. Synth. Catal., 2018, 360, 1026 CrossRef CAS.
-
(a) C. Sambiagio, D. Schonbauer, R. Blieck, T. Dao-Huy, G. Pototschnig, P. Schaaf, T. Wiesinger and M. F. Zia, A comprehensive overview of directing groups applied in metal-catalysed C–H functionalisation chemistry, Chem. Soc. Rev., 2018, 47, 6603 RSC;
(b) G. Rouquet and N. Chatani, Catalytic functionalization of C(sp2)–H and C(sp3)–H bonds by using bidentate directing groups, Angew. Chem., Int. Ed., 2013, 52, 11726 CrossRef CAS;
(c) D. D. Subhedar, A. A. Mishra and B. M. Bhanage,
N-Methoxybenzamide: A versatile directing group for palladium-, rhodium- and ruthenium-catalyzed C–H bond activations, Adv. Synth. Catal., 2019, 361, 4149 CrossRef CAS;
(d) R.-Y. Zhu, M. E. Farmer, Y. Q. Chen and J. Q. Yu, A simple and versatile amide directing group for C–H functionalizations, Angew. Chem., Int. Ed., 2016, 55, 10578 CrossRef CAS.
- For reviews of directing group removal, see:
(a) R. Rej and N. Chatani, Rhodium-catalyzed C(sp2)- or C(sp3)–H bond functionalization assisted by removable directing groups, Angew. Chem., Int. Ed., 2019, 58, 8304 CrossRef PubMed;
(b) L. S. Fitzgerald and M. L. O'Duill, A guide to directing group removal: 8-Aminoquinoline, Chem. – Eur. J., 2021, 27, 1 CrossRef PubMed.
- Y. Wu, C. Pi, Y. Wu and X. Cui, Directing group migration strategy in transition-metal-catalysed direct C–H functionalization, Chem. Soc. Rev., 2021, 50, 3677 RSC.
- X. F. Cheng, Y. Li, Y. M. Su, F. Yin, J. Y. Wang, J. Sheng, H. U. Vora, X. S. Wang and J. Q. Yu, Pd(II)-Catalyzed enantioselective C–H activation/C–O bond formation: Synthesis of chiral benzofuranones, J. Am. Chem. Soc., 2013, 135, 1236–1239 CrossRef CAS.
- L. Grigorjeva and O. Daugulis, Cobalt-catalyzed, aminoquinoline-directed coupling of sp2 C−H bonds with alkenes, Org. Lett., 2014, 16, 4684 CrossRef CAS.
- D. Tu, J. Luo and C. Jiang, Copper-mediated domino C–H iodination and nitration of indoles, Chem. Commun., 2018, 54, 2514 RSC.
-
(a) M. R. Yadav, M. Nagaoka, M. Kashihara, R.-L. Zhong, T. Miyazaki, S. Sakaki and Y. Nakao, The Suzuki–Miyaura coupling of nitroarenes, J. Am. Chem. Soc., 2017, 139, 9423 CrossRef CAS;
(b) S. S. Ng, Z. Chen, O. Y. Yuen and C. M. So, An indole-amide-based phosphine ligand enabling a general palladium-catalyzed sterically hindered Suzuki–Miyaura cross-coupling reaction, Org. Biomol. Chem., 2022, 20, 1373 RSC.
|
This journal is © the Partner Organisations 2023 |
Click here to see how this site uses Cookies. View our privacy policy here.