DOI:
10.1039/D2QO01585D
(Research Article)
Org. Chem. Front., 2023,
10, 74-82
Silver-catalysed [3 + 2] annulation reaction of aryldiazonium salts with allenes enabled by boronate direction†
Received
7th October 2022
, Accepted 8th November 2022
First published on 9th November 2022
Abstract
Allenes are a unique type of powerful synthon used for the construction of various carbocycles and heterocycles, but their annulation reactions with N–N triple bond electrophiles have not been disclosed. Here we report an efficient silver-catalysed boronate-directed [3 + 2] annulation reaction of aryl diazonium salts with allenylboronates. This transformation offers unprecedented access to a wide scope of N1-aryl-1H-pyrazoles with high regioselectivities under mild conditions. Preliminary experimental and computational studies support a transmetalation/stepwise cycloaddition/pyrazolyl silver hydrolysis pathway involving propargyl silver species as the key intermediate in enabling reactivity and controlling regioselectivity.
Introduction
Allenes are a powerful class of reactive synthons with a unique cumulative double bond structure.1 Allenes, especially allenoates, have been widely explored to react with carbon–carbon double bonds, carbon–nitrogen double bonds, carbon–oxygen double bonds, and carbon–carbon triple bonds to synthesize cyclopentenes, dihydropyrroles, dihydrofurans, four-membered carbocycles, and others.2 However, the reaction of allenes with nitrogen–nitrogen unsaturated bonds is still in its infancy.3 Notably, allenylboronates could exhibit umpolung nucleophilic reactivity compared with their electrophilic parent counterparts, and have thus emerged as a novel type of useful reagent over the past decade.4 For example, the propargylation and allenylation reactions of aldehydes, ketones, imines, and activated alkenes have been extensively studied with allenylboronates for the construction of various homopropargylic and allenylic alcohols, amines, and so on (Scheme 1a).5 In sharp contrast, the annulation reaction of allenylboronates remains largely underexploited. To the best of our knowledge, the only example of using allenylboronates as a C3-synthon to generate carbocycles was reported by Jarvo under palladium catalysis conditions in a cascade 1,4-nucleophilic addition/carbopalladation sequence with conjugate acceptors (Scheme 1b).6 Therefore, the method of engaging allenylboronates as all-carbon formal 1,3-dipoles with dipolarophiles to synthesize heterocycles is still under-developed.
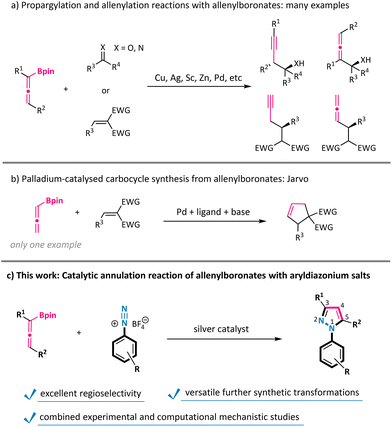 |
| Scheme 1 Reaction development of allenylboronates. | |
N
1-Aryl-1H-pyrazoles have found an increasing number of applications in pharmaceuticals, agrochemicals, and related biologically active molecules.7 Generally, the construction of N1-aryl-1H-pyrazoles is achieved by Knorr-type condensation/cyclization transformations of various arylhydrazines with the corresponding 1,3-dicarbonyl compounds.8 However, these processes inherently deliver regioisomeric mixtures with respect to substituents incorporated at the 3- and 5-positions of the pyrazole ring. In addition, arylhydrazine substrates are often produced by troublesome reduction of aryldiazonium salts.9 Therefore, the development of new protocols for the regioselective synthesis of N-aryl pyrazoles directly from readily accessible and abundant aryldiazonium salts is still highly desirable.10 We have recently disclosed silver-mediated cycloaddition reactions of trifluorodiazoethane with nitroolefins or alkynes for the synthesis of 4- or 5-substituted 3-trifluoromethylpyrazoles.11 In the present study, we envisioned that the combination of allenylboronates with a silver catalyst could be capable of generating all-carbon formal 1,3-dipoles, thus holding promise for the subsequent annulation with aryldiazonium salts.12 The realization of this transformation would further expand the utility of both aryldiazonium salts and allenes in heterocycle synthesis.13 Herein, we documented that N1-aryl-1H-pyrazoles were constructed by the direct exploitation of a variety of aryldiazonium salts in the silver-catalysed [3 + 2] annulation reaction of allenylboronates (Scheme 1c). In this context, the boronate moiety could function as a traceless activating and directing group to facilitate regioselective allene incorporation, as supported by combined experimental and computational mechanistic studies.
Results and discussion
Reaction optimization
Our study commenced by employing allenylboronic acid pinacol ester 1a and aryl diazonium salt 2a as the model substrates. In the presence of 20 mol% of silver oxide and 1 equivalent of potassium hydrogen carbonate, the annulation process could occur smoothly in THF at 40 °C in only 20 minutes, giving the corresponding N-aryl pyrazole 3a in 86% yield (Table 1, entry 1). Other silver salts could also catalyse this transformation, albeit with variable reaction efficiencies (entries 2–6). Notably, gold, copper, palladium, and zinc all proved to be invalid catalysts for promoting the formation of the desired pyrazole products (entries 7–10). Further screening of the base, solvent, amount of silver catalyst, and reaction temperature led to no obvious enhancement of the reaction outcome (entries 11–20). Allenylboronate 1a could also be added in one portion at the expense of a compromised reaction yield (entry 21). Moreover, a one-pot protocol using 4-bromoaniline directly to undergo the diazotization/[3 + 2] annulation process was also attempted, but the desired pyrazole product 3a was not obtained.
Table 1 Optimized reaction conditions and variationsa
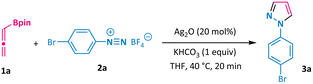
|
Entry |
Variation from the standard conditions |
Yield of 3a b |
General reaction procedure: to a mixture of phenyl diazonium salt 2a (81 mg, 0.3 mmol), a catalyst (0.04 mmol) and a base (0.2 mmol) in 1 mL of a solvent was added allenylboronate 1a (33 mg, 0.2 mmol) in 1 mL of a solvent slowly in 3 minutes, and the mixture reacted at 40 °C for 20 minutes unless otherwise noted.
Yield of the isolated pyrazole 3a. Safety note: handling of aryl diazonium salts should be carried out in a well-ventilated fume cupboard. “nd” means “not detected”.
|
1 |
None |
86 |
2 |
Ag2CO3 instead of Ag2O |
56 |
3 |
AgOAc instead of Ag2O |
64 |
4 |
AgF instead of Ag2O |
41 |
5 |
AgPF6 instead of Ag2O |
14 |
6 |
AgBF4 instead of Ag2O |
58 |
7 |
PPh3AuOTf instead of Ag2O |
Trace |
8 |
Cu(OTf)2 instead of Ag2O |
nd |
9 |
PdCl2(MeCN)2 instead of Ag2O |
Trace |
10 |
Zn(OAc)2 instead of Ag2O |
nd |
11 |
K2CO3 instead of KHCO3 |
55 |
12 |
KOAc instead of KHCO3 |
68 |
13 |
NaHCO3 instead of KHCO3 |
63 |
14 |
DIPEA instead of KHCO3 |
Trace |
15 |
DBU instead of KHCO3 |
Trace |
16 |
1,4-Dioxane instead of THF |
27 |
17 |
DMF instead of THF |
58 |
18 |
10 mol% of Ag2O was employed |
69 |
19 |
50 mol% of Ag2O was employed |
78 |
20 |
25 °C instead of 40 °C |
43 |
21 |
1a was added in one portion |
50 |
Substrate scope
The substrate scope of this silver-catalysed annulation reaction is quite general in terms of aryldiazonium salts 2 (Scheme 2). A broad range of substitution groups, such as alkyl, alkoxyl, and halogens, located at different positions on the phenyl ring, were all well accommodated and afforded pyrazoles 3c–3m in moderate to good yields. The presence of electron-withdrawing substituents in aryl diazonium salts was found to be beneficial for this reaction, as exemplified by trifluoromethyl (–CF3), pentafluorosulfanyl (–SF5), nitro (–NO2), cyano (–CN), and ester (–CO2Me) substituents (products 3n–3t with up to 95% isolated yield). Among them, the scalability of this method was validated via the 10 mmol scale reactions of compounds 3j and 3r (see the ESI†). The presence of a labile boronate moiety in the aryl diazonium salt proved to be no problem, and the B(pin)-containing pyrazole 3v was obtained, which leaves a good reactive site for further derivatizations. Furthermore, this reaction could also be extended to aryl diazonium salts possessing naphthyl and heteroaryl groups (products 3w and 3x). More importantly, aryl diazonium salts derived from several biologically relevant molecules are also well compatible in this annulation reaction, offering the corresponding pyrazole-decorated phenylalanine (3y), aminoglutethimide (3z), pomalidomide (3a′), and the lapatinib fragment (3b′).
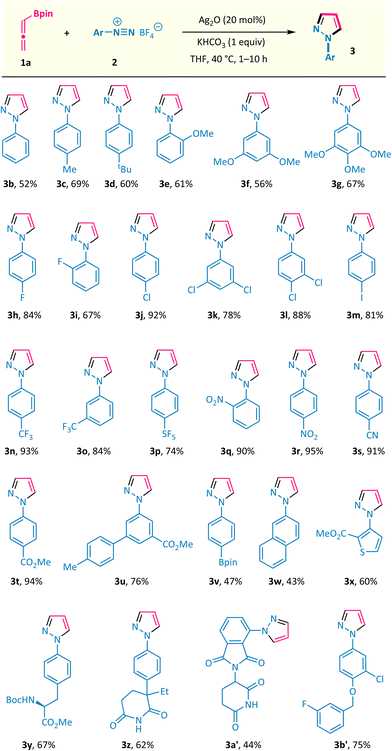 |
| Scheme 2 Substrate scope of aryldiazonium salts in the annulation reaction with allenylboronate 1a. | |
Subsequently, the scope of allenylboronates 1 was probed with aryl diazonium salt 2a under the optimal reaction conditions (Scheme 3).14 α,α-Disubstituted allenylboronates participated in the annulation reaction smoothly and gave the 3-alkyl-N1-aryl-pyrazoles 3ba and 3ca in practical yields with exclusive regioselectivity. More significantly, a series of 3,5-disubstituted-N1-aryl-pyrazoles 3da–3ja were readily obtained from the corresponding trisubstituted allenylboronates and diazonium salt 2a under the standard conditions. Among them, the substituents at the γ position of allenylboronates (R2) could be changed from alkyl groups to aromatic moieties possessing different functionalities (alkyl, trifluoromethyl, and chlorine). It is worth noting that a single regioisomer was characterized in reactions performed with these substrates, whatever the nature of the substituents of the allenylboronate. The structure of compound 3ha was ascertained by X-ray crystallographic analysis.
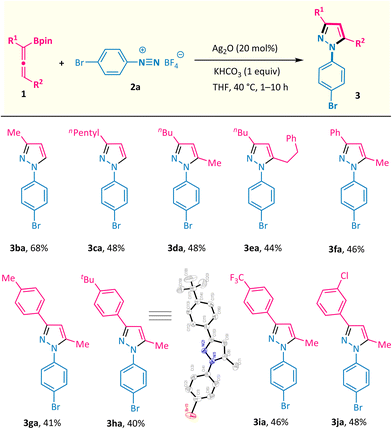 |
| Scheme 3 Substrate scope of allenylboronates in the annulation reaction with the aryldiazonium salt. | |
Synthetic utility
Furthermore, direct electrophilic chlorination of the obtained pyrazoles 3a and 3ba was readily attainable by using trichloroisocyanuric acid (TCICA) as the chlorinating reagent, thus giving 4-Cl-N1-aryl-pyrazoles 4a and 4b in 95% yields (Scheme 4a). We also performed bromination and iodination of the obtained pyrazoles with N-bromo-succinimide (NBS) and N-iodo-succinimide (NIS) and obtained the corresponding 4-Br-N1-aryl-pyrazoles 4c–4e and 4-I-N1-aryl-pyrazoles 4f and 4g in good to high yields (Scheme 4a). Electrophilic fluorination of pyrazole 3b was realized by using Selectfluor in nitromethane to give 4-F-N1-phenyl-pyrazole 5a in a moderate yield (Scheme 4b).15 In a similar vein, the introduction of a formyl group and a nitro group at the 4-position of the pyrazole ring was readily possible with urotropin and nitric acid, respectively (products 5b and 5c in Scheme 4b). Alternatively, treating pyrazole 3b with butyl lithium and 1-bromo-3-methylbut-2-ene allowed the formation of the alkylation product 5d in 68% yield with exclusive 5-position regioselectivity. Further derivatization on the aryl ring of the annulation products was also viable, as shown by the C–H alkylation of 3a and 3j with a diazo reagent under rhodium catalysis conditions, leading to the formation of malonate derivatives 6a and 6b in good yields with excellent regioselectivity (Scheme 4c).16 Importantly, pyrazole 3c′ was obtained in 84% yield under the standard reaction conditions (Scheme 4d). Zinc-promoted reduction of the nitro group in 3c′ gave the corresponding aryl primary amine 7, which could serve as a key intermediate for the synthesis of the phosphodiesterase 10A (PDE10A) inhibitor.17 This newly developed transformation was also smoothly applied in the synthesis of the gout drug Niraxostat intermediate 8 in a good overall yield from the readily available diazonium salt and allenylboronate 1a through a sequence of annulation and bromination with excellent regioselectivity in each step (Scheme 4e).18
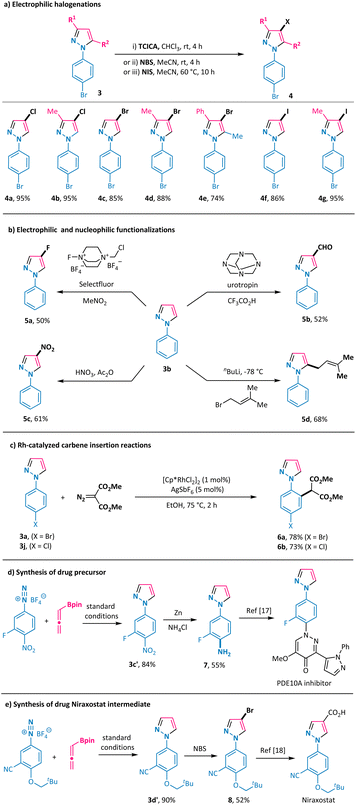 |
| Scheme 4 Synthetic transformation and applications. | |
Mechanistic studies
To shed some light on the reaction mechanism, a series of control experiments were performed (Scheme 5). First, the use of simple allene 9a or its propargyl counterpart 9b under otherwise model reaction conditions with phenyl diazonium salt 2a did not furnish the desired pyrazole, implying the crucial role of the boronate moiety (Scheme 5a). Notably, propargyl boronates 10a and 10b proved to be suitable partners in the annulation reaction with 2a under the standard conditions and resulted in the formation of 3-substituted N1-aryl-pyrazoles 3ka and 3la with identical regioselectivity (Scheme 5b, compound 3la has been confirmed by X-ray analysis). These results support the generation of a common intermediate from either allenylic or propargylic starting materials that are involved in the mechanistic pathway. Furthermore, we conducted the model reaction in a solvent mixture consisting of deuterated water and tetrahydrofuran (Scheme 5c). In this scenario, the deuterium atom was exclusively incorporated at the 4-position of the pyrazole ring (D-3a), which is clearly indicative of a protonation event occurring on an organosilver intermediate at this 4-position.
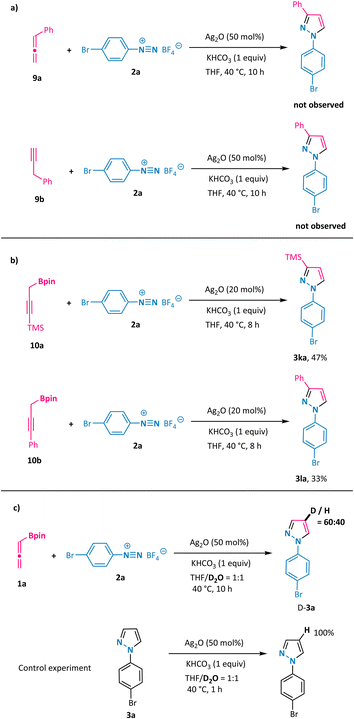 |
| Scheme 5 Control experiments for mechanistic studies. | |
Subsequently, the reaction mechanism was further explored via DFT calculations (Scheme 6).19 The computational results show that allenylboronate could undergo transmetalation smoothly with silver oxide via a six-membered cyclic transition state TS2 to give the propargyl silver intermediate Int3 with a barrier of 9.7 kcal mol−1. The reaction of the propargyl silver species with aryl diazonium was predicted to be a stepwise process consisting of nucleophilic addition to produce η2-coordinated silver complex Int4 and intramolecular cyclization to form Int5, with an overall barrier of only 6.7 kcal mol−1. The direct protonolysis of Int5viaTS5′ was found to be unfavorable. Base-promoted deprotonation was proved to be a more operative path, leading to a relatively stable pyrazolyl silver intermediate Int6, which is consistent with the experimental observation that 4-deuterated product D-3a was obtained (Scheme 5c). The final protonolysis of this organosilver species would generate the desired pyrazole product 3a. Based on the DFT-computed free energy profile, this reaction is an exergonic process (106.2 kcal mol−1) and the protonolysis of pyrazolyl silver is found to be the rate-determining step (26.1 kcal mol−1). Importantly, we have probed into the regioselectivity-determining step (TS3) for α-methyl-substituted allenylboronate 1b, which exclusively led to N1-aryl-3-methyl pyrazole 3ba. As illustrated in Scheme 6b, TS3-1 is more favorable than TS3-1′ by 4.5 kcal mol−1, owing to the increased steric hindrance in the latter case, which is in line with the excellent regioselectivity observed for 3ba. On the basis of these results and previous studies,20 a plausible mechanism is outlined in Scheme 6c. Transmetalation of allenylboronate 1 with a silver catalyst provides a propargylic silver species Int-3. Subsequent [3 + 2] cycloaddition of Int-3 with aryl diazonium salt 2 proceeds in a step-wise manner to form cyclic intermediate Int-5, which could undergo deprotonation to generate a more stable pyrazole-4-silver complex Int-6. Finally, protonolysis releases the silver catalyst and provides the pyrazole product 3.
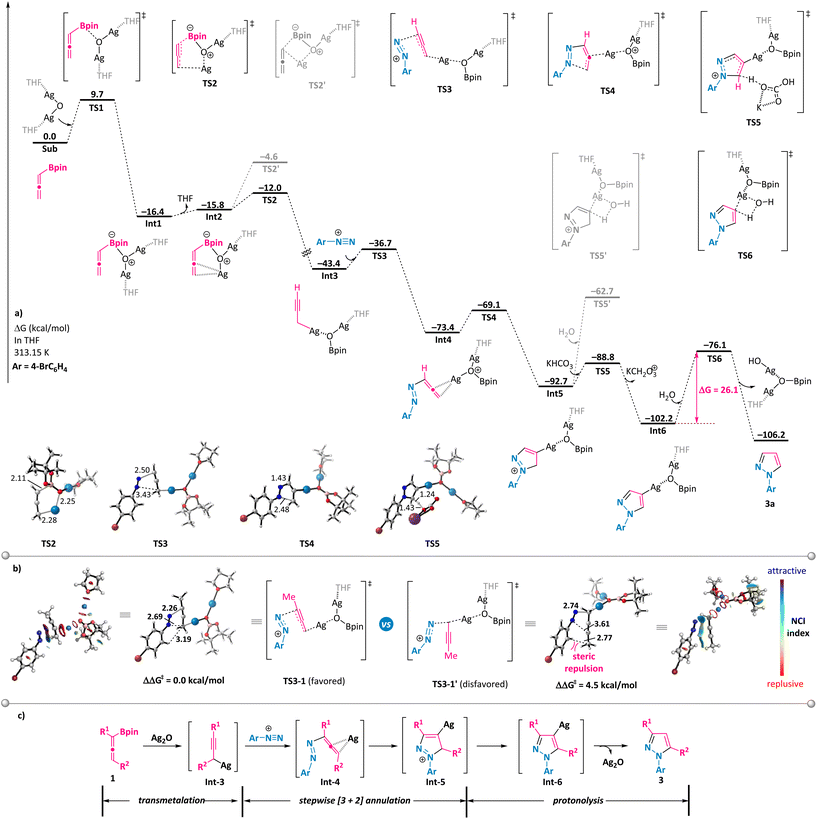 |
| Scheme 6 Computational mechanistic studies and the proposed mechanism. | |
Conclusions
In summary, we have developed a new silver-catalysed annulation reaction of aryldiazonium salts with allenes by taking advantage of a boronate motif as a traceless activating and directing group. The mild reaction conditions, wide substrate scope, excellent regioselectivity, and decent scalability render this protocol highly useful for the synthesis of N1-aryl-1H-pyrazoles. Further application of this method in the synthesis of related drug intermediates illustrated the potential utility. The reaction mechanism has been explored via experimental and computational approaches, revealing the critical role of the propargyl silver intermediate. This study discloses the practicality of allenylboronates as all-carbon formal 1,3-dipoles for heterocycle synthesis, and future applications are currently underway in our laboratory.
Author contributions
X. P. and P. Q. carried out the experimental and data analysis work. M.-M. Z. and X.-S. X. conducted the DFT studies. F.-G. Z. and J.-A. M. conceived and directed the project. F.-G. Z. and J.-A. M. wrote the manuscript with input from all authors.
Conflicts of interest
There are no conflicts to declare.
Acknowledgements
This work was supported by the National Key Research and Development Program of China (2019YFA0905100) and the National Natural Science Foundation of China (no. 92156025, 21901181, 22122104, and 21961142015). We would like to thank Dr Guosheng Ding and Dr Xiaojuan Deng at the Analysis and Testing Center of Tianjin University for their assistance with NMR testing and analysis. This work is dedicated to the 60th anniversary of the Institute of Elemento-organic Chemistry at Nankai University.
References
-
(a) D. R. Taylor, The chemistry of allenes, Chem. Rev., 1967, 67, 317–359 CrossRef CAS;
(b)
N. Krause and A. S. K. Hashmi, Modern Allene Chemistry, Wiely-VCH, Weinheim, 2004 CrossRef.
-
(a) X. Lu, C. Zhang and Z. Xu, Reactions of electron-deficient alkynes and allenes under phosphine catalysis, Acc. Chem. Res., 2001, 34, 535–544 CrossRef CAS PubMed;
(b) S. Ma, Some typical advances in the synthetic applications of allenes, Chem. Rev., 2005, 105, 2829–2872 CrossRef PubMed;
(c) B. J. Cowen and S. J. Miller, Enantioselective catalysis and complexity generation from allenoates, Chem. Soc. Rev., 2009, 38, 3102–3116 RSC;
(d) B. Alcaide, P. Almendros and C. Aragoncillo, Exploiting [2+2] cycloaddition chemistry: achievements with allenes, Chem. Soc. Rev., 2010, 39, 783–816 RSC;
(e) S. Yu and S. Ma, Allenes in catalytic asymmetric synthesis and natural product syntheses, Angew. Chem., Int. Ed., 2012, 51, 3074–3112 CrossRef CAS;
(f) Z. Wang, X. Xu and O. Kwon, Phosphine catalysis of allenes with electrophiles, Chem. Soc. Rev., 2014, 43, 2927–2940 RSC;
(g) L.-J. Yang and J.-A. Ma, New advances on nucleophilic phosphine-triggered annulation reactions of allenoates, Acta Chim. Sin., 2016, 74, 130–148 CrossRef CAS;
(h) Y. Wei and M. Shi, Lu's [3+2] cycloaddition of allenes with electrophiles: discovery, development and synthetic application, Org. Chem. Front., 2017, 4, 1876–1890 RSC;
(i) H. Ni, W.-L. Chan and Y. Lu, Phosphine-catalyzed asymmetric organic reactions, Chem. Rev., 2018, 118, 9344–9411 CrossRef CAS;
(j) J. L. Mascareñas, I. Varela and F. López, Allenes and derivatives in gold(I)- and platinum(II)-catalyzed formal cycloadditions, Acc. Chem. Res., 2019, 52, 465–479 CrossRef PubMed;
(k) A. L. Cardoso and M. I. L. Soares, The chemistry of allenes, Curr. Org. Chem., 2019, 23, 3064–3134 CrossRef CAS;
(l) E.-Q. Li and Y. Huang, Recent advances in phosphine catalysis involving γ-substituted allenoates, Chem. Commun., 2020, 56, 680–694 RSC.
-
(a) V. Nair, A. T. Biju, K. Mohanan and E. Suresh, Novel synthesis of highly functionalized pyrazolines and pyrazoles by triphenylphosphine-mediated reaction of dialkyl azodicarboxylate with allenic esters, Org. Lett., 2006, 8, 2213–2216 CrossRef CAS PubMed;
(b) Q. Zhang, L.-G. Meng, J. Zhang and L. Wang, DMAP-catalyzed [2+4] cycloadditions of allenoates with N-Acyldiazenes: direct method to 1,3,4-Oxadiazine derivatives, Org. Lett., 2015, 17, 3272–3275 CrossRef CAS PubMed;
(c) S. Xu, J. Chen, J. Shang, Z. Qing, J. Zhang and Y. Tang, Divergent amine-catalyzed [2+2] annulation of allenoates with azodicarboxylates: facile synthesis of 1,2-diazetidines, Tetrahedron Lett., 2015, 56, 6456–6459 CrossRef CAS.
-
(a) H. M. Wisniewska and E. R. Jarvo, Enantioselective propargylation and allenylation reactions of ketones and imines, J. Org. Chem., 2013, 78, 11629–11636 CrossRef CAS;
(b) S. Manna, K. K. Das, D. Aich and S. Panda, Synthesis and reactivity of allenylboron compounds, Adv. Synth. Catal., 2021, 363, 2444–2463 CrossRef CAS.
-
(a) R. Haruta, M. Ishiguro, N. Ikeda and H. Yamamoto, Chiral allenylboronic esters: a practical reagent for enantioselective carbon-carbon bond formation, J. Am. Chem. Soc., 1982, 104, 7667–7669 CrossRef CAS;
(b) E. J. Corey, C.-M. Yu and D.-H. Lee, A practical and general enantioselective synthesis of chiral propa-1,2-dienyl and propargyl carbinols, J. Am. Chem. Soc., 1990, 112, 878–879 CrossRef CAS;
(c) M. L. Maddess and M. Lautens, Preparation of homoallylic homopropargylic alcohols from 2-Vinyloxiranes, Org. Lett., 2005, 7, 3557–3560 CrossRef CAS PubMed;
(d) S.-L. Shi, L.-W. Xu, K. Oisaki, M. Kanai and M. Shibasaki, Identification of modular chiral bisphosphines effective for Cu(I)-catalyzed asymmetric allylation and propargylation of ketones, J. Am. Chem. Soc., 2010, 132, 6638–6639 CrossRef CAS PubMed;
(e) H. M. Wisniewska and E. R. Jarvo, Enantioselective silver-catalyzed propargylation of imines, Chem. Sci., 2011, 2, 807–810 RSC;
(f) P. Jain, H. Wang, K. N. Houk and J. C. Antilla, Brønsted acid catalyzed asymmetric propargylation of aldehydes, Angew. Chem., Int. Ed., 2012, 51, 1391–1394 CrossRef CAS;
(g) M. Chen and W. R. Roush, Enantioselective synthesis of anti- and syn-homopropargyl alcohols via chiral brønsted acid catalyzed asymmetric allenylboration reactions, J. Am. Chem. Soc., 2012, 134, 10947–10952 CrossRef CAS PubMed;
(h) B. L. Kohn, N. Ichiishi and E. R. Jarvo, Silver-catalyzed allenylation and enantioselective propargylation reactions of ketones, Angew. Chem., Int. Ed., 2013, 52, 4414–4417 CrossRef CAS;
(i) H. Wu, F. Haeffner and A. H. Hoveyda, An efficient, practical, and enantioselective method for synthesis of homoallenylamides catalyzed by an aminoalcohol-derived, boron-based catalyst, J. Am. Chem. Soc., 2014, 136, 3780–3783 CrossRef CAS PubMed;
(j) F. Meng, X. Li, S. Torker, Y. Shi, X. Shen and A. H. Hoveyda, Catalytic enantioselective 1,6-conjugate additions of propargyl and allyl groups, Nature, 2016, 537, 387–393 CrossRef CAS PubMed;
(k) K. A. Lee, D. L. Silverio, S. Torker, D. W. Robbins, F. Haeffner, F. W. van der Mei and A. H. Hoveyda, Catalytic enantioselective addition of organoboron reagents to fluoroketones controlled by electrostatic interactions, Nat. Chem., 2016, 8, 768–777 CrossRef CAS;
(l) Y. Zhou, Y. Shi, S. Torker and A. H. Hoveyda, SN2′′-selective and enantioselective substitution with unsaturated organoboron compounds and catalyzed by a sulfonate-containing NHC-Cu complex, J. Am. Chem. Soc., 2018, 140, 16842–16854 CrossRef CAS;
(m) Q. Chong, S. Zhang, F. Cheng, J. Wang, X. Hong and F. Meng, N-heterocyclic carbene–Cu-catalyzed enantioselective allenyl conjugate addition, Org. Lett., 2018, 20, 6896–6900 CrossRef CAS PubMed.
- B. L. Kohn and E. R. Jarvo, Palladium-catalyzed annulation reactions for diastereoselective cyclopentene synthesis, Org. Lett., 2011, 13, 4858–4861 CrossRef CAS.
-
(a) S. Fustero, M. Sánchez-Roselló, P. Barrio and A. Simón-Fuentes, From 2000 to Mid-2010: A fruitful decade for the synthesis of pyrazoles, Chem. Rev., 2011, 111, 6984–7034 CrossRef CAS PubMed;
(b) P. K. Mykhailiuk, Fluorinated pyrazoles: From synthesis to applications, Chem. Rev., 2021, 121, 1670–1715 CrossRef CAS PubMed;
(c) J. Regan, S. Breitfelder, P. Cirillo, T. Gilmore, A. G. Graham, E. Hickey, B. Klaus, J. Madwed, M. Moriak, N. Moss, C. Pargellis, S. Pav, A. Proto, A. Swinamer, L. Tong and C. Torcellini, Pyrazole urea-based inhibitors of p38 MAP kinase: From lead compound to clinical candidate, J. Med. Chem., 2002, 45, 2994–3008 CrossRef CAS PubMed;
(d) G. R. Bebernitz, G. Argentieri, B. Battle, C. Brennan, B. Balkan, B. F. Burkey, M. Eckhardt, J. Gao, P. Kapa, R. J. Strohschein, H. F. Schuster, M. Wilson and D. D. Xu, The effect of 1,3-Diaryl-[1H]-pyrazole-4-acetamides on glucose utilization in ob/ob mice, J. Med. Chem., 2001, 44, 2601–2611 CrossRef CAS;
(e) C. Pargellis, L. Tong, L. Churchill, P. F. Cirillo, T. Gilmore, A. G. Graham, P. M. Grob, E. R. Hickey, N. Moss, S. Pav and J. Regan, Inhibition of p38 MAP
kinase by utilizing a novel allosteric binding site, Nat. Struct. Biol., 2002, 9, 268–272 CrossRef CAS PubMed;
(f) G. Dannhardt and W. Kiefer, Cyclooxygenase inhibitors–current status and future prospects, Eur. J. Med. Chem., 2001, 36, 109–126 CrossRef CAS PubMed;
(g) D. Hainzl and J. E. Casida, Fipronil insecticide: Novel photochemical desulfinylation with retention of neurotoxicity, Proc. Natl. Acad. Sci. U. S. A., 1996, 93, 12764–12767 CrossRef CAS PubMed.
-
(a) L. Knorr, Einwirkung von acetessigester auf phenylhydrazin, Ber. Dtsch. Chem. Ges., 1883, 16, 2597–2599 CrossRef;
(b) A. Schmidt and A. Dreger, Recent advances in the chemistry of pyrazoles. Properties, biological activities, and syntheses, Curr. Org. Chem., 2011, 15, 1423–1463 CrossRef CAS;
(c) A. W. Brown, Chapter Two - Recent Developments in the Chemistry of Pyrazoles, Adv. Heterocycl. Chem., 2018, 126, 55–107 CrossRef CAS;
(d) X. Li, Y. Yu and Z. Tu, Pyrazole Scaffold Synthesis, Functionalization, and Applications in Alzheimer's Disease and Parkinson's Disease Treatment (2011–2020), Molecules, 2021, 26, 1202 CrossRef CAS PubMed;
(e) M. Konwar, R. Hazarika and D. Sarma, Synthetic advances in C(sp2)-H/N–H arylation of pyrazole derivatives through activation/substitution, Tetrahedron, 2021, 102, 132504 CrossRef CAS.
-
(a) F. G. James, M. J. Perkins, O. Porta and B. V. Smith, (η4-Cyclo-octa-1,4-diene)(η6-cyclo-octa-1,3,5-triene)ruthenium(0) chemistry: the role of molecular hydrogen in a new synthetic route to cyclo-olefin ruthenium complexes, J. Chem. Soc., Chem. Commun., 1977, 131–132 RSC;
(b) B. P. Bandgar and C. S. Thite, Selective reduction of aryl diazonium fluoroborates, Synth. Commun., 1997, 27, 635–639 CrossRef CAS;
(c) F. Mo, G. Dong, Y. Zhang and J. Wang, Recent applications of arene diazonium salts in organic synthesis, Org. Biomol. Chem., 2013, 11, 1582–1593 RSC;
(d) F. Mo, D. Qiu, L. Zhang and J. Wang, Recent development of aryl diazonium chemistry for the derivatization of aromatic compounds, Chem. Rev., 2021, 121, 5741–5829 CrossRef CAS PubMed.
-
(a) F.-G. Zhang, Z. Chen, C. W. Cheung and J.-A. Ma, Aryl diazonium salt-triggered cyclization and cycloaddition reactions: past, present, and future, Chin. J. Chem., 2020, 38, 1132–1152 CrossRef CAS;
(b) F.-G. Zhang, Z. Chen, X. Tang and J.-A. Ma, Triazines: syntheses and inverse electron-demand Diels–Alder reactions, Chem. Rev., 2021, 121, 14555–14593 CrossRef CAS PubMed;
(c) K. Matcha and A. P. Antonchick, Cascade multicomponent synthesis of indoles, pyrazoles, and pyridazinones by Functionalization of alkenes, Angew. Chem., Int. Ed., 2014, 53, 11960–11964 CrossRef CAS PubMed;
(d) Y. Shao, H. Zheng, J. Qian and X. Wan, In situ generation of nitrilimines from aryldiazonium salts and diazo esters: Synthesis of fully substituted pyrazoles under room Temperature, Org. Lett., 2018, 20, 2412–2415 CrossRef CAS;
(e) M. Wang, B.-C. Tang, J.-C. Xiang, X.-L. Chen, J.-T. Ma, Y.-D. Wu and A.-X. Wu, Aryldiazonium salts serve as a dual synthon: Construction of fully substituted pyrazoles via rongalite-mediated three-component radical annulation reaction, Org. Lett., 2019, 21, 8934–8937 CrossRef CAS PubMed;
(f) J. Liu, E. Xu, J. Jiang, Z. Huang, L. Zheng and Z.-Q. Liu, Copper-mediated tandem ring-opening/cyclization reactions of cyclopropanols with aryldiazonium salts: synthesis of N-arylpyrazoles, Chem. Commun., 2020, 56, 2202–2205 RSC;
(g) L. Cardinale, M. Neumeier, M. Majek and A. J. von Wangelin, Aryl pyrazoles from photocatalytic cycloadditions of arenediazonium, Org. Lett., 2020, 22, 7219–7224 CrossRef CAS.
-
(a) F. Li, J. Nie, L. Sun, Y. Zheng and J.-A. Ma, Silver-Mediated cycloaddition of alkynes with CF3CHN2: Highly regioselective synthesis of 3-trifluoromethylpyrazoles, Angew. Chem., Int. Ed., 2013, 52, 6255–6258 CrossRef CAS;
(b) Z. Chen, Y. Zheng and J.-A. Ma, Use of a traceless activating and directing group for the construction of trifluoromethylpyrazoles: One-Pot transformation of nitroolefins and trifluorodiazoethane, Angew. Chem., Int. Ed., 2017, 56, 4569–4574 CrossRef CAS;
(c) C.-F. Gao, Y. Zhou, H. Ma, Y. Zhang, J. Nie, F.-G. Zhang and J.-A. Ma, Dual incorporation of trifluoromethyl and cyano groups into pyrazole pharmcophores via silver-catalyzed cycloaddition reaction of trifluorodiazoethane, CCS Chem., 2022 DOI:10.31635/ccschem.022.202201923.
-
(a) Z. Zhang, Q. Zhang, Z. Ni and Q. Liu, Ag-containing all-carbon 1,3-dipoles: generation and formal cycloaddition for furo[3,2-b]-β/γ-lactams, Chem. Commun., 2010, 46, 1269–1271 RSC;
(b) Z. Zhang, S. Fang, Q. Liu and G. Zhang, Microwave-assisted facile synthesis of furo[3,2-b]indole derivatives via silver(I)-mediated [3+2] cycloaddition, Adv. Synth. Catal., 2012, 354, 927–932 CrossRef CAS;
(c) B. Yu, A. Perfetto, L. Allievi, S. Dhambri, M.-N. Rager, M. Selkti, I. Ciofini, M.-I. Lannou and G. Sorin, Silver(I) oxide-/DBU-promoted synthesis of dihydrofuran units through allenyl silver formation, Chem. – Eur. J., 2020, 26, 17455–17461 CrossRef CAS;
(d) B. Yu, M. Selkti, J. Ardisson, M.-I. Lannou and G. Sorin, Access to conjugated enynes via allenyl silver formation/cyclization/decarboxylation reaction catalyzed by silver carbonate(I), Org. Lett., 2022, 24, 5721–5725 CrossRef CAS;
(e) B. Yu, S. Mohamed, J. Ardisson, M.-I. Lannou and G. Sorin, Silver oxide(i) promoted conia-ene/radical cyclization for a straightforward access to furan derivatives, Chem. Commun., 2022, 58, 1374–1377 RSC.
-
(a) B. Chen, C. Zhu, Y. Tang and S. Ma, Copper-mediated pyrazole synthesis from 2,3-allenoates or 2-alkynoates, amines and nitriles, Chem. Commun., 2014, 50, 7677–7679 RSC;
(b) F.-G. Zhang, Y. Wei, Y.-P. Yi, J. Nie and J.-A. Ma, Regioselective cycloaddition of trifluorodiazoethane with electron-deficient allenic esters and ketones: Access to CF3-substituted pyrazolines and pyrazoles, Org. Lett., 2014, 16, 3122–3125 CrossRef CAS;
(c) D. Sarkar and S. R. Sahoo, Monohydrochloride assisted synthesis of functionalized isoxazoles and pyrazoles from allenic Ketones: First synthesis of (Z,)-2-methyl-7H-benzo[b]pyrazolo[5,1-d][1,5]oxazocines, Eur. J. Org. Chem., 2019, 2035–2049 CrossRef CAS;
(d) Y.-T. Tian, F.-G. Zhang and J.-A. Ma, Et3N-catalyzed direct cycloaddition reaction of allenoates with acceptor diazo compounds, Tetrahedron, 2021, 81, 131922 CrossRef CAS;
(e) Y.-T. Tian, F.-G. Zhang and J.-A. Ma, Regioselective [3+2] cycloaddition reaction of 3-alkynoates with Seyferth–Gilbert reagent, J. Org. Chem., 2021, 86, 3574–3582 CrossRef CAS PubMed.
-
(a) H. Ito, Y. Sasaki and M. Sawamura, Copper(I)-catalyzed substitution of propargylic carbonates with diboron: selective synthesis of multisubstituted allenylboronates, J. Am. Chem. Soc., 2008, 130, 15774–15775 CrossRef CAS PubMed;
(b) T. S. N. Zhao, Y. Yang, T. Lessing and K. J. Szabó, Borylation of propargylic substrates by bimetallic catalysis. synthesis of allenyl, propargylic, and butadienyl Bpin derivatives, J. Am. Chem. Soc., 2014, 136, 7563–7566 CrossRef CAS PubMed;
(c) H. L. Sang, S. Yu and S. Ge, Copper-catalyzed asymmetric hydroboration of 1,3-enynes with pinacolborane to access chiral allenylboronates, Org. Chem. Front., 2018, 5, 1284–1287 RSC;
(d) H. Miura, Y. Hachiya, H. Nishio, Y. Fukuta, T. Toyomasu, K. Kobayashi, Y. Masaki and T. Shishido, Practical synthesis of allyl, allenyl, and benzyl boronates through SN1′-Type borylation under heterogeneous gold catalysis, ACS Catal., 2021, 11, 758–766 CrossRef CAS.
- W. Wang, T. Huo, X. Zhao, Q. Qin, Y. Liang, S. Song, G. Liu and N. Jiao, Nitromethane-enabled fluorination of styrenes and arenes, CCS Chem., 2020, 2, 566–575 CrossRef CAS.
- X. Yu, S. Yu, J. Xiao, B. Wan and X. Li, Rhodium(III)-catalyzed azacycle-directed intermolecular insertion of arene C–H bonds into α-diazocarbonyl compounds, J. Org. Chem., 2013, 78, 5444–5452 CrossRef CAS PubMed.
-
(a) J. Kunitomo, M. Yoshikawa, M. Fushimi, A. Kawada, J. F. Quinn, H. Oki, H. Kokubo, M. Kondo, K. Nakashima, N. Kamiguchi, K. Suzuki, H. Kimura and T. Taniguchi, Discovery of 1-[2-Fluoro-4-(1H-pyrazol-1-yl)phenyl]-5-methoxy-3-(1-phenyl-1H-pyrazol-5-yl)pyridazin-4(1H)-one (TAK-063), a highly potent, selective, and orally active phosphodiesterase 10A (PDE1(0A) inhibitor, J. Med. Chem., 2014, 57, 9627–9643 CrossRef CAS PubMed;
(b) A. Suzuki, N. Fukuda, T. Kajiwara and T. Ikemoto, Practical preparation of a 1,3,5-Trisubstituted pyridazin-4(1H)-one using selective C1 unit insertion and cyclization, Org. Process Res. Dev., 2019, 23, 484–492 CrossRef CAS.
- S. Ishibuchi, H. Morimoto, T. Oe, T. Ikebe, H. Inoue, A. Fukunari, M. Kamezawa, I. Yamada and Y. Naka, Synthesis and structure–activity relationships of 1-Phenylpyrazoles as xanthine oxidase inhibitors, Biorg. Med. Chem. Lett., 2001, 11, 879–882 CrossRef CAS PubMed.
- DFT calculations were performed at the B3LYP-D3(BJ)/6-311+G(2d,p)+SDD(Ag) level of theory. Detailed references on the computational methods and all the optimized structures are provided in the ESI.†.
-
(a) J.-M. Weibel, A. Blanc and P. Pale, Ag-mediated reactions: Coupling and heterocyclization reactions, Chem. Rev., 2008, 108, 3149–3173 CrossRef CAS PubMed;
(b) M. P. Muñoz, Silver and platinum-catalysed addition of O–H and N–H bonds to allenes, Chem. Soc. Rev., 2014, 43, 3164–3183 RSC;
(c) G. Fang and X. Bi, Silver-catalysed reactions of alkynes: recent advances, Chem. Soc. Rev., 2015, 44, 8124–8173 RSC;
(d)
D. Lee and S. Ghorai, in Silver-catalyzed cycloaddition reactions in silver catalysis in organic synthesis, ed. C.-J. Li and X. Bi, Wiley-VCH, 2019, ch. 2, pp. 33–83 Search PubMed;
(e) X. Qi, H. Zhang, A. Shao, L. Zhu, T. Xu, M. Gao, C. Liu and Y. Lan, Silver migration facilitates Isocyanide-Alkyne [3+2] cycloaddition reactions: combined experimental and theoretical study, ACS Catal., 2015, 5, 6640–6647 CrossRef CAS;
(f) J. Zhu, A. C. Durham, Y. Wang, J. C. Corcoran, X.-D. Zuo, S. J. Geib and Y.-M. Wang, Regiocontrolled coupling of alkynes and dipolar reagents: Iron-mediated [3+2] cycloadditions revisited, Organometallics, 2021, 40, 2295–2304 CrossRef CAS.
|
This journal is © the Partner Organisations 2023 |
Click here to see how this site uses Cookies. View our privacy policy here.