DOI:
10.1039/D2QO01647H
(Research Article)
Org. Chem. Front., 2023,
10, 99-103
Copper-catalyzed dearomative 1,4-carboxylate rearrangement of 2-carbonateindoles†
Received
18th October 2022
, Accepted 11th November 2022
First published on 14th November 2022
Abstract
A novel dearomative 1,4-rearrangement reaction of 2-carbonateindoles with aryl diazoacetates has been developed in the presence of a copper catalyst, which might proceed through a tandem formation of a zwitterionic intermediate, intramolecular cyclization and ring-opening reaction to give the final rearrangement products containing an all-carbon quaternary center with two newly formed C–C bonds. In this sequence, the disruption of the aromaticity of indole has been realized, associated with 1,4-carboxylate rearrangements.
Introduction
Rearrangement reactions are one of the most important methods to increase molecular complexity in organic synthesis.1 In particular, the rearrangements involving onium ylides that originate from the decomposition of diazo compounds have attracted much attention, and versatile transformations have been developed to prepare structurally diverse and complicated compounds.2 Among them, [2,3]- and [1,2]-sigmatropic rearrangements remain the most common ones in the field of carbene-mediated rearrangement reactions,3,4 in which a metal-bound ylide or a free ylide is responsible for the subsequential sigmatropic rearrangements.5 Despite the great achievements that have been made,6 these reports mainly focused on the rearrangement of aliphatic systems. In sharp contrast, the dearomative rearrangement reactions are rare due to the difficulty in disrupting the aromaticity of arenes and heterocycles.7 Recently, we reported a rhodium-catalyzed dearomative 1,4-acyl rearrangement by the reaction of 2-oxypyridines and diazo compounds (Scheme 1b).8 A density functional theory calculation indicates the sequential formation of a metal-bound pyridinium ylide and a free ylide, which undergo intramolecular cyclization and ring-opening to give the final dearomative rearrangement products. Later, using triazoles, pyridotriazoles and cyclopropenes as the carbene precursors, such types of dearomative rearrangements have been developed.9 After that, we wanted to extend this rearrangement to heterocycles rather than 2-oxypyridines. Herein, we wish to report our endeavour to find a novel dearomative 1,4-rearrangement of indoles under copper catalysis. As shown in Scheme 1c, the reaction of 2-carbonateindole with a diazo compound in the presence of a copper salt might generate the metal-bound zwitterionic intermediate I, which may form the metal-free intermediate II with the release of the copper catalyst. Intramolecular cyclization either from I or II will give the zwitterionic intermediate III, which undergoes a ring-opening reaction to give the dearomative product with two newly formed C–C bonds.
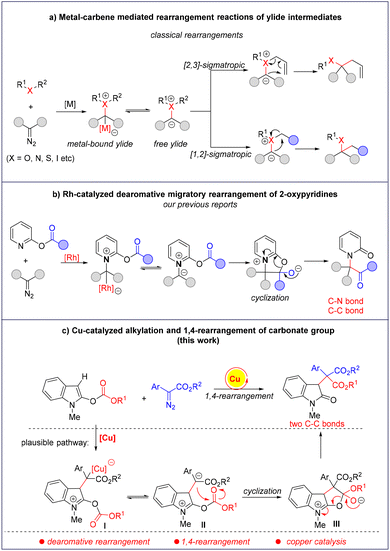 |
| Scheme 1 Previous reports and our strategy. | |
Results and discussion
We started our studies by using indole 1a and phenyl diazoacetate 2a as the model substrates in dichloromethane at room temperature to optimize the reaction conditions (Table 1). Unfortunately, the use of rhodium complexes led to unsatisfactory results. For example, when Rh2(OAc)4 was used as the catalyst, only 30% yield of 3aa was isolated in 30 min (entry 1) and Rh2(esp)2 gave a much lower yield (entry 2). Palladium catalysts, such as Pd(OAc)2, did not work at all (entry 3). Then a series of copper salts were screened. Gratifyingly, 65% yield of 3aa was obtained by using copper(I) thiophene-2-carboxylate (CuTc) as the catalyst, although with a longer reaction time (entry 4). In contrast, CuOTf·Tol1/2 and Cu(MeCN)4PF6 afforded 3a in 45% and 41% yields, respectively (entries 5 and 6). Next, a screening of solvents was conducted. It was found that chloroform, 1,2-dichloroethane, and toluene gave moderate yields of 3aa (entries 8–10). However, no 3aa was formed when acetonitrile was used (entry 11). Next, the influence of substrate 2a at different ratios was examined. Using 1.5 equiv. of 2a delivered 3aa in 69% yield (entry 12), which was further improved to 78% when 1.8 equiv. of 2a was used (entry 13). Next, on performing the reaction at 40 °C, the reaction could reach completion in 12 h with a yield of 77% (entry 14), and the reaction time could be reduced to 6 h at 60 °C (entry 15). As a result, we chose entry 15 as the best conditions for further investigation.
Table 1 Optimization of the reaction conditionsa
With the optimal reaction conditions in hand, we next explored the scope of diazoacetates in this dearomative 1,4-methyl carbonate rearrangement reaction (Scheme 2). para-Substituted electron-rich and electron-deficient phenyl diazoacetates all worked well in this reaction, providing the corresponding products (3ab–3ag) in 59–81% yields. Aryl diazoacetates containing a chloro or a methyl group on the benzene ring also reacted well, affording 3ah–3aj in 72–78% yields. In the case of sterically hindered 2-chloro phenyl diazoacetate, a moderate yield of the desired product was observed (3ak, 50%). The reaction of 2-naphthyl diazoacetate with 1a delivered 3al in 92% yield. The simple methyl 2-diazoacetate was tolerated, providing the corresponding product 3am in 66% yield. It should be noted that alkyl diazoacetates and donor–donor diazo compounds did not work in this reaction.
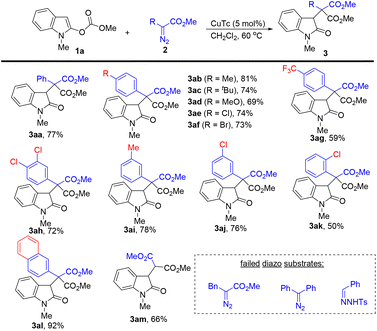 |
| Scheme 2 Substrate scope for diazo compounds. The reactions were carried out with 1a (0.2 mmol), 2 (0.36 mmol), and CuTc (5 mol%) in CH2Cl2 (4 mL) at 60 °C for 6 h. Isolated yields. | |
Next, the scope of indole substrates 1 was examined using phenyl diazoacetate 2a as the reaction partner (Scheme 3). In the case of indoles containing different N-substituents, the benzyl substituted indole gave the corresponding product 3ba in 68% yield, and the indole with the N-isopropyl group afforded 75% yield of 3ca. We next investigated the influence of substituents on the phenyl ring of the indoles. The use of the 4-bromo indole substrate led to 85% yield of 3da. Indoles bearing functional groups such as methyl, methoxy, fluoro, and bromo at the C5 position all worked well in this reaction, furnishing the corresponding products 3ea–3ha in 72–83% yields. The 6-bromo and 6-methoxy indole substrates also reacted well, providing 3ia and 3ja in 74% and 67% yields, respectively. Indoles containing a substituent at the C7 position were tolerated in this reaction, affording the desired products (3ka and 3la) in good yields. Different ester groups at the C2 position of the indole led to different results, for example, ethyl ester led to 76% yield of 3mn and isopropyl ester gave 3no in 53% yield. Typically, the reaction of N-methyl ethyl carbonate with 2a gave 3ma in 80% yield with 1
:
1 dr. The structure of 3ma (one isomer) was confirmed by X-ray diffraction.
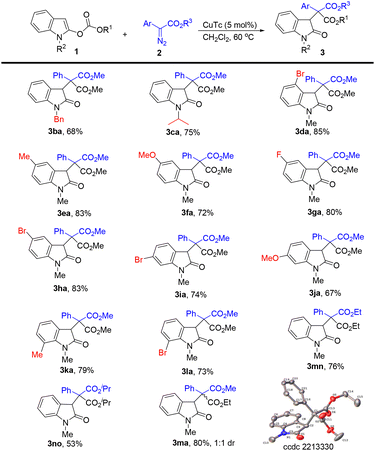 |
| Scheme 3 Substrate scope for indoles. The reactions were carried out with 1 (0.2 mmol), 2 (0.36 mmol), and CuTc (5 mol%) in CH2Cl2 (4 mL) at 60 °C for 6 h. Isolated yields. | |
Gram-scale reaction and further elaboration were then conducted (Scheme 4). The reaction of 4 mmol of 1a with 7.2 mmol of 2a afforded 1.03 g of 3aa (Scheme 4a). The Suzuki coupling reaction of 3ha with aryl boronic acid delivered 4 in 84% yield (Scheme 4b). The treatment of 3aa with iodomethane provided compound 5 bearing an all-carbon quaternary center in 87% yield, providing an effective approach for the construction of heterocycles containing two adjacent all-carbon quaternary centers (Scheme 4c).
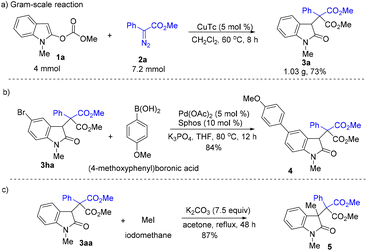 |
| Scheme 4 Gram-scale reaction and further exploration. | |
Conclusions
In summary, a novel rearrangement that originated from the reaction of 2-carbonateindoles with diazo compounds has been developed in the presence of a copper catalyst under mild reaction conditions. In this reaction, the disruption of aromaticity of indoles has been realized associated with the 1,4-rearrangement of a carboxylate group, affording indolin-2-ones containing an all-carbon quaternary center in acceptable yields.
Conflicts of interest
There are no conflicts to declare.
Acknowledgements
We are grateful to the National Natural Science Foundation of China (21971026 and 22171028) and the Jiangsu Key Laboratory of Advanced Catalytic Materials and Technology (BM2012110) for financial support. The Analysis and Testing Center, NERC Biomass of Changzhou University is also acknowledged.
Notes and references
-
(a) E. A. Ilardi, C. E. Stivala and A. Zakarian, [3,3]-Sigmatropic rearrangements: recent applications in the total synthesis of natural products, Chem. Soc. Rev., 2009, 38, 3133–3148 RSC;
(b) A. C. Jones, J. A. May, R. Sarpong and B. M. Stoltz, Toward a Symphony of Reactivity: Cascades Involving Catalysis and Sigmatropic Rearrangements, Angew. Chem., Int. Ed., 2014, 53, 2556–2591 CrossRef CAS PubMed;
(c) T. H. West, S. S. M. Spoehrle, K. Kasten, J. E. Taylor and A. D. Smith, Catalytic Stereoselective [2,3]-Rearrangement Reactions, ACS Catal., 2015, 5, 7446–7479 CrossRef CAS.
-
(a) J. B. Sweeney, Sigmatropic rearrangements of ‘onium’ ylids, Chem. Soc. Rev., 2009, 38, 1027–1038 RSC;
(b) A. Ford, H. Miel, A. Ring, C. N. Slattery, A. R. Maguire and M. A. McKervey, Modern Organic Synthesis with α-Diazocarbonyl Compounds, Chem. Rev., 2015, 115, 9981–10080 CrossRef CAS PubMed;
(c) V. N. Nair and U. K. Tambar, Catalytic rearrangements of onium ylides in aromatic systems, Org. Biomol. Chem., 2022, 3427–3439 RSC.
-
(a) Y. Xia, D. Qiu and J. Wang, Transition-Metal-Catalyzed Cross-Couplings through Carbene Migratory Insertion, Chem. Rev., 2017, 117, 13810–13889 CrossRef CAS;
(b) H. J. Dequina and J. M. Schomaker, Aziridinium Ylides: Underused Intermediates for Complex Amine Synthesis, Trends Chem., 2020, 2, 874–887 CrossRef CAS;
(c) S. Jana, Y. Guo and R. M. Koenigs, Recent Perspectives on Rearrangement Reactions of Ylides via Carbene Transfer Reactions, Chem. – Eur. J., 2021, 27, 1270–1281 CrossRef CAS.
- For representative examples, see:
(a) G. A. Moniz and J. L. Wood, Catalyst-based control of [2,3]- and [3,3]-rearrangement in α-diazoketone-derived propargyloxy enols, J. Am. Chem. Soc., 2001, 123, 5095–5097 CrossRef CAS;
(b) Z. Li, B. T. Parr and H. M. L. Davies, Highly stereoselective C-C bond formation by rhodium-catalyzed tandem ylide formation/[2,3]-sigmatropic rearrangement between donor/acceptor carbenoids and chiral allylic alcohols, J. Am. Chem. Soc., 2012, 134, 10942–10946 CrossRef CAS PubMed;
(c) Z. Li, V. Boyarskikh, J. H. Hansen, J. Autschbach, D. G. Musaev and H. M. L. Davies, Scope and mechanistic analysis of the enantioselective synthesis of allenes by rhodium-catalyzed tandem ylide formation/[2,3]-sigmatropic rearrangement between donor/acceptor carbenoids and propargylic alcohols, J. Am. Chem. Soc., 2012, 134, 15497–15504 CrossRef CAS;
(d) M. P. Doyle, W. H. Tamblyn and V. Bagheri, Highly Effective Catalytic Methods for Ylide Generation from Diazo Compounds. Mechanism of the Rhodium- and Copper-Catalyzed Reactions with Allylic Compounds, J. Org. Chem., 1981, 46, 5094–5102 CrossRef CAS;
(e) M. Ma, L. Peng, C. Li, X. Zhang and J. Wang, Highly stereoselective [2,3]-sigmatropic rearrangement of sulfur ylide generated through Cu(I) carbene and sulfides, J. Am. Chem. Soc., 2005, 127, 15016–15017 CrossRef CAS PubMed;
(f) Z. Zhang, Z. Sheng, W. Yu, G. Wu, R. Zhang, W. D. Chu, Y. Zhang and J. Wang, Catalytic asymmetric trifluoromethylthiolation via enantioselective [2,3]-sigmatropic rearrangement of sulfonium ylides, Nat. Chem., 2017, 9, 970–976 CrossRef CAS;
(g) X.-S. Liu, M. Li, K. Dong, S. Peng and L. Liu, Highly Stereoselective Synthesis of Tetrasubstituted Vinyl Selenides via Rhodium-Catalyzed [1,4]-Acyl Migration of Selenoesters and Diazo Compounds, Org. Lett., 2022, 24, 2175–2180 CrossRef CAS PubMed;
(h) B. Xu and U. K. Tambar, Ligand-Controlled Regiodivergence in the Copper-Catalyzed [2,3]- and [1,2]-Rearrangements of Iodonium Ylides, J. Am. Chem. Soc., 2016, 138, 12073–12076 CrossRef CAS;
(i) B. Xu and U. K. Tambar, Copper-Catalyzed Enantio-, Diastereo-, and Regioselective [2,3]-Rearrangements of Iodonium Ylides, Angew. Chem., Int. Ed., 2017, 56, 9868–9871 CrossRef CAS. For reviews and examples on [1,2]-sigmatropic rearrangement, see:
(j) J. A. Vanecko, H. Wan and F. G. West, Recent advances in the Stevens rearrangement of ammonium ylides. Application to the synthesis of alkaloid natural products, Tetrahedron, 2006, 62, 1043–1062 CrossRef CAS;
(k) J. A. Vanecko and F. G. West, A novel, stereoselective silyl-directed stevens [1,2]-shift of ammonium ylides, Org. Lett., 2002, 4, 2813–2816 CrossRef CAS;
(l) S. A. Pujari, C. Besnard, T. Bürgi and J. Lacour, A mild and efficient CH2-extrusion reaction for the enantiospecific synthesis of highly configurationally stable tröger bases, Angew. Chem., Int. Ed., 2015, 54, 7520–7523 CrossRef CAS PubMed;
(m) W. Cai, J. Wu, H. Zhang, H. B. Jalani, G. Li and H. Lu, Rh-catalyzed chemoselective [4 + 1] cycloaddition reaction toward diverse 4-methyleneprolines, J. Org. Chem., 2019, 84, 10877–10891 CrossRef CAS PubMed;
(n) D. C. Miller, R. G. Lal, L. A. Marchetti and F. H. Arnold, Biocatalytic One-Carbon Ring Expansion of Aziridines to Azetidines via a Highly Enantioselective [1,2]-Stevens Rearrangement, J. Am. Chem. Soc., 2022, 144, 4739–4745 CrossRef CAS.
- For reviews, see:
(a) K. J. Hock and R. M. Koenigs, Enantioselective [2,3]-Sigmatropic Rearrangements: Metal-Bound or Free Ylides as Reaction Intermediates?, Angew. Chem., Int. Ed., 2017, 56, 13566–13568 CrossRef CAS;
(b) C. J. Laconsay and D. J. Tantillo, Metal Bound or Free Ylides as Reaction Intermediates in Metal-Catalyzed [2,3]-Sigmatropic Rearrangements? It Depends, ACS Catal., 2021, 11, 829–839 CrossRef CAS.
- For other metal–carbene involved rearrangements, see:
(a) Y. Li, Y. Shi, Z. Huang, X. Wu, P. Xu, J. Wang and Y. Zhang, Catalytic thia-Sommelet-Hauser rearrangement: Application to the synthesis of oxindoles, Org. Lett., 2011, 13, 1210–1213 CrossRef CAS;
(b) Q. Q. Cheng, M. Lankelma, D. Wherritt, H. Arman and M. P. Doyle, Divergent Rhodium-Catalyzed Cyclization Reactions of Enoldiazoacetamides with Nitrosoarenes, J. Am. Chem. Soc., 2017, 139, 9839–9842 CrossRef CAS PubMed;
(c) S. C. Schmid, I. A. Guzei, I. Fernández and J. M. Schomaker, Ring Expansion of Bicyclic Methyleneaziridines via Concerted, Near-Barrierless [2,3]-Stevens Rearrangements of Aziridinium Ylides, ACS Catal., 2018, 8, 7907–7914 CrossRef CAS;
(d) K. Liu, G. Xu and J. Sun, Gold-catalyzed stereoselective dearomatization/metal-free aerobic oxidation: Access to 3-substituted indolines/oxindoles, Chem. Sci., 2018, 9, 634–639 RSC;
(e) Z. Liu, P. Sivaguru, G. Zanoni, E. A. Anderson and X. Bi, Catalyst-Dependent Chemoselective Formal Insertion of Diazo Compounds into C–C or C–H Bonds of 1,3-Dicarbonyl Compounds, Angew. Chem., Int. Ed., 2018, 57, 8927–8931 CrossRef CAS PubMed;
(f) J. Wang and S. S. Li, Cu(I)/chiral bisoxazoline-catalyzed enantioselective sommelet-hauser rearrangement of sulfonium ylides, J. Org. Chem., 2020, 85, 12343–12358 CrossRef PubMed;
(g) J. R. Combs, Y. C. Lai and D. L. Van Vranken, Tandem Insertion/[3,3]-Sigmatropic Rearrangement Involving the Formation of Silyl Ketene Acetals by Insertion of Rhodium Carbenes into S-Si Bonds, Org. Lett., 2021, 23, 2841–2845 CrossRef CAS PubMed;
(h) K. Dong, R. Gurung, X. Xu and M. P. Doyle, Enantioselective Catalytic Cyclopropanation-Rearrangement Approach to Chiral Spiroketals, Org. Lett., 2021, 23, 3955–3959 CrossRef CAS;
(i) A. V. Sasane, T. C. Kuo, M. J. Cheng and R. S. Liu, Gold-Catalyzed Rearrangement of α-Carbonyl Cyclopropanes to Form 3-(Cyclopenta-1,3-dien-1-ylmethyl)oxindoles via a Postulated 1,5-Enolate Shift, Org. Lett., 2022, 24, 5220–5225 CrossRef CAS PubMed.
-
(a) C. Pan, W. Guo and Z. Gu, Unusual biaryl torsional strain promotes reactivity in Cu-catalyzed Sommelet-Hauser rearrangement, Chem. Sci., 2018, 9, 5850–5854 RSC;
(b) V. N. Nair, V. Kojasoy, C. J. Laconsay, W. Y. Kong, D. J. Tantillo and U. K. Tambar, Catalyst-Controlled Regiodivergence in Rearrangements of Indole-Based Onium Ylides, J. Am. Chem. Soc., 2021, 143, 9016–9025 CrossRef CAS.
- G. Xu, P. Chen, P. Liu, S. Tang, X. Zhang and J. Sun, Access to N-Substituted 2-Pyridones by Catalytic Intermolecular Dearomatization and 1,4-Acyl Transfer, Angew. Chem., Int. Ed., 2019, 58, 1980–1984 CrossRef CAS.
-
(a) G. Xu, Y. Shao, S. Tang, Q. Chen and J. Sun, Dearomative Migratory Rearrangement of 2-Oxypyridines Enabled by α-Imino Rhodium Carbene, Org. Lett., 2020, 22, 9303–9307 CrossRef CAS PubMed;
(b) H. Cui, G. Xu, J. Zhu and J. Sun, Rhodium-catalyzed dearomative rearrangement of 2-oxypyridines with cyclopropenes: access to N-alkylated 2-pyridones, Org. Chem. Front., 2022, 9, 1295–1299 RSC;
(c) J. Su, Q. Li, Y. Shao and J. Sun, Catalytic Transformations of 2-Pyridones by Rhodium-Mediated Carbene Transfer, Org. Lett., 2022, 24, 1637–1641 CrossRef CAS.
|
This journal is © the Partner Organisations 2023 |
Click here to see how this site uses Cookies. View our privacy policy here.