DOI:
10.1039/D2QO01654K
(Research Article)
Org. Chem. Front., 2023,
10, 92-98
Enantioselective sulfonylation using sodium hydrogen sulfite, 4-substituted Hantzsch esters and 1-(arylethynyl)naphthalen-2-ols†
Received
19th October 2022
, Accepted 10th November 2022
First published on 11th November 2022
Abstract
Synthesis of sulfonyl-containing axially chiral styrenes through a catalytic asymmetric three-component reaction of 4-substituted Hantzsch esters, NaHSO3 (sodium hydrogen sulfite), and 1-(arylethynyl)naphthalen-2-ols in the presence of a photocatalyst under visible light irradiation is reported. This transformation proceeds through a radical process under mild conditions, leading to axially chiral (S,E)-1-(1-(alkylsulfonyl)-2-arylvinyl)naphthalen-2-ols in good yields with excellent enantioselectivities. During the reaction process, excellent regioselectivity and chemoselectivity were observed as well. Notably, sodium hydrogen sulfite is used as the sulfur dioxide surrogate in this organocatalytic enantioselective radical sulfonylation under photoinduced conditions.
Significant progress has been achieved in the generation of sulfonyl compounds with the insertion of sulfur dioxide.1,2 In such transformations, sulfur dioxide surrogates including DABCO·(SO2)2 and inorganic sulfites have been used widely, which show remarkable advantages namely easy availability and operational simplicity. Over the past decade, we have focused on radical reactions for the preparation of sulfonyl compounds from sulfur dioxide surrogates.3 The key intermediate of a sulfonyl radical can be formed via a combination of an alkyl/alkenyl radical with sulfur dioxide. Although various strategies with regard to the radical reaction of sulfur dioxide have been developed, asymmetric radical reactions for the enantioselective synthesis of sulfonyl compounds from sulfur dioxide are rare. The first example of asymmetric sulfonylation from sulfur dioxide was reported by Han, Pan and coworkers (Scheme 1, eqn (a)),4 who developed a reaction of an unsaturated carboxylic acid, aryldiazonium tetrafluoroborate, and DABCO·(SO2)2. However, the yields and enantioselectivities were low to moderate and were not satisfactory. A reaction of MBH acetate, 4-substituted Hantzsch ester and NaHSO3 (sodium hydrogen sulfite) catalyzed by a chiral tertiary amine by using Mes-Acr+ as the photocatalyst under visible light irradiation was described, although the corresponding chiral allylic sulfone was afforded in 43% yield with 33% ee.5 Subsequently, Gong and co-workers demonstrated a photoinduced asymmetric sulfonyl radical addition to α,β-unsaturated carbonyl compounds under chiral nickel catalysis (Scheme 1, eqn (b)).6 The sulfonyl radical was generated in situ from a reaction of the C(sp3)–H precursor and DABCO·(SO2)2. Lian and coworkers disclosed the synthesis of chiral β-sulfonyl nitriles via a copper-catalyzed four-component enantioselective arylsulfonylcyanation of vinylarenes with a bench-stable SO2 surrogate (SOgen) (Scheme 1, eqn (c)).7 Very recently, we developed a photoinduced asymmetric radical approach to access sulfonyl-containing axially chiral styrenes through a three-component reaction of potassium alkyltrifluoroborates, potassium metabisulfite and 1-(arylethynyl)naphthalen-2-ols (Scheme 1, eqn (d)).8 Later, the synthesis of chiral β-sulfonyl carbonyl compounds through an organocatalytic enantioselective radical reaction of potassium alkyltrifluoroborates, DABCO·(SO2)2 and α,β-unsaturated carbonyl compounds under photoinduced conditions was achieved (Scheme 1, eqn (d)).9
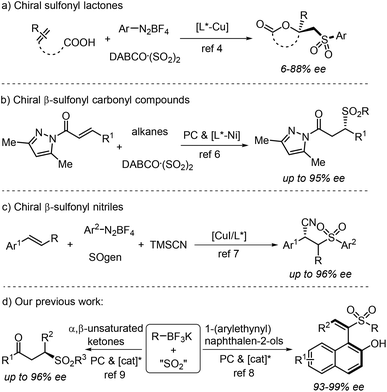 |
| Scheme 1 Synthesis of chiral sulfonyl compounds. | |
In the past few years, the development of methods for the generation of axially chiral alkenes has attracted continuous interest due to their great potential in the development of chiral ligands, catalysts and functional materials.10 Compared with the well-established atropisomeric aryl-dihydronaphthyl skeleton,11 the catalytic asymmetric synthesis of axially chiral styrenes with non-cyclic alkenes is less explored due to the challenges of a low rotational barrier and a more flexible framework.12 In 2017, Tan and co-workers reported a seminal work on the atroposelective construction of axially chiral styrenes through an organocatalytic asymmetric nucleophilic addition to alkynals.13 Later, several strategies were successively developed to access axially chiral styrenes including our work related to asymmetric radical reactions (Scheme 1, eqn (d)).8,14 Encouraged by the recent advances in sulfur dioxide insertion chemistry with inorganic sulfites via a radical process, we envisioned that axially chiral styrenes would also be accessible through a photoinduced asymmetric radical sulfonylation using inorganic sulfite as the sulfur dioxide surrogate and 4-substituted Hantzsch ester as the radical precursor (Scheme 2). We conceived that 1-(arylethynyl)naphthalen-2-ol 1 would be an ideal choice as the substrate, which would convert to the allene intermediate Int-I
8,13 in the presence of a chiral organocatalyst. Meanwhile, a radical formed in situ from the 4-substituted Hantzsch ester 2 under suitable conditions would be trapped by sulfur dioxide, giving rise to a sulfonyl radical. Subsequently, the sulfonyl radical would react with the allene intermediate Int-I, producing a radical intermediate. After reductive single electron transfer and protonation, sulfonyl-containing axially chiral styrene 3 would be formed. During the transformation, chirality could be introduced via a coordination of a substrate with a chiral organocatalyst. Herein, we report the synthesis of sulfonyl-containing axially chiral styrenes through a catalytic asymmetric three-component reaction of 4-substituted Hantzsch esters, NaHSO3 (sodium hydrogen sulfite), and 1-(arylethynyl)naphthalen-2-ols in the presence of a photocatalyst under visible light irradiation. This transformation proceeds through a radical process under mild conditions, leading to axially chiral (S,E)-1-(1-(alkylsulfonyl)-2-arylvinyl)naphthalen-2-ols in good yields with excellent enantioselectivity and regioselectivity. Notably, sodium hydrogen sulfite is used as the sulfur dioxide surrogate in this asymmetric reaction.
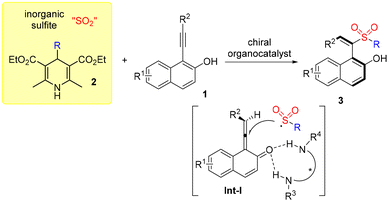 |
| Scheme 2 Generation of axially chiral styrenes from inorganic sulphite and 4-substituted Hantzsch ester. | |
Recently, we demonstrated that 4-substituted Hantzsch esters were excellent radical precursors in the sulfonylation process with sulfur dioxide under visible light irradiation in the presence of a photocatalyst.15 Inspired by these results, we commenced our study by using 1-(phenylethynyl)naphthalen-2-ol 1a and 4-cyclohexyl Hantzsch ester 2a as the model substrates with inorganic sulfite as the sulfur dioxide surrogate to explore the optimal reaction conditions (Table 1). At the outset, potassium metabisulfite was employed in the reaction by using compound A as the organocatalyst and Mes-Acr+ClO4− as the photocatalyst in PhCF3 at 20 °C under visible light irradiation (Table 1, entry 1). However, no reaction occurred under these conditions. Interestingly, the desired product 3a was afforded in 21% yield and 99% ee when sodium metabisulfite was used instead (Table 1, entry 2). Further investigation showed that the result was dramatically improved when sodium hydrogen sulfite was utilized as the source of sulfur dioxide (80% yield, 99% ee, Table 1, entry 3). Encouraged by this result, we then examined the reaction with other bifunctional organocatalysts B–D (Table 1, entries 4–6). However, no better result was obtained. Other solvents including CH2Cl2, MeCN, 1,4-dioxane, THF and CHCl3 were screened subsequently (Table 1, entries 7–11). Remarkably, excellent enantioselectivities were observed in all cases, although the yields were not satisfactory. No reaction took place for control experiments without light irradiation or photocatalysts. A similar result was achieved when DABCO·(SO2)2 was used as a replacement for sodium hydrogen sulfite (Table 1, entry 12). Considering the easy availability and cost, using sodium hydrogen sulfite as the source of sulfur dioxide would be ideal for reaction development.
Table 1 Initial studies on the reaction of 1-(phenylethynyl)naphthalen-2-ol 1a, 4-substituted Hantzsch ester 2a and sodium hydrogen sulfite
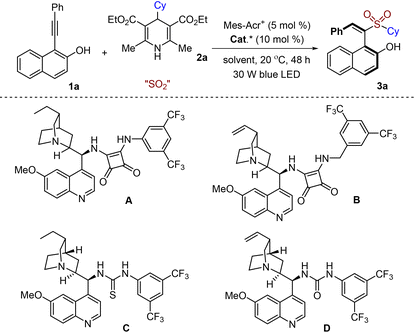
|
Entry |
“SO2” |
Cat.* (mol%) |
Solvent |
Yielda (%) |
eeb (%) |
Reaction conditions: 1-(phenylethynyl)naphthalen-2-ol 1a (0.2 mmol), 4-substituted Hantzsch ester 2a (0.4 mmol), NaHSO3 (0.4 mmol), Mes-Acr+ClO4− (5 mol%), Cat.* (10 mol%), solvent (2.0 mL), blue LEDs, 48 h. Isolated yield based on 1-(phenylethynyl)naphthalen-2-ol 1a. Determined by HPLC analysis on a chiral stationary phase. |
1 |
K2S2O5 |
A
|
PhCF3 |
n.r. |
— |
2 |
Na2S2O5 |
A
|
PhCF3 |
21 |
99 |
3 |
NaHSO3 |
A
|
PhCF3 |
80 |
99 |
4 |
NaHSO3 |
B
|
PhCF3 |
78 |
94 |
5 |
NaHSO3 |
C
|
PhCF3 |
76 |
96 |
6 |
NaHSO3 |
D
|
PhCF3 |
80 |
90 |
7 |
NaHSO3 |
A
|
CH2Cl2 |
79 |
96 |
8 |
NaHSO3 |
A
|
MeCN |
43 |
96 |
9 |
NaHSO3 |
A
|
1,4-Dioxane |
5 |
96 |
10 |
NaHSO3 |
A
|
THF |
23 |
96 |
11 |
NaHSO3 |
A
|
CHCl3 |
76 |
98 |
12 |
DABCO·(SO2)2 |
A
|
PhCF3 |
83 |
98 |
With the above-mentioned optimal conditions in hand, we then explored the substrate scope of this organocatalytic asymmetric three-component reaction of 1-(arylethynyl)naphthalen-2-ols 1, 4-substituted Hantzsch esters 2 and sodium hydrogen sulfite in the presence of a photocatalyst under visible light irradiation (Table 2). In general, the reaction proceeded efficiently with a wide range of substrates, leading to the corresponding products 3 in moderate to good yields with universally excellent enantioselectivities. For example, 1-(phenylethynyl)naphthalen-2-ol 1a reacted well with 4-substituted Hantzsch esters 2 and sodium hydrogen sulfite, giving rise to the desired products 3a–3d in 97–99% ee. Subsequently, diverse 1-(arylethynyl)naphthalen-2-ols 1 were examined in the reaction of 4-cyclopentyl Hantzsch ester and sodium hydrogen sulfite under the standard conditions. Various functional groups were compatible with satisfactory results. Changing the aryl group of R2 to alkyl and heteroaryl groups had little effect on the outcome of enantioselectivities. The absolute configuration of the product was determined to compare with a previous report.8
Table 2 Scope exploration for the reaction of 1-alkynylnaphthalen-2-ols 1, 4-substituted Hantzsch esters 2 and NaHSO3a
Isolated yield based on 1-(phenylethynyl)naphthalen-2-ols 1.
|
|
To further evaluate the practicality of this method, the large-scale synthesis of axially chiral (S,E)-1-(1-(alkylsulfonyl)-2-arylvinyl)naphthalen-2-ol 3e was performed at the 2 mmol scale with 1-(phenylethynyl)naphthalen-2-ol 1a, 4-cyclopentyl Hantzsch ester 2b and sodium hydrogen sulfite in the presence of a photocatalyst under visible light irradiation. As shown in Scheme 3, the desired product 3e was obtained in 53% yield and 97% ee.
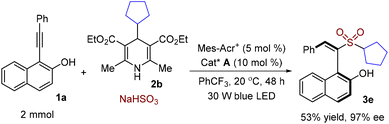 |
| Scheme 3 Large-scale synthesis. | |
Next, several control experiments were carried out to elucidate the reaction pathway. No reaction took place without the addition of a chiral organocatalyst or photocatalyst. Additionally, the reaction failed to provide the desired product 5 (Scheme 4) when the hydroxyl group in 1-(phenylethynyl)naphthalen-2-ol 1a was protected as the acetyl group (OAc). This result showed that the hydroxyl group played a crucial role during the reaction process. Moreover, the transformation was completely hampered with the addition of 4.0 equiv. of the radical scavenger TEMPO (2,2,6,6-tetramethylpiperidine-1-oxyl) under the standard conditions (Scheme 4). Consequently, the radical trapping adduct was detected by HRMS (high-resolution mass spectrometry). These preliminary results suggested that a radical process would be involved in this organocatalytic asymmetric reaction.
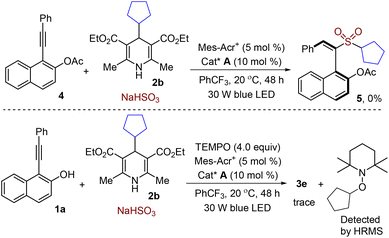 |
| Scheme 4 Control experiments. | |
On the basis of the above experiment results and previous reports on organocatalytic asymmetric radical transformations,14 we proposed a plausible reaction pathway for this photoinduced enantioselective radical sulfonylation (Scheme 5). We reasoned that 1-(arylethynyl)naphthalen-2-ol 1 would be converted to allene Int-I with the assistance of the chiral organocatalyst. Meanwhile, an alkyl radical would be produced from the 4-substituted Hantzsch ester 2 through a single electron transfer by the photocatalyst under visible light irradiation, which would be trapped by sulfur dioxide, affording the alkylsulfonyl radical. Subsequently, the enantioselective addition of the alkylsulfonyl radical to allene Int-I would occur, giving rise to the radical intermediate Int-II. After a reductive single electron transfer with an excited photocatalyst, the anion intermediate Int-III would be produced, which would be isomerized to intermediate Int-IV. Further protonation of intermediate Int-IV would provide the corresponding product 3.
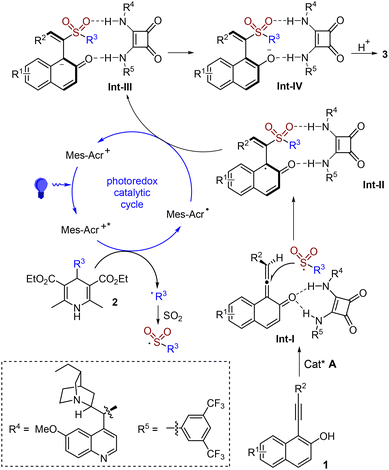 |
| Scheme 5 Proposed mechanism. | |
In conclusion, we report an efficient strategy for the synthesis of sulfonyl-containing axially chiral styrenes through a catalytic asymmetric three-component reaction of 4-substituted Hantzsch esters, NaHSO3 (sodium hydrogen sulfite), and 1-(arylethynyl)naphthalen-2-ols in the presence of a photocatalyst under visible light irradiation. This transformation proceeds through a radical process under mild conditions, leading to axially chiral (S,E)-1-(1-(alkylsulfonyl)-2-arylvinyl)naphthalen-2-ols in good yields with excellent enantioselectivities. During the reaction process, excellent regioselectivity and chemoselectivity are observed as well. Notably, sodium hydrogen sulfite is used as the sulfur dioxide surrogate in this organocatalytic enantioselective radical sulfonylation under photoinduced conditions.
Data availability
The data supporting this study are available within the article and the ESI.†
Conflicts of interest
There are no conflicts to declare.
Acknowledgements
Financial support from the National Natural Science Foundation of China (no. 22171206), the Leading Innovative and Entrepreneur Team Introduction Program of Zhejiang (no. 2019R01005), and the Open Research Fund of School of Chemistry and Chemical Engineering, Henan Normal University (2020ZD04) is gratefully acknowledged.
Notes and references
- For selected reviews, see:
(a)
D. Zheng and J. Wu, Sulfur dioxide insertion reactions for organic synthesis, Nature Springer, Berlin, 2017 CrossRef;
(b) G. Qiu, K. Zhou, L. Gao and J. Wu, Insertion of sulfur dioxide via a radical process: an efficient route to sulfonyl compounds, Org. Chem. Front., 2018, 5, 691 RSC;
(c) K. Hofman, N. Liu and G. Manolikakes, Radicals and sulfur dioxide: a versatile combination for the construction of sulfonyl-containing molecules, Chem. – Eur. J., 2018, 24, 11852 CrossRef CAS PubMed;
(d) G. Qiu, L. Lai, J. Cheng and J. Wu, Recent advances in the sulfonylation of alkenes with the insertion of sulfur dioxide via radical reactions, Chem. Commun., 2018, 54, 10405 RSC;
(e) G. Qiu, K. Zhou and J. Wu, Recent advances in the sulfonylation of C-H bonds with the insertion of sulfur dioxide, Chem. Commun., 2018, 54, 12561 RSC;
(f) S. Ye, G. Qiu and J. Wu, Inorganic sulfites as the sulfur dioxide surrogates in sulfonylation reactions, Chem. Commun., 2019, 55, 1013 RSC;
(g) S. Ye, M. Yang and J. Wu, Recent advances in sulfonylation reactions using potassium/sodium Metabisulfite, Chem. Commun., 2020, 56, 4145 RSC;
(h) D. Zeng, M. Wang, W.-P. Deng and X. Jiang, The same oxygenation-state introduction of hypervalent sulfur under transition-metal-free conditions, Org. Chem. Front., 2020, 7, 3956 RSC;
(i) F.-S. He, M. Yang, S. Ye and J. Wu, Sulfonylation from sodium dithionite or thiourea dioxide, Chin. Chem. Lett., 2021, 32, 461 CrossRef CAS;
(j) S. P. Blum, K. Hofman, G. Manolikakes and S. R. Waldvogel, Advances in photochemical and electrochemical incorporation of sulfur dioxide for the synthesis of value-added compounds, Chem. Commun., 2021, 57, 8236 RSC.
- For selected examples, see:
(a) X. Jia, S. Kramer, T. Skrydstrup and Z. Lian, Design and applications of a SO2 surrogate in palladium-catalyzed direct aminosulfonylation between aryl iodides and amines, Angew. Chem., Int. Ed., 2021, 60, 7353 CrossRef CAS;
(b) Y. Li, S. Chen, M. Wang and X. Jiang, Sodium dithionite-mediated decarboxylative sulfonylation: facile access to tertiary sulfones, Angew. Chem., Int. Ed., 2020, 59, 8907 CrossRef CAS PubMed;
(c) X.-L. Chen, B.-C. Tang, C. He, J.-T. Ma, S.-Y. Zhuang, Y.-D. Wu and A.-X. Wu, Rongalite as a sulfone source: a novel copper-catalyzed sulfur dioxide anion incorporation process, Chem. Commun., 2020, 56, 13653 RSC;
(d) F. Herrera, A. Luna and P. Almendros, Visible-light-mediated Ru-catalyzed synthesis of 3-(arylsulfonyl)but-3-enals via coupling of α-allenols with diazonium salts and sulfur dioxide, Org. Lett., 2020, 22, 9490 CrossRef CAS;
(e) S. P. Blum, T. Karakaya, D. Schollmeyer, A. Klapars and S. R. Waldvogel, Metal-free electrochemical synthesis of sulfonamides directly from (hetero)arenes, SO2, and amines, Angew. Chem., Int. Ed., 2021, 60, 5056 CrossRef CAS;
(f) Y. Lv, J. Luo, Y. Ma, Q. Dong and L. He, Visible-light-promoted sulfonylation of thiols with aryldiazonium and sodium metabisulphite leading to unsymmetrical thiosulfonates, Org. Chem. Front., 2021, 8, 2461 RSC;
(g) X. Jia, C. Huang, X. Zhang and Z. Lian, Metal-free sulfonylative annulations of alkyl diiodides with sulfur dioxide: synthesis of cyclic aliphatic sulfones, Org. Chem. Front., 2021, 8, 5310 RSC;
(h) M. A. Idris and S. Lee, One-pot synthesis of pentafluorophenyl sulfonic esters via copper-catalyzed reaction of aryl diazonium salts, DABSO, and pentafluorophenol, Org. Lett., 2021, 23, 4516 CrossRef CAS PubMed;
(i) Z. Chen, H. Zhang, S.-F. Zhou and X. Cui, Metal-free sulfonylative spirocyclization of indolyl-ynones via insertion of sulfur dioxide: access to sulfonated spiro[cyclopentenone-1,3′-indoles], Org. Lett., 2021, 23, 7992 CrossRef CAS;
(j) T. S.-B. Lou, Y. Kawamata, T. Ewing, G. A. Correa-Otero, M. R. Collins and P. S. Baran, Scalable, chemoselective nickel electrocatalytic sulfinylation of aryl halides with SO2, Angew. Chem., Int. Ed., 2022, 61, e2022080 Search PubMed;
(k) Z. Chen, H. Zhang, S.-F. Zhou and X. Cui, Photoredox-catalyzed synthesis of sulfonated oxazolines from N-allylamides through the insertion of sulfur dioxide, Org. Chem. Front., 2022, 9, 364 RSC;
(l) R. Ding, Y.-H. Deng, S. Luo, X.-F. Tang, L. Liu and P.-L. Wang, Metal-free regioselective cascade sulfonylation–cyclization of 3-aza-1,5-enynes with sulfur dioxide and aryldiazonium tetrafluoroborates to construct 1,2-dihydropyridines, Org. Chem. Front., 2022, 9, 2228 RSC;
(m) Y. Li, X. Zhang and Z. Lian, Copper catalyzed cyano-sulfonylation of allenes via the insertion of sulfur dioxide toward the synthesis of (E)-α-cyanomethyl vinylsulfones, Org. Chem. Front., 2022, 9, 5141 RSC;
(n) H. Konishi, R. Fujita, M. Yamaguchi and K. Manabe, Synthesis of symmetrical sulfides Enabled by a sulfur dioxide surrogate acting as a divalent sulfur source, Org. Lett., 2022, 24, 3663 CrossRef CAS.
- For selected examples, see:
(a) D. Zheng, J. Yu and J. Wu, Generation of sulfonyl radicals from aryldiazonium tetrafluoroborates and sulfur dioxide: The synthesis of 3-sulfonated coumarins, Angew. Chem., Int. Ed., 2016, 55, 11925 CrossRef CAS;
(b) J. Zhang, M. Yang, J.-B. Liu, F.-S. He and J. Wu, A copper-catalyzed insertion of sulfur dioxide via radical coupling, Chem. Commun., 2020, 56, 3225 RSC;
(c) F.-S. He, P. Bao, F. Yu, L.-H. Zeng, W.-P. Deng and J. Wu, Copper-catalyzed regioselective 1,4-selenosulfonylation of 1,3-enynes to access cyanoalkylsulfonylated allenes, Org. Lett., 2021, 23, 7472 CrossRef CAS PubMed;
(d) F.-S. He, P. Bao, Z. Tang, F. Yu, W. Deng and J. Wu, Photoredox-catalyzed α-sulfonylation of ketones from sulfur dioxide and thianthrenium salts, Org. Lett., 2022, 24, 2955 CrossRef CAS PubMed;
(e) X. Wang, Y. Kuang, S. Ye and J. Wu, Photoredox-catalyzed synthesis of sulfones through deaminative insertion of sulfur dioxide, Chem. Commun., 2019, 55, 14962 RSC;
(f) J. Zhang, X. Wang, Q. Chen, J. Liu, W. Zhou and J. Wu, (E)-β-Trifluoromethyl vinylsulfones as antitumor agents: Synthesis and biological evaluations, Eur. J. Med. Chem., 2022, 232, 114197 CrossRef CAS PubMed;
(g) Y. Liu, X. Zhang, J. Lv, C. Zhang, X. Chang, S. Ye and J. Wu, A photocatalytic radical relay reaction of 2-methylthiolated phenylalkynones and potassium metabisulfite, Org. Chem. Front., 2022, 9, 450 RSC;
(h) J. Zhang, J. Wu, X. Chang, P. Wang, J. Xia and J. Wu, An iron-catalyzed multicomponent reaction of cycloketone oxime esters, alkenes, DABCO·(SO2)2 and trimethylsilyl azide, Org. Chem. Front., 2022, 9, 917 RSC;
(i) M. Yang, B. Ye, J. Chen and J. Wu, Visible-light photocatalytic alkylsulfonylation of aroylhydrazides with alkylsulfonyl radicals, Acta Chim. Sin., 2022, 80, 11 CrossRef;
(j) X. Wang, J. Zhang, Q. Chen, W. Zhou and J. Wu, Generation of sulfonylureas under photoredox catalysis and their biological evaluations, Chin. Chem. Lett., 2022, 33, 4860 CrossRef CAS;
(k) Y. Lv, J. Luo, Y. Ma, Q. Dong and L. He, Visible-light-promoted sulfonylation of thiols with aryldiazonium and sodium metabisulphite leading to unsymmetrical thiosulfonates, Org. Chem. Front., 2021, 8, 2461 RSC;
(l) J. Liu, J. Xu, H. Mei and J. Han, Electrochemical multi-component reaction of potassium metabisulfite with alkenes and alcohols enabling synthesis of sulfonate esters, Green Chem., 2022, 24, 6113 RSC;
(m) Y. Lv, J. Luo, M. Lin, L. He, H. Yue, R. Liu and W. Wei, Metal-free multi-component sulfur dioxide insertion reaction leading to quinoxalin-2-one-containing vinyl sulfones under visible-light photoredox catalysis, Adv. Synth. Catal., 2021, 363, 1 CrossRef.
- Y. Wang, L. Deng, J. Zhou, X. Wang, H. Mei, J. Han and Y. Pan, Synthesis of chiral sulfonyl lactones via copper-catalyzed asymmetric radical reaction of DABCO·(SO2)2, Adv. Synth. Catal., 2018, 360, 1060 CrossRef CAS.
- X. Wang, R. Liu, Q. Ding, W. Xiao and J. Wu, Synergistic photoredox and tertiary amine catalysis: Generation of allylic sulfones from Morita-Baylis-Hillman acetates and sulfur dioxide, Org. Chem. Front., 2021, 8, 3308 RSC.
- S. Cao, W. Hong, Z. Ye and L. Gong, Photocatalytic three-component asymmetric sulfonylation via direct C(sp3)-H functionalization, Nat. Commun., 2021, 12, 2377 CrossRef CAS PubMed.
- L. Chen, X. Zhang, M. Zhou, L. Shen, S. Kramer and Z. Lian, Enantioselective four-component arylsulfonylcyanation of vinylarenes via the insertion of SO2 enabled by SOgen as SO2 surrogate, ACS Catal., 2022, 12, 10764 CrossRef CAS.
- C. Zhang, Z. Tang, Y. Qiu, J. Tang, S. Ye, Z. Li and J. Wu, Access to axially chiral styrenes via a photoinduced asymmetric radical reaction involving a sulfur dioxide insertion, Chem. Catal., 2022, 2, 164 CrossRef.
- F.-S. He, C. Zhang, M. Jiang, L. Lou, J. Wu and S. Ye, Access to chiral β-sulfonyl carbonyl compounds via photoinduced organocatalytic asymmetric radical sulfonylation with sulfur dioxide, Chem. Sci., 2022, 13, 8834 RSC.
-
(a) K. Mori, K. Ohmori and K. Suzuki, Stereochemical relay via axially chiral styrenes: Asymmetric synthesis of the antibiotic TAN-1085, Angew. Chem., Int. Ed., 2009, 48, 5633 CrossRef CAS;
(b) K. Mori, K. Ohmori and K. Suzuki, Hydrogen-bond control in axially chiral styrenes: Selective synthesis of enantiomerically pure C2-symmetric paracyclophanes, Angew. Chem., Int. Ed., 2009, 48, 5638 CrossRef CAS PubMed;
(c) F. Glorius, Chiral olefin ligands-new “spectators” in asymmetric catalysis, Angew. Chem., Int. Ed., 2004, 43, 3364 CrossRef CAS PubMed;
(d) C. Defieber, H. Gruṳtzmacher and E. M. Carreira, Chiral olefins as steering ligands in asymmetric catalysis, Angew. Chem., Int. Ed., 2008, 47, 4482 CrossRef CAS PubMed;
(e) P. Tian, H. H.-Q. Dong and G.-Q. Lin, Rhodium-catalyzed asymmetric arylation, ACS Catal., 2012, 2, 95 CrossRef CAS;
(f) X. Feng and H. Du, Synthesis of chiral olefin ligands and their application in asymmetric catalysis, Asian J. Org. Chem., 2012, 1, 204 CrossRef CAS;
(g) Y. Li and M.-H. Xu, Simple sulfur-olefins as new promising chiral ligands for asymmetric catalysis, Chem. Commun., 2014, 50, 3771 RSC;
(h) M. Nagamoto and T. Nishimura, Asymmetric transformations under iridium/chiral diene catalysis, ACS Catal., 2017, 7, 833 CrossRef CAS.
-
(a) J. D. Jolliffe, R. J. Armstrong and M. D. Smith, Catalytic enantioselective synthesis of atropisomeric biaryls by a cation-directed O-alkylation, Nat. Chem., 2017, 9, 558 CrossRef CAS PubMed;
(b) J. Feng, B. Li, Y. He and Z. Gu, Enantioselective synthesis of atropisomeric vinyl arene compounds by palladium catalysis: A carbene strategy, Angew. Chem., Int. Ed., 2016, 55, 2186 CrossRef CAS PubMed;
(c) C. Pan, Z. Zhu, M. Zhang and Z. Gu, Palladium-catalyzed enantioselective synthesis of 2-aryl cyclohex-2-enone atropisomers: Platform molecules for the divergent synthesis of axially chiral biaryl compounds, Angew. Chem., Int. Ed., 2017, 56, 4777 CrossRef CAS PubMed;
(d) Q.-Y. Sun, W.-Y. Ma, K.-F. Yang, J. Cao, Z. Zheng, Z.-J. Xu, Y.-M. Cui and L.-W. Xu, Enantioselective synthesis of axially chiral vinyl arenes through palladium-catalyzed C-H olefination, Chem. Commun., 2018, 54, 10706 RSC;
(e) Y. Liang, J. Ji, X. Zhang, Q. Jiang, J. Luo and X. Zhao, Enantioselective construction of axially chiral amino sulfide vinyl arenes by chiral sulfide-catalyzed electrophilic carbothiolation of alkynes, Angew. Chem., Int. Ed., 2020, 59, 4959 CrossRef CAS PubMed;
(f) Y.-H. Chen, H.-H. Li, X. Zhang, S.-H. Xiang, S. Li and B. Tan, Organocatalytic enantioselective synthesis of atropisomeric aryl-p-quinones: Platform molecules for diversity-oriented synthesis of biaryldiols, Angew. Chem., Int. Ed., 2020, 59, 11374 CrossRef CAS PubMed;
(g) Z. Li, X. Wang, Y.-M. Cui, J.-H. Ma, L.-L. Fang, L.-L. Han, Q. Yang, Z. Xu and L.-W. Xu, Combined dynamic kinetic resolution and C-H functionalization for facile synthesis of non-biaryl-atropisomer-type axially chiral organosilanes, Chem. – Eur. J., 2021, 27, 4336 CrossRef CAS PubMed.
-
(a) X. Bao, J. Rodriguez and D. Bonne, Enantioselective synthesis of atropisomers with multiple stereogenic axes, Angew. Chem., Int. Ed., 2020, 59, 12623 CrossRef CAS PubMed;
(b) J. Feng and Z. Gu, Atropisomerism in styrene: Synthesis, stability, and applications, SynOpen, 2021, 5, 68 CrossRef CAS;
(c) J.-K. Cheng, S.-H. Xiang, S. Li, L. Ye and B. Tan, Recent advances in catalytic asymmetric construction of atropisomers, Chem. Rev., 2021, 121, 4805 CrossRef CAS PubMed.
- S.-C. Zheng, S. Wu, Q. Zhou, L. Chung, L. Ye and B. Tan, Organocatalytic atroposelective synthesis of axially chiral styrenes, Nat. Commun., 2017, 8, 15238 CrossRef PubMed.
-
(a) Y.-B. Wang, Q.-H. Wu, Z.-P. Zhou, S.-H. Xiang, Y. Cui, P. Yu and B. Tan, Asymmetric construction of axially chiral 2-arylpyrroles by chirality transfer of atropisomeric alkenes, Angew. Chem., Int. Ed., 2019, 58, 13443 CrossRef CAS PubMed;
(b) C. Sun, X. Qi, X.-L. Min, X.-D. Bai, P. Liu and Y. He, Asymmetric allylic substitution-Isomerization to axially chiral enamides via hydrogen-bonding assisted central-to-axial chirality transfer, Chem. Sci., 2020, 11, 10119 RSC;
(c) C. Ma, F.-T. Sheng, H.-Q. Wang, S. Deng, Y. Zhang, Y.-C. Jiao, W. Tan and F. Shi, Atroposelective access to oxindole-based axially chiral styrenes via the strategy of catalytic kinetic resolution, J. Am. Chem. Soc., 2020, 142, 15686 CrossRef CAS PubMed;
(d) S. Jia, Z. Chen, N. Zhang, Y. Tan, Y. Liu, J. Deng and H. Yan, Organocatalytic enantioselective construction of axially chiral sulfone-containing styrenes, J. Am. Chem. Soc., 2018, 140, 7056 CrossRef CAS PubMed;
(e) Y. Tan, S. Jia, F. Hu, Y. Liu, L. Peng, D. Li and H. Yan, Enantioselective construction of vicinal diaxial styrenes and multiaxis system via organocatalysis, J. Am. Chem. Soc., 2018, 140, 16893 CrossRef CAS PubMed;
(f) S. Li, D. Xu, F. Hu, D. Li, W. Qin and H. Yan, Organocatalytic asymmetric atroposelective construction of axially chiral 1,4-distyrene 2,3-naphthalene Diols, Org. Lett., 2018, 20, 7665 CrossRef CAS PubMed;
(g) D. Li, Y. Tan, L. Peng, S. Li, N. Zhang, Y. Liu and H. Yan, Asymmetric Mannich reaction and construction of axially chiral sulfone-containing styrenes in one pot from α-amido sulfones based on the Waste-Reuse strategy, Org. Lett., 2018, 20, 4959 CrossRef CAS PubMed;
(h) A. Huang, L. Zhang, D. Li, Y. Liu, H. Yan and W. Li, Asymmetric one-pot construction of three stereogenic
elements: Chiral carbon center, stereoisomeric alkenes, and chirality of axial styrenes, Org. Lett., 2019, 21, 95 CrossRef CAS PubMed;
(i) L. Jin, Q.-J. Yao, P.-P. Xie, Y. Li, B.-B. Zhan, Y.-Q. Han, X. Hong and B.-F. Shi, Atroposelective synthesis of axially chiral styrenes via an asymmetric C-H functionalization strategy, Chem, 2020, 6, 497 CrossRef CAS;
(j) H. Song, Y. Li, Q.-J. Yao, L. Jin, L. Liu, Y.-H. Liu and B.-F. Shi, Synthesis of axially chiral styrenes through Pd-catalyzed asymmetric C-H olefination enabled by an amino amide transient directing group, Angew. Chem., Int. Ed., 2020, 59, 6576 CrossRef CAS PubMed;
(k) C. Yang, T.-R. Wu, Y. Li, B.-B. Wu, R.-X. Jin, D.-D. Hu, Y.-B. Li, K.-J. Bian and X.-S. Wang, Facile synthesis of axially chiral styrene-type carboxylic acids via palladium-catalyzed asymmetric C-H activation, Chem. Sci., 2021, 12, 3726 RSC.
-
(a) X. Wang, Y. Tang, S. Ye, J. Zhang, Y. Kuang and J. Wu, Access to sulfoxides under NHC/photocatalysis via a radical pathway, Org. Lett., 2022, 24, 2059 CrossRef CAS PubMed;
(b) X. Wang, H. Li, G. Qiu and J. Wu, Substituted Hantzsch esters as radical reservoirs with the insertion of sulfur dioxide under photoredox catalysis, Chem. Commun., 2019, 55, 2062 RSC;
(c) X. Wang, M. Yang, W. Xie, X. Fan and J. Wu, Photoredox-catalyzed hydrosulfonylation reaction of electron-deficient alkenes with substituted Hantzsch esters and sulfur dioxide, Chem. Commun., 2019, 55, 6010 RSC;
(d) S. Ye and J. Wu, 4-Substituted Hantzsch esters as alkylation reagents in organic synthesis, Acta Chim. Sin., 2019, 77, 814 CrossRef CAS.
Footnote |
† Electronic supplementary information (ESI) available: Experimental details and spectral data, copies of 1H and 13C NMR spectra. See DOI: https://doi.org/10.1039/d2qo01654k |
|
This journal is © the Partner Organisations 2023 |
Click here to see how this site uses Cookies. View our privacy policy here.