DOI:
10.1039/D2QO01730J
(Research Article)
Org. Chem. Front., 2023,
10, 209-215
Hydrogen Bonding-directed Sequential 1,6/1,4-Addition of Heteroatom Nucleophiles onto Electron-deficient 1,3-Diynes†
Received
31st October 2022
, Accepted 20th November 2022
First published on 29th November 2022
Abstract
A sequential inter- and intramolecular 1,6/1,4-addition on electron-deficient 1,3-diynes conjugated with a ketone, ester, or imide functionality is described. This tandem process is effectively directed by a propargylic –OH or –NH functionality. Alcohols, amines, and thiols show excellent reactivity for the initial 1,6-addition. The addition of amines occurs at ambient temperature, whereas other nucleophiles require high temperatures up to 100 °C and a base additive such as DABCO. Substrates with propargylic hydrogen undergo double bond isomerization at higher temperatures (70–100 °C) to form furan and pyrrole rings. Nonenolizable 1,3-diynones with an electron-withdrawing group provide good yields (72–86%) with most nucleophiles.
Introduction
Conjugated additions with non-organometallic reagents onto unsaturated carbonyl compounds constitute an important class of synthetic transformations among which 1,4-additions are the most pronounced process.1 Although less developed, metal-free 1,6-additions onto activated alkenyl π-systems are reported with a limited substrate scope.2 While 1,6-addition mainly engages alkenes, the corresponding reactions with alkynes have been sporadically reported.3
In this regard, we envisioned that the reactions of electron-deficient conjugated 1,3-diynes should critically depend not only on the steric and electronic factors of the substituents but also on the nature of the nucleophiles (Scheme 1).4 We recently reported on a novel protocol for 4-pyrone synthesis, which involves 1,4-addition of piperidine onto 1,3-diynone A to form intermediate 1N-A followed by 6-endo-dig cyclization and subsequent hydrolysis of an iminium intermediate to generate pyrone B (eqn (1)).5 This outcome contrasts the reported preference of the 1,6-addition of secondary amines including piperidine onto diynones.6 We speculated that a hydrogen bonding7 element at the propargylic position in C will ensure intermolecular 1,6-addition. This in turn would promote a tandem process8via intermediate IN-C, which should undergo an intramolecular 1,4-addition to generate product D (eqn (2)).
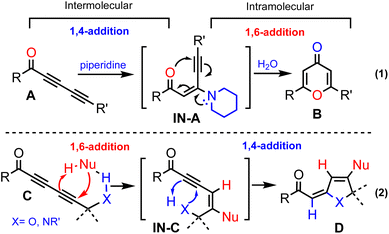 |
| Scheme 1 Sequential conjugate additions without or with a hydrogen bonding element. | |
The hydrogen bonding-directed switch from intermolecular 1,4-addition into 1,6-addition would in turn allow for the hydrogen bonding element to participate in an intramolecular 1,4-addition. This tandem sequence of bond-forming events would constitute a versatile approach for the construction of novel molecular scaffolds in step- and atom-economical manners.9 Moreover, the envisioned tandem conjugated additions do not require metal catalysts or organometallic reagents, which will provide unique merits to perform these reactions under mild conditions without rigorous exclusion of moisture and oxygen.
Results and discussion
We commenced our exploration of the hydrogen bonding effect on 1,6-addition with tert-hydroxyl-containing 1,3-diynone 1a and its TBS-protected form 1a-TBS (Table 1). Under identical conditions (3 equiv. of piperidine, toluene, rt), TBS-ether 1a-TBS was converted to 4-pyrone 2a-TBS (63%),5 whereas tert-hydroxyl-containing 1a provided 1,6-piperidine addition–cyclization product 2aa (78%).10 With this encouraging result, we continued to treat 1a with various nucleophiles including amines, alcohols, and thiols, which afforded products 2ab–2ai. A slight excess of amine is enough to provide good yields of the products without any external base whereas at least 3 equivalents of alcohols and DABCO (20 mol%)11 at higher temperatures (70–100 °C) are needed for comparable yields. 1,3-Propanediol participated in the reaction as a mono-nucleophile to generate product 2af (78%). Phenol and aniline addition products 2ah and 2ad were isolated in low yields (20% and 40%) due to the low nucleophilicity of both nucleophiles. N,N-Dimethylhydroxylamine generated the adduct 2ae (78%) at ambient temperature.12 On the other hand, 1a
13 reacted with thiophenol14 only at elevated temperature (100 °C) to provide the corresponding product 2ai (76%). The reaction with carboxylic acids did not afford the expected products; instead, pyrone 2aj′ was obtained15 and the yield depends on the structure of the carboxylic acid.
Table 1 OH-directed sequential 1,6/1,4-additions
Condition A: Nu–H (2 equiv.) in toluene at rt.
Condition B: Nu–H (2 equiv.), DABCO (20 mol%) in toluene at 100 °C.
Condition B but at 90 °C.
Condition B but with Nu–H (5 equiv.) at 70 °C.
Condition C: amine salt (2 equiv.) with Et3N (3 equiv.) in CH3CN at rt.
Other complexed by-products were formed. All reactions were performed using undistilled solvents and reagents.
|
|
After the initial screening of nucleophiles that readily undergo 1,6-additions with phenyl ketone-conjugated tert-alcohol 1a, we next turned our attention to the structural variation of the substituent on the ketone and at the propargylic carbon center (Table 2). The reactions of aryl ketones with tertiary (1b, 1c, 1d), secondary (1e), and primary (1f, 1g) propargylic hydroxyl groups, and alkyl ketones of ethyl (1h),13b isopropyl (1i), cyclohexyl (1j), and tert-butyl (1k) groups with a tertiary propargylic hydroxyl moiety revealed a salient reactivity trend. Aryl ketones 1b–f provided higher yields of amine-addition products 2ba–fa (68–81%) than those of alkyl ketone-derived products 2ha–ja (23–34%) with 2ka (76%) as an exception. This is most likely because of enolization when amines are used as a nucleophile, which is evidenced by isolation of 2ia′,17 which could be converted to 2ia upon heating.18 Reactions of aryl ketones with tert-propargylic carbon (1c and 1d) also provided higher yields of methanol addition products 2cb and 2db (85% and 77%) than those of alkyl ketones 1h–k (48–70%) indicating that electron withdrawing aryl groups make the substrate more electrophilic for conjugate addition than electron donating alkyl groups. The yields of amine addition products 2da–fa (76–81%) are not affected by the substituent at the propargylic carbon but the yield of methanol adducts 2db–fb (0–77%) drops as the steric bulk of the propargylic carbon decreases. The reaction of 1g with N-boc-protected cysteine methyl ester14g at high temperature (70–75 °C) gave a sulfide-containing furan 2ga
16 (50%) along with 1,6-adduct 2ga′ (12%). Also, it is evident that at higher temperatures substrates with propargylic H, 1e and 1g, undergo double bond isomerization to form more stable furan ring containing products 2eb and 2ga. Unlike 1,3-propanediol, 1,3-propanedithiol reacts as a dinucleophile generating a mixture of vinyl sulfide 2hc (10%) and dithioketal 2hc′ (20%) in a 1
:
2 ratio.
Table 2 OH-directed sequential 1,6/1,4-additions with structural variation on 1,3-diynones
Condition A: Nu–H (2 equiv.) in toluene at rt.
Condition B: Nu–H (2 equiv.), DABCO (20 mol%) in toluene at 100 °C.
Condition B but at 90 °C.
Condition B but with Nu–H (5 equiv.) at 70 °C.
Mixed with a double bond isomerized furan product in a 5 : 1 ratio.
Dimerized compound 2ia′ was isolated in 18% yield.
Polymerized products were observed.
1f decomposed. All reactions were performed using undistilled solvents and reagents.
|
|
Next, we examined the 1,6-addition directed by hydrogen bonding with –HNBoc and –HNTs moieties (Table 3).19 When 1l and 1m containing a primary propargylic –HNBoc moiety were reacted with piperidine, the corresponding addition products 2la and 2ma were obtained in slightly higher yields (81% and 86%) than 2oa and 2pa (70% and 71%) containing a propargylic tert-NHTs moiety. But a significant difference was observed with ephedrine, where the product with 1o was obtained in trace amounts. This discrepancy is likely to be the consequence of increased steric hindrance caused by both the gem-dimethyl moiety and the tosyl group when relatively bulky secondary amines approach the reaction center. The reactions of 1l and 1m with benzylamine, methanol, thiophenol, and Boc-protected cysteine methyl ester generated the corresponding products 2mb, 2lb, 2mc, and 2md in 44–72% yields. It is worth noting that 2mc and 2md are obtained exclusively as pyrrole derivatives due to double bond isomerization. Compared to the failed reaction with a primary propargylic alcohol 1f in generating methanol adduct 2fb, the corresponding primary Boc-bearing propargyl amine 1l (R = H) generated 2lb although in a marginal yield (45%). The reaction of 1o and 1p containing a propargylic tert-NHTs moiety with methanol, ethanol and propane-1,3-diol provided 2oc, 2pb, and 2pc (61–80%) in good yields. The reaction of 1p with 1,3-propanedithiol generated a mixture of vinyl sulfides 2pd (23%) and dithioketal 2pd′ (47%) in a 1
:
2 ratio. The reaction of 1,3-diynone with a propargylic tert-NHBoc moiety gave trace amounts of products with piperidine and ephedrine, whereas with methanol, it formed a 4-pyrone product.
Table 3 NH-directed sequential 1,6/1,4-additions
Condition A: Nu–H (2 equiv.) in toluene at rt.
Condition B: Nu–H (2 equiv.), DABCO (20 mol%) in toluene at 100 °C.
Condition B but at 90 °C.
Condition B but with Nu–H (5 equiv.) at 70 °C.
Mixed with a double bond isomerized furan product in a 5 : 1 ratio. All reactions were performed using undistilled solvents and reagents.
|
|
We further explored the hydrogen bonding effect on the 1,6-addition with tert-hydroxyl-containing 1,3-diynoate 1q and 1,3-diynimide 1r (Table 4). Both 1q and 1r reacted with piperidine and β-alanine ethyl ester salt to generate the corresponding products 2q and 2r (71% and 77%) in good yields. But the reactions with phenylalanine methyl ester salt, phenylglycine methyl ester salt, and N-Boc-protected cysteine methyl ester provided moderate yields (50–62%), whereas the reaction with proline benzyl ester generated 2qd (40%) in a low yield due to the increased steric hindrance. The reaction of 1q and 1r with methanol provided the 1,4-methanol addition product where the imide group of 1r was also replaced by methanol.
Table 4 OH-directed sequential 1,6/1,4-additions of conjugated esters and imides
Condition A: Nu–H (2 equiv.) in toluene at rt.
Condition B: Nu–H (5 equiv.), DABCO (20 mol%) in toluene at 80 °C.
Condition C: amine salt (2 equiv.) with Et3N (3 equiv.) in CH3CN at rt. All reactions were performed using undistilled solvents and reagents.
|
|
The effects of propargylic and homopropargylic hydroxyl groups on the efficiency of 1,6-addition and 1,4-addition are compared with probes 1s–u (Scheme 2). Treating diol 1s with piperidine (2 equiv., toluene, rt) provided only 5-membered ring product 2sa (65%); the corresponding 6-membered ring adduct 2sa′ was not observed.20 Primary and tertiary homopropargylic substrates 1t and 1u were treated with piperidine under forcing conditions. Although the expected product 2ta could not be obtained, 2ua was obtained in a 20% yield. This clearly demonstrates that the hydrogen bonding element at the propargylic position is crucial for the effective 1,6-addition of nucleophiles.
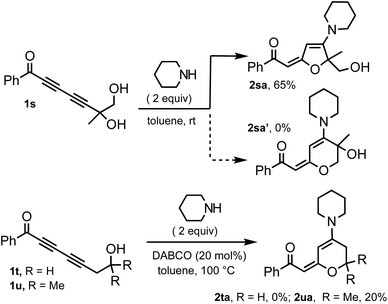 |
| Scheme 2 Probing the effect of a hydrogen bonding element at the homopropargylic position. | |
Conclusions
We describe sequential inter- and intramolecular 1,6- and 1,4-addition with electron-deficient 1,3-diynes that are conjugated with an electron-withdrawing group such as a ketone, ester, or imide functionality. These addition processes are directed by hydrogen bonding elements such as –OH, –NHTs and –NHBoc moieties at the propargylic position. The initial 1,6-addition renders these hydrogen bonding elements to become an internal nucleophile for the subsequent intramolecular 1,4-addition to generate the final products. Alcohols, amines, and thiols show excellent reactivity for the initial 1,6-addition, among which amines are the most reactive and their addition occurs at ambient temperature. On the other hand, alcohols and thiols require a base additive such as DABCO and higher temperatures (70–100 °C). Tertiary, secondary and primary propargylic –OH and –NHR functional groups are effective promoters for the sequential 1,6/1,4-additions although primary alcohols have a limited scope. 1,3-Diyne substrates conjugated with aromatic ketones display better yields with amines whereas alkyl ketones resulted in relatively lower yields due to enolization. The hydrogen bonding-directed sequential 1,6/1,4-additions developed herein allow for creating unique heterocycles with structural diversity under mild conditions without using metal catalysts or organometallic reagents. Moreover, the hydrogen bonding-based control for reaction pathways allows for the development of many unprecedented tandem reactions which will be reported in due course.
Conflicts of interest
The authors declare no competing financial interests.
Acknowledgements
The authors thank the Donors of the American Chemical Society Petroleum Research Fund for financial support. The Mass Spectrometry Laboratory at UIUC is acknowledged.
References
- Reviews on conjugate additions of non-organometallic reagents.
(a) S. B. Tsogoeva, Recent Advances in Asymmetric Organocatalytic 1,4-Conjugate Additions, Eur. J. Org. Chem., 2007, 1701 CrossRef CAS;
(b) D. Almasxi, D. A. Alonso and C. Ńajera, Organocatalytic asymmetric conjugate additions, Tetrahedron: Asymmetry, 2007, 18, 299 CrossRef;
(c) J. L. Vicario, D. Badía and L. Carrillo, Organocatalytic Enantioselective Michael and Hetero-Michael Reactions, Synthesis, 2007, 2065 CrossRef CAS.
- 1,6-Additions: a review:
(a) A. G. Csákÿ, G. de la Herrán and M. C. Murcia, Conjugate addition reactions of carbon nucleophiles to electron-deficient dienes, Chem. Soc. Rev., 2010, 39, 4080 RSC A highlight:
(b) A. T. Biju, Organocatalytic Asymmetric 1,6-Addition Reactions, ChemCatChem, 2011, 3, 1847 CrossRef CAS;
(c) D. Uraguchi, K. Yoshioka, Y. Ueki and T. Ooi, Highly Regio-, Diastereo-, and Enantioselective 1,6- and 1,8-Additions of Azlactones to Di- and Trienyl N-Acylpyrroles, J. Am. Chem. Soc., 2012, 134, 19370 CrossRef CAS PubMed;
(d) T. Gallagher, I. Derrick, P. M. Durkin, C. A. Haseler, C. Hirschhauser and P. Magrone, Intramolecular 1,6-Addition to 2-Pyridones. Mechanism and Synthetic Scope, J. Org. Chem., 2010, 75, 3766 CrossRef CAS;
(e) L. Bernardi, J. López-Cantarero, B. Niess and K. A. Jørgensen, Organocatalytic Asymmetric 1,6-Additions of β-Ketoesters and Glycine Imine, J. Am. Chem. Soc., 2007, 129, 5772 CrossRef CAS;
(f) J. J. Murphy, A. Quintard, P. McArdle, A. Alexakis and J. C. Stephens, Asymmetric to Dienic Sulfones, Angew. Chem., Int. Ed., 2011, 50, 5095 CrossRef CAS PubMed;
(g) X. Tian, Y. Liu and P. Melchiorre, Aminocatalytic Enantioselective 1,6-Additions of Alkyl Thiols to Cyclic Dienones: Vinylogous Iminium Ion Activation, Angew. Chem., Int. Ed., 2012, 51, 6439 CrossRef CAS Examples with transition metal catalysts:
(h) T. Nishimura, Y. Yasuhara and T. Hayashi, Highly Selective 1,6-Addition of Aryl BoronicAcids to a,b,g,d-Unsaturated Carbonyl Compounds Catalyzed by an Iridium Complex, Angew. Chem., Int. Ed., 2006, 45, 5164 CrossRef CAS;
(i) T. den Hartog, S. R. Harutyunyan, D. Font, A. J. Minnaard and B. L. Feringa, Catalytic Enantioselective 1,6-Conjugate Addition of Grignard Reagents to Linear Dienoates, Angew. Chem., Int. Ed., 2008, 47, 398 CrossRef CAS.
- A review of transformations with activated alkynes:
(a) L. Wang, H. Zhu, T. Peng and D. Yang, Conjugated ynones in catalytic enantioselective reactions, Org. Biomol. Chem., 2021, 19, 2110 RSC;
(b) M. Bella and K. A. Jørgensen, Organocatalytic Enantioselective Conjugate Addition to Alkynones, J. Am. Chem. Soc., 2004, 126, 5672 CrossRef CAS;
(c) D. Uraguchi, Y. Ueki, A. Sugiyama and T. Ooi, Highly stereoselective Michael addition of azlactones to electron-deficient triple bonds under g-spiro chiral iminophosphorane catalysis: importance of protonation pathway, Chem. Sci., 2013, 4, 1308 RSC;
(d) X. Wang, M. Kitamura and K. Maruoka, New, Chiral Phase Transfer Catalysts for Effecting Asymmetric Conjugate Additions of α-Alkyl-α-cyanoacetates to Acetylenic Esters, J. Am. Chem. Soc., 2007, 129, 1038 CrossRef CAS;
(e) T. Misaki, T. Kawano and T. Sugimura, Highly Z-Selective Asymmetric 1,4-Addition Reaction of 5H-Oxazol-4-ones with Alkynyl Carbonyl Compounds Catalyzed by Chiral Guanidines, J. Am. Chem. Soc., 2011, 133, 5695 CrossRef CAS PubMed.
- The addition of heteroatom-based nucleophiles to electron-deficient alkynes:
(a) J. C. Worch, C. J. Stubbs, M. J. Price and A. P. Dove, Click Nucleophilic Conjugate Additions to Activated Alkynes: Exploring Thiol-yne, Amino-yne, and Hydroxyl-yne Reactions from (Bio)Organic to Polymer Chemistry, Chem. Rev., 2021, 121, 6744 CrossRef CAS PubMed;
(b) G. Büchi and D. E. Vogel, Preparation and Rearrangement of trans-3-(Allyloxy)acrylie Acids: A Claisen Sequence That Avoids Mercury Catalysis, J. Org. Chem., 1983, 48, 5406 CrossRef;
(c) Y. Sarrafi, M. Sadatshahabi, K. Alimohammadi and M. Tajbakhsh, A green and rapid approach for the stereoselective vinylation of phenol, thiol and amine derivatives in water, Green Chem., 2011, 13, 2851 RSC;
(d) A. W. McCulloch and A. G. McInnes,
Can. The Reaction of Propiolic Acid Esters with Tertiary Amines, J. Chem., 1974, 52, 3569 CAS;
(e) C. W. Downey, S. Craciun, C. Vivelo, A. M. Neferu, C. J. Mueller, B. Southall and S. Corsi, One-pot synthesis of (Z)-β-sulfonyl enoates from ethyl propiolate, Tetrahedron Lett., 2012, 53, 5763 CrossRef CAS;
(f) A. W. Feldman, S. I. Ovaska and T. V. Ovaska, Facile access to cyclooctanoid ring systems via microwave-assisted tandem 6-exo dig, cyclization–rearrangement sequence, Tetrahedron, 2014, 70, 4147 CrossRef CAS PubMed Alkoxide addition onto unactivated alkynes:
(g) X. Li, R. E. Kyne and T. V. Ovaska, Synthesis of Seven-Membered Carbocyclic Rings via a Microwave-Assisted Tandem Oxyanionic 5-exodig, Cyclization−Claisen Rearrangement Process, J. Org. Chem., 2007, 72, 6624 CrossRef CAS PubMed;
(h) X. Li, A. E. Keon, J. A. Sullivan and T. V. Ovaska, Org. Lett., 2008, 10, 3287 CrossRef CAS;
(i) T. V. Ovaska, J. A. Sullivan, S. I. Ovaska, J. B. Winegrad and J. D. Fair, Studies toward Frondosin A and Its Analogues. Formal Total Synthesis of (±)-Frondosin A, Org. Lett., 2009, 11, 2715 CrossRef CAS;
(j) X. Li and T. V. Ovaska, Total Synthesis of (±)-Frondosin B, Org. Lett., 2007, 9, 3837 CrossRef CAS PubMed;
(k) W. C. DeLomba, E. A. Stone, K. A. Alley, V. Iannarone, E. Tarsis, S. I. Ovaska and T. V. Ovaska, Utilization of the Thorpe–Ingold Effect in the Synthesis of Cyclooctanoid Ring Systems via Anionic 6-exo-dig, Cyclization/Claisen Rearrangement Sequence, J. Org. Chem., 2020, 85, 9464 CrossRef CAS PubMed Nitrogen and sulfur nucleophile addition:
(l) S. Roy, M. P. Davydova, R. Pal, K. Gilmore, G. A. Tolstikov, S. F. Vasilevsky and I. V. Alabugin, Dissecting Alkynes: Full Cleavage of Polarized C
C Moiety via Sequential Bis-Michael Addition/Retro-Mannich Cascade, J. Org. Chem., 2011, 76, 7482 CrossRef CAS;
(m) D. S. Baranov, B. Gold, S. F. Vasilevsky and I. V. Alabugin, Divergent Cyclizations of 1-R-Ethynyl-9,10-anthraquinones: Use of Thiourea as a “S2−” Equivalent in an “Anchor-Relay” Addition Mediated by Formal C–H Activation, J. Org. Chem., 2013, 78, 2074 CrossRef CAS PubMed A review of metal-catalyzed addition of oxygen nucleophiles on alkynes:
(n) J. A. Goodwin and A. Aponick, Regioselectivity in the Au-catalyzed hydration and hydroalkoxylation of alkynes, Chem. Commun., 2015, 51, 8730 RSC.
- E. Liyanage Perera and D. Lee, Synthesis of 4-Pyrones by Formal Hydration of 1,3-Diynones Promoted by 1,4-Addition of Piperidine, Org. Lett., 2022, 24, 7042 CrossRef CAS.
-
(a) N. H. Cromwell and I. H. Witt, α, β-Unsaturated Aminoketones. VII.1 Reaction of Piperidine and N-Methylbenzylamine with Bromine Derivatives of Benzalacetone and Benzalacetophenone, J. Am. Chem. Soc., 1943, 65, 308 CrossRef CAS;
(b) Y. Kishida, T. Hiraoka, M. Yoshimoto and M. Studies, on Acetylenic Compounds. XLIX. Reactions of Linear-conjugated Diynones, Chem. Pharm. Bull., 1969, 17, 2126 CrossRef CAS;
(c) R. Mestres, Addition of secondary amines to diacetylenic ketones, J. Chem. Soc. Perkin 1, 1972, 805 RSC;
(d) E. A. Hamed, S. M. Sharaf, S. A. Abdel-Baky, M. F. Ibrahim and A. H. A. Youssef, Stereochemistry and kinetics of addition of amines to acetylenic ketones, J. Phys. Org. Chem., 1990, 3, 627 CrossRef CAS;
(e) S. S. Palimkar, V. S. More and K. V. Srinivasan, Simple and Efficient One–Pot, Three–Component, Solvent–Free Synthesis of β–Enaminones via Sonogashira Coupling–Michael Addition Sequences, Synth. Commun., 2008, 38, 1456 CrossRef CAS;
(f) D. R. Chisholm, R. Valentine, E. Pohl and A. Whiting, Conjugate Addition of 3-Buytn-2-one to Anilines in Ethanol: Alkene Geometric Insights through In Situ FTIR Monitoring, J. Org. Chem., 2016, 81, 7557 CrossRef CAS;
(g) M. Sengee and L. K. Sydnes, Michael Addition of Various Nitrogen and Oxygen Nucleophiles to 1,1-Diethoxybut-3-yn-2-one, Synthesis, 2011, 3899 CAS;
(h) H. Li, X. Xu, J. Yang, X. Xie, H. Huang and Y. Li, Iron-catalyzed cascade reaction of ynone with o-aminoaryl compounds: a Michael addition–cyclization approach to 3-carbonyl quinolines, Tetrahedron Lett., 2011, 52, 530 CrossRef CAS;
(i) Q. Zhu, Z. Ye, W. Yang, X. Cai and B. Z. Tang, One-Pot Synthesis and Structure–Property Relationship of Aminomaleimides: Fluorescence Efficiencies in Monomers and Aggregates Easily Tuned by Switch of Aryl and Alkyl, J. Org. Chem., 2017, 82, 1096 CrossRef CAS;
(j) X. Hu, X. Zhao, B. He, Z. Zhao, Z. Zheng, P. Zhang, X. Shi, R. T. K. Kwok, J. W. Y. Lam, A. Qin and B. Z. Tang, A Simple Approach to Bioconjugation at Diverse Levels: Metal-Free Click Reactions of Activated Alkynes with Native Groups of Biotargets without Prefunctionalization, Research, 2018, 3152870 Search PubMed;
(k) Y. Zhao, Z. Zhang, X. Liu, Z. Wang, Z. Cao, L. Tian, M. Yue and J. You, TBAF-Catalyzed O-Nucleophilic Cyclization of Enaminones: A Process for the Synthesis of Dihydroisobenzofuran Derivatives, J. Org. Chem., 2019, 84, 1379 CrossRef CAS PubMed;
(l) Y. Zhang, J. Shen, R. Hu, X. Shi, X. Hu, B. He, A. Qin and B. Z. Tang, Fast surface immobilization of native proteins through catalyst-free amino-yne click bioconjugation, Chem. Sci., 2020, 11, 3931 RSC.
- A review of hydrogen bonding-promoted transformations:
(a) A. G. Doyle and E. N. Jacobsen, Small-Molecule H-Bond Donors in Asymmetric Catalysis, Chem. Rev., 2007, 107, 5713 CrossRef CAS Hydrogen bond activation of dienophiles in standard DA reactions:
(b) T. R. Kelly, P. Meghani and V. S. Ekkundi, Diels-alder reactions: Rate acceleration promoted by a biphenylenediol, Tetrahedron Lett., 1990, 31, 3381 CrossRef CAS;
(c) P. R. Schreiner and A. Wittkopp, H-Bonding Additives Act Like Lewis Acid Catalysts, Org. Lett., 2002, 4, 217 CrossRef CAS;
(d) Y. Huang and V. H. Rawal, Hydrogen-Bond-Promoted Hetero-Diels-Alder Reactions of Unactivated Ketones, J. Am. Chem. Soc., 2002, 124, 9662 CrossRef CAS;
(e) Y. Huang, A. K. Unni, A. N. Thadani and V. H. Rawal, Single enantiomers from a chiral-alcohol catalyst, Nature, 2003, 424, 146 CrossRef CAS PubMed;
(f) A. K. Unni, N. Takenaka, H. Yamamoto and V. H. Rawal, Axially Chiral Biaryl Diols Catalyze Highly Enantioselective Hetero-Diels-Alder Reactions through Hydrogen Bonding, J. Am. Chem. Soc., 2005, 127, 1336 CrossRef CAS PubMed.
- Tandem reactions initiated by a conjugate addition:
(a) C. Grondal, M. Jeanty and D. Enders, Organocatalytic cascade reactions as a new tool in total synthesis, Nat. Chem., 2010, 2, 167 CrossRef CAS PubMed;
(b) D. Roca-Lopez, D. Sadaba, I. Delso, R. P. Herrera, T. Tejero and P. Merino, Asymmetric organocatalytic synthesis of g-nitrocarbonyl compounds through Michael and Domino reactions, Tetrahedron: Asymmetry, 2010, 21, 2561 CrossRef CAS;
(c) L. Y. Wu, G. Bencivenni, M. Mancinelli, A. Mazzanti, G. Bartoli and P. Melchiorre, Organocascade Reactions of Enones Catalyzed by a Chiral Primary Amine, Angew. Chem., Int. Ed., 2009, 48, 7196 CrossRef CAS PubMed;
(d) Y. Wang and D. M. Du, Recent advances in organocatalytic asymmetric oxa-Michael addition triggered cascade reactions, Org. Chem. Front., 2020, 7, 3266 RSC Examples with unactivated 1,3-diynes:
(e) L. Zhang and X. Zhao, Regioselective Formation of 2,4,5-Trisubstituted Oxazoles through Transition-Metal Free Heterocyclization of 1,3-Diynes with N,O-Bis(trimethylsiyl)acetamide, Org. Lett., 2015, 17, 184 CrossRef CAS;
(f) L. Zhang, M. Zhao and X. Zhao, The synthesis of carbonyl 2-amino-pyrimidines via tandem regioselective heterocyclization of 1,3-diynes with guanidine and selective oxidation, Chem. Commun., 2015, 51, 9370 RSC.
-
(a)
T. L. Ho, Tandem organic reactions, Wiley, New York, 1992 Search PubMed;
(b) L. F. Tietze, Domino Reactions in Organic Synthesis, Chem. Rev., 1996, 96, 115 CrossRef CAS A review of the oxa-Michael reaction:
(c) Y. Wang and D. M. Du, Recent advances in organocatalytic asymmetric oxa-Michael addition triggered cascade reactions, Org. Chem. Front., 2020, 7, 3266 RSC.
- A review on base-mediated hydroamination with unactivated alkynes: M. Patel, R. K. Saunthwal and A. K. Verma, Base-Mediated Hydroamination of Alkynes, Acc. Chem. Res., 2017, 50, 240 CrossRef CAS PubMed.
- A review on the addition of nucleophiles in the presence of DABCO: D. K. Jangid, DABCO as a Base and an Organocatalyst in Organic Synthesis: A Review, Curr. Green Chem., 2020, 7, 146 CrossRef.
- Selected reactions of unactivated 1,3-diynes:
(a) L. Wang, X. Yu, X. Feng and M. Bao, Synthesis of 3,5-Disubstituted Isoxazoles via Cope-Type Hydroamination of 1,3-Dialkynes, Org. Lett., 2012, 14, 2418 CrossRef CAS PubMed For the Cope-type hydroamination reaction of alkynes, see:
(b) J. Moran, S. I. Gorelsky, E. Dimitrijevic, M. E. Lebrun, A. C. Bedard, C. Seguin and A. M. Beauchemin, Intermolecular Cope-Type Hydroamination of Alkenes and Alkynes using Hydroxylamines, J. Am. Chem. Soc., 2008, 130, 17893 CrossRef CAS PubMed;
(c) A. M. Beauchemin, J. Moran, M. E. Lebrun, A. C. Bedard, C. Seguin, E. Dimitrijevic, L. Zhang and S. I. Gorelsky, Intermolecular Cope-Type Hydroamination of Alkenes and Alkynes, Angew. Chem., Int. Ed., 2008, 47, 1410 CrossRef CAS PubMed;
(d) G. Zhou, X. Zhao and W. Dan, Synthesis of 2,3,6-trisubstituted pyridines by transition-metal free cyclization of 1,3-diynes with amino acids, Tetrahedron Lett., 2017, 58, 3085 CrossRef CAS.
-
(a) S. N. Danilov, L. N. Yastrebov, A. L. Galka and A. S. Zanina, Kinetics of the reaction of hexyl azide with mono- and disubstituted acetylene compounds, Org. Khim., 1979, 15, 1146 CAS;
(b) L. I. Vereshchagin, S. R. Gainulina and L. P. Kirillova, Biol. Akt. Soedin., 1968, 154 CAS.
- For selected examples of addition of thiols to alkynes, see:
(a) W. E. Truce and G. J. W. Tichenor, Effect of Activating Group on Trans Stereoselectivity of Thiolate Additions to Activated Acetylenes, J. Org. Chem., 1972, 37, 2391 CrossRef CAS;
(b) O. De Lucchi, V. Lucchini, C. Marchioro, G. Valle and G. Modena, Self-induced diastereoselective oxidation of vinyl sulfoxides bearing a chiral hydroxy group as a way of preparation of optically active sulfinyl dienophiles and their use in the asymmetric Diels-Alder reactions to cyclopentadiene, J. Org. Chem., 1986, 51, 1457 CrossRef CAS;
(c) M. Journet, A. Rouillard, D. Cai and R. D. Larsen, Double Radical Cyclization/β-Fragmentation of Acyclic ω-Yne Vinyl Sulfides. Synthesis of 3-Vinyldihydrothiophene and Dihydrothiopyran Derivatives. A New Example of a 5-endo-trig Radical Cyclization, J. Org. Chem., 1997, 62, 8630 CrossRef CAS;
(d) O. Arjona, R. Medel, J. Rojas, A. M. Costa and J. Vilar-rasa, Chemoselective protection of thiols versus alcohols and phenols. The Tosvinyl group, Tetrahedron Lett., 2003, 44, 6369 CrossRef CAS;
(e) J. C. Henise and J. Taunton, Irreversible Nek2 Kinase Inhibitors with Cellular Activity, J. Med. Chem., 2011, 54, 4133 CrossRef CAS PubMed;
(f) R. Ekkebus, S. I. van Kasteren, Y. Kulathu, A. Scholten, I. Berlin, P. P. Geurink, A. de Jong, S. Goerdayal, J. Neefjes, A. J. R. Heck, D. Komander and H. Ovaa, On Terminal Alkynes That Can React with Active-Site Cysteine Nucleophiles in Proteases, J. Am. Chem. Soc., 2013, 135, 2867 CrossRef CAS PubMed;
(g) P. Fricero, L. Bialy, W. Czechtizky, M. Méndez and J. P. A. Harrity, Org. Lett., 2018, 20, 198 CrossRef CAS PubMed;
(h) T. Cheng, W. Huang, D. Gao, Z. Yang, C. Zhang, H. Zhang, J. Zhang, H. Li and X.-F. Yang, Michael Addition/S,N-Intramolecular Rearrangement Sequence Enables Selective Fluorescence Detection of Cysteine and Homocysteine, Anal. Chem., 2019, 91, 10894 CrossRef CAS PubMed;
(i) K. McAulay, E. A. Hoyt, M. Thomas, M. Schimpl, M. S. Bodnarchuk, H. J. Lewis, D. Barratt, D. Bhavsar, D. M. Robinson, M. J. Deery, D. J. Ogg, G. J. L. Bernardes, R. A. Ward, M. J. Waring and J. G. Kettle, Alkynyl Benzoxazines and Dihydroquinazolines as Cysteine Targeting Covalent Warheads and Their Application in Identification of Selective Irreversible Kinase Inhibitors, J. Am. Chem. Soc., 2020, 142, 10358 CrossRef CAS PubMed.
- The identity of pyrone 2al′ was established by comparison with 2a-TBS. For a plausible mechanism, see: M. J. Fan, G. Q. Li and Y. M. Liang, DABCO-catalyzed reaction of various nucleophiles with activated alkynes leading to the formation of alkenoic acid esters, 1,4-dioxane, morpholine, and piperazinone derivatives, Tetrahedron, 2006, 62, 6782 CrossRef CAS Alternatively, under acidic conditions 1,6/14-adduct 2al rearranges to pyrone 2al′, see: T. H. Nguyen, V. J. T. Anders, Q. Ma, R. Herbs-Irmer and P. Langer, Synthesis of 5-alkylidene-2,5-dihydropyrrol-2-ones and their ring-transformation into 5,6-dihydrobenzo[h]chromones, 5,6,7,8-tetrahydrochromones and pyran-4-ones, Tetrahedron, 2007, 63, 12975 CrossRef.
- An example of intramolecular nucleophilic addition followed by proton transfer to form an oxazole moiety: P. Wipf, Y. Aoyama and T. E. Benedum, A Practical Method for Oxazole Synthesis by Cycloisomerization of Propargyl Amides, Org. Lett., 2004, 6, 3593 CrossRef CAS PubMed.
- Related dimerization of alkynyl ketones in the presence of DABCO:
(a) P. V. Ramachandran, M. T. Rudd and V. R. Reddy, 1,4-diazabicyclo[2.2.2]octane-catalyzed self- and cross-condensation of α-acetylenic ketones, Tetrahedron Lett., 1999, 40, 3819 CrossRef CAS;
(b) A. V. Mareev, M. V. Andreev and I. A. Ushakov, Base-Catalyzed Hydration of Silicon-Containing Activated Alkynes: The Effect of Substituents at the Triple Bond, ChemistrySelect, 2020, 5, 10736 CrossRef CAS.
- The mechanism involves the 1,6-addition of piperidine with 2ja′ followed by elimination of the enolate moiety.
- Substrates containing a free amino group (–NH2) could not be prepared because of the instability.
- The identity of 2sa was established by converting it to its acetate, which shows a chemical shift change of the methylene protons to a lower field (from 3.87 to 4.39 ppm). No sizable chemical shift change is expected by acetylation if it is 2sa′.
|
This journal is © the Partner Organisations 2023 |
Click here to see how this site uses Cookies. View our privacy policy here.