DOI:
10.1039/D2RA06560F
(Paper)
RSC Adv., 2023,
13, 4195-4201
Efficient analyses of triazole fungicides in water, honey and soy milk samples by popping candy-generated CO2 and sugaring-out-assisted supramolecular solvent-based microextraction prior to HPLC determinations
Received
18th October 2022
, Accepted 18th January 2023
First published on 31st January 2023
Abstract
An enrichment method, namely popping candy-generated CO2 and sugaring-out-assisted supramolecular solvent-based microextraction (PGS-SUPRA), was investigated for the determination of triazole fungicide residues in water, honey and soy milk samples. The extraction process was carried out by adding popping candies into a centrifuge tube. Consequently, rapid dispersion and mass transfer of extractants can be achieved without using dispersants and auxiliary devices, and therefore, the extraction efficiency increased. The extraction parameters affecting the efficiency of the developed method were investigated. The presented method was then analysed by high-performance liquid chromatography. Under the selected condition, the wide linearity of triazole fungicides after preconcentration by the proposed microextraction method ranged from 30 to 1000 μg L−1 for triadimefon and from 90 to 1000 μg L−1 for myclobutanil, tebuconazole and hexaconazole, with a coefficient for determination (R2) greater than 0.992. The limits of detection (LODs) and limits of quantitation (LOQs) were in the range of 10–30 μg L−1 and 30–90 μg L−1, respectively. The precisions were assessed from the relative standard deviations (RSDs) of the retention time and peak area obtained from intra- (n = 3) and inter-day (n = 3 × 5) experiments, and were greater than 1.66% and 13.52%, respectively. Moreover, the proposed method provided high enhancement factors (EnFs) ranging from 14 to 51 folds. This technique has been prosperously applied for the extraction of fungicide residues in water, honey and soy milk samples with a recovery within the range of 60–114%. Overall, the developed method was found to be advantageous as compared with other sample preparation methods.
Introduction
Fungicides, such as triazoles, are used to control fungal pathogens in agricultural production in order to increase the quality of crops.1 Triazole fungicides (TFs) are a class of highly effective systemic fungicides, in which the main chain consists of a hydroxyl group (ketone group), a substituted phenyl group and a 1,2,4-triazole group.2 They are difficult to degrade in a short period of time.3 Their residues can cause harm to the environment and human beings when consumed.4 In order to reduce the harmfulness of pesticide residues, the permissible level in food is usually defined by the maximum residue limit (MRL). The MRL established by the EU for hexaconazole and triadimefon is 0.01–0.02 mg kg−1; tebuconazole is 0.02–5.0 mg kg−1; and myclobutanil is 0.05–3.0 mg kg−1.5 Consequently, it is necessary to establish a simple and effective method for the determination of triazole fungicides from the matrices of agricultural products.
Owing to their extremely low concentration levels and real-sample matrices, it is difficult to detect triazoles by direct analysis using various instruments. A proper sample preparation technique is inevitable to preconcentrate target analytes and matrice removal, which provides high accuracy and reliability of the results.6 Miniaturized sample preparation is a new trend for green analytical chemistry because it reduces or eliminates the use of volatile organic solvents. Alternative solvents, namely supramolecular solvents (SUPRASs), have been widely used instead of organic solvents, due to their high ability to extract various kinds of substances7 in real-sample matrices. The SUPRASs are generated via two steps, namely, aggregation of amphiphiles providing supramolecular association in homogeneous solutions and coacervation producing water-immiscible liquids (or SUPRAS phase) that separate from bulk solutions.8 The SUPRAS extraction using surfactants is called cloud-point extraction (CPE), where the coacervation involves neutrally charged (non-ionic or zwitterionic) surfactants, or coacervative extraction (CAE), where coacervation involves ionic amphiphiles. Although these techniques are simple, cost-effective, and rapid and consume less organic solvents, there are some limitations of gas chromatographic systems.
To enhance the dispersion and mass transfer of fine droplets of extraction solvents into an aqueous solution, various agitators were used for the extraction process including ultrasound,9 vortex,10 magnetic stirrers,11 in-syringe12 and air-agitators.13 Very recently, popping candy-assisted dispersive liquid–liquid microextraction has been investigated.14 In this method, the popping candy, which is produced from a mixture of sugar and high-pressure-compressed carbon dioxide, was investigated as a solid dispersant in the extraction process. No dispersant, which usually uses acetonitrile, methanol, and acetone as liquid dispersants, is necessary. In this method, the extractant disperses into the sample solution and in situ releases carbon dioxide from the popping candy, and the dispersion of extractants can be achieved without using any auxiliary devices. Moreover, the mass transfer of analytes into extractants is increased due to sugaring-out effects. Sugaring-out may provide an entirely new platform for the extraction of organic compounds and other products from the aqueous phase.15 Consequently, it would be interesting to couple such approach to the chromatographic technique. The application for the popping candy in sample preparation was first reported by Jing et al.14 for the determination of prothioconazole and its chiral metabolite in water, beer, baijiu, and vinegar. In this work, a high temperature (90 °C) was applied.
The present study is focused on the development of a simple, fast, and environmentally friendly pre-treatment technique for the determination of triazole fungicide residues by popping candy-generated CO2 and sugaring-out-assisted supramolecular solvent-based microextraction combined with HPLC. A supramolecular solvent base, tributyl dodecyl phosphonium bromide ([P44412]Br), as the starting extraction solvent was used to avoid the use of toxic extractants, and the popping candy was used as a solid dispersant and sugaring-out effect to disperse fine droplets of the extractant into the sample solution. The dispersion of extractants can be achieved under mild conditions without any auxiliary devices. The phase separation was achieved after the centrifugation step. The factors affecting the extraction efficiency were optimized and the proposed method was applied to analyze complex sample matrices. The proposed method was compared with other extraction methods reported previously.
Experimental
Chemicals and reagents
All chemicals used were at least of analytical grade. Triazole standards including myclobutanil (MCBT), triadimefon (TDF), tebuconazole (TBZ), and hexaconazole (HCZ), were purchased from Dr Ehren-storfer GmbH (Germany). The stock standard solution of each triazole (1000 mg L−1) were prepared by dissolving an appropriate amount in methanol and stored in a refrigerator at 4 °C. Methanol and acetonitrile of HPLC-grade were obtained from Merck (Darmstadt, Germany). 1-Dodecanol (Sigma-Aldrich, Germany) and tributyldodecylphosphonium bromide (P44412Br) (Sigma-Aldrich, Switzerland) were used. Popping candies were obtained from local markets in Maha Sarakham, (Thailand). Type I deionized water (18.2 MΩ cm) used throughout this work was prepared using a RiOs simplicity 185 water purification system (Millipore, USA). Before subjecting to the HPLC system, all reagents were filtered through a 0.45 μm membrane filter.
Instruments
High-performance liquid chromatographic analysis was performed using a waters 1525 binary HPLC pump (Water, Massachusetts, USA) equipped with an in-line degasser and a waters 2489 UV/visible detector. A rheodyne injector was equipped. The Empower 3 software was used to analyze the chromatographic data. Separation was performed using a Purospher® STAR RP-18 endcapped (4.6 × 150 mm, 5 μm) column (Merck, Germany) at ambient temperature with a mobile phase comprising acetonitrile and water (50
:
50, v/v) at a flow rate of 1.0 mL min−1. The detection wavelength was set at 220 nm with an injection volume of 20 μL. Furthermore, a centrifuge (Centurion, England) was used for phase separation.
Popping candy-generated CO2 and sugaring-out-assisted supramolecular solvent-based microextraction (PGS-SUPRA) method
A mixed standard/sample solution (10 mL) was placed into a centrifuge tube containing 0.5 g of popping candy (solid sugaring-out reagent) and 0.1 g of P44412Br. Then, the solution was mixed well until the popping candy was dissolved. Then, 100 μL of 1-dodecanol was added. A supramolecular solvent was in situ generated and then dispersed into the sample solution within 20 s by using CO2 bubbles. After that, the tube was centrifuged at 3000 rpm for 3 min until complete phase separation was achieved at room temperature. Finally, the floating supramolecular solvent droplets were collected and dissolved in 150 μL of acetonitrile to decrease the viscosity before injection into the HPLC. The microextraction method is demonstrated schematically in Fig. 1.
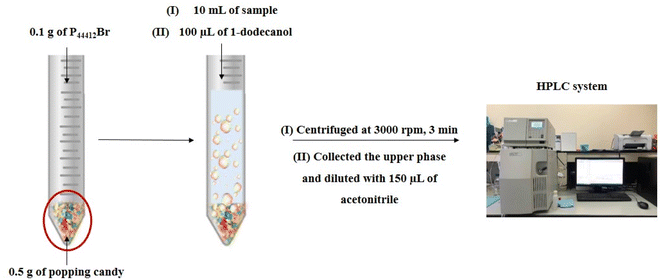 |
| Fig. 1 Schematic diagram of the popping candy-generated CO2 and sugaring-out-assisted supramolecular solvent-based microextraction (PGS-SUPRA) method prior to HPLC analysis. | |
Sample preparation
Water samples. Tap and surface water samples were collected from Maha Sarakham province. All water samples were filtered through a 0.45 μm membrane filter and kept in a refrigerator at 4 °C until extraction (see section Popping candy-generated CO2 and sugaring-out-assisted supramolecular solvent-based microextraction (PGS-SUPRA) method).
Honey samples. A honey sample was purchased from a supermarket in Kantharawichai district, Maha Sarakham province, Northeast of Thailand. For honey samples, 5 g of honey was diluted to 50 mL with water. The sample solution was mixed by shaking with hands for 30 s and then filtered using Whatman (no. 1) filter paper to remove the particulate matter. The diluted honey was passed through a 0.45 μm nylon membrane filter and then extracted using the developed method (see section Popping candy-generated CO2 and sugaring-out-assisted supramolecular solvent-based microextraction (PGS-SUPRA) method).
Soy milk samples. Two kinds of soy milk samples were purchased from a supermarket in Kantharawichai district, Maha Sarakham province, Northeast of Thailand. Soy milk samples were deproteinized and defatted before analysis using the previously reported method16 with some modifications. Briefly, the deproteinization of soy milk samples was operated using 1 mL sample, 5 mL of acetonitrile and 1 mL of 0.01 mol L−1 trifluoroacetic acid. After that, the solution was mixed using a vortex for 3 min and then centrifuged at 4500 rpm for 10 min. The supernatant solution was filtered through a 0.45 μm membrane filter and the final volume of the obtained solution was adjusted to 10 mL with deionized water, and then, the solution was extracted by the developed method (see section Popping candy-generated CO2 and sugaring-out-assisted supramolecular solvent-based microextraction (PGS-SUPRA) method).
Evaluation of enrichment factor (EF) and relative recovery (RR). In order to study the effect of experimental extraction conditions on the extraction efficiency, the enrichment factor (EF) was evaluated between the analyte concentration in the final phase (Csed) and the initial concentration in the analyte in an aqueous sample solution (C0), according to the following equations:The percentage relative recovery (RR, %) was calculated as the % amount of analyte recovered from the matrix with reference to the extracted standard (standard spiked into the same matrix).
|
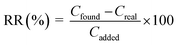 | (2) |
where
Cfound is the concentration of analyte after addition of a known amount of working standard to the real sample,
Creal is the analyte concentration in the real sample, and
Cadded represents the concentration of a known amount of working standard that was spiked into the real samples.
Result and discussion
Optimization of popping candy-generated CO2 and sugaring-out-assisted supramolecular solvent-based microextraction (PGS-SUPRA) conditions
Several experimental parameters including the type and amount of SUPRA, amount of popping candies, as well as the centrifugation speed and time of the extraction system were investigated and optimized by the univariate method, in order to obtain high extraction efficiencies of studied triazoles. A mixed standard solution containing 100 μg L−1 of each standard was used to examine the extraction performance of the microextraction method under different experimental conditions. All optimization experiments were carried out in triplicate (n = 3). Peak areas were used to evaluate the extraction efficiency of the developed procedure.
The popping candy plays an important role as a solid dispersant to decrease the consumption of toxic organic solvents and generate CO2 to induce the mass transfer (without other agitators) and sugaring-out effects (without salt addition). The amount of popping candies was investigated (0.3, 0.5, and 0.7 g), and the results are shown in Fig. 2(a). It was found that the extraction efficiency increased between 0.3 and 0.5 g due to the addition of solid dispersant, the release of CO2, and sugaring-out effects. It was speculated that sugar addition caused the surfactant to form an aggregated colloidal state and then decreased as the viscosity of the solution increased with the increase in the amount of popping candies, which decreased the mass transfer and recovery of analytes.17 As a result, 0.5 g popping candy was selected.
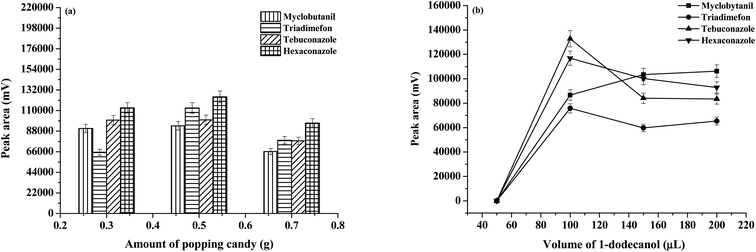 |
| Fig. 2 Effect of the experimental condition on the extraction efficiency: (a) amount of popping candies and (b) volume of 1-dodecanol (μL). | |
Without any surfactant, the organic phase microdrops re-aggregate and adhere to the surface of the injector, and thus, the extraction solvent cannot be efficiently collected.18 To ensure that the extraction solvent is evenly dispersed in the water sample, appropriate surfactants were examined including sodium dodecylsulphate (SDS), cetyltrimethylammonium bromide (CTAB), and [P44412]Br (data not shown). The extraction solvents in the emulsions that were dispersed by SDS and CTAB could not be completely separated from the aqueous phase. The addition of [P44412]Br provided high extraction efficiency, therefore a starting extraction solvent ([P44412]Br) capable of completely dissolving in the water sample was used. The amount of [P44412]Br was studied in the range of 0.05–0.5 g. When [P44412]Br 0.05 g was used, the viscous of extracted phase was obtained. Moreover, [P44412]Br 0.3 and 0.5 g were used, and the co-elution of triadimefon and tebuconazole was obtained. Therefore, [P44412]Br 0.1 g was selected.
The SUPRAS (as extraction solvent) was frequently preferred in the microextraction studies due to its interesting properties such as low density relative to water, commercial availability, green, and high dissolubility.19 The type of SUPRAS is a primary factor to be studied. To generate the supramolecular solvents, solvents such as alcohols and organic acids (i.e., 1-dodecanol (mp 24 °C), decanoic acid (mp 31.5 °C), and undecanol (mp 15.9 °C), with long carbon chains and apolar property) were studied. It was found that 1-dodecanol provided a higher extraction efficiency, better phase separation behavior and superior repeatability. Thus, 1-dodecanol was used as the extraction solvent. Moreover, the effect of 1-dodecanol volume was studied (0–200 μL). Fig. 2(b) exhibits that the extraction efficiency in terms of peak area improved with the increase in the volume of 1-dodecanol up to 100 μL and then decreased owing to dilution effects. Therefore, the volume of 100 μL was chosen.
The sample volume is a crucial factor to improve the response, which enables the analysis of a lower analyte concentration in the real sample medium.20 The sample volume was studied in the range of 7, 10, and 12 mL (data not shown). It was found that the highest responses of all analytes were obtained as the sample volume reached 10 mL. Therefore, a sample solution of 10 mL was used.
Different solvents were studied including acetonitrile, ethanol and methanol in order to decrease the viscosity of the extract before injecting into the chromatographic system (data not shown). It was found that acetonitrile provided high responses. Therefore, acetonitrile was used and the volume of acetonitrile was evaluated to be 50, 100, 150, 200 and 250 μL (data not shown). It was found that the highest response was obtained when acetonitrile 100 μL was added. Therefore, 100 μL of acetonitrile was used.
The optimum extraction conditions for analysis of triazole fungicides were popping candy (0.5 g), [P44412]Br (0.1 g), 1-dodecanol (100 μL), sample volume (10 mL) and 100 μL of acetonitrile.
Analytical performance and method validations
The analytical performance of the proposed popping candy-generated CO2 and sugaring-out-assisted supramolecular solvent-based microextraction (PGS-SUPRA) method coupled with the HPLC procedure for the quantification of four triazole fungicides was studied under optimized conditions. Linear ranges (LRs), limits of detection (LODs), limits of quantification (LOQs), repeatability, reproducibility, extraction recovery and matrix effect (ME) were employed. The analytical features of the proposed method are summarized in Table 1. The linearity of triazole fungicides after preconcentration by the proposed microextraction method ranged from 30 to 1000 μg L−1 for triadimefon and from 90 to 1000 μg L−1 for myclobutanil, tebuconazole and hexaconazole, with the coefficient for determination (R2) greater than 0.992. The LODs and LOQs were calculated based on the signal-to-noise ratios of 3 and 10, respectively. LODs and LOQs were in the range of 10–30 μg L−1 and 30–90 μg L−1, respectively. The precisions were evaluated from the relative standard deviations (RSDs) of retention time, and peak area obtained from intra- (n = 3) and inter-day (n = 3 × 5) experiments, were greater than 1.66% and 13.52%, respectively. Moreover, the proposed method provided high enhancement factors (EnFs) ranging from 14 to 51 folds, calculated from the analyte concentration in the final phase (Csed) and the initial concentration in the analyte in an aqueous sample solution (C0). Chromatograms of the studied triazoles obtained by direct HPLC and the proposed microextraction method are presented in Fig. 3. The result shows that the chromatographic signals of triazoles were increased after the microextraction process.
Table 1 Analytical features of the proposed microextraction method for determination of triazole fungicides
Analyte |
Linear range (μg L−1) |
Linear equation |
R2 |
LOD (μg L−1) |
LOQ (μg L−1) |
EF (Cex/C0) |
Intra-day precision (n = 3), RSD (%) |
Inter-day precision (n = 5 × 3 days), RSD (%) |
tR |
Peak area |
tR |
Peak area |
Myclobutanil |
90–1000 |
y = 8 457 83x + 31 098 |
0.998 |
30 |
90 |
14.25 |
0.87 |
3.77 |
1.38 |
13.52 |
Triadimefon |
30–1000 |
y = 5 969 77x + 15 342 |
0.992 |
10 |
30 |
30.47 |
0.92 |
4.06 |
1.66 |
6.23 |
Tebuconazole |
90–1000 |
y = (1 × 106)x + 34 610 |
0.994 |
30 |
90 |
42.80 |
0.85 |
4.04 |
1.06 |
8.64 |
Hexaconazole |
90–1000 |
y = (1 × 106)x + 7088.4 |
0.999 |
30 |
90 |
50.36 |
0.45 |
3.96 |
0.80 |
9.64 |
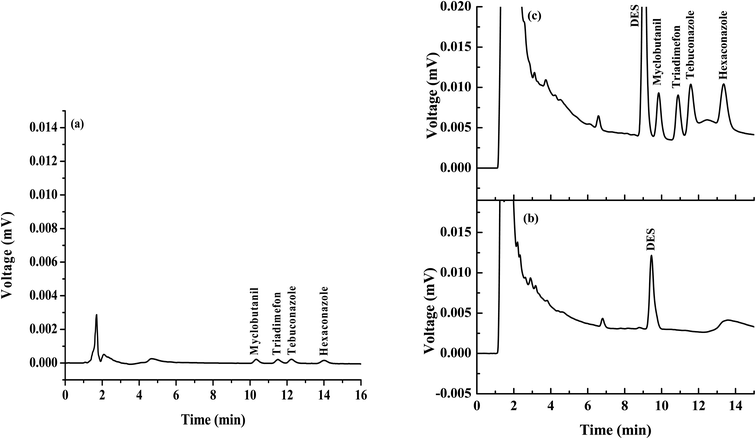 |
| Fig. 3 Chromatograms of standard triazole fungicides obtained by (a) direct HPLC injection and (b) blank after preconcentration by the proposed microextraction method combined with HPLC and (c) standard triazole fungicides after preconcentration by the proposed microextraction method combined with HPLC; the concentration of all standards was 100 μg L−1. | |
To study the matrix effect of real samples, a matrix-match calibration method was used. The matrix-match calibration was studied using spiked real samples in the range of 100–500 μg L−1 of each target analyte. The target analytes exhibit wide linearity with R2 greater than 0.9. In addition, the matrix effect (ME, %) of each calibration graph in the soil sample was calculated using eqn (3).
|
ME(%) = (Sm/Ss) × 100
| (3) |
where
Sm and
Ss are the slopes of the calibration curve in the matrix and solvent, respectively. Generally, a ME between 80 and 120% indicates no matrix effects, a ME between 50 and 80% or 120 and 150% refers to minor matrix effects, and a ME <50% or >150% indicates major matrix effects.
21 The ME values were in the range of −63.7 to −78.6% in water samples, −38.1 to −58.6% in honey samples and −43.1 to −68.6% in soy milk samples. The result indicates that the honey and soy milk samples showed major effects, while the water samples showed minor effects for triazole fungicide analysis.
Application to real samples
The proposed microextraction procedure was then applied for the analysis of water, honey and soy milk samples. Each sample was prepared (as described in section Sample preparation) and extracted by the proposed method (as described in section Popping candy-generated CO2 and sugaring-out-assisted supramolecular solvent-based microextraction (PGS-SUPRA) method) prior to their analysis by HPLC. However, matrix match calibration was used for the determination of studied compounds in real samples to compensate for the matrix effect. It was found that no residues of the investigated triazole fungicides were detected in all studied samples (data not shown). To investigate the accuracy of the proposed method, the water samples were spiked with 90, 150, and 250 μg L−1 of each triazole before applying the proposed microextraction procedure. The recoveries of the studied triazoles were obtained in the range of 60–114% (data not shown). The overlaid chromatograms of samples and spiked samples are shown in Fig. 4.
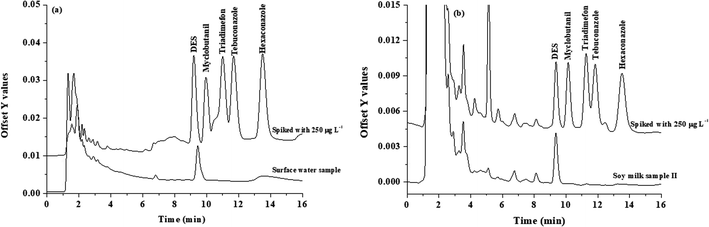 |
| Fig. 4 Chromatograms of the (a) water sample and spike water sample (250 μg L−1), and (b) soy milk sample and spike soy milk sample (250 μg L−1). | |
Comparison of the proposed method with previous microextraction methods
The extraction procedure and analytical performance of the proposed microextraction method were compared to the previous microextraction procedures5,22–25 for the determination of triazoles in real samples (as shown in Table 2). Other liquid–liquid microextraction approaches require a relatively high volume of the extraction solvent to be used and more agitators to increase the mass transfer. Unlike these reported microextraction procedures for triazole determinations, the dispersion of extractant and extraction of analytes were performed using the proposed technique without any need for extraction equipment. Additionally, our work presented a shorter extraction time, lower LOQs, and comparable accuracy for the simultaneous extraction and determination of triazole fungicides. These results indicated that the extraction method developed in this work is a simple, sensitive, and high-performance method.
Table 2 Comparisons of the proposed method with reported methods for the analysis of triazole fungicidesa
Method |
Analyte/sample |
Conditions |
Analytical technique |
LR |
LODs |
% Recovery |
Ref. |
DES-SDME-GC-FID, deep eutectic solvents as extraction phase in head-space single-drop microextraction coupled to gas chromatography-flame ionization detection; VA-DLLME, vaporization assisted dispersive liquid–liquid microextraction coupled to gas chromatography-flame ionization detection; CD-DLLME-HPLC-DAD, cyclodextrin-based dispersive liquid–liquid microextraction coupled to high performance liquid chromatography-diode array detector; EA-SHS-ME-SFO-HPLC-DAD, effervescent assisted switchable hydrophilicity solvent-based microextraction with solidification of floating organic droplets coupled to high performance liquid chromatography-diode array detector. |
Ionic liquid combined with liquid–liquid microextraction |
Triazole fungicides/honey, fruit juices, and egg yolk samples |
Extraction solvent: IL |
HPLC-DAD |
150–1000 μg L−1 |
30–50 μg L−1 |
61–112 |
22 |
Disperser solvent: ACN |
Sample volume: 10 mL |
Extraction time: 12 min |
DES-SDME |
Triazole fungicides/fruit juice and vegetable samples |
DES: 2 μL ChCl-chlorophenol |
GC-FID |
0.01–100 mg L−1 |
0.00085–0.001 mg L−1 |
93–97 |
23 |
Sample volume: 10 mL |
Sample temperature: 85 °C |
Extraction time: 30 min |
CD-DLLME |
Triazole and strobilurin fungicides/water, juice, and vinegar samples |
Extraction solvent: 200 μL undecanol |
HPLC-DAD |
1–100 μg L−1 |
0.3 μg L−1 |
83.103.2 |
24 |
Disperser solvent: 600 μL HP-β-CD |
Sample volume: 10 mL |
Extraction time: <0.02 min |
VA-DLLME |
Triazole fungicide, herbicides, pesticide and insecticide/fruit juice samples |
Extraction solvent: 95 μL 1,2-dibromoethane |
GC-FID |
149–500000 ng L−1 |
45–78 ng L−1 |
55–89 |
25 |
Disperser solvent: 1 mL iso-propanol |
Sample volume: 5 mL |
Extraction time: 5 min |
EA-SHS-ME-SFO |
Triazole fungicides/honey samples |
SHS mixture: 300 mg citric aid + 25 mg sodium octanoate + 50 mg sodium bicarbonate |
HPLC-DAD |
3–100 μg L−1 |
1 μg L−1 |
60.26–99.72 |
5 |
Dissolving solvent: 100 μL acetonitrile |
Sample volume: 10 mL |
Extraction time: 10 min |
PGS-SUPRA |
Triazole fungicides/water, honey and soy milk samples |
SUPRA solvent: P44412Br + 1-dodecanol |
HPLC-DAD |
30–1000 μg L−1 |
10–30 μg L−1 |
60–114% |
This method |
Disperser + agitator: popping candy |
Sugaring-out |
Sample volume: 10 mL |
Extraction time: 3 min |
Conclusions
An eco-friendly and effective method using the popping candy-generated CO2 and sugaring-out-assisted supramolecular solvent-based microextraction (PGS-SUPRA) method has been investigated for the enrichment and analysis of triazole fungicides prior to HPLC analysis. Popping candies were used as dispersants and auxiliary devices; as a result, the extraction efficiency increased. No agitators were needed. The proposed preconcentration method exhibited good linearity, high sensitivity, and satisfactory accuracy and precision. In addition, this method was used as an alternative extraction method for the determination of triazole fungicides in water, honey, and soy milk samples.
Conflicts of interest
There are no conflicts to declare.
Acknowledgements
The authors gratefully acknowledge financial supports for this research from Thailand Science Research and Innovation (TSRI), Mahasarakham University and Center of Excellence for Innovation in Chemistry (PERCH-CIC). R. Kachangoon and J. Vichapong would like to thank the National Research Council of Thailand (NRCT): (N41A640218).
References
- X. Jing, X. Huang, Y. Zhang, M. Wang, H. Xue, X. Wang and L. Jia, Food Chem., 2022, 367, 130664 CrossRef CAS PubMed.
- Y. Wang, M. He, B. Chen and B. Hu, J. Chromatogr. A, 2020, 1633, 461628 CrossRef CAS PubMed.
- H. Sun, J. Feng, J. Feng, M. Sun, Y. Feng and M. Sun, Food Chem., 2022, 395, 133633 CrossRef CAS PubMed.
- J. Li, F. Dong, Y. Cheng, X. Liu, J. Xu, Y. Li, X. Chen, Z. Kong and Y. Zheng, Anal. Bioanal. Chem., 2012, 404, 2017–2031 CrossRef CAS PubMed.
- J. Palasak, R. Buppasang, R. Kachangoon, J. Vichapong, R. Burakham, Y. Santaladchaiyakit and S. Srijaranai, Microchem. J., 2022, 182, 107882 CrossRef CAS.
- H. Xiaofei, C. Jia, L. Zhan, Q. Kaijun and Q. Hongdeng, Magnetic solid-phase extraction of triazole fungicides based on magnetic porous carbon prepared by combustion combined with solvothermal method, Anal. Chim. Acta, 2020, 1129, 85–97 CrossRef.
- A. Ballesteros-Gomez, M. D. Sicalia and S. Rubio, Anal. Chim. Acta, 2010, 677, 108 CrossRef CAS PubMed.
- K. Seebunrueng, C. Dejchaiwatana, Y. Santaladchaiyakit and S. Srijaranai, RSC Adv., 2017, 7, 50143 RSC.
- J. Vichapong and R. Burakham, Anal. Methods, 2012, 4, 2101 RSC.
- J. Vichapong, R. Burakham and S. Srijaranai, Talanta, 2013, 117, 221 CrossRef CAS PubMed.
- J. Vichapong, Y. Santaladchaiyakit, R. Burakham and S. Srijaranai, Anal. Lett., 2015, 48, 617 CrossRef CAS.
- J. Vichapong, R. Burakham and S. Srijaranai, Talanta, 2015, 139, 21 CrossRef CAS PubMed.
- J. Vichapong, R. Burakham and S. Srijaranai, Food Anal. Methods, 2017, 10, 1645 CrossRef.
- X. Jing, X. Huang, H. Wang, H. Xue, B. Wu, X. Wang and L. Jia, Food Chem., 2021, 348, 129147 CrossRef CAS.
- B. Wang, T. Ezejias, H. Feng and H. Blaschek, Chem. Eng. Sci., 2008, 63, 2595 CrossRef CAS.
- N. Gissawong, S. Boonchiangma, S. Mukdasai and S. Srijaranai, Talanta, 2019, 200, 203 CrossRef CAS PubMed.
- M. A. Farajzadeh, P. Khorram and A. Pazhohan, J. Chromatogr. B, 2016, 1017, 62 CrossRef PubMed.
- H. Qian, L. Hu, C. Liu, H. Wang, H. Gao and W. Zhou, J. Chromatogr. A, 2018, 1559, 86 CrossRef CAS PubMed.
- N. Altunaya and A. Elik, Food Chem., 2020, 332, 127395 CrossRef PubMed.
- J. Vichapong, R. Burakham, S. Srijaranai and K. Grudpan, Talanta, 2011, 84, 1253 CrossRef CAS PubMed.
- T. Boontongto and R. Burakham, Anal. Chim. Acta, 2021, 1186, 339128 CrossRef CAS.
- R. Kachangoon, J. Vichapong, Y. Santaladchaiyakit and S. Srijaranai, Molecules, 2022, 27, 3416 CrossRef CAS.
- D. Li, M. He, B. Chen and B. Hu, J. Chromatogr. A, 2019, 1601, 1 CrossRef CAS PubMed.
- M. M. Abolghasemi, M. Piryaei and R. M. Imani, Microchem. J., 2020, 158, 105041 CrossRef CAS.
- M. A. Farajzadeh, L. Kiavar and S. Pezhhanfar, J. Chromatogr. A, 2021, 1653, 462427 CrossRef CAS.
|
This journal is © The Royal Society of Chemistry 2023 |
Click here to see how this site uses Cookies. View our privacy policy here.