DOI:
10.1039/D2RA07095B
(Review Article)
RSC Adv., 2023,
13, 3505-3519
A review on adsorption characteristics and influencing mechanism of heavy metals in farmland soil
Received
8th November 2022
, Accepted 31st December 2022
First published on 25th January 2023
Abstract
The accumulation of heavy metals in soil and crops is considered to be a severe environmental problem due to its various harmful effects on animals and plants. Soil adsorption is an essential characteristic of mud, which is the fundamental reason for soil to have a specific self-purification capacity and environmental capacity for heavy metals. The adsorption of heavy metals by soil reduces the uptake of these pollutants by crops, thereby limiting food contamination. Therefore, the adsorption of heavy metals in crop soils was taken as the primary research object. Based on the entire reading of the literature, the previous research results were compared and discussed from the four aspects of heterogeneity, physical and chemical properties, competitive adsorption, and external factors. The influencing mechanism of heavy metal adsorption characteristics in soil was reviewed. Finally, suggestions and prospects for future research on heavy metal adsorption were put forward.
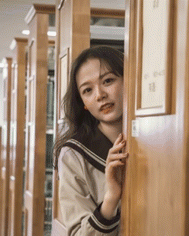 Hanjing Yu | Hanjing Yu was born in Xinjiang, China in 1998 and was studying for a master's degree at Kunming University of Science and Technology in 2021. She has been engaged in soil chemistry research under the guidance of Professor of P. Dong at the School of Metallurgical and Energy Engineering. |
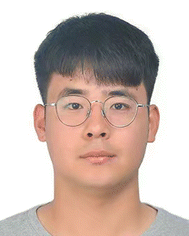 Chenchen Li | Chenchen Li was born in Hebei Province, China in 1995. He is now studying at Kunming University of Technology and is a doctoral student. Under the guidance of Prof. Dong Peng, he is engaged in research on the migration and transformation of heavy metals in smelting sites and affected areas. |
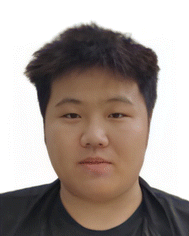 Jin Yan | Jin Yan was born in Xing tai, Hebei Province, China in 1998 and received his bachelor's degree in environmental engineering from Kunming University of Science and Technology in 2019. Since 2019, he has continued his master's and doctoral studies at Kunming University of Science and Technology. His research interests are the migration and transformation of heavy metals in soils. |
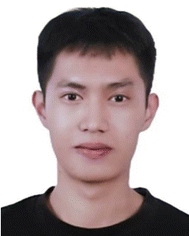 Yaoqiang Ma | Yaoqiang Ma was born in Pu 'er City, Yunnan Province, China in 1999 and studied for the master's degree in Kunming University of Science and Technology in 2021. He has worked on soil chemistry research under the supervision of Professor P. Dong at the Faculty of Metallurgical and Energy Engineering, Kunming University of Science and Technology. |
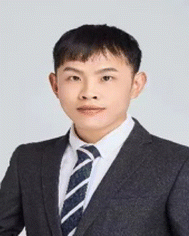 Qi Meng | Qi Meng was born in Qufu City, Shandong Province, China in 1989. In 2008, he received a bachelor's degree in engineering from Shandong University of Science and Technology. In 2012, he received a master's degree in engineering from Kunming University of Science and Technology. In 2018, he received a doctor's degree in engineering from Kunming University of Science and Technology. Since 2018, he has worked in Kunming University of Science and Technology, mainly focusing on the research of site causes and pollution prevention. |
1 Introduction
Soil is one of the natural resources for human survival, and it is also an essential part of the human ecological environment. In the past 30 years, China has experienced a rapid industrial revolution and urbanization, resulting in increased energy consumption and environmental pollution problems. Among all kinds of pollution, heavy metal pollution is one of the most critical ecological problems. The primary heavy metal pollutants in the environment are arsenic (As), cadmium (Cd), chromium (Cr), copper (Cu), lead (Pb), mercury (Hg), nickel (Ni) and zinc (Zn).1 Heavy metals show great ecological significance because of high toxicity, persistence and bioaccumulation capacity. As shown in Fig. 1, heavy metals enter the human body through three media: atmosphere, soil, and water. In agricultural soils, with the application of fertilizers, sewage sludge discharge, incorrect soil improvement, mining, and nearby automobile exhaust, a large amount of heavy metals enter agricultural soils and are adsorbed by crops and eventually enter people's bodies. The use of fertilizers is one of the key factors affecting the content of heavy metals in the soil, and today most rural areas still maintain the habit of applying “farmyard manure”. As we all know, mineral additives are commonly used in animal feeds to meet the demand for minerals and to improve the growth performance of animals. Minerals such as Cu, Zn, Fe, Cr, Mn, and Co are essential for livestock. Due to the low purity of mineral additives used in animal feed, non-essential trace elements such as cadmium, mercury, arsenic, lead, and other heavy metals are introduced into livestock. Only small amounts of heavy metals in feed are absorbed by animals; most are excreted in manure and later used as “farmyard manure”, thus changing the content of heavy metals in farmland soils.2 In Hubei, Shandong and other places, the discharge of heavy metal pollutants from chemical materials and the manufacturing industry accounts for more than 90%. In addition, according to the national pollution census data, there are more than 1.5 million heavy metal exposure points in China. From 2005 to 2011, the total discharge of heavy metals in wastewater, exhaust gas and solid waste was about 900
000 tons per year.3 At the same time, the area of agricultural soil polluted by Cd in China is about 2.786 × 109 m.4 In 2013, the chronic daily intake dose of residents near a lead-zinc mining area in Jiangsu Province exceeded the safe reference dose by 15 times.5 Exposure to high concentrations of heavy metals can also cause permanent mental health problems, cancer, skin diseases, paralysis, tooth loss, eye problems, kidney and lung dysfunction, muscle and joint pain and many other complications.3,6
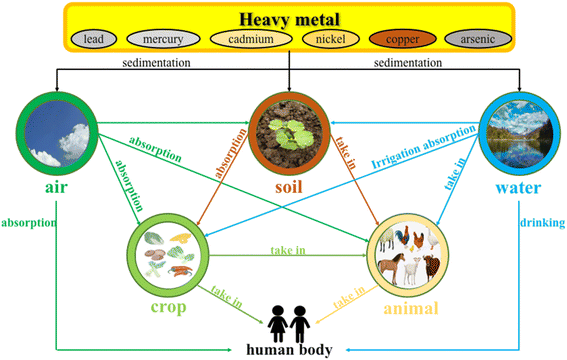 |
| Fig. 1 Pathway of heavy metals entering the human body. | |
Heavy metal pollution is the most severe problem in farmland soil pollution because of its long residual time, irreversibility, small transfer amount, high toxicity, strong concealment, complex chemical properties and strong ecological response.7 And a series of migration and transformation processes occur in the soil, including adsorption, coordination, oxidation and reduction, and precipitation–dissolution, among which adsorption process as the most common characteristic is one of the most important processes of heavy metals in the soil, and is the fundamental reason for the soil to have a certain self-purification capacity and environmental capacity for heavy metals.8 It also directly affects the bioavailability and ecotoxicity of heavy metals in soil-plant system, and ultimately affects the normal growth of animals and plants and even human health.9 Therefore, it is of great practical significance to study the adsorption characteristics of heavy metals in soil to understand and control the environmental behavior of heavy metals in soil-plant-human systems and predict the ecological effects of heavy metals. In this paper, the research results of the adsorption of heavy metals in soil by scholars at home and abroad in recent years were reviewed from four aspects: (1) the effect of soil heterogeneity on the adsorption of heavy metals in soil; (2) the effect of physical and chemical properties of soil itself on the adsorption of heavy metal; (3) the effects of competitive adsorption between heavy metals on the adsorption of heavy metals in soil were discussed, and the effects of pH and ionic strength in competitive adsorption were introduced. (4) Other external factors affect soil's physical and chemical properties and indirectly affect the adsorption of heavy metals in soil.
2 The heterogeneity of soil
This section focuses on the effects of significant soil heterogeneities, including soil type, soil texture, soil stratification, soil organic matter and iron and aluminum oxides, and clay minerals, on the adsorption of heavy metals in soils. The physical and chemical properties of soil vary with soil type, soil stratification, and texture. Similarly, clay minerals, organic matter, iron, and aluminum oxides, organic minerals, and natural zeolites act as “natural adsorbents” for heavy metals in the gas field.10 Their physical and chemical properties are different, and each soil adsorbent contains various functional groups which can bind to heavy metals, which also significantly impacts the kinetic reaction of heavy metals with soil.
2.1 Soil composition and type
Soil contains a variety of iron oxides, in which magnetite, hematite and hematite are the most common; in addition, ferrihydrite, lepidocrocite, and schwertmannite exist as intermediate products in the transformation process.11 Due to the differences in specific surface area and adsorption sites, different iron oxides have different adsorption capacities for heavy metals (amorphous iron oxides > maghemite > lepidocrocite > goethite).12 The formation modes of iron oxides include (1) direct precipitation of Fe2+ or Fe3+; (2) dissolution and reprecipitation or crystal transformation of iron oxides under the interference of external factors.13 It is affected by pH, temperature, and organic matter in the natural environment, and there are differences in crystallinity and specific surface area of different iron oxides. Under the interference of humic acid (HA) and Pb2+, the conversion rate of ferrihydrite to hematite slows down, and the formed hematite has a loose and porous structure.14 On the other hand, ferrihydrite is converted into hematite, and its ability to adsorb heavy metals is reduced. Xu et al. conducted waterlogged incubation experiments on six different soils. They found that C(Fe) and C(Mn) in soil solution were significantly positively correlated with C(As), indicating that the reduction and dissolution of iron and manganese oxides released As in solid soil phase.15 They found that C(Fe) and C(Mn) in soil solution were significantly positively correlated with C(As), indicating that the reduction and dissolution of iron and manganese oxides released As the in solid soil phase. However, when Fe2+ combines with dissolved oxygen in the sediment overlying water with high oxygen content, amorphous or microcrystalline iron oxides with solid adsorption capacity for free heavy metal ions can be formed.16
There are about 15 manganese oxides in the soil, and the most common natural manganese oxide is hydromica.17 Both vernadite and birnessite are of the layered structure, and vernadite is called weakly crystalline birnessite. The synthetic birnessite/vernadite used in many studies belongs to the birnessite series, abbreviated δ-MnO2.18 δ-MnO2 has low zero-point potential and a large specific surface area, and it can adsorb certain heavy metals; manganese has multiple valence states and strong oxidation ability and catalytic ability. The adsorption capacity of birnessite for several cations is Pb2+ > Cu2+ > Zn2+ > Co2+ > Cd2+. Birnessite has two kinds of adsorption sites: vacancy sites and edge sites. Cationic heavy metals (such as Ni2+, Zn2+, and Pb2+) are preferentially adsorbed on the vacancy sites, then adsorbed on the edge site.19
In soil, Se is a metal-like element with dual properties of metal and nonmetal, which is generally divided into plant active selenium and inorganic selenium. In soil, Se is a metal-like element with dual properties of metal and nonmetal, which is generally divided into plant active selenium and inorganic selenium. Inorganic selenium generally refers to sodium selenite and sodium selenate. Selenium exists in the soil in the form of selenate, selenite, elemental selenium, selenide, organic selenium and gaseous selenium. As one of the essential trace elements for the human body, a large number of agricultural products have been grown naturally in selenium-rich soil environments or through selenium-fortified methods in China in recent years.20 It is reported that selenium can absorb heavy metals well. Zhida et al. found that the adsorption capacity of Pb can be significantly improved by more than 2.56 times when a very small amount of Se is introduced into the material.21 Gailer's blood analysis of rabbits found that Se can form Se–As and Se–Hg compounds with As and Hg, thus reducing the toxic effects of heavy metals on the body, but whether this mechanism exists in soil remains to be further studied, and although some progress has been made in the study of heavy metal interaction, the mechanism of their interaction is still unclear.22
Clay minerals have large cation exchange capacities. Most clay minerals are negatively charged, capable of absorbing both positive and negative ions by ion exchange. They widely absorb metal cations from solution.23 At low pH (e.g., pH 3–6), it first undergoes ion exchange with cationic heavy metals, and at high pH, it forms surface complexes. The content of aluminum oxide in the soil is low, so its contribution to the adsorption and fixation of heavy metals is less than that of iron oxide. Sipos et al. found that the swelling clay particles had the highest absorption rate for the study product, followed by the mixed clay structure containing swelling components and at least pathological details.24 Jensen et al. found that the affinity of Pb to different soil components was humus > clay minerals > iron (hydroxide) oxides.25 Covelo et al. studied the adsorption of Cd, Cr, Cu, Ni, Pb, and Zn by kaolin, vermiculite, mica, and other standard soil components when they competed with each additional.26 According to the distribution coefficient, the following conclusions were drawn: kaolin preferentially adsorbed Cr, vermiculite preferentially adsorbed Cu and Zn, mica only adsorbed Cr; Kaolin cannot fix Cu. Dalacorte et al. studied the swellable interlayer of clay minerals in the adsorption site of basalt, founding that the desorption of Cu and Zn by basalt was lower than that by standard clay minerals.27
The soil types in China include red earth, red-yellow earth, yellow-brown earth, yellow-brown earth and gray-brown soil, dark brown forest soil, podzolic soil, chernozem from northeast to northwest, chestnut soil, brown dirt, gray-brown desert soil, and brown desert soil. The soil types of Qinghai-Tibet Plateau are alpine meadow soil, alpine steppe soil, alpine desert soil, and frozen alpine soil from east to west.28 However, at present, the influence of soil types on heavy metal adsorption in the world is limited to typical soils in some regions, such as Nan and Zhao, where determined heavy metal contents in grey soils of two basins in Baiyin City29 and Adhikari and Singh, which determined heavy metal contents in five agricultural ecological zones (AEZ) in India.30 Ghrair et al. studied the adsorption–desorption process of heavy metals in soils of karst areas in southwest China,31 and Hamidpour et al. studied the adsorption reversibility of Cd2+ and Pb2+ on bentonite.32 Zhong et al. studied the effects of basalt soils in tropical Hainan on Cu2+ and Cd2+.33 It was found that the cation exchange capacity of the soil decreased with the age of the basalt, and the content of free iron oxide in the soil decreased with the age of the basalt.
2.2 Soil texture (particle size)
Soil particle size is the basic structural unit of soil. The impermeability of aggregates with different particle sizes is different, and the adsorption and migration of heavy metals in soil are further.34 According to the size and nature of soil particles, the earth can be divided into several particle fractions. According to the standard of the United States Department of Agriculture, the particle size is divided into sand (50–2000 μm), silt (2–50 μm), and clay (<2 μm). The sand is further subdivided into coarse sand (250–2000 μm) and fine sand (53–250 μm), and the silt is further subdivided into rough mud (20–53 μm) and fine silt (2–20 μm).35
Gove et al. studied the migration of Pb, Cu, Zn, and Ni in sandy soil and sandy loam under constant flow conditions. They found that soil particle size significantly affected the migration of heavy metal ions.36 Acosta found that aggregates with different particle sizes may have different abilities to adsorb heavy metals. The larger the surface area of soil particles, the more organic matter, and metal oxides content.37 Fine particles have a higher capacity to carry heavy metals than coarse particles. Studied the adsorption characteristics of Cu, Zn, and Cd in paddy soil with different particle sizes and found that the organic matter content of soil aggregates with <0.002 mm particle size was very large (>5%).38 The maximum adsorption capacity of Cu, Zn and Cd was 1.14–1.49 times, 1.45–2.22 times, and 1.21–1.44 times of other particle sizes, respectively. Similarly, in the study of heavy metals relative to soil particle size, Zhang and Zhang, Y. et al. found that heavy metal pollution hindered the formation of large particle size (reduced by about 15%) and led to the increase of small particle size, thus significantly enhancing the distribution of heavy metals in silt and clay aggregates.39
2.3 Soil stratification
Different disciplines have different vertical stratification of soil, different disciplines have different vertical soil stratification, and in agriculture, the four layers of soil are divided according to the four layers of agricultural soil as follows:40 plow layer, plough pan, subsoil layer and parent material layer to explore the adsorption and desorption characteristics of heavy metal Zn in different layered soils and understand the migration law of Zn in layered soils. The adsorption and desorption behaviors of Zn in four soil layers were analyzed, and the adsorption capacity of Zn in four soil layers was in the order of plough layer > plow pan > subsoil > parent material layer. The mass fraction of soil organic matter decreased gradually, and the adsorption capacity of four layered soils for zinc decreased gradually. The amount of Zn desorption decreased by 13.7%, 16.1%, 17.2% and 17.7% in the plow layer, plow pan, subsoil, and parent material layer, respectively. Zhong et al. studied zinc's adsorption and desorption behavior in layered chernozem with different parent materials.33 The results showed that the soil with the highest organic matter content had the most muscular adsorption capacity for zinc, which was greater than that of subsoil and parent soil, respectively. Xiao et al. once divided soil organic matter into dissolved and pore-filling phases.41 The fast adsorption stage is the adsorption of the dissolved phase, with a significant diffusion coefficient. The fast and slow adsorption stage is the adsorption in the pore-filling phase, with a slow adsorption rate. Zn has the most considerable adsorption in the plow layer, mainly due to years of cultivation, higher organic matter mass fraction on the soil surface, and more adsorption sites. However, with the increase in depth, the mass fraction of organic matter decreases gradually. The adsorption sites on the soil surface are progressively saturated.42 It was found that the electron effect of Cd, Pb, and Zn in the surface soil was significantly higher than that in the subsoil and increased with depth. Therefore, it is not difficult to see that the content of organic matter causes the difference of heavy metal adsorption in each layer of soil.
2.4 Soil organic matter (SOM)
Organic matter is one of the essential soil components for the adsorption of heavy metals in soils. Pérez-Novo, a C. P, used acid soils in northern Spain and two tested soils with organic matter removed as controls to adsorb heavy metal Zn. The results showed that the adsorption of Zn in soils with organic matter removed was greatly reduced, which was the same as the results of Yuan and Lavkulich.43 It was proved that organic matter was one of the main factors affecting the adsorption of heavy metals in soil.44 Jiang et al. studies found that after removing organic matter, the adsorption capacity decreased significantly, and the activity of Cr increased.45 Soil organic matter can be divided into four types: free particulate organic matter (fPOM), occluded particulate organic matter (oPOM), intra-aggregate organic matter (iPOM) and dissolved organic matter.38,46 They increase the adsorption capacity of soil to heavy metals through surface complexation, ion exchange and surface precipitation. The complexation of dissolved organic matter (DOM) makes more heavy metal ions remain in the soil solution, thus reducing the adsorption of heavy metal ions by the soil. DOM is an ill-defined term for a complex mixture of different, partially known, water-soluble organic molecules and partially decomposed plant matter, and is generally defined as the proportion of organic molecules that can pass through a 0.45 μm filter.47 Kalbitz et al. believe that adsorption of DOM on the surface of soil minerals is an important reason for the content reduction of soluble organic matter in soil solution.48
Humus is an integral part of SOM and a kind of natural macromolecular organic compound which can be derived from soil, sediment and water. Humus accounts for about 60–80% in soil and sediment.49 Humus is divided into humic substance (HS) and non-humic substance (non-HS) according to whether specific organic compounds with unknown chemical structures are formed in the process of decay and decomposition.50 Non-HS is a joint organic compound, such as amino acids, sugars, proteins and so on. Humic acid (HA), fulvic acid (FA) and humin (HM) are unique organic compounds that are ubiquitous in soils. By comparison of aggregation degree and molecular weight, HM > HA > FA. HA has the highest aggregation degree and is insoluble in alkaline and acidic solution.51 HA is the main active component in soil humus, which has a complex chemical structure and contains a variety of active functional groups (hydroxyl, carboxyl, phenolic hydroxyl, alcoholic hydroxyl, quinolinyl, and carbonyl, etc.). Its large cation exchange capacity in structure makes it have high affinity and binding force to heavy metal cations in soil, and it can adsorb heavy metal ions in the ground.52 Yang et al. found that the ability of HA to adsorb heavy metals mainly depends on acidic functional groups, especially carboxyl groups.53 Arias et al. measured the equilibrium isotherms of Cu and Cd on kaolinite and found that HAs could enhance the metal adsorption capacity of the mineral surface, especially kaolinite.54 Wang's research has proved that HM extracted from lignite and its calcium-based modified materials have strong adsorption and passivation effects on heavy metal cadmium.55
3 Physical and chemical properties of soil
The adsorption capacity of farmland soil to heavy metals is not only related to the heterogeneity of soil but also the physical and chemical properties of soil. In general, the soil's composition and physical and chemical properties have a more significant impact on the adsorption and retention capacity of heavy metals than the nature of heavy metals themselves. The physical and chemical properties include pH, redox potential and temperature, moisture content and cation exchange capacity, etc. Only the first three are described here.
3.1 The pH of the soil
The pH is an important parameter to determine the adsorption capacity of heavy metals in farmland soil, which affects the hydrolysis of metal ions, the formation of ion pairs, the solubility of organic matter, and the surface charge of the earth. The pH of soil greatly impacts the adsorption capacity and solubility of heavy metals in soil comminuted by heavy metals.56 It has been found that the adsorption of most heavy metals in soil can be increased from 0 to nearly 100% in a small pH range.57 In general, the ability to adsorb heavy metals is positively correlated with soil pH; that, the lower the pH value, the more likely the metal ions exist in the soil solution, so they are more easily transported and absorbed.58
Huang et al. found that the adsorption capacity of Cu and Zn increased significantly with the increase of pH;34 Markiewiez-Patkowska et al. studied the adsorption behavior of heavy metals in urban contaminated soil, and founding that the maximum adsorption capacity of Cd in soil increased from 0.246 mg g−1 to 2.294 mg g−1, which may be due to the increase of pH, resulting in the increase of negative charge on the soil surface, which is conducive to increasing the adsorption of Cd by the soil.59 Liu et al. proposed that the adsorption capacity of adsorbents was closely related to pH, and pH might change the existing form of Cr, thereby changing the leaching rate of heavy metals in soil.60 Azeez et al. found that alkaline soil had the highest adsorption efficiency for Zn and Pb, and slightly acidic soil had the highest adsorption efficiency for Cd and Cu, so somewhat acidic soil was good adsorption for heavy metals.61 With the increase of pH, the adsorption capacity of Cr on soil and sediment increased first and then decreased, and higher and lower pH values were not conducive to the adsorption of Cr on sediment and soil.45 The results showed that the specific adsorption of Cu and Pb by variable charge soils was mainly based on a coordination mechanism at low pH value, At the same time, for cationic heavy metals, the desorption of heavy metals was more accessible when the pH value decreased, mainly due to the following reasons: (1) when pH value decreased, protons competed with cationic heavy metals for restricted sites, and heavy metals were released into soil solution; (2) the decrease of pH makes some minerals dissolve, and the heavy metals adsorbed on the minerals are released into the soil solution; (3) when pH > the point zero of charge (PZC) of the component, the repulsive force between the component and the heavy metal increases with the decrease of pH. When the pH decreases to the PZC of the component, the component is not charged, and when the pH further decreases, the repulsive force between the component and the heavy metal increases. Heavy metals quickly to decompose and adsorb from this group, and the hydrolysis adsorption mechanism is dominant at a high pH value.62 Cr, As, and other ions, they mainly exist in the form of oxyanions in the soil, and their adsorption capacity decreases with the increase of pH value, mainly because with the increase of pH value, the amount of negative charge on the soil surface increases, and the mutual repulsion between Cr, As, and oxyanions with the same charge leads to the decrease of adsorption capacity. Some studies have also shown that the adsorption of Cr6+ by soil decreases with the increase in pH value.
3.2 Oxidation–reduction potential
Redox potential is the comprehensive embodiment of the chemical reactions of various oxidants and reducing substances in farmland soil, representing the relative degree of soil oxidation and reduction, reflecting the index of redox state of farmland soil, affecting farmland soil pH, organic matter, and soil heterogeneity, and also the key factor affecting the activity of heavy metals.63 Khalid et al. pointed out that water-soluble cadmium increased with the increase of redox potential in soil-suspended matter.64 For heavy metals with variable valencies, such as Cr, its existing form in the soil is greatly affected by Eh, so its adsorption capacity in the soil is greatly affected by Eh.8
Reddy et al. found that with the increase of soil Eh, the content of soluble lead in soil decreased due to the combination of lead in soil with high-valent iron and manganese hydroxides under oxidizing conditions, which reduced the solubility.65 Therefore, Lindsay and Sadiq introduced the concept of PE + pH to explain better the change of iron and manganese oxides at different Eh and pH. With the decrease of PE + pH, iron oxides change from amorphous form with strong adsorption capacity to microcrystalline form (lepidocrocite γ-FeOOH, goethite α-FeOOH) with relatively weak adsorption capacity.66
Guo et al. found that when Eh was (−216)–(−50) mV, large free Fe2+ appeared in the sediment. With the reduction and dissolution of iron and manganese oxides, the original adsorbed and stable heavy metal ions were released into the soil solution.67 In addition, Vodyanitskii et al. found that when Eh is (−75)–150 mV, obligate anaerobes such as the genus desulfovibrio vibrio reduce SO42− to H2S, which promotes the conversion of high-valent sulfate compounds to low-valent sulfides, thus reducing the activity of heavy metals.68
3.3 Temperature and freezing–thaw
The temperature of the reaction system is also one of the critical environmental factors affecting the adsorption–desorption of heavy metals. According to the change of adsorption–desorption caused by the change of temperature, the thermodynamic parameters of the adsorption reaction can be calculated. According to the thermodynamic parameters, the exothermic process and endothermic process of the response can be judged, as well as the reaction's spontaneity. The endothermic or exothermic reactions of heavy metals in soils vary with soil types, possibly due to the different components of different farmland soils. In general, the study of adsorption–desorption of heavy metals in soil should be carried out at a constant temperature, and the temperature should be recorded simultaneously to better explain the experimental results.
Aharoni's research based on the principle of thermodynamics showed that the adsorption of heavy metals increased with the increase of soil temperature.69 Since then, some scholarshave used the improved Freundlich equation to describe the effect of time and temperature on zinc adsorption, which is as follow:70,71
|
S = acb1(A(−E/RT)t)b2
| (1) |
where,
S is the adsorption capacity (μmol g
−1);
C is the zero-point concentration of zinc in the solution (μmol L
−1)and
b1 is the slope of the Freundlich adsorption curve;
E is the activation energy (kJ mol
−1);
R is the gas constant;
T is the temperature (K);
t is the incubation time (d);
a and
b2 are coefficients, where
b1 = 0.58283.
70
Freezing–thaw also occurs when the soil temperature drops below zero and rises above zero, resulting in freezing and thawing. Soil freezing–thaw is a common natural phenomenon in the high and middle latitudes of the Northern Hemisphere. China is the world's third largest country of frozen soil, and the frozen soil areas are mainly distributed in Northeast and the Qinghai-Tibet Plateau.72 It is generally believed that the amount of adsorption and desorption of heavy metals in unfrozen soil is higher than that in freezing–thawing soil,73 which is the same as Christense's finding that the destruction of soil aggregates under the action of freezing–thawing alternation increases the organic matter in soil.74 However, Li and Wu found that under the same solid–liquid ratio, the adsorption properties of soil to Pb and Cr were different under different freezing–thawing conditions, most of the adsorption kinetic models of soil to Cr under freezing and thawing conditions were faster than those under unfrozen conditions, and most of the adsorption amounts of soil to Cr under freezing and thawed conditions were higher than those under unfrozen conditions.75 Dang et al. found that the adsorption of cadmium in the soil with one, three and six freezing–thawing cycles was 84, 74, and 70%, respectively.40 Compared with the soil without the freezing–thaw cycle, about 33.1–50.0% of Cd in the soil without the freezing–thaw cycle was desorbed at the same initial Cd concentration. However, about 32.1–73.0% of Cd was desorbed from the freezing–thawing soil, indicating that the higher the frequency of freezing–thawing, the higher the desorption rate of Cd. It can be seen that freezing–thaw has a certain impact on soil's physical and chemical properties, thus affecting the adsorption and desorption of heavy metals.
4 Competitive adsorptions
In practice, the contaminated farmland soil often contains not only one heavy metal, but also a mixture of multiple heavy metals. Different heavy metal ions may interact with each other, resulting in different characteristics from a single metal ion.76
Abd-Elfattah and Wada studied the competitive adsorption of several heavy metals on seven soils with different mineral composition characteristics, and evaluated the particular adsorption order of heavy metals in soils according to the distribution coefficient.77 Two general selective adsorption sequences were obtained: Cr > Pb > Cu > Cd > Zn > Ni and Pb > Cr > Cu > Cd > Ni > Zn. The adsorption capacity of trivalent metal Cr is more vital than that of divalent metal. Bibak studied the competitive adsorption of Cu, Zn and Ni on oxisols, and found that the presence of Cu seriously inhibited the adsorption of Zn and Ni, while the presence of Zn and Ni had little effect on the adsorption of Cu.78 Jalali et al. found that competition had a specific impact on heavy metals' adsorption, reducing the adsorption of Cd and Cu, and more Ni and Zn were adsorbed by soil regardless of pH value and solid–liquid ratio.79 Campillo-Cora's competition experiment showed that the retention rate of Cu, Zn, Ni and Pb was always higher than that of the competition experiment, but Cr showed a synergistic effect in the competition.80 According to the Langmuir constant, the changing trend of the maximum adsorption capacity of soil obtained by Fonseca below is: Cu ≈ Zn > Cd > Pb > Cr. In the dynamic system, the reaction is not in equilibrium, so the change trend of the retardation factor is different: Zn > Cd > Pb > Cu > Cr.81 The competitive adsorption of Cu and Zn by soils was significantly correlated with the electronegativity of ions, the first-order hydrolysis coefficient, the ratio of charge to a radius, and the Misono softness coefficient.82
Competitive adsorption between heavy metals does not necessarily reduce their retention in the soil, as it depends on the concentration, pH, and type of heavy metal involved. At the same time, it was found that the adsorption capacity of some metal ions decreased with the addition of ionic strength, which may be due to the formation of outer ring compounds. The adsorption capacity of some metal ions increased with the increase of ionic strength or did not change with the change of ionic strength. This may be due to the reaction of ions with soil surface functional groups to form inner ring compound.83 At the same time, the competitive adsorption of heavy metals was also affected by organic matter content, mineral composition, pH, CEC and ionic composition of soil solution. Covelo et al. studied the competitive adsorption kinetics of Cr, Cu, Pb, Cd, Ni, and Zn ions in four humus soils, and found that the adsorption process of Cr, Cu and Pb was very fast, while the other three were slower.84 Li's study found that the competition effect of Pb on Cd mainly occurred on SOM, not on clay minerals.85 Therefore, the competitive effect of Pb on Cd should not be ignored in soils with high organic matter content. Heidmann et al. studied the competitive adsorption of Cu and Pb on kaolinite. They found that the presence of Pb reduced the adsorption of Cu, and the higher the concentration of Pb, the greater the degree of reduction.86
5 Environmental and external factors
It is well known that crop roots absorb heavy metals from the soil and deposit them in crops. Finally, it is served on the table and eaten by people. Similarly, microorganisms compete with other soil components to adsorb heavy metal ions. The results show that the adsorption of Cd2+ by bacterial cells is more significant than that by minerals such as montmorillonite. Thereby reducing the adsorption amount of the heavy metals in the soil. Biochar can also adsorb heavy metals in soil. At present, many researchers are studying heavy metals' adsorption and desorption behavior on the surface of microplastics. However, the purpose of this paper is to explore the adsorption of heavy metals in soil. Therefore, the adsorption of heavy metals in the soil will not be repeated.
5.1 Microplastics
In the 1950s, the plastic film began to be used in agriculture. In the 1970s, China introduced the plastic film and promoted it in large quantities. Now it has become the largest user of plastic film. By 2017, the use of plastic film has reached 1.47 million tons, accounting for 90% of the world's total. Microplastics are a new type of non-degradable environmental pollutants with particle size D < 5 mm, which are ubiquitous in the environment, produce a series of ecological and environmental toxicity effects, and have become a global environmental hot issue. Plastic fragments or particles with a particle size of <5 mm were first proposed by the National Oceanic and Atmospheric Administration in 2009. The soil microplastics' sources in soil can be divided into primary microplastics (PMPs) and secondary microplastics (SMPs) according to their production pathways. PMPs refer to the particles, such as plastic beads added in cosmetics or plastic particles as industrial raw materials, discharged into soil through rivers, sewage treatment plants, etc.;87 SMPs are plastic particles formed by the fragmentation of large plastic waste through weathering, which reduces its volume.88 As shown in the Fig. 2, the microplastics entering the soil environment through various ways are gradually distributed from the surface layer of the soil ecosystem to the deep soil: the activities of soil animals such as feeding and excretion and the elongation of plant roots can promote the downward transport of plastics with small particle size.89 MPs entering deep soil can penetrate aquifers and may enter groundwater. On the one hand, MPs in soil can adsorb or desorb other environmental pollutants through physical or chemical effects, on the other hand, MPs can directly or indirectly affect soil aggregates.90
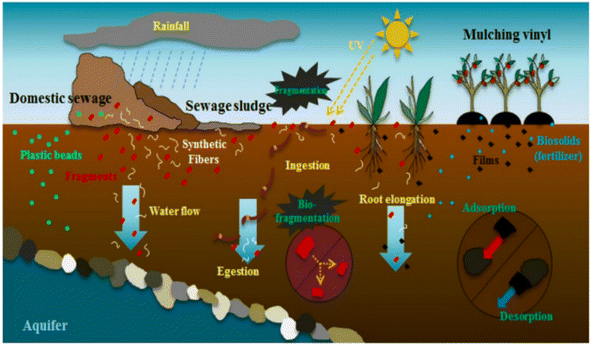 |
| Fig. 2 Sources and distribution of plastic waste in soil environment.90 | |
There are few studies on the changes in soil physical and chemical properties under the action of microplastics and their effects on the adsorption of heavy metals. Zhang and Liu found that 72% of the soil plastic particles were related to soil aggregates and participated in the formation of soil aggregates in the farmland soil of Dianchi Lake in Yunnan Province, and 28% of the plastic particles were dispersed in the ground.91 The study on the morphology of plastics further shows that fiber microplastics are mainly involved in the aggregation of soil macro aggregates. Film and fragment microplastics are dominant in the collection of soil macro aggregates. Rillig and Lehmann pointed out that microplastics have an essential impact on the structure and function of soil microbial communities.92 Microplastics participating in soil aggregate aggregation will affect the physical and chemical properties of soil. Bai et al. found that the formation of soil aggregates was mainly driven by the role of microorganisms, and this role was mutual.93 Any change in totals would in turn affect the structure and function of microorganisms, and jointly affect soil chemical properties. Machado et al. pointed out that microplastics of different materials have different changes in soil porosity and interaction forces between particles, such as polyacrylic fiber and polyethylene fragment density, although only 86% and 71% of polyester fiber density.94 At present, the effect of microplastics on soil physical and chemical properties is not enough to reflect its mechanism of action. The effect of microplastics with different particle sizes on soil physical and chemical properties can be considered in the future. The impact on heavy metal adsorption in soil can be explained by the change of soil physical, chemical properties.
5.2 Biochar
Biochar is a highly carbon-rich product produced by low-temperature pyrolysis of biomass under anoxic or anaerobic conditions. A certain amount of biochar will be applied to the soil when planting crops to keep the soil fertile. Biochar has the characteristics of rich pore structure, large specific surface area, high pH, diverse surface functional groups, high and stable carbon content, etc. It has a strong adsorption capacity for heavy metal ions.95 Wu et al. found that biochar could significantly enhance the Cd adsorption capacity of degraded alpine grassland soils in Northwest Sichuan.96
Mansoor et al. have shown that biochar can increase the adsorption capacity of heavy metals by affecting soil pH, CEC, organic matter and mineral composition.97 The pH value of biochar is generally above 8, which mainly depends on the ash content in the production process of biochar. The ash contains oxides or carbonates of Na+, K+, Mg2+ and Ca2+ cations, which are alkaline when dissolved in water,98 Houben et al. determined the concentrations of heavy metals Zn, Cd and Pb in 0.01 mol L−1 CaCl2 extraction solution, and found that the concentration of extraction solution decreased significantly with the increase of biochar dosage after different proportions of biochar were applied to soil for 1 h, which was also due to the fact that biochar could increase the pH value of soil.99
When biochar was introduced into soil, the soil would undergo complex aging processes, such as oxidation, surface coverage, dissolution and destruction of pore structure, thus changing the adsorption capacity of soil system for heavy metals. Puga et al. applied sugarcane straw biochar to heavy metal contaminated mining soil, and the concentrations of Zn, Cd and Pb were reduced by 54%, 56% and 50%, respectively.100 It also affected the activity of soil microorganisms. When biochar is added to soil, its porosity, surface characteristics and some nutrients can promote microbial reproduction, enhance microbial activity, and provide a larger space and attachment sites for the survival of microorganisms.101 Xu et al. found that biochar changed soil bacterial community structure and significantly increased bacterial diversity and microbial biomass, and there was a positive correlation between bacterial diversity and biochar addition ratio in biochar-amended soil.102
5.3 Vegetation-microorganisms
Different vegetation types and vegetation diversity directly or indirectly affect soil moisture, temperature, particle size and soil organic matter.103 Othert vegetation types and vegetation diversity directly or indirectly affect soil moisture, temperature, particle size and dirt organic matter.104 However, after continuous planting of the same crop, the structure of soil aggregates will be destroyed, making the soil hardened seriously, the soil pH generally shows a downward trend, soil fertility decreases, soil bulk density will also decrease, and even cause the phenomenon of soil salinization. Fischer et al. pointed out that species richness and plant functional groups had significant effects on soil moisture, and the composition of plant functional groups (species, richness of functional groups, appearance of a particular active group).105 And the content of soil organic carbon affects the soil water content. Wang et al. added three kinds of crop straws to two variable charge soils (pH values of 4.30 and 4.59) and a constant charge soil (pH value of 6.11).106 The results showed that the pH values of the two variable charge soils increased rapidly within 3 days of incubation, and then increased slightly. The pH values of the constant charge soil decreased with the extension of incubation time. At the same time, due to plant root respiration and root microbial trophic respiration, some studies have found that the Eh value of root soil is generally lower than that of non-root earth, but there are also some vegetation, such as rice and some wetland plants, whose roots have a particular function of oxygen secretion, and vice versa. Moreover, rhizosphere minerals also changed, affecting heavy metals adsorption.107 Courchesne and Gobran found that the abundance of rhizosphere minerals was significantly different from that of non-rhizosphere. After root action, the color of amorphous iron minerals became dark, while goethite became yellow.108
Regarding microorganisms, Huang et al. found that the surface area of kaolinite and soil colloids increased by 3.0–8.8% after mixing with bacteria, and bacteria could promote the adsorption of heavy metals Cu and Cd by kaolinite and soil colloids.109 At the same time, in the soil environment, especially in the micro-domain environment of the root–soil interface of vegetation, there is always a synergistic effect between roots and microorganisms. James reported that in the root–soil interface of vegetation, Cr6+ was not easily adsorbed by soil, while Cr3+ was readily adsorbed by soil or formed chromium hydroxide precipitate.110 Moreover, due to microbial metabolism, root secretion, decomposition of organic matter, dissolution of minerals, application of fertilizers and decomposition of organisms, there are a number of low molecular weight anionic ligands in soil, which can complex with heavy metals, thus affecting the adsorption process of heavy metals in soil. Such as oxalic acid, tartaric acid, citric acid, phthalates, water, salicylates, phosphates, and sulfates111,112 Ping et al. concluded through adsorption experiments that the presence of chloride ions in soil can increase the availability of lead and cadmium, while phosphate is a good stabilizer for lead and cadmium.113 Under the action of microorganisms, the decomposition of vegetation litter and the decay of plant roots can increase soil fertility, and also affect soil structure and properties. Xu et al. found that the decomposition process of plant residues in soil can lead to changes in soil organic matter properties.114
Similarly, the content of heavy metals in soils can affect vegetation-microorganisms. In general, the toxicity of heavy metals affects the species, biomass, population structure and physiological and biochemical properties of soil microorganisms and disrupts the normal composition of microbial compartments. The correlation between heavy metals Pb, Cd, Zn and As and microbial C-cycle related amylase, P-cycle related acid phosphatase and redox related catalase activities were found to be significant and positive (P < 0.05).115 When heavy metal contamination is severe, the effect on microorganisms in the environment changes from stimulation to toxicity, inhibiting their biomass. For example, Jose et al. found that heavy metal pollution reduced the diversity of bacterial distribution and the expression of microbial enzymes, and when the concentration of heavy metals (Zn, Co, Ni, Cu) was at 5 mmol L−1, the diversity of isolated bacteria decreased from 90–100% to 40%, and the secreted hydrolases decreased by 25%.116 At the microscopic level, the toxicity of heavy metals to microorganisms includes damage to cell membranes, alteration of enzyme specificity, disruption of cellular functions, and damage to the structure of DNA. For example, Zhou et al. found that bacterial cyclic DNA was damaged to varying degrees at concentrations of As3+: 0.05 mg L−1, Hg2+: 0.2 mg L−1, Cd2+, Cd3+ and Cu2+: 0.5 mg L−1, and Pb2+ and Zn2+: 1 mg L−1, respectively, when bacteria were isolated from contaminated water bodies for culture experiments.117
6 Conclusion and prospect
With the rapid development of the social economy and the improvement of people's living standards, heavy metal pollution in farmland soil and related ecological problems have become the focus of attention. So how to effectively repair heavy metal pollution has attracted more and more attention. At the same time, soil is a rich ecosystem, containing metal oxides, organic matter, clay minerals, pore water, microorganisms and so on. Adsorption–desorption, precipitation–dissolution, oxidation–reduction and other reactions occur, of which adsorption–desorption is the basis of these reactions, affecting the environmental behavior of heavy metals. It is closely related to the availability of nutrient elements in soil and the transformation and fate of pollutants. It can also characterize the process of soil genesis and formation and provide an essential basis for soil classification. Therefore, understanding the adsorption and desorption mechanism of heavy metals in farmland soil can provide theoretical basis and control measures for studying the environmental effects of heavy metals in farmland soil. To sum up, the current research progress on the influencing factors of heavy metal adsorption and desorption in the soil is reviewed from four aspects, and we believe that future research on freezing and thawing, microplastics and microorganisms should focus on:
(1) Freezing–thaw is a typical seasonal phenomenon in northern China's soil area, affecting ecosystems' biogeochemical processes in many ways. As abiotic stress, freezing–thaw can directly change soil aggregate, permeability, mechanical properties and microbial communities, and indirectly affect the cycling of carbon, nitrogen, phosphorus and other nutrients. However, there are still many gaps in the specific research on the impact of freezing–thaw on the adsorption and desorption of heavy metals in soil. However, with the change of global climate, the freeze–thaw phenomenon may not only exist in the northern region.
(2) Pay attention to the adsorption and desorption of heavy metals in soil by microplastics. In recent years, plastic products have been produced in large quantities. In 2017 alone, global plastic production reached 348 million tons, an annual increase of about 5%. These plastic wastes are degraded into tiny plastic particles by physical or photochemical processes and eventually form microplastics, but the research on the distribution characteristics of MPs in terrestrial environment, pollution status and the mechanism of adsorption and desorption of heavy metals is still about ten years behind the research on MPs in water environment. Therefore, strengthening the study on the adsorption and desorption of heavy metals in soil by microplastics may help to solve a series of current problems.
(3) In the real natural environment, there are many kinds of microorganisms, which are distributed in both bacterial and archaeal domains. At the same time, various “secretions” produced by microorganisms in the soil environment will also affect the adsorption and desorption of heavy metals in the soil. Therefore, this is a vast and unknown field that needs to be explored and studied.
Author contributions
Hanjing Yu and Qi Meng: conceptualization and visualization; Peng Dong: project administration; Jin Yan: methodology; Chenchen Li: formal analysis; Hanjing Yu and Yaoqiang Ma: writing—original draft preparation; Xinyu Zhou, Wanquan Yu and Huiying Kan: writing—review and editing; Ruosong Xie and Qi Meng: supervision. All authors were involved in writing and revising the manuscript.
Conflicts of interest
The authors declare that they have no known competing financial interests or personal relationships that could have appeared to influence the work reported in this paper.
Acknowledgements
This work was supported by the National Key Research Development Program of China (2019YFC1803501).
References
- Y. T. Yang, Y. Chen, F. Leng, L. Huang, Z. J. Wang and W. Q. Tian, Recent Advances on Surface Modification of Halloysite Nanotubes for Multifunctional Applications, Appl. Sci., 2017, 7(12) DOI:10.3390/app7121215.
- Z. Feng, H. Zhu, Q. Deng, Y. He, J. Li, J. Yin, F. Gao, R. Huang and T. Li, Environmental pollution induced by heavy metal(loid)s from pig farming, Environ. Earth Sci., 2018, 77(3) DOI:10.1007/s12665-018-7300-2.
- H. Hu, Q. Jin and P. Kavan, A Study of Heavy Metal Pollution in China: Current Status, Pollution-Control Policies and Countermeasures, Sustainability, 2014, 6(9), 5820–5838, DOI:10.3390/su6095820.
- H. Li, N. Luo, Y. W. Li, Q. Y. Cai, H. Y. Li, C. H. Mo and M. H. Wong, Cadmium in rice: transport mechanisms, influencing factors, and minimizing measures, Environ. Pollut., 2017, 224, 622–630, DOI:10.1016/j.envpol.2017.01.087.
- C. S. Qu, Z. W. Ma, J. Yang, Y. Liu, J. Bi and L. Huang, Human Exposure Pathways of Heavy Metals in a Lead-Zinc Mining Area, Jiangsu Province, China, PLoS One, 2012, 7(11) DOI:10.1371/journal.pone.0046793.
- J. F. Durand, The impact of gold mining on the Witwatersrand on the rivers and karst system of Gauteng and North West Province, South Africa, Journal of African Earth Sciences, 2012, 68, 24–43, DOI:10.1016/j.jafrearsci.2012.03.013; L. M. Huang, C. B. Deng, N. Huang and X. J. Huang, Multivariate statistical approach to identify heavy metal sources in agricultural soil around an abandoned Pb-Zn mine in Guangxi Zhuang Autonomous Region, China, Environ. Earth Sci., 2013, 68(5), 1331–1348, DOI:10.1007/s12665-012-1831-8.
- Q. C. Zhang and C. C. Wang, Natural and Human Factors Affect the Distribution of Soil Heavy Metal Pollution: a Review, Water, Air, Soil Pollut., 2020, 231(7) DOI:10.1007/s11270-020-04728-2.
- H. B. Bradl, Adsorption of heavy metal ions on soils and soils constituents, J. Colloid Interface Sci., 2004, 277(1), 1–18, DOI:10.1016/j.jcis.2004.04.005.
- S. Zhao, J. Wang, S. Feng, Z. Xiao and C. Chen, Effects of ecohydrological interfaces on migrations and transformations of pollutants: a critical review, Sci. Total Environ., 2022, 804, 150140, DOI:10.1016/j.scitotenv.2021.150140.
- M. Arias, C. Perez-Novo, F. Osorio, E. Lopez and B. Soto, Adsorption and desorption of copper and zinc in the surface layer of acid soils, J. Colloid Interface Sci., 2005, 288(1), 21–29, DOI:10.1016/j.jcis.2005.02.053.
- H. B. Guo and A. S. Barnard, Naturally occurring iron oxide nanoparticles: morphology, surface chemistry and environmental stability, J. Mater. Chem. A, 2013, 1(1), 27–42, 10.1039/c2ta00523a.
- A. Violante, E. Barberis, M. Pigna and V. Boero, Factors affecting the formation, nature, and properties of iron precipitation products at the soil-root interface, J. Plant Nutr., 2003, 26(10–11), 1889–1908, DOI:10.1081/PLN-120024252.
- A. M. Jones, R. N. Collins, J. Rose and T. D. Waite, The effect of silica and natural organic matter on the Fe(II)-catalysed transformation and reactivity of Fe(III) minerals, Geochim. Cosmochim. Acta, 2009, 73(15), 4409–4422, DOI:10.1016/j.gca.2009.04.025; E.-H. Han and R. B. Rebak, Book Reviews. Announcements, 1997, 15 3–4 533–559, DOI:10.1515/corrrev.1997.15.3-4.533.
- X. Li, N. J. D. Graham, W. Deng, M. Liu, T. Liu and W. Yu, The formation of planar crystalline flocs of γ-FeOOH in Fe(II) coagulation and the influence of humic acid, Water Res., 2020, 185, 116250, DOI:10.1016/j.watres.2020.116250.
- X. W. Xu, C. Chen, P. Wang, R. Kretzschmar and F. J. Zhao, Control of arsenic mobilization in paddy soils by manganese and iron oxides, Environ. Pollut., 2017, 231, 37–47, DOI:10.1016/j.envpol.2017.07.084.
- J. Zhang, P. Hua and P. Krebs, The influences of dissolved organic matter and surfactant on the desorption of Cu and Zn from road-deposited sediment, Chemosphere, 2016, 150, 63–70, DOI:10.1016/j.chemosphere.2016.02.015.
- J. E. Post, Manganese oxide minerals: crystal structures and economic and environmental significance, Proc. Natl. Acad. Sci. U. S. A., 1999, 96(7), 3447–3454, DOI:10.1073/pnas.96.7.3447.
- P. Yang, S. Lee, J. E. Post, H. F. Xu, Q. Wang, W. Q. Xu and M. Q. Zhu, Trivalent manganese on vacancies triggers rapid transformation of layered to tunneled manganese oxides (TMOs): implications for occurrence of TMOs in low-temperature environment, Geochim. Cosmochim. Acta, 2018, 240, 173–190, DOI:10.1016/j.gca.2018.08.014.
- S. Grangeon, B. Lanson and M. Lanson, Solid-state transformation of nanocrystalline phyllomanganate into tectomanganate: influence of initial layer and interlayer structure, Acta Crystallogr., Sect. B: Struct. Sci., Cryst. Eng. Mater., 2014, 70, 828–838, DOI:10.1107/S2052520614013687; S. Grangeon, A. Fernandez-Martinez, F. Warmont, A. Gloter, N. Marty, A. Poulain and B. Lanson, Cryptomelane formation from nanocrystalline vernadite precursor: a high energy X-ray scattering and transmission electron microscopy perspective on reaction mechanisms, Geochem. Trans., 2015, 16 DOI:10.1186/s12932-015-0028-y.
- G.-X. Sun, A. A. Meharg, G. Li, Z. Chen, L. Yang, S.-C. Chen and Y.-G. Zhu, Distribution of soil selenium in China is potentially controlled by deposition and volatilization?, Sci. Rep., 2016, 6(1), 1–9 CrossRef CAS PubMed; X. Xiao, Z. Shao and L. Yu, A perspective of the engineering applications of carbon-based selenium-containing materials, Chin. Chem. Lett., 2021, 32(10), 2933–2938, DOI:10.1016/j.cclet.2021.03.047.
- Z. Zhu, S. Sun and X. Jing, Carbon-based selenium: an easily fabricated environmental material for removing lead from the electrolytic wastewater, Chem. Pap., 2022, 76(1), 401–408 CrossRef CAS.
- J. Gailer, Arsenic–selenium and mercury–selenium bonds in biology, Coord. Chem. Rev., 2007, 251(1–2), 234–254 CrossRef CAS.
- M. K. Uddin, A review on the adsorption of heavy metals by clay minerals, with special focus on the past decade, Chem. Eng. J., 2017, 308, 438–462, DOI:10.1016/j.cej.2016.09.029.
- P. Sipos, T. Nemeth, V. K. Kis and I. Mohai, Sorption of copper, zinc and lead on soil mineral phases, Chemosphere, 2008, 73(4), 461–469, DOI:10.1016/j.chemosphere.2008.06.046.
- P. E. Jensen, L. M. Ottosen and A. J. Pedersen, Speciation of Pb in industrially polluted soils, Water, Air, Soil Pollut., 2006, 170(1–4), 359–382, DOI:10.1007/s11270-005-9008-7.
- E. F. Covelo, F. A. Vega and M. L. Andrade, Competitive sorption and desorption of heavy metals by individual soil components, J. Hazard. Mater., 2007, 140(1–2), 308–315, DOI:10.1016/j.jhazmat.2006.09.018.
- L. Dalacorte, P. A. V. Escosteguy and E. C. Bortoluzzi, Sorption of Copper and Zinc from Aqueous Solution by Metabasalt Residue and its Mineralogical Behavior, Water, Air, Soil Pollut., 2019, 230(4) DOI:10.1007/s11270-019-4141-x.
- T. H. Sodango, X. Li, J. Sha and Z. Bao, Review of the Spatial Distribution, Source and Extent of Heavy Metal Pollution of Soil in China: Impacts and Mitigation Approaches, Journal of Health and Pollution, 2018, 8(17), 53–70, DOI:10.5696/2156-9614-8.17.53.
- Z. R. Nan and C. Y. Zhao, Heavy metal concentrations in gray calcareous soils of Baiyin region, Gansu Province, PR China, Water, Air, Soil Pollut., 2000, 118(1–2), 131–141, DOI:10.1023/A:1005135618750.
- T. Adhikari and M. V. Singh, Sorption characteristics of lead and cadmium in some soils of India, Geoderma, 2003, 114(1–2), 81–92, DOI:10.1016/S0016-7061(02)00352-X.
- A. M. Ghrair, J. Ingwersen and T. Streck, Immobilization of heavy metals
in soils amended by nanoparticulate zeolitic tuff: Sorption-desorption of cadmium, J. Plant Nutr. Soil Sci., 2010, 173(6), 852–860, DOI:10.1002/jpln.200900053.
- M. Hamidpour, M. Kalbasi, M. Afyuni, H. Shariatmadari, P. E. Holm and H. C. B. Hansen, Sorption hysteresis of Cd(II) and Pb(II) on natural zeolite and bentonite, J. Hazard. Mater., 2010, 181(1–3), 686–691, DOI:10.1016/j.jhazmat.2010.05.067.
- K. Zhong, R. K. Xu, A. Z. Zhao, J. Jiang, D. Tiwari and H. Li, Adsorption and desorption of Cu(II) and Cd(II) in the tropical soils during pedogenesis in the basalt from Hainan, China, Carbonates Evaporites, 2010, 25(1), 27–34, DOI:10.1007/s13146-009-0003-8.
- B. Huang, Z. W. Li, J. Q. Huang, L. Guo, X. D. Nie, Y. Wang, Y. Zhang and G. M. Zeng, Adsorption characteristics of Cu and Zn onto various size fractions of aggregates from red paddy soil, J. Hazard. Mater., 2014, 264, 176–183, DOI:10.1016/j.jhazmat.2013.10.074.
- J. F. Chau, A. C. Bagtzoglou and M. R. Willig, The Effect of Soil Texture on Richness and Diversity of Bacterial Communities, Environ. Forensics, 2011, 12(4), 333–341, DOI:10.1080/15275922.2011.622348; S. Stanchi, E. Bonifacio, E. Zanini and E. Perfect, Chemical and Physical Treatment Effects on Aggregate Breakup in the 0- to 2-mm Size Range, Soil Sci. Soc. Am. J., 2008, 72(5), 1418–1421, DOI:10.2136/sssaj2007.0413N.
- L. Gove, C. M. Cooke, F. A. Nicholson and A. J. Beck, Movement of water and heavy metals (Zn, Cu, Pb and Ni) through sand and sandy loam amended with biosolids under steady-state hydrological conditions, Bioresour. Technol., 2001, 78(2), 171–179, DOI:10.1016/S0960-8524(01)00004-9.
- J. A. Acosta, A. F. Cano, J. M. Arocena, F. Debela and S. Martínez-Martínez, Distribution of metals in soil particle size fractions and its implication to risk assessment of playgrounds in Murcia City (Spain), Geoderma, 2009, 149(1), 101–109, DOI:10.1016/j.geoderma.2008.11.034; X. Bi, S. Liang and X. Li, A novel in situ method for sampling urban soil dust: particle size distribution, trace metal concentrations, and stable lead isotopes, Environ. Pollut., 2013, 177, 48–57, DOI:10.1016/j.envpol.2013.01.045; K. Ljung, O. Selinus, E. Otabbong and M. Berglund, Metal and arsenic distribution in soil particle sizes relevant to soil ingestion by children, Appl. Geochem., 2006, 21(9), 1613–1624, DOI:10.1016/j.apgeochem.2006.05.005; G. Xu, Z. Li and P. Li, Fractal features of soil particle-size distribution and total soil nitrogen distribution in a typical watershed in the source area of the middle Dan River, China, Catena, 2013, 101, 17–23, DOI:10.1016/j.catena.2012.09.013.
- M. Huang, Z. W. Li, B. Huang, N. L. Luo, Q. Zhang, X. Q. Zhai and G. M. Zeng, Investigating binding characteristics of cadmium and copper to DOM derived from compost and rice straw using EEM-PARAFAC combined with two-dimensional FTIR correlation analyses, J. Hazard. Mater., 2018, 344, 539–548, DOI:10.1016/j.jhazmat.2017.10.022.
- L.-Y. Zhang, L.-Q. Li, G.-X. Pan, L.-Q. Cui, H.-L. Li, X.-Y. Wu and J.-Q. Shao, Effects of heavy metals pollution on paddy soil aggregates composition and heavy metals distribution, J. Appl. Ecol., 2009, 20(11), 2806–2812 CAS; Y. Zhang, M. Chen, X. Zhang, F. He, X. Liu, R. Bian, K. Cheng, L. Li and G. Pan, Effects of Different Heavy Metal Pollution Levels on Soil Aggregates Composition in a Paddy Soil, Soils, 2017, 49(2), 337–344 Search PubMed.
- X. L. Dang, Y. L. Zhang, N. Yu and Y. L. Zhang, Cadmium adsorption-desorption of brown soil with freeze-thaw cycles in northeast China, Int. J. Environ. Pollut., 2012, 49(1–2), 89–99, DOI:10.1504/IJEP.2012.049770.
- F. Xiao, B. Gamiz and J. J. Pignatello, Adsorption and desorption of nitrous oxide by raw and thermally air-oxidized chars, Sci. Total Environ., 2018, 643, 1436–1445, DOI:10.1016/j.scitotenv.2018.06.280.
- Q. Y. Zhang, H. Y. Liu, F. Liu, X. H. Ju, F. Dinis, E. J. Yu and Z. Yu, Source Identification and Superposition Effect of Heavy Metals (HMs) in Agricultural Soils at a High Geological Background Area of Karst: A Case Study in a Typical Watershed, Int. J. Environ. Res. Public Health, 2022, 19(18) DOI:10.3390/ijerph191811374.
- G. Yuan and L. M. Lavkulich, Sorption behavior of copper, zinc, and cadmium in response to simulated changes in soil properties, Commun. Soil Sci. Plant Anal., 1997, 28(6–8), 571–587, DOI:10.1080/00103629709369812.
- C. Perez-Novo, M. Pateiro-Moure, E. Osorio, J. C. Novoa-Munoz, E. Lopez-Periago and M. Arias-Estevez, Influence of organic matter removal on competitive and noncompetitive adsorption of copper and zinc in acid soils, J. Colloid Interface Sci., 2008, 322(1), 33–40, DOI:10.1016/j.jcis.2008.03.002.
- Y. Jiang, X. L. Ma, F. Y. Sun, Y. X. Guo, L. J. Ren, Y. J. Wang and Z. L. Xie, A comparative study on the adsorption properties of heavy metal cr in lake sediment and soil, Appl. Ecol. Environ. Res., 2021, 19(2), 901–914, DOI:10.15666/aeer/1902_901914.
- L. I. U. Wei and W. Shutao, Review of Researches on Dissolved Organic Matter in Soil and Its Affecting Factors, J. Soil Sci., 2011, 42(4), 997–1002 Search PubMed.
- A. Zsolnay, Dissolved organic matter: artefacts, definitions, and functions, Geoderma, 2003, 113(3–4), 187–209, DOI:10.1016/S0016-7061(02)00361-0; B. W. Strobel, O. K. Borggaard, H. C. B. Hansen, M. K. Andersen and K. Raulund-Rasmussen, Dissolved organic carbon and decreasing pH mobilize cadmium and copper in soil, Eur. J. Soil Sci., 2005, 56(2), 189–196, DOI:10.1111/j.1365-2389.2004.00661.x; P. Hubova, V. Tejnecky, C. Ash, L. Boruvka and O. Drabek, Low-Molecular-Mass Organic Acids in the Forest Soil Environment, Mini-Rev. Org. Chem., 2017, 14(1), 75–84, DOI:10.2174/1570193X14666161130163034; O. K. Borggaard, P. E. Holm and B. W. Strobel, Potential of dissolved organic matter (DOM) to extract As, Cd, Co, Cr, Cu, Ni, Pb and Zn from polluted soils: a review, Geoderma, 2019, 343, 235–246, DOI:10.1016/j.geoderma.2019.02.041.
- K. Kalbitz, S. Solinger, J. H. Park, B. Michalzik and E. Matzner, Controls on the dynamics of dissolved organic matter in soils: a review, Soil Sci., 2000, 165(4), 277–304, DOI:10.1097/00010694-200004000-00001.
- E. Lipczynska-Kochany, Humic substances, their microbial interactions and effects on biological transformations of organic pollutants in water and soil: a review, Chemosphere, 2018, 202, 420–437, DOI:10.1016/j.chemosphere.2018.03.104.
- A. G. Zavarzina, N. N. Danchenko, V. V. Demin, Z. S. Artemyeva and B. M. Kogut, Humic Substances: Hypotheses and Reality (a Review), Eurasian Soil Sci., 2021, 54(12), 1826–1854, DOI:10.1134/S1064229321120164.
- P. Calvo, L. Nelson and J. W. Kloepper, Agricultural uses of plant biostimulants, Plant Soil, 2014, 383(1–2), 3–41, DOI:10.1007/s11104-014-2131-8.
- J. Zhang, H. L. Yin, H. Wang, L. Xu, B. Samuel, J. J. Chang, F. Liu and H. H. Chen, Molecular structure-reactivity correlations of humic acid and humin fractions from a typical black soil for hexavalent chromium reduction, Sci. Total Environ., 2019, 651, 2975–2984, DOI:10.1016/j.scitotenv.2018.10.165; Q. Liu, J. Tang, C. S. He and C. C. K. Chan, Use of Temperature Sensors in Testing Soil Humus Content in Saline Wetland in Response to Freeze-Thaw Cycles, Sens. Mater., 2020, 32(10), 3355–3372, DOI:10.18494/SAM.2020.2930; P. Conte, A. Agretto, R. Spaccini and A. Piccolo, Soil remediation: humic acids as natural surfactants in the washings of highly
contaminated soils, Environ. Pollut., 2005, 135(3), 515–522, DOI:10.1016/j.envpol.2004.10.006.
- K. Yang, G. F. Miao, W. H. Wu, D. H. Lin, B. Pan, F. C. Wu and B. S. Xing, Sorption of Cu2+ on humic acids sequentially extracted from a sediment, Chemosphere, 2015, 138, 657–663, DOI:10.1016/j.chemosphere.2015.07.061.
- M. Arias, M. T. Barral and J. C. Mejuto, Enhancement of copper and cadmium adsorption on kaolin by the presence of humic acids, Chemosphere, 2002, 48(10), 1081–1088, DOI:10.1016/S0045-6535(02)00169-8.
- P. Wang, F. J. Ding, Z. B. Huang, Z. Y. Fu, P. Zhao and S. H. Men, Adsorption behavior and mechanism of Cd(II) by modified coal-based humin, Environ. Technol. Innovation, 2021, 23 DOI:10.1016/j.eti.2021.101699.
- F. S. Miller, K. L. Kilminster, B. Degens and G. W. Firns, Relationship between Metals Leached and Soil Type from Potential Acid Sulphate Soils under Acidic and Neutral Conditions in Western Australia, Water, Air, Soil Pollut., 2010, 205(1–4), 133–147, DOI:10.1007/s11270-009-0061-5; K. M. Spark, J. D. Wells and B. B. Johnson, Characterizing trace metal adsorption on kaolinite, Eur. J. Soil Sci., 1995, 46(4), 633–640, DOI:10.1111/j.1365-2389.1995.tb01359.x; M. McBride, S. Sauve and W. Hendershot, Solubility control of Cu, Zn, Cd and Pb in contaminated soils, Eur. J. Soil Sci., 1997, 48(2), 337–346, DOI:10.1111/j.1365-2389.1997.tb00554.x.
- S. Sauve, M. McBride and W. Hendershot, Soil solution speciation of lead(II): effects of organic matter and pH, Soil Sci. Soc. Am. J., 1998, 62(3), 618–621, DOI:10.2136/sssaj1998.03615995006200030010x.
- F. Zeng, S. Ali, H. Zhang, Y. Ouyang, B. Qiu, F. Wu and G. Zhang, The influence of pH and organic matter content in paddy soil on heavy metal availability and their uptake by rice plants, Environ. Pollut., 2011, 159(1), 84–91, DOI:10.1016/j.envpol.2010.09.019; D. Fernandez-Calvino, M. Pateiro-Moure, J. C. Novoa-Munoz, B. Garrido-Rodriguez and M. Arias-Estevez, Zinc distribution and acid-base mobilisation in vineyard soils and sediments, Sci. Total Environ., 2012, 414, 470–479, DOI:10.1016/j.scitotenv.2011.10.033; X. W. Zhang, J. X. Tong, B. X. Hu and W. S. Wei, Adsorption and desorption for dynamics transport of hexavalent chromium (Cr(VI)) in soil column, Environ. Sci. Pollut. Res., 2018, 25(1), 459–468, DOI:10.1007/s11356-017-0263-0.
- J. Markiewiez-Patkowska, A. Hursthouse and H. Przybyla-Kij, The interaction of heavy metals with urban soils: sorption behaviour of Cd, Cu, Cr, Pb and Zn with a typical mixed brownfield deposit, Environ. Int., 2005, 31(4), 513–521, DOI:10.1016/j.envint.2004.09.004.
- W. Liu, L. D. Jin, J. Xu, J. Liu, Y. Y. Li, P. P. Zhou, C. C. Wang, R. A. Dahlgren and X. D. Wang, Insight into pH dependent Cr(VI) removal with magnetic Fe3S4, Chem. Eng. J., 2019, 359, 564–571, DOI:10.1016/j.cej.2018.11.192.
- J. O. Azeez, A. O. Hassan and T. B. Olowoboko, Differential Sorption Behavior of Cadmium, Lead, Zinc, and Copper in Some Tropical Soils and Their Environmental Implications, Commun. Soil Sci. Plant Anal., 2018, 49(14), 1707–1718, DOI:10.1080/00103624.2018.1474908.
- M. Uchimiya, D. Bannon, H. Nakanishi, M. B. McBride, M. A. Williams and T. Yoshihara, Chemical Speciation, Plant Uptake, and Toxicity of Heavy Metals in Agricultural Soils, J. Agric. Food Chem., 2020, 68(46), 12856–12869, DOI:10.1021/acs.jafc.0c00183.
- J. Rinklebe, S. Antic-Mladenovic, T. Frohne, H. J. Stark, Z. Tomic and V. Licina, Nickel in a serpentine-enriched fluvisol: redox affected dynamics and binding forms, Geoderma, 2016, 263, 203–214, DOI:10.1016/j.geoderma.2015.09.004.
- R. A. Khalid, R. P. Gambrell and W. H. Patrick Jr, Chemical Availability of Cadmium in Mississippi River Sediment, J. Environ. Qual., 1981, 10(4), 523–528, DOI:10.2134/jeq1981.00472425001000040021x.
- C. N. Reddy, A. Jugsujinda and W. H. Patrick Jr, System for Growing Plants under Controlled Redox Potential-PH Conditions1, Agron. J., 1976, 68(6), 987–989, DOI:10.2134/agronj1976.00021962006800060038x.
- W. L. Lindsay and M. Sadiq, Use of pe + pH to predict and interpret metal solubility relationships in soils, Sci. Total Environ., 1983, 28(1), 169–178, DOI:10.1016/S0048-9697(83)80016-3.
- S. H. Guo, Z. L. Liu, Q. S. Li, P. Yang, L. L. Wang, B. Y. He, Z. M. Xu, J. S. Ye and E. Y. Zeng, Leaching heavy metals from the surface soil of reclaimed tidal flat by alternating seawater inundation and air drying, Chemosphere, 2016, 157, 262–270, DOI:10.1016/j.chemosphere.2016.05.019.
- Y. N. Vodyanitskii, Effect of reduced iron on the degradation of chlorinated hydrocarbons in contaminated soil and ground water: a review of publications, Eurasian Soil Sci., 2014, 47(2), 119–133, DOI:10.1134/S1064229314020136; Y. Zhao, Z. J. Fu, X. F. Chen and G. Y. Zhang, Bioremediation Process and Bioremoval Mechanism of Heavy Metal Ions in Acidic Mine Drainage, Chem. Res. Chin. Univ., 2018, 34(1), 33–38, DOI:10.1007/s40242-018-7255-6.
- C. Aharoni and M. Ungarish, Kinetics of activated chemisorption. Part 3—Amount and distribution of adsorbate at varying temperatures and pressures, J. Chem. Soc., Faraday Trans. 1, 1977, 73, 1943–1950 RSC.
- N. Barrow and T. Shaw, The slow reactions between soil and anions: 3. The effects of time and temperature on the decrease in isotopically exchangeable phosphate, Soil Sci., 1975, 119(3), 190–197 CrossRef CAS.
- N. Barrow, Effects of time and temperature on the sorption of cadmium, zinc, cobalt, and nickel by a soil, Soil Res., 1998, 36(6), 941–950 CrossRef CAS.
- Y. H. Ran, X. Li, G. D. Cheng, T. J. Zhang, Q. B. Wu, H. J. Jin and R. Jin, Distribution of Permafrost in China: An Overview of Existing Permafrost Maps, Permafr. Periglac. Process., 2012, 23(4), 322–333, DOI:10.1002/ppp.1756.
- X. Wang, Y. M. Li, N. Mao, Y. Q. Zhou and P. Guo, The Adsorption Behavior of Pb2+ and Cd2+ in the Treated Black Soils with Different Freeze-Thaw Frequencies, Water, Air, Soil Pollut., 2017, 228(5) DOI:10.1007/s11270-017-3376-7.
- S. Christensen and B. T. Christensen, Organic matter available for denitrification in different soil fractions: effect of freeze/thaw cycles and straw disposal, J. Soil Sci., 1991, 42(4), 637–647, DOI:10.1111/j.1365-2389.1991.tb00110.x.
- L. M. Li and J. Wu, Lead and Chromium Immobilization Process Subjected to Different Freeze-Thaw Treatments in Soils of the Northeastern Qinghai-Tibet Plateau, J. Chem., 2021 DOI:10.1155/2021/5286278.
- H. A. Elliott, M. R. Liberati and C. P. Huang, Competitive Adsorption of Heavy Metals by Soils, J. Environ. Qual., 1986, 15(3), 214–219, DOI:10.2134/jeq1986.00472425001500030002x.
- A. L. Y. Abd-Elfattah and K. Wada, Adsorption of lead, copper, zinc, cobalt, and cadmium by soils that differ in cation-exchange materials, J. Soil Sci., 1981, 32(2), 271–283, DOI:10.1111/j.1365-2389.1981.tb01706.x.
- A. Bibak, Competitive sorption of copper, nickel, and zinc by an oxisol, Commun. Soil Sci. Plant Anal., 2008, 28(11–12), 927–937, DOI:10.1080/00103629709369843.
- M. Jalali, Z. Vafaee and R. Fakhri, Selectivity Sequences of Heavy Metals in Single and Competitive Systems under Different Soil/Solution Ratios and pH in a Calcareous Soil, Commun. Soil Sci. Plant Anal., 2020, 51(3), 341–351, DOI:10.1080/00103624.2019.1709483.
- C. Campillo-Cora, M. Conde-Cid, M. Arias-Estévez, D. Fernández-Calviño and F. Alonso-Vega, Specific Adsorption of Heavy Metals in Soils: Individual and Competitive Experiments, Agronomy, 2020, 10, 13–21, DOI:10.3390/agronomy10081113.
- B. Fonseca, H. Figueiredo, J. Rodrigues, A. Queiroz and T. Tavares, Mobility of Cr, Pb, Cd, Cu and Zn in a loamy sand soil: a comparative study, Geoderma, 2011, 164(3), 232–237, DOI:10.1016/j.geoderma.2011.06.016.
- K. Flogeac, E. Guillon and M. Aplincourt, Competitive sorption of metal ions onto a north-eastern France soil. Isotherms and XAFS studies, Geoderma, 2007, 139(1), 180–189, DOI:10.1016/j.geoderma.2007.01.016.
- M. B. McBride, A Critique of Diffuse Double Layer Models Applied to Colloid and Surface Chemistry, Clays Clay Miner., 1997, 45(4), 598–608, DOI:10.1346/CCMN.1997.0450412.
- E. F. Covelo, M. L. Andrade and F. A. Vega, Heavy metal adsorption by humic umbrisols: selectivity sequences and competitive sorption kinetics, J. Colloid Interface Sci., 2004, 280(1), 1–8, DOI:10.1016/j.jcis.2004.07.024.
- Y. R. Li, J. Liu, Y. H. Wang, X. J. Tang, J. M. Xu and X. M. Liu, Contribution of components in natural soil to Cd and Pb competitive adsorption: semi-quantitative to quantitative analysis, J. Hazard. Mater., 2023, 441 DOI:10.1016/j.jhazmat.2022.129883.
- I. Heidmann, I. Christl, C. Leu and R. Kretzschmar, Competitive sorption of protons and metal cations onto kaolinite: experiments and modeling, J. Colloid Interface Sci., 2005, 282(2), 270–282, DOI:10.1016/j.jcis.2004.08.019.
- Q. Sun, S. Y. Ren and H. G. Ni, Incidence of microplastics in personal care products: an appreciable part of plastic pollution, Sci. Total Environ., 2020, 742 DOI:10.1016/j.scitotenv.2020.140218.
- W. R. Waldman and M. C. Rillig, Microplastic Research Should Embrace the Complexity of Secondary Particles, Environ. Sci. Technol., 2020, 54(13), 7751–7753, DOI:10.1021/acs.est.0c02194.
- E. H. Lwanga, H. Gertsen, H. Gooren, P. Peters, T. Salanki, M. van der Ploeg, E. Besseling, A. A. Koelmans and V. Geissen, Microplastics in the Terrestrial Ecosystem: Implications for Lumbricus terrestris (Oligochaeta, Lumbricidae), Environ. Sci. Technol., 2016, 50(5), 2685–2691, DOI:10.1021/acs.est.5b05478.
- Y. Chae and Y.-J. An, Current research trends on plastic pollution and ecological impacts on the soil ecosystem: a review, Environ. Pollut., 2018, 240, 387–395, DOI:10.1016/j.envpol.2018.05.008.
- G. S. Zhang and Y. F. Liu, The distribution of microplastics in soil aggregate fractions in southwestern China, Sci. Total Environ., 2018, 642, 12–20, DOI:10.1016/j.scitotenv.2018.06.004.
- M. C. Rillig and A. Lehmann, Microplastic in terrestrial ecosystems, Science, 2020, 368(6498), 1430–1431, DOI:10.1126/science.abb5979.
- N. L. Bai, H. L. Zhang, S. Zhou, H. F. Sun, Y. H. Zhao, X. Q. Zheng, S. X. Li, J. Q. Zhang and W. G. Lv, Long-term effects of straw return and straw-derived biochar amendment on bacterial communities in soil aggregates, Sci. Rep., 2020, 10(1) DOI:10.1038/s41598-020-64857-w.
- A. A. D. Machado, C. W. Lau, J. Till, W. Kloas, A. Lehmann, R. Becker and M. C. Rillig, Impacts of Microplastics on the Soil Biophysical Environment, Environ. Sci. Technol., 2018, 52(17), 9656–9665, DOI:10.1021/acs.est.8b02212.
- J. X. Sun, L. Q. Cui, G. X. Quan, J. L. Yan, H. Wang and L. M. Wu, Effects of Biochar on Heavy Metals Migration and Fractions Changes with Different Soil Types in Column Experiments, Bioresources, 2020, 15(2), 4388–4406, DOI:10.15376/biores.15.2.4388-4406; F. Jing, C. Chen, X. Chen, W. Liu, X. Wen, S. Hu, Z. Yang, B. Guo, Y. Xu and Q. Yu, Effects of wheat straw derived biochar on cadmium availability in a paddy soil and its accumulation in rice, Environ. Pollut., 2020, 257, 113592, DOI:10.1016/j.envpol.2019.113592; L. Beesley, E. Moreno-Jiménez, J. L. Gomez-Eyles, E. Harris, B. Robinson and T. Sizmur, A review of biochars' potential role in the remediation, revegetation and restoration of contaminated soils, Environ. Pollut., 2011, 159(12), 3269–3282, DOI:10.1016/j.envpol.2011.07.023.
- C. Wu, Y. Li, M. Chen, X. Luo, Y. Chen, N. Belzile and S. Huang, Adsorption of Cadmium on Degraded Soils Amended with Maize-Stalk-Derived Biochar, Int. J. Environ. Res. Public Health, 2018, 15, 21–34, DOI:10.3390/ijerph15112331.
- S. Mansoor, N. Kour, S. Manhas, S. Zahid, O. A. Wani, V. Sharma, L. Wijaya, M. N. Alyemeni, A. A. Alsahli and H. A. El-Serehy, et al., Biochar as a tool for effective management of drought and heavy metal toxicity, Chemosphere, 2021, 271, 129458, DOI:10.1016/j.chemosphere.2020.129458.
- M. Uchimiya, L. H. Wartelle, K. T. Klasson, C. A. Fortier and I. M. Lima, Influence of Pyrolysis Temperature on Biochar Property and Function as a Heavy Metal Sorbent in Soil, J. Agric. Food Chem., 2011, 59(6), 2501–2510, DOI:10.1021/jf104206c.
- D. Houben, L. Evrard and P. Sonnet, Mobility, bioavailability and pH-dependent leaching of cadmium, zinc and lead in a contaminated soil amended with biochar, Chemosphere, 2013, 92(11), 1450–1457, DOI:10.1016/j.chemosphere.2013.03.055.
- A. P. Puga, C. A. Abreu, L. C. A. Melo and L. Beesley, Biochar application to a contaminated soil reduces the availability and plant uptake of zinc, lead and cadmium, J. Environ. Manage., 2015, 159, 86–93, DOI:10.1016/j.jenvman.2015.05.036.
- B. Glaser, L. Haumaier, G. Guggenberger and W. Zech, The 'Terra Preta' phenomenon: a model for sustainable agriculture in the humid tropics, Naturwissenschaften, 2001, 88(1), 37–41, DOI:10.1007/s001140000193.
- N. Xu, G. Tan, H. Wang and X. Gai, Effect of biochar additions to soil on nitrogen leaching, microbial biomass and bacterial community structure, Eur. J. Soil Biol., 2016, 74, 1–8, DOI:10.1016/j.ejsobi.2016.02.004.
- G. Rodríguez-Loinaz, M. Onaindia, I. Amezaga, I. Mijangos and C. Garbisu, Relationship between vegetation diversity and soil functional diversity in native mixed-oak forests, Soil Biol. Biochem., 2008, 40(1), 49–60, DOI:10.1016/j.soilbio.2007.04.015.
- Q. Zhang, Z. W. Li, B. Huang, N. L. Luo, L. Z. Long, M. Huang, X. Q. Zhai and G. M. Zeng, Effect of land use pattern change from paddy soil to vegetable soil on the adsorption-desorption of cadmium by soil aggregates, Environ. Sci. Pollut. Res., 2017, 24(3), 2734–2743, DOI:10.1007/s11356-016-7853-0.
- C. Fischer, S. Leimer, C. Roscher, J. Ravenek, H. de Kroon, Y. Kreutziger, J. Baade, H. Bessler, N. Eisenhauer and A. Weigelt, et al., Plant species richness and functional groups have different effects on soil water content in a decade-long grassland experiment, J. Ecol., 2019, 107(1), 127–141, DOI:10.1111/1365-2745.13046.
- Y. F. Wang, C. X. Tang, J. J. Wu, X. M. Liu and J. M. Xu, Impact of organic matter addition on pH change of paddy soils, J. Soils Sediments, 2013, 13(1), 12–23, DOI:10.1007/s11368-012-0578-x.
- W. Jiang, P. C. Struik, J. Lingna, H. Van Keulen, Z. Ming and T. J. Stomph, Uptake and distribution of root-applied or foliar-applied 65Zn after flowering in aerobic rice, Ann. Appl. Biol., 2007, 150(3), 383–391, DOI:10.1111/j.1744-7348.2007.00138.x.
- F. Courchesne and G. R. Gobran, Mineralogical Variations of Bulk and Rhizosphere Soils from a Norway Spruce Stand, Soil Sci. Soc. Am. J., 1997, 61(4), 1245–1249, DOI:10.2136/sssaj1997.03615995006100040034x.
- Q. Y. Huang, W. L. Chen and L. H. Xu, Adsorption of copper and cadmium by Cu- and Cd-resistant bacteria and their composites with soil colloids and kaolinite, Geomicrobiol. J., 2005, 22(5), 227–236, DOI:10.1080/01490450590947779.
- B. R. James, Peer reviewed: the challenge of remediating chromium-contaminated soil, Environ. Sci. Technol., 1996, 30(6), 248A–251A, DOI:10.1021/es962269h.
- Y. S. Hwang and J. J. Lenhart, Adsorption of C4-Dicarboxylic Acids at the Hematite/Water Interface, Langmuir, 2008, 24(24), 13934–13943, DOI:10.1021/la801793p.
- S. O. Lee, T. Tran, B. H. Jung, S. J. Kim and M. J. Kim, Dissolution of iron oxide using oxalic acid, Hydrometallurgy, 2007, 87(3–4), 91–99, DOI:10.1016/j.hydromet.2007.02.005.
- L. I. U. Ping, X. U. Minggang and S. Zhengguo, Effects of Accompanying Anions on Adsorption-desorption of Pb and Cd by Two Typical Soils of China, J. Agro-Environ. Sci., 2007, 26(1), 252–256 Search PubMed.
- M. G. Xu, Y. L. Lou, X. L. Sun, W. Wang, M. Baniyamuddin and K. Zhao, Soil organic carbon active fractions as early indicators for total carbon change under straw incorporation, Biol. Fertil. Soils, 2011, 47(7), 745–752, DOI:10.1007/s00374-011-0579-8.
- J. Yang, F. Yang, Y. Yang, G. Xing, C. Deng, Y. Shen, L. Luo, B. Li and H. Yuan, A proposal of “core enzyme” bioindicator in long-term Pb-Zn ore pollution areas based on topsoil property analysis, Environ. Pollut., 2016, 213, 760–769 CrossRef CAS PubMed.
- J. Jose, R. Giridhar, A. Anas, P. L. Bharathi and S. Nair, Heavy metal pollution exerts reduction/adaptation in the diversity and enzyme expression profile of heterotrophic bacteria in Cochin estuary, India, Environ. Pollut., 2011, 159(10), 2775–2780 CrossRef CAS PubMed.
- Z. Sheng, W. Chaohai, L. Chaodeng and W. Haizhen, Damage to DNA of effective microorganisms by heavy metals: impact on wastewater treatment, J. Environ. Sci., 2008, 20(12), 1514–1518 CrossRef PubMed.
|
This journal is © The Royal Society of Chemistry 2023 |
Click here to see how this site uses Cookies. View our privacy policy here.