DOI:
10.1039/D2RA07510E
(Paper)
RSC Adv., 2023,
13, 8890-8900
Synthesis of tetrazoles catalyzed by a new and recoverable nanocatalyst of cobalt on modified boehmite NPs with 1,3-bis(pyridin-3-ylmethyl)thiourea†
Received
25th November 2022
, Accepted 1st March 2023
First published on 17th March 2023
Abstract
In the first part of this work, boehmite nanoparticles (BNPs) were synthesized from aqueous solutions of NaOH and Al(NO3)3·9H2O. Then, the BNPs surface was modified using 3-choloropropyltrimtoxysilane (CPTMS) and then 1,3-bis(pyridin-3-ylmethyl)thiourea ((PYT)2) was anchored on the surface of the modified BNPs (CPTMS@BNPs). In the final step, a complex of cobalt was stabilized on its surface (Co-(PYT)2@BNPs). The final obtained nanoparticles were characterized by FT-IR spectra, TGA analysis, SEM imaging, WDX analysis, EDS analysis, and XRD patterns. In the second part, Co-(PYT)2@BNPs were used as a highly efficient, retrievable, stable, and organic–inorganic hybrid nanocatalyst for the formation of organic heterocyclic compounds such as tetrazole derivatives. Co-(PYT)2@BNPs as a novel nanocatalyst are stable and have a heterogeneous nature; therefore, they can be recovered and reused again for several consecutive runs without any re-activation.
1 Introduction
In recent years, boehmite nanoparticles (BNPs) have attracted interest from both practical and fundamental viewpoints.1,2 In fact, boehmite is aluminum oxyhydroxide (γ-AlOOH) and it is the most stable phase of alumina after gibbsite.3–6 Boehmite consists of double sheets of oxygen octahedron with Al-atoms at their centers.7–10 The boehmite sheets themselves are composed of octahedral chains with a cubic orthorhombic unit cell.2,11 Also, BNPs are very stable and they are not moisture or air sensitive.12,13 Therefore, BNPs can synthesized in aqueous media without inert atmosphere by available materials such as inexpensive aluminum salts.14 The physical and chemical properties of boehmite are strongly dependent on the experimental condition of its synthesis.13 For example, BNPs were synthesized by different methods such as hydrolysis of aluminum salts,2 precipitation in an aqueous solution from aluminum salt solutions,15 hydrothermal procedures,2 solid state decomposition of gibbsite,16 sol–gel procedures,17 and solvothermal procedures.2 Boehmite contains high aggregation of hydroxyl groups on its surface, that supply suable places for modify of its surface with other functional groups such as electrophilic or nucleophilic sites which are enable to immobilization of suitable ligands or metal complexes.18–22 Therefore BNPs can be used as an excellent support for fabrication of wide range of heterogeneous catalysts.2 BNPs were utilized as support for stabilization of acidic,23 basic,24 metallic catalysts25,26 and organo- or ionic22 supported catalysts. More addition, boehmite nanoparticle have several unique attributes such as good surface area, easy availability, non-toxicity, chemical resistance, mechanical strength, thermal stability, good conductivity, high hardness, low cost, excellent biocompatibility, high abrasive and corrosion resistance.1,2,22 However, BNPs are also have some disadvantages, such as impurities content (e.g. nitrate ions) that led to lower their crystallinity. This impurities concentration may affect properties of the surface property and pore structure of boehmite. In the other hand, BNPs may converts into a γ-Al2O3 in the high temperatures, but this cannot effect on the catalysis application of BNPs in organic reactions. Because organic reactions take place at temperatures lower than the BNPs phase change. Therefore, Boehmite nanomaterials have also attracted attention in absorbent,27 coatings,28 flame retardant,29 optical material,30 ceramics,31 vaccine adjuvants,32 cosmetic products,2,33 pillared clays and sweep-flocculation for fresh water treatment.13 Consequently, we investigated a new complex of cobalt with 1,3-bis(pyridin-3-ylmethyl)thiourea on boehmite nanoparticle (Co-(PYT)2@BNPs) as a reusable nanocatalyst in the synthesis of tetrazole derivatives. Because tetrazole compounds are an important group of medicinal and organic compounds which possess many uses in several fields such as coordination chemistry, synthetic organic chemistry, drugs, medicinal chemistry as surrogates for carboxylic acids, the photographic industry, catalysis technology, and organometallic chemistry as effective stabilizers of metallopeptide structures.34–41
2 Experimental
2.1 Materials and instruments
Solvents and chemical materials in this project bought from Iranian companies, Aldrich, Merck or Fluka and used sans any purification.
The particle morphology and particle diameters of synthesized catalyst studied via FESEM-TESCAN MIRA III Scanning-Electron-Microscope (SEM) from Czechia. In addition, FESEM-TESCAN MIRA III used for type, content and number of elements (via WDX and SEM-EDS analysis) of the nanocatalyst. XRD diffraction of the nanocatalyst recorded by a PW1730 device madding Philips Company of Netherlands. IR spectra recorded using KBr pills in a VRTEX 70 model Bruker IR spectrometer. TGA diagram of the nanocatalyst recorded by a SDT Q600 V20.9 Build 20 Thermal Analysis device under air atmosphere in the temperature range of 30–800 °C. NMR spectra of the tetrazoles registered via Bruker-DRX-400 spectrometer.
2.2 Synthesis of 1,3-bis(pyridin-3-ylmethyl)thiourea ((PYT)2) ligand (3)
In a round-bottomed flask, 3-(aminomethyl)pyridine (1, 10 mmol) added to CS2 (5 mmol) in H2O and stirred at room temperature for 7 h (Scheme 1). The reaction progress consecutively checked by TLC (EtOAc: n-hexane, 1
:
2). Since this reaction is exothermic, the temperature increases during the reaction and so this temperature is sufficient for release H2S (confirmed by smell and blackening of lead acetate paper). After performance of the reaction, the water-insoluble product filtered, and then recrystallized from hot water and ethanol (1
:
1 v/v).
 |
| Scheme 1 Synthesis of (PYT)2 ligand (3). | |
The structure of (PYT)2 ligand was characterized by 1H NMR and FT-IR spectroscopies:
2.2.1 1,3-bis(pyridin-3-ylmethyl)thiourea ((PYT)2). 1H NMR (400 MHz, DMSO-d6): δH = 5.50 (s, 2H), 8.46–8.44 (d, J = 8 Hz, 2H), 8.22 (br, 2H), 7.69–7.66 (d, J = 12 Hz, 2H), 7.37–7.33 (d of d, J = 8 Hz, J = 4 Hz, 2H), 4.69 (s, 4H) ppm.IR (KBr) cm−1: 3272, 3184, 3000, 2923, 2853, 2359, 1913, 1529, 1473, 1422, 1298, 1237, 1193, 1101, 1027, 973, 918, 805, 770, 708, 616, 535.
2.3 Synthesis of the catalyst
50 mL of aqueous solution of sodium hydroxide (6.490 g) was added to 30 mL of aqueous solution of aluminum nitrate (20 g) as drop to drop under vigorous stirring. The resulting milky mixture was transferred in the ultrasonic bath (for 3 h at room temperature). The resulted BNPs was filtered and washed by distilled water. The obtained BNPs were kept in the oven at 220 °C for 4 h. Then, BNPs were modified by (3-chloropropyl)triethoxysilane (CPTMS) to preparation of CPTMS@BNPs. The CPTMS@BNPs formed matching to reported method in literature.41,42 As reported, the BNPs (1.5 g) dispersed in normal hexane, and then CPTMS (2 mL) injected and the mixture stirred for 24 h under reflux conditions that the modified BNPs by CPTMS (CPTMS@BNPs) were produced. The prepared CPTMS@BNPs were filtered, washed by ethanol (EtOH) and dried at room temperature. In order to immobilization of (PYT)2 ligand (3) on CPTMS@BNPs, 1 g of CPTMS@BNPs refluxed with (PYT)2 in toluene for 40 h. After then, obtained (PYT)2@BNPs isolated via filtration, washed by DMSO and EtOH, afterward dried at 60 °C. Finally, (PYT)2@BNPs (1 g) was dispersed in EtOH, and then Co(NO3)2·6H2O injected to the obtained mixture and then stirred for 24 h under reflux conditions. The resulting catalyst (Co-(PYT)2@BNPs) filtered, washed and dried at 60 °C (Scheme 2).
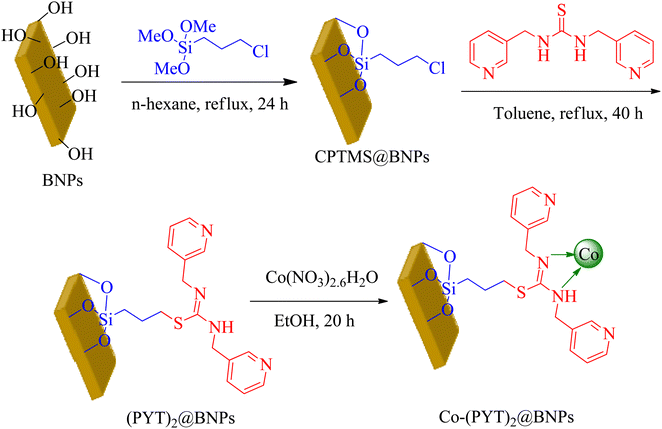 |
| Scheme 2 Synthesis of Co-(PYT)2@BNPs. | |
2.4 General procedure for the synthesis of tetrazoles catalyzed by Co-(PYT)2@BNPs
[3 + 2] cycloaddition of NaN3 with organic nitrile derivatives was used for the formation of tetrazoles in the attendance of Co-(PYT)2@BNPs as nanocatalyst. In this regard, NaN3 (1.4 mmol) and nitrile (1 mmol) stirred in the attendance of Co-(PYT)2@BNPs (50 mg) in PEG-400 (2 mL) at 120 °C. In the end of the reaction (which checked by TLC), the mixture cooled and was dilute by H2O and ethyl acetate. Co-(PYT)2@BNPs nanocatalyst isolated via simple filtration. Then, HCl (10 mL, 4 N) added and tetrazoles extracted in ethyl acetate. The ethyl acetate solvent dried by anhydrous sodium sulfate and then evaporated (Scheme 3).
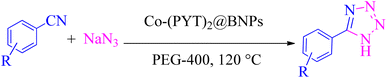 |
| Scheme 3 Synthesis of tetrazoles in the attendance of Co-(PYT)2@BNPs. | |
2.5 Spectral data
2.5.1 5-Phenyl-1H-tetrazole. 1H NMR (400 MHz, DMSO-d6): δH = 16.89 (br, 1H), 8.06–8.03 (d of d, J = 8 Hz, J = 4 Hz, 2H), 7.63–7.58 (m, 3H) ppm.
2.5.2 5-(3-nitrophenyl)-1H-tetrazole. 1H NMR (400 MHz, DMSO-d6): δH = 17.39 (br, 1H), 8.85–84 (t, J = 4 Hz, 1H), 8.50–8.47 (d of t, J = 12 Hz, J = 4 Hz, 1H), 8.45–8.41 (d of q, J(d) = 8 Hz, J(q) = 4 Hz, 1H), 7.94–7.89 (t, J = 12 Hz, 1H) ppm. 13C NMR (400 MHz, DMSO-d6): δH = 153.9, 147.1, 131.9, 130.0, 125.2, 124.3, 120.3 ppm. IR (KBr) cm−1: 3439, 3092, 2923, 2856, 2700, 1734, 1620, 1527, 1464, 1374, 1161, 1070, 991, 864, 816, 728, 665, 449.
2.5.3 2-(1H-tetrazol-5-yl)phenol. 1H NMR (400 MHz, DMSO-d6): δH = 7.99–7.96 (d of d, J = 12 Hz, J = 4 Hz, 1H), 7.42–7.37 (t of d, J = 12 Hz, 1H), 7.07–7.04 (d, J = 12 Hz, 1H), 7.02–6.96 (t, J = 12 Hz, 1H) ppm. 13C NMR (400 MHz, DMSO-d6): δC = 155.3, 151.8, 132.5, 128.9, 119.7, 116.3, 110.6 ppm. IR (KBr) cm−1:3253, 3058, 2941, 2708, 2565, 1892, 1735, 1610, 1546, 1476, 1393, 1358, 1294, 1230, 1150, 1114, 1067, 808, 742, 681, 538, 465.
3 Results and discussion
3.1 Characterization of the catalyst
At first step, functionalized BNPs by (3-chloropropyl)trimethoxysilane (CPTMS) was produced based on new reported strategy.41,42 Subsequently, a new complex of cobalt was fabricated on the surface of functionalized BNPs (Co-(PYT)2@BNPs). The catalytic activity of Co-(PYT)2@BNPs was confirmed in the synthesis of tetrazoles. This nanocatalyst was characterized using Scanning Electron Microscope (SEM), X-ray diffraction (XRD), Fourier transform infrared spectroscopy (FT-IR), wavelength dispersive X-ray spectroscopy (WDX), energy dispersive X-ray spectroscopy (EDS), and thermogravimetric analysis (TGA) techniques.
The shape, morphology, and diameters size of Co-(PYT)2@BNPs studied by FESEM-TESCAN MIRA III Scanning Electron Microscope (SEM) devoice. The SEM images of Co-(PYT)2@BNPs illustrated in Fig. 1. As indicate, the particles of Co-(PYT)2@BNPs formed in uniform spherical shapes and quite homogeneous diameter less than 70 nm.
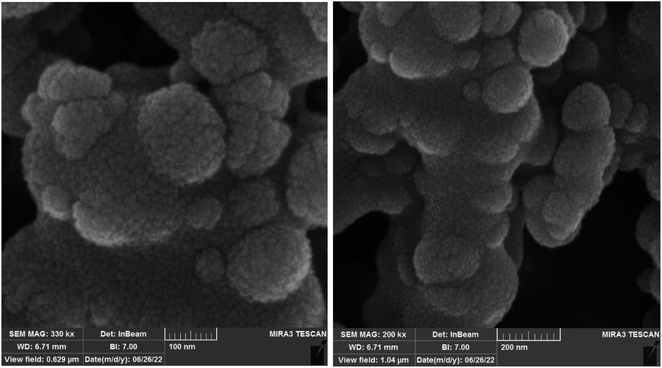 |
| Fig. 1 SEM images of Co-(PYT)2@BNPs. | |
The obtained results from energy-dispersive X-ray spectroscopy (EDS) analysis of Co-(PYT)2@BNPs are summarized in Fig. 2. As shown, Co-(PYT)2@BNPs is organize from aluminum, oxygen, silicon, nitrogen, carbon, sulfur and cobalt elements. As accepted, the intensity peaks of Al and O elements is sharped than other elements which are formed skeleton of BNPs. Also, the presence of Si, C, N, S and Co elements indicate the successful stabilization of the cobalt complex on BNPs. Also, wavelength dispersive X-ray spectroscopy (WDX) analysis (Fig. 3) illustrate homogeneous distribution of aluminum, oxygen, silicon, nitrogen, carbon, sulfur and cobalt elements in the structure of Co-(PYT)2@BNPs.
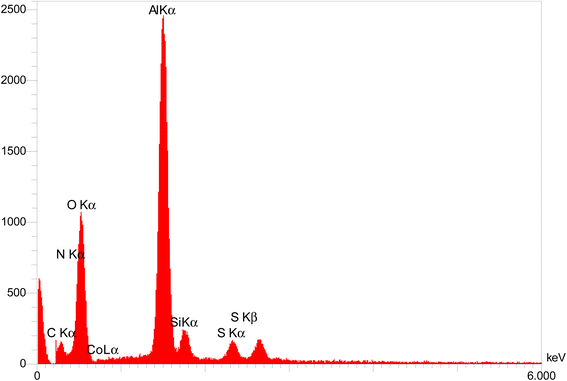 |
| Fig. 2 EDS diagram of Co-(PYT)2@BNPs. | |
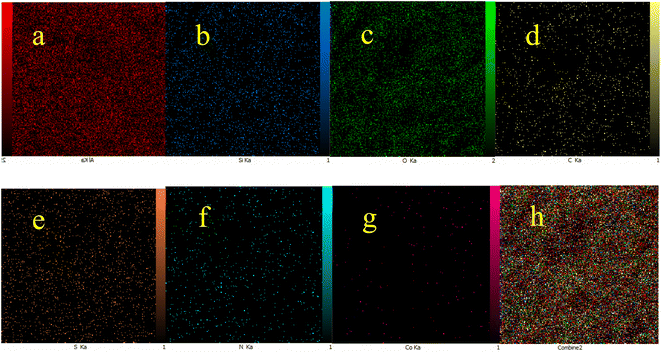 |
| Fig. 3 Elemental mapping of (a) aluminum, (b) silicon, (c) oxygen, (d) carbon, (e) sulfur, (f) nitrogen, (g) cobalt and (h) combine of all elements for Co-(PYT)2@BNPs. | |
TGA analysis can used to determine amount of organic and inorganic content in an organic–inorganic composite samples and also can employed to calculate the thermal stability of materials. Therefore, TGA analysis of Co-(PYT)2@BNPs was performed from 25 °C to 800 °C within increasing temperature rate of 10 °C min−1 under air atmosphere (Fig. 4). In TGA diagram of Co-(PYT)2@BNPs, a small weight losses (8% of weight) up to 150 °C is corresponded to the evaporation of solvents.43 As shown, any weight loss was not indicate up to 250 °C except evaporation of solvents which showed excellent thermal stability of Co-(PYT)2@BNPs. Therefore Co-(PYT)2@BNPs can be used as catalyst under hard conditions in wide range of organic reactions. TGA analysis of Co-(PYT)2@BNPs illustrated a considerable mass loss (35% of weight) between 250–650 °C which due to the decomposition of immobilized organic layers on the surface of modified BNPs.44
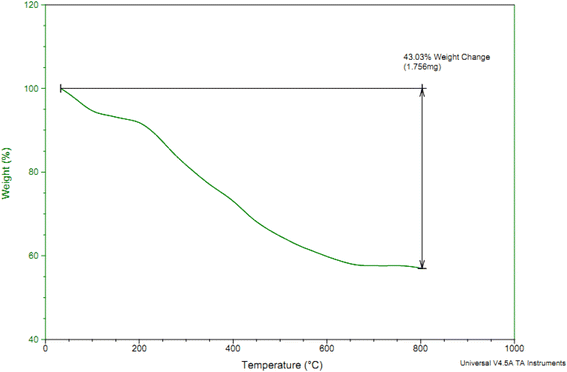 |
| Fig. 4 TGA diagram of Co-(PYT)2@BNPs. | |
X-ray diffraction (XRD) pattern of Co-(PYT)2@BNPs is obtained with Cu Kα radiation (λ = 0.154 nm). As shown in Fig. 5, the XRD pattern of Co-(PYT)2@BNPs shows several peaks of 2θ = 14.69 (0 2 0), 27.89 (1 2 0), 40.34° (0 3 1), 46.84° (1 3 1), 49.89° (0 5 1), 53.99° (2 0 0), 56.54° (1 5 1), 58.59° (0 8 0), 63.74° (2 3 1), 65.64° (0 0 2), 67.74° (1 7 1), and 72.89° (2 5 1) that confirm BNPs is stable in orthorhombic unit cell2,4 after stabilization of cobalt complex. The intensity of all peaks was decreased than BNPs due to the chemical modifications of BNPs.33 Also, a broad peak of 2θ from 15° to 25° related to the amorphous SiO2.45 Also, XRD pattern of Co-(PYT)2@BNPs showed four peaks at 2θ = 15.79° (1 1 0), 32.44° (2 2 0), 54.19° (1 4 1) and 63.14° (5 0 3) which can be related to Cobalt(II) species.33
 |
| Fig. 5 Normal XRD pattern of Co-(PYT)2@BNPs. | |
The FT-IR spectrum of CPTMS@BNPs, (b) (PYT)2@BNPs, and (c) Co-(PYT)2@BNPs shown in Fig. 6. Bands vibration at low wavenumbers <750 cm−1 in the FT-IR spectra related to the vibrations of the Al–O bonds.4 O–H and N–H bands appeared above 3000 cm−1 in the FT-IR spectra.46 In addition, the stretching vibrations of Si–O identified in region 805 cm−1 and 1075 cm−1 of FT-IR spectra.41,47 In addition, stretching vibrations of the C
N groups have appeared in the 1635 cm−1 region.4,48
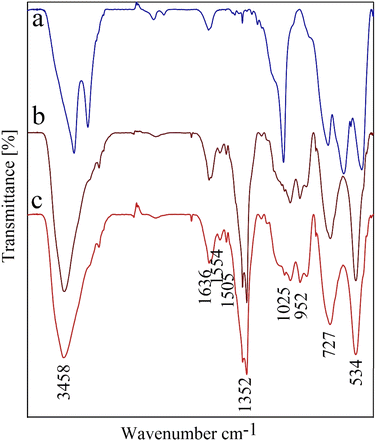 |
| Fig. 6 FT-IR spectra of (a) CPTMS@BNPs, (b) (PYT)2@BNPs, and (c) Co-(PYT)2@BNPs. | |
3.2 Catalytic studying of the catalyst
After characterization of Co-(PYT)2@BNPs, it was used as efficient, recyclable and biocompatible nanocatalyst in the synthesis of tetrazole heterocyclic compounds. The best reaction conditions obtained through [3 + 2] cycloaddition of NaN3 and benzonitrile as model reaction (Table 1). The model reaction did not taken place in the absent of Co-(PYT)2@BNPs nanocatalyst (Table 1, entry 1). While, the presentence of Co-(PYT)2@BNPs is required for the synthesis of 5-substituted 1H-tetrazole heterocyclic compounds. As expected, the model reaction occurs with the addition of catalyst and it faster proceeded by increasing in amount of Co-(PYT)2@BNPs catalyst. As shown, the model reaction completed within acceptable time when the amount of catalyst increased up to 50 mg (Table 1, entry 3). Among of several solvents (such as H2O, DMSO and PEG-400) which are examined, PEG-400 was provided the best results in term of reaction time and isolated yield of the pure product (Table 1, entry 3). Also, the effect of equivalent amount of NaN3 to benzonitrile and temperature on the model reaction was studied, which the best results were obtained with 1.4 mmol of NaN3 per 1 mmol of benzonitrile at 120 °C (Table 1, entry 3).
Table 1 Optimizing the best conditions for the synthesis of tetrazoles in the presence of Co-(PYT)2@BNPs nanocatalyst
Entry |
Amount of the catalyst (mg) |
Solvent |
NaN3 (mmol) |
Time (min) |
Temperature (°C) |
Yield (%)a |
Isolated yield within 120 min. No reaction. |
1 |
— |
PEG |
1.4 |
150 |
120 |
N. R.b |
2 |
40 |
PEG |
1.4 |
310 |
120 |
85 |
3 |
50 |
PEG |
1.4 |
100 |
120 |
98 |
4 |
50 |
PEG |
1.3 |
120 |
120 |
80 |
5 |
50 |
DMSO |
1.4 |
100 |
120 |
81 |
6 |
50 |
H2O |
1.4 |
100 |
Reflux |
20 |
7 |
50 |
PEG |
1.4 |
100 |
100 |
49 |
The scope of catalytic application of Co-(PYT)2@BNPs nanocatalyst was extended in the [3 + 2] cycloaddition of NaN3 and other benzonitrile derivatives (Table 2). In this regard, several benzonitrile compounds with an electron-withdrawing or electron-donating groups on para- meta- or ortho-position of aromatic ring were examined under optimized reaction conditions in hand. As shown in Table 2, all corresponding heterocyclic tetrazoles were produced in good yields. Also, phthalonitrile was employed as nitrile substrate which has two similar cyano groups on 1,2 position of its aromatic ring (Table 2, entry 4). As shown in Table 2 (entry 4), this methodology was provided only monoaddition which may be related to steric hindrance or selectivity of this catalyst. Also [1,1′-biphenyl]-4-carbonitrile (4-phenyl benzonitrile) was synthesized based on recently reported literature49 and it was investigated in the [3 + 2] cycloaddition reaction with NaN3 (Table 2, entry 11).
Table 2 Synthesis of 5-substituted 1H-tetrazole derivatives catalyzed by Co-(PYT)2@BNPs nanocatalyst
Entry |
Nitrile |
Product |
Time (min) |
Yield (%)a |
Melting point |
Reference |
Isolated yield. |
1 |
 |
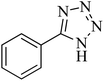 |
120 |
98 |
214–215 |
36 |
2 |
 |
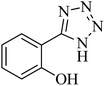 |
180 |
94 |
223–226 |
41 |
3 |
 |
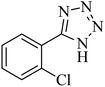 |
200 |
95 |
179–181 |
36 |
4 |
 |
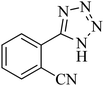 |
50 |
93 |
210–211 |
41 |
5 |
 |
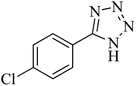 |
190 |
96 |
261–262 |
36 |
6 |
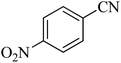 |
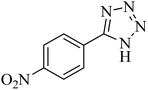 |
405 |
98 |
217–220 |
40 |
7 |
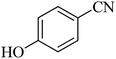 |
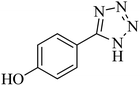 |
50 |
93 |
229–231 |
40 |
9 |
 |
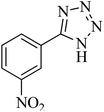 |
360 |
95 |
149–151 |
44 |
10 |
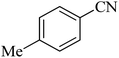 |
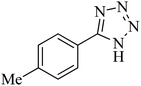 |
16 h |
89 |
247–249 |
50 and 51 |
11 |
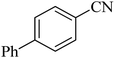 |
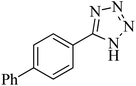 |
46 h |
71 |
245–248 |
52 and 53 |
Based on reported authentic methodologies about synthesis of tetrazoles in the presence of immobilized transition metal catalysts,46,54 a mechanism cycle for the synthesis of tetrazoles in the presence of Co-(PYT)2@BNPs catalyst offered in Scheme 4.
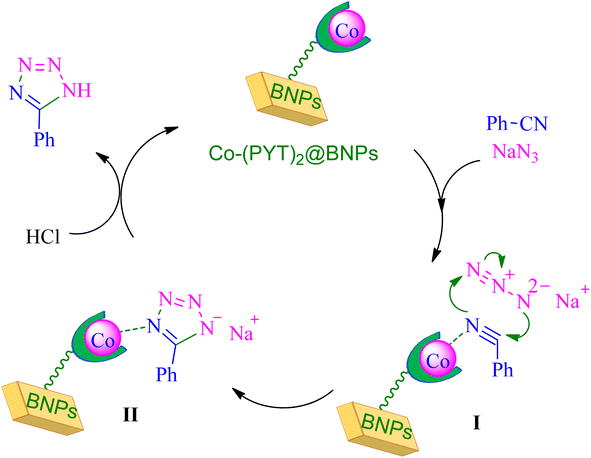 |
| Scheme 4 Expected mechanism for the synthesis of tetrazoles in the presence of Co-(PYT)2@BNPs nanocatalyst. | |
3.3 Reusability of the catalyst
As mentioned, Co-(PYT)2@BNPs catalyst is stable and it has heterogeneity nature. Therefore the reusability and retrievability of Co-(PYT)2@BNPs nanocatalyst were investigated in the [3 + 2] cycloaddition of benzonitrile and NaN3 for the synthesis of 5-phenyl-1H-tetrazole. As shown in Fig. 7, Co-(PYT)2@BNPs catalyst can be recovered and reused up to 6 runs without any further activation.
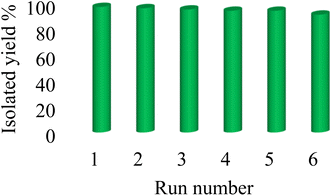 |
| Fig. 7 The reusability of Co-(PYT)2@BNPs in the synthesis of 5-phenyl-1H-tetrazole. | |
3.4 Comparison of the catalyst
The efficiency and advantages of Co-(PYT)2@BNPs catalyst than previous reported catalysts were compared in the [3 + 2] cycloaddition of benzonitrile with sodium azide in the presence of Co-(PYT)2@BNPs and previous catalysts (Table 3). As shown, Co-(PYT)2@BNPs catalyst afford 98% of 5-phenyl-1H-tetrazole product in 2 h which is better than previous reported catalysts in terms of time and yields. Also, some of previous catalysts have several disadvantages, limitations or drawbacks such as low yield of the products, long reaction times, expensive catalysts, non-environmental conditions, non or difficult separation of the catalysts and utilize hazard solvents. While, in this work, the synthesis of tetrazoles was introduced in the presence of Co-(PYT)2@BNPs as reusable catalyst in green solvent such as PEG, in short reaction time with acceptable yield.
Table 3 Comparison results of Co-(PYT)2@BNPs nanocatalyst with other catalysts for synthesis of 5-phenyl-1H-tetrazole
Entry |
Catalyst |
Time (h) |
Yield (%) |
Ref. |
1 |
CoY zeolite |
14 |
90 |
37 |
2 |
Cu–Zn alloy nanopowder |
10 |
95 |
55 |
3 |
B(C6F5)3 |
8 |
94 |
56 |
4 |
Fe3O4@SiO2/Salen Cu(II) |
7 |
90 |
57 |
5 |
Fe3O4/ZnS HNSs |
24 |
81.1 |
58 |
6 |
Pd-isatin-boehmite |
8 |
94 |
59 |
7 |
Mesoporous ZnS |
36 |
86 |
60 |
8 |
AgNO3 |
5 |
83 |
61 |
9 |
CuFe2O4 |
12 |
82 |
62 |
10 |
Nano ZnO/Co3O4 |
12 |
90 |
63 |
11 |
Pd-SMTU@boehmite |
2.5 |
95 |
64 |
12 |
Cu-TBA@biochar |
7 |
98 |
41 |
13 |
L-cysteine-Pd@MCM-41 |
3 |
98 |
65 |
14 |
Ni-MP(AMP)2@Fe-biochar |
3.8 |
92 |
34 |
15 |
Cu(II)-adenine-MCM-41 |
5 |
92 |
66 |
16 |
Pd-Arg@boehmite |
7 |
97 |
36 |
17 |
Cu-DABP@Fe3O4/MCM-41 |
2 |
99 |
46 |
18 |
Fe3O4@boehmite NPs |
4 |
97 |
67 |
19 |
Co-(PYT)2@BNPs |
2 |
98 |
This work |
4 Conclusions
In Conclusion, we synthesized a new stabilized complex of cobalt on modified boehmite NPs by 1,3-bis(pyridin-3-ylmethyl)thiourea (Co-(PYT)2@BNPs) as highly practical, retrievable, stable, and maintainable organic–inorganic hybrid nanocatalyst. Co-(PYT)2@BNPs was characterized by various techniques such as XRD, TGA, SEM, EDS, WDX and FT-IR. Catalytic activity of this catalyst was studied in the formation of organic heterocyclic compounds such as tetrazole derivatives. Co-(PYT)2@BNPs display high activity, stability and recyclability in the synthesis of tetrazoles.
Conflicts of interest
There are no conflicts to declare.
Acknowledgements
Pecuniary support for this work by the research affairs of Islamic Azad University, Qeshm Branch, Qeshm, Iran is gratefully acknowledged. Also, authors appreciate Ilam University for pecuniary support of this research project.
References
- J. Karger-Kocsis and L. Lendvai, Polymer/boehmite nanocomposites: A review, J. Appl. Polym. Sci., 2018, 135, 45573 CrossRef
. - M. Mohammadi, M. Khodamorady, B. Tahmasbi, K. Bahrami and A. Ghorbani-Choghamarani, Boehmite nanoparticles as versatile support for organic–inorganic hybrid materials: Synthesis, functionalization, and applications in eco-friendly catalysis, J. Ind. Eng. Chem., 2021, 97, 1 CrossRef CAS
. - Y. Xie, D. Kocaefe, Y. Kocaefe, J. Cheng and W. Liu, The Effect of Novel Synthetic Methods and parameters Control on Morphology of nano-alumina Particles, Nanoscale Res. Lett., 2016, 11, 259 CrossRef PubMed
. - A. Jabbari, P. Moradi, M. Hajjami and B. Tahmasbi, Tetradentate copper complex supported on boehmite nanoparticles as an efficient and heterogeneous reusable nanocatalyst for the synthesis of diaryl ethers, Sci. Rep., 2022, 12, 11660 CrossRef CAS PubMed
. - L. Rajabi and A. A. Derakhshan, Room Temperature Synthesis of Boehmite and Crystallization of Nanoparticles: Effect of Concentration and Ultrasound, Sci. Adv. Mater., 2010, 2, 163 CrossRef CAS
. - M. F. Peintinger, M. J. Kratz and T. Bredow, Quantum-chemical study of stable, meta-stable and high-pressure alumina polymorphs and aluminum hydroxides, J. Mater. Chem. A, 2014, 2, 13143 RSC
. - S. Bruhne, S. Gottlieb, W. Assmus, E. Alig and M. U. Schmidt, Atomic structure analysis of nanocrystalline boehmite AlO(OH), Cryst. Growth Des., 2008, 8, 489 CrossRef
. - J. Karger-Kocsis and L. Lendvai, Polymer/boehmite nanocomposites: A review, J. Appl. Polym. Sci., 2018, 135, 45573 CrossRef
. - A. Ghorbani-Choghamarani, M. Hajjami, B. Tahmasbi and N. Noori, Boehmite silica sulfuric acid: as a new acidic material and reusable heterogeneous nanocatalyst for the various organic oxidation reactions, J. Iran. Chem. Soc., 2016, 13, 2193–2202 CrossRef CAS
. - J. Fankhanel, D. Silbernagl, M. Ghasem Zadeh Khorasani, B. Daum, A. Kempe, H. Sturm and R. Rolfes, Mechanical Properties of Boehmite Evaluated by Atomic Force Microscopy Experiments and Molecular Dynamic Finite Element Simulations, J. Nanomater., 2016, 2016, 13 Search PubMed
. - A. Ghorbani-Choghamarani, Z. Heidarnezhad and B. Tahmasbi, New Complex of Copper on Boehmite Nanoparticles as Highly Efficient and Reusable Nanocatalyst for Synthesis of Sulfides and Ethers, ChemistrySelect, 2019, 4, 1 CrossRef
. - M. Faisal, Z. U. Rehman, Q. Aein and A. Saeed, Terpyridine-Pr-Fe3O4@boehmite nanoparticles; a novel and highly effective magnetic nanocatalyst for preparation of cyclic carbonates from carbon dioxide and epoxides under solventless conditions, Mater. Chem. Phys., 2019, 231, 272 CrossRef CAS
. - R. P. C. Rajan, V. Sekar and V. Sivan, Boehmite an Efficient and Recyclable Acid–Base Bifunctional Catalyst for Aldol Condensation Reaction, J. Nanosci. Nanotechnol., 2018, 18, 4270 CrossRef PubMed
. - A. Ghorbani-Choghamarani, P. Moradi and B. Tahmasbi, Modification of boehmite nanoparticles with Adenine for the immobilization of Cu(II) as organic–inorganic hybrid nanocatalyst in organic reactions, Polyhedron, 2019, 163, 98 CrossRef CAS
. - D. Mishra, S. Anand, R. K. Panda and R. P. Das, Hydrothermal preparation and characterization of boehmites, Mater. Lett., 2000, 42, 38 CrossRef CAS
. - L. Candela and D. D. Perlmutter, Kinetics of boehmite formation by thermal decomposition of gibbsite, Ind. Eng. Chem. Res., 1992, 31, 694 CrossRef CAS
. - M. Nguefack, A. F. Popa, S. Rossignol and C. Kappenstein, Preparation of alumina through a sol–gel process. Synthesis, characterization, thermal evolution and model of intermediate boehmite, Phys. Chem. Chem. Phys., 2003, 5, 4279 RSC
. - D. G. Lewis and V. C. Farmer, Infrared absorption of surface hydroxyl groups and lattice vibrations in lepidocrocite (γ-FeOOH) and boehmite (γ-AlOOH), Clay Miner., 1986, 21, 93 CrossRef CAS
. - Y. Jia, B. S. Zhu, Z. Jin, B. Sun, T. Luo, X. Y. Yu, L. T. Kong and J. H. Liu, Fluoride removal mechanism
of bayerite/boehmite nanocomposites: Roles of the surface hydroxyl groups and the nitrate anions, J. Colloid Interface Sci., 2015, 440, 60 CrossRef CAS PubMed
. - P. Raybaud, M. Digne, R. Iftimie, W. Wellens, P. Euzen and H. Toulhoat, Morphology and Surface Properties of Boehmite (γ-AlOOH): A Density Functional Theory Study, J. Catal., 2001, 201, 236 CrossRef CAS
. - S. A. Dickie and A. J. McQuillan, In Situ Infrared Spectroscopic Studies of Adsorption Processes on Boehmite Particle Films: Exchange of Surface Hydroxyl Groups Observed upon Chelation by Acetylacetone, Langmuir, 2004, 20, 11630 CrossRef CAS PubMed
. - A. Ghorbani-Choghamarani, Z. Seydyosefi and B. Tahmasbi, Tribromide ion supported on boehmite nanoparticles as a reusable catalyst for organic reactions, C. R. Chim., 2018, 21, 1011 CrossRef CAS
. - R. Doosti, M. Bakherad, M. Mirzaee and K. Jadidi, Boehmite Silylpropyl Amine Sulfamic Acid as an Efficient and Recyclable Catalyst for the Synthesis of some Pyrazole Derivatives, Lett. Org. Chem., 2017, 14, 450 CrossRef CAS
. - A. Ghorbani-Choghamarani, Z. Heidarnezhad, B. Tahmasbi and G. Azadi, TEDETA@BNPs as a basic and metal free nanocatalyst for Knoevenagel condensation and Hantzsch reaction, J. Iran. Chem. Soc., 2018, 15, 2281 CrossRef CAS
. - M. Mirzaee, B. Bahramian, P. Gholampour, S. Teymouri and T. Khorsand, Preparation and characterization of Fe3O4@Boehmite core–shell nanoparticles to support molybdenum or vanadium complexes for catalytic epoxidation of alkenes, Appl. Organomet. Chem., 2019, 33, e4792 CrossRef
. - S. Roy, K. Pal, S. Bardhan, S. Maity, D. K. Chanda, S. Ghosh, P. Karmakar and S. Das, Gd(III)-Doped Boehmite Nanoparticle: An Emergent Material for the Fluorescent Sensing of Cr(VI) in Wastewater and Live Cells, Inorg. Chem., 2019, 58, 8369 CrossRef CAS PubMed
. - A. P. Panda, U. Jha and S. K. Swain, Synthesis of nanostructured copper oxide loaded boehmite (CuO_Boehmite) for adsorptive removal of As(III/V) from aqueous solution, J. Water Process. Eng., 2020, 37, 101506 CrossRef
. - V. Claude, J. G. Mahy, T. Lohay, J. Geens and S. D. Lambert, Coating Process of Honeycomb Cordierite Support with Ni/Boehmite Gels, Processes, 2022, 10, 875 CrossRef CAS
. - X. Li, Influence of melamine cyanurate and boehmite on flame retardancy of PA6, Iran. Polym. J., 2022, 31, 975 CrossRef CAS
. - C. B. Nettar, R. N. Bhowmik and A. K. Sinha, A comparative study of the lattice structure, optical band gap, electrical conductivity and polarization at different stages of the heat treatment of chemical routed Al(OH)3, Ceram. Int., 2022, 48, 10677 CrossRef CAS
. - H. Wang, S. T. B. Lundin, K. Takanabe and S. T. Oyama, Synthesis of size-controlled boehmite sols: application in high-performance hydrogen-selective ceramic membranes, Mater. Chem. A, 2022, 10, 12869 RSC
. - Z. Liang, X. Wang, G. Yu, M. Li, S. Shi, H. Bao, C. Chen, D. Fu, W. Ma, C. Xue and B. Sun, Mechanistic understanding of the aspect ratio-dependent adjuvanticity of engineered aluminum oxyhydroxide nanorods in prophylactic vaccines, Nano Today, 2022, 43, 101445 CrossRef CAS PubMed
. - A. Mohammadinezhad and B. Akhlaghinia, Fe3O4@Boehmite-NH2-CoII NPs: an inexpensive and highly efficient heterogeneous magnetic nanocatalyst for the Suzuki–Miyaura and Heck–Mizoroki cross-coupling reactions, Green Chem., 2017, 19, 5625 RSC
. - P. Moradi and M. Hajjami, Magnetization of biochar nanoparticles as a novel support for fabrication of organo nickel as a selective, reusable and magnetic nanocatalyst in organic reactions, New J. Chem., 2021, 45, 2981 RSC
. - M. A. K. Zarchi and F. Nazem, Using a polymer-supported azide ion in [2 + 3] cycloaddition reaction of azide ion with nitriles, J. Appl. Polym. Sci., 2012, 123, 1977 CrossRef CAS
. - B. Tahmasbi and A. Ghorbani-Choghamarani, First report of the direct supporting of palladium–arginine complex on boehmite nanoparticles and application in the synthesis of 5-substituted tetrazoles, Appl. Organomet. Chem., 2017, 31, e3644 CrossRef
. - V. Rama, K. Kanagaraj and K. Pitchumani, Syntheses of 5-Substituted
1H-Tetrazoles Catalyzed by Reusable CoY Zeolite, J. Org. Chem., 2011, 76, 9090 CrossRef CAS PubMed
. - S. S. E. Ghodsinia and B. Akhlaghinia, A rapid metal free synthesis of 5-substituted-1H-tetrazoles using cuttlebone as a natural high effective and low cost heterogeneous catalyst, RSC Adv., 2015, 5, 49849 RSC
. - Z. P. Demko and K. B. Sharpless, Preparation of 5-Substituted 1H-Tetrazoles from Nitriles in Water, J. Org. Chem., 2001, 66, 7945 CrossRef CAS PubMed
. - M. Nikoorazm, B. Tahmasbi, S. Gholami and P. Moradi, Copper and nickel immobilized on cytosine@MCM-41: as highly efficient, reusable and organic–inorganic hybrid nanocatalysts for the homoselective synthesis of tetrazoles and pyranopyrazoles, Appl. Organomet. Chem., 2020, 34, e5919 CAS
. - P. Moradi, M. Hajjami and B. Tahmasbi, Fabricated copper catalyst on biochar nanoparticles for the synthesis of tetrazoles as antimicrobial agents, Polyhedron, 2020, 175, 114169 CrossRef
. - B. Tahmasbi, M. Nikoorazm, P. Moradi and Y. Abbasi Tyula, A Schiff base complex of lanthanum on modified MCM-41 as a reusable nanocatalyst in the homoselective synthesis of 5-substituted 1H-tetrazoles, RSC Adv., 2022, 12, 34303–34317 RSC
. - A. Rezaei, A. Ghorbani-Choghamarani and B. Tahmasbi, Synthesis and Characterization of Nickel Metal-Organic Framework Including 4,6-diamino-2-mercaptopyrimidine and its Catalytic Application in Organic Reactions, Catal. Lett., 2022 DOI:10.1007/s10562-022-04135-8
. - M. Nikoorazm, Z. Rezaei and B. Tahmasbi, Two Schiff-base complexes of copper and zirconium oxide supported on mesoporous MCM-41 as an organic–inorganic hybrid catalysts in the chemo and homoselective oxidation of sulfides and synthesis of tetrazoles, J. Porous Mater., 2020, 27, 671 CrossRef CAS
. - B. Tahmasbi, A. Ghorbani-Choghamarani and P. Moradi, Palladium fabricated on boehmite as an organic–inorganic hybrid nanocatalyst for C–C cross coupling and homoselective cycloaddition reactions, New J. Chem., 2020, 44, 3717 RSC
. - T. Kikhavani, P. Moradi, M. Mashari-Karir and J. Naji, A new copper Schiff-base complex of 3,4-diaminobenzophenone stabilized on magnetic MCM-41 as a homoselective and reusable catalyst in the synthesis of tetrazoles and pyranopyrazoles, Appl. Organomet. Chem., 2022, 36, e6895 CrossRef CAS
. - P. Moradi and M. Hajjami, Magnetization of graphene oxide nanosheets using nickel magnetic nanoparticles as a novel support for the fabrication of copper as a practical, selective, and reusable nanocatalyst in C–C and C–O coupling reactions, RSC Adv., 2021, 11, 25867 RSC
. - M. Nikoorazm, P. Moradi, N. Noori and G. Azadi, L-Arginine complex of copper on modified core–shell magnetic nanoparticles as reusable and organic–inorganic hybrid nanocatalyst for the chemoselective oxidation of organosulfur compounds, J. Iran. Chem. Soc., 2021, 18, 467 CrossRef CAS
. - P. Moradi and M. Hajjami, Stabilization of ruthenium on biochar-nickel magnetic nanoparticles as a heterogeneous, practical, selective, and reusable nanocatalyst for the Suzuki C–C coupling reaction in water, RSC Adv., 2022, 12, 13523–13534 RSC
. - E. Aali, M. Gholizadeh and N. Noroozi-Shad, 1-Disulfo-[2,2-bipyridine]-1,1-diium chloride ionic liquid as an efficient catalyst for the green synthesis of 5-substituted 1H-tetrazoles, J. Mol. Struct., 2022, 1247, 131289 CrossRef CAS
. - M. Aqeel Ashraf, Z. Liu, C. Li and D. Zhang, Fe3O4@L-lysine-Pd(0) organic–inorganic hybrid: As a novel heterogeneous magnetic nanocatalyst for chemo and homoselective [2 + 3] cycloaddition synthesis of 5-substituted 1H-tetrazoles, Appl. Organomet. Chem., 2020, 35, e6133 Search PubMed
. - K. Uchida and H. Togo, Transformation of aromatic bromides into aromatic nitriles with n-BuLi, pivalonitrile, and iodine under metal cyanide-free conditions, Tetrahedron, 2019, 75, 130550 CrossRef
. - B. Sreedhar, A. Suresh Kumar and D. Yada, CuFe2O4 nanoparticles: a magnetically recoverable and reusable catalyst for the synthesis of 5-substituted 1H-tetrazoles, Tetrahedron Lett., 2011, 52, 3565–3569 CrossRef CAS
. - P. Moradi, B. Zarei, Y. Abbasi Tyulaa and M. Nikoorazm, Novel neodymium complex on MCM-41 magnetic nanocomposite as a practical, selective and returnable nanocatalyst in the synthesis of tetrazoles with antifungal properties in agricultural, Appl. Organometal. Chem., 2023, 37, e7020 Search PubMed
. - G. Aridoss and K. K. Laali, Highly Efficient Synthesis of 5-Substituted 1H-Tetrazoles Catalyzed by Cu–Zn Alloy Nanopowder, Conversion into 1,5- and 2,5-Disubstituted Tetrazoles, and Synthesis and NMR Studies of New Tetrazolium Ionic Liquids, Eur. J. Org. Chem., 2011, 6343 CrossRef CAS
. - S. Kumar Prajapti, A. Nagarsenkar and B. Nagendra Babu, An efficient synthesis of 5-substituted 1H-tetrazoles via B(C6F5)3 catalyzed [3 + 2] cycloaddition of nitriles and sodium azide, Tetrahedron Lett., 2014, 55, 3507 CrossRef
. - F. Dehghani, A. R. Sardarian and M. Esmaeilpour, Salen complex of Cu(II) supported on superparamagnetic Fe3O4@SiO2 nanoparticles: An efficient and recyclable catalyst for synthesis of 1- and 5-substituted 1H-tetrazoles, J. Organomet. Chem., 2013, 743, 87 CrossRef CAS
. - G. Qi, W. Liu and Z. Bei, Fe3O4/ZnS Hollow Nanospheres: A Highly Efficient Magnetic Heterogeneous Catalyst for Synthesis of 5-Substituted 1H -Tetrazoles from Nitriles and Sodium Azide, Chin. J. Chem., 2011, 29, 131 CrossRef CAS
. - A. Jabbari, B. Tahmasbi, M. Nikoorazm and A. Ghorbani-Choghamarani, A new Pd-Schiff-base complex on boehmite nanoparticles: Its application in Suzuki reaction and synthesis of tetrazoles, Appl. Organometal. Chem., 2018, 32, e4295 CrossRef
. - L. Lang, H. Zhou, M. Xue, X. Wang and Z. Xu, Mesoporous ZnS hollow spheres-catalyzed synthesis of 5-substituted 1H-tetrazoles, Mater. Lett., 2013, 106, 443 CrossRef CAS
. - P. Mani, A. K. Singh and S. K. Awasthi, AgNO3 catalyzed synthesis of 5-substituted-1H-tetrazole via [3 + 2] cycloaddition of nitriles and sodium azide, Tetrahedron Lett., 2014, 55, 1879 CrossRef CAS
. - B. Sreedhar, A. Suresh Kumar and D. Yada, CuFe2O4 nanoparticles: a magnetically recoverable and reusable catalyst for the synthesis of 5-substituted 1H-tetrazoles, Tetrahedron Lett., 2011, 52, 3565 CrossRef CAS
. - S. M. Agawane and J. M. Nagarkar, Synthesis of 5-substituted 1H-tetrazoles using a nano ZnO/Co3O4 catalyst, Catal. Sci. Technol., 2012, 2, 1324 RSC
. - P. Moradi and A. Ghorbani-Choghamarani, Efficient synthesis of 5-substituted tetrazoles catalysed by palladium–S-methylisothiourea complex supported on boehmite nanoparticles, Appl. Organometal. Chem., 2017, 31, e3602 CrossRef
. - M. Nikoorazm, P. Moradi and N. Noori, L-cysteine complex of palladium onto mesoporous channels of MCM-41 as reusable, homoselective and organic–inorganic hybrid nanocatalyst for the synthesis of tetrazoles, J. Porous Mater., 2020, 27, 1159 CrossRef CAS
. - M. Nikoorazm, A. Ghorbani-Choghamaranai, M. Khanmoradi and P. Moradi, Synthesis and characterization of Cu(II)-Adenine-MCM-41 as stable and efficient mesoporous catalyst for the synthesis of 5-substituted 1H-tetrazoles and 1H-indazolo [1,2-b]phthalazine-triones, J. Porous Mater., 2018, 25, 1831 CrossRef CAS
. - P. Moradi, Investigation of Fe3O4@boehmite NPs as efficient and magnetically recoverable nanocatalyst in the homoselective synthesis of tetrazoles, RSC Adv., 2022, 12, 33459–33468 RSC
.
|
This journal is © The Royal Society of Chemistry 2023 |
Click here to see how this site uses Cookies. View our privacy policy here.