DOI:
10.1039/D2RA07582B
(Paper)
RSC Adv., 2023,
13, 7848-7856
In-vial solid-phase extraction of polycyclic aromatic hydrocarbons in drug formulations stored in packaging containing rubber†
Received
29th November 2022
, Accepted 2nd March 2023
First published on 9th March 2023
Abstract
Polycyclic aromatic hydrocarbons (PAHs) are a class of ubiquitous and persistent organic compounds that are significantly teratogenic, carcinogenic and mutagenic. Rubber stoppers commonly used in sterile formulation packaging materials often contain carbon black as the additive to enhance mechanical strength. However, PAHs may be formed during the production of carbon black, which could cause the drug formulations to be contaminated when contacting with the rubber stopper, and then enter the patient's body. The determination of PAHs in drug formulations is challenging, due to their trace amounts and matrix interference. Therefore, sample pretreatment is necessary and important. In this work, a novel technique, named in-vial solid-phase extraction (IVSPE), was developed for the selective extraction and enrichment of 16 PAHs in pharmaceuticals. The coated sample vial was directly used as the container for the whole process of sample pretreatment. As the solid-phase adsorbent, the coating was prepared by successively modifying the inner surface of a sample vial with polydopamine film and octadecylamine. PAHs could be selectively extracted through π–π stacking interaction and hydrophobic interaction, and then desorbed and enriched by a small amount of organic solvent. After systematic optimization of the coating preparation and the extraction process, the limits of detection and quantification of 16 PAHs were in the range of 0.002–0.60 ng mL−1 and 0.007–2.00 ng mL−1, respectively. Good linearities and precision of six repeated injections were obtained. The recoveries at three spiked concentration levels in normal saline were in the range of 62.72–106.90% with the relative standard deviation between 0.83% and 6.78%. Finally, PAHs in normal saline and powders for injection were extracted by established IVSPE, followed by separation and detection with high-performance liquid chromatography with a fluorescence detector and diode array detector (HPLC-FLD/DAD). It is worth noting that the preparation conditions of the adsorbent in the IVSPE method are mild, simple and green. Moreover, IVSPE has the advantages of having few work steps and avoiding the risk of contamination, because no special instrumentation or sample transfer is required. IVSPE could also be used for the pretreatment of multiple samples at the same time, which is beneficial to practical applications.
1 Introduction
Polycyclic aromatic hydrocarbons (PAHs) are a class of compounds formed by two or more fused aromatic rings, without a heteroatom or substituent.1,2 Besides natural biosynthesis, the major source of PAHs is anthropogenic activities, such as the incomplete combustion of coal, petroleum, wood and organic polymers.3–5 It has been paid a lot of attention that many PAHs possess significant teratogenicity, carcinogenicity and mutagenicity6–9. Heavy occupational exposure to the mixtures of PAHs entails a substantial risk of lung, skin, or bladder cancer. The metabolites of certain PAHs could interact with DNA and are genotoxic, causing malignancies and heritable genetic damage in humans. In addition, PAHs can be biomagnified in the food chain and bioaccumulated in vivo because of their lipophilicity and low metabolic rate,10–13 which can affect human health. Therefore, the US Environmental Protection Agency (USEPA) has recommended a list of 16 PAH compounds as the priority pollutants.
Humans are widely exposed to PAHs everyday through multiple ways, including respiratory system,14,15 skin contact,16 digestive system,17–19 medicine1,20,21 and so on. Therefore, the monitoring of PAHs in the environment, food and pharmaceuticals is an important measure to reduce the related diseases.22–24 As the basic therapeutic products in clinic, drug formulations may introduce PAHs to patients if rubbers are used as the packaging materials.21,25 It is because carbon black (CB) is the most commonly used as the reinforcing agent to reinforce the mechanical strength and increase the pigmentation capacity in the production of rubber.26 And as a material produced by the incomplete combustion of biomass and fossil fuel,27 CB is a known source of PAHs and has been considered possibly carcinogenic to humans by the International Agency for Research on Cancer.28 This means PAHs could enter the body and impart adverse effect on human health through the rubber closures. Therefore, it is very important to detect PAHs in pharmaceutical formulations.
Gas chromatography (GC) and high-performance liquid chromatography (HPLC) with fluorescence detector (FLD), diode array detector (DAD) or mass spectrometer (MS) are the most frequently employed techniques for the determination of PAHs in different types of matrixes.29–31 In order to meet the needs of analytical instruments and sensitivity, sample pretreatment has already been used extensively for the extraction and preconcentration of the analytes in real samples, such as soxhlet extraction,32 potassium hydroxide saponification,33 ultrasonic assisted treatment,34 microwave assisted extraction,35 pressurized liquid extraction,36 liquid–liquid extraction,37 liquid phase microextraction,1 solid phase microextraction,38–40 solid phase dispersive microextraction,41 and magnetic solid-phase extraction (MSPE). There are some drawbacks with these methods, including the complex steps, time-consuming, large consumption of organic solvents, low extraction efficiency, excessive dependence on extraction conditions, and interference of co-eluting substances. Therefore, it is necessary to develop a simple, green and efficient pretreatment technology for the determination of PAHs.
Dopamine (DA), containing both catechol and amino groups, is a simple and widely used molecule for the inspired synthesis due to its self-polymerization. Under weak alkaline condition, DA molecules can be easily deposited on a variety of inorganic or organic substrates to form stable and hydrophilic polydopamine (PDA) film just like glue.42,43 The self-polymerized procedure is environmentally friendly and mild. The formed PDA film which possesses an assortment of reactive functional groups could provide the anchors for the secondary conjugation of interesting functionalities without surface pretreatment. For example, the catechols are easily converted into highly reactive quinones, which can further react with thiols and amines via Michael type addition or Schiff base formation.44–46 The PDA film is composed of roughly planar oligomers and can be used as the adsorbent for extracting aromatic compounds through π-π stacking interaction and van der Waals forces. Wang et al. prepared PDA-coated Fe3O4 nanoparticles (Fe3O4@PDA NPs) for the enrichment of 6 PAHs in the environmental water samples.47 Ma et al. used Fe3O4@PDA NPs as an adsorbent and matrix for the detection of benzo(a)pyrene (BaP) by matrix-assisted laser desorption ionization time-of-flight mass spectrometry (MALDI-TOF-MS) in the tap water and lake water samples.48 In these methods, Fe3O4 NPs with sufficient magnetism need to be prepared first, which are susceptible to aggregation. Moreover, external magnetic field is needed for the collection of the analytes. PDA-modified 3D nickel foam (NF@PDA) was also used as the adsorbent to extract PAHs from water samples.49 It is necessary to take NF@PDA in and out of the sampling container using tweezers or the like, which is considered to increase the contamination risk of the analytes from the external environment.
In this study, we proposed in-vial SPE (IVSPE) technology for the sample extraction, and the vial that was often used for loading sample in HPLC was chosen as the medium to complete the whole pretreatment process without sample transfer after its internal surface modification. The schematic of the principle for the preparation of coating on the inner surface of vial was shown in Fig. 1. PDA film was first deposited on the inner wall of vial through oxidative self-polymerization. Then octadecylamine (ODA) was further grafted on the surface of formed PDA via Schiff base formation. Since there are abundant phenyls on PDA film and long alkyl chain of ODA, the composite layer of IVSPE is expected to offer strong adsorption affinity to PAHs, due to their π–π stacking interaction, as well as hydrophobic interaction. After the optimization of the coating preparation and sample extraction, 16 priority-controlled PAHs could be simultaneously extracted efficiently. Finally, IVSPE coupled with HPLC-FLD/DAD was successfully used for the detection of PAHs in drug formulations, including normal saline and powders for injection.
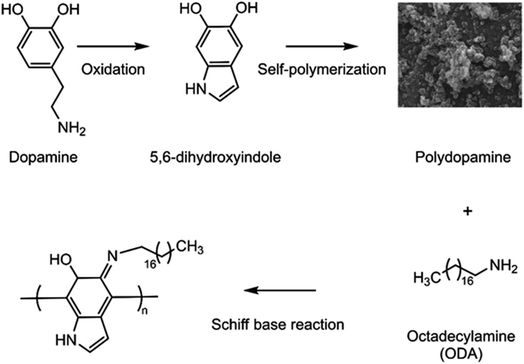 |
| Fig. 1 Schematic of the principle for the preparation of coating on the inner surface of vial. | |
2 Experimental
2.1 Reagents and materials
Dopamine Hydrochloride (DA·HCl, 98%) and octadecylamine (GC, >97%) were purchased from Shanghai Aladdin Biochemical Technology Co., Ltd. (China). Tris(hydroxymethyl)-amino methane (Tris, 99%) was obtained from Nanjing SunShine Biotechnology Co., Ltd. (China). Acetonitrile (ACN) was of HPLC grade and purchased from TEDIA (USA). Other chemicals were of analytical grade. The sample vials were obtained from ANW Technologies (China). The normal saline for injection (Shijiazhuang Four Drugs Co., Ltd., 0.9%), benzylpenicillin sodium for injection (Shandong Lukang Pharmaceutical Co., Ltd., 160 million units per 96 g) and omeprazole sodium for injection (Jiangsu Wuzhong Pharmaceutical Group Co., Ltd., 40 mg) were commercial products.
Standard mixtures of the 16 PAHs with 200 μg mL−1 of each compound dissolved in acetonitrile (for HPLC analysis) was obtained from Manhage Bio-Technology Co., Ltd. (China). The 16 PAHs were naphthalene (NAP), acenaphthylene (ANY), acenaphthene (ANA), fluorene (FLU), phenanthrene (PHE), anthracene (ANT), fluoranthene (FLT), pyrene (PYR), benz[a]anthracene (BaA), chrysene (CHR), benzo[b]fluoranthene (BbF), benzo[k]fluoranthene (BkF), benzo[a]pyrene (BaP), indeno[1,2,3 cd]pyrene (IPY), dibenz[a,h]anthracene (DBA) and benzo[ghi]perylene (BPE). The PAHs stock solution was prepared with acetonitrile at the concentration of each at 2 μg mL−1, and kept at 4 °C in darkness. PAHs working solutions were prepared by the dilution of the stock solution.
2.2 HPLC analytical conditions
The HPLC analysis was performed on a SHIMADZU LC-20AT series HPLC system (Kyoto, Japan) with RF-20A fluorescent spectrophotometric detector and SPD-M20A photodiode array ultraviolet-visible light detector. A Zorbax Eclipse PAH (4.6 × 150 mm i.d., 5 μm, Agilent Technologies) column was used for PAHs separation. The column temperature was 30 °C. The mobile phase consisting of ACN and water was in gradient mode (Table 1). And the initial flow rate was 2 mL min−1. The injection volume was 20 μL. ANY was detected by DAD at 228 nm, and the other PAHs were detected by FLD. The excitation and emission wavelengths of FLD were summarized in Table 1.
Table 1 HPLC chromatographic conditions
Time (min) |
Mobile phase water/acetonitrile |
FLD wavelength (nm) |
Detected compounds |
Excitation |
Emission |
0.01 |
60/40 |
275 |
350 |
NAP, ANA, FLU, PHE |
9.50 |
60/40 |
260 |
420 |
ANT |
10.50 |
60/40 |
270 |
440 |
FLT, PYR |
13.00 |
60/40 |
260 |
420 |
BaA, CHR |
15.25 |
60/40 |
290 |
430 |
BbF, BkF, BaP, IPY, DBA |
20.00 |
0/100 |
20.20 |
0/100 |
250 |
500 |
BPE |
22.50 |
0/100 |
25.50 |
60/40 |
28.00 |
60/40 |
2.3 Preparation of SPE vials
The procedure of preparation was shown in Fig. 2A. PDA film was formed through the self-polymerization of DA dissolved in 10 mM Tris buffer at pH 8.5. Firstly, 1 mL of DA solution was added into the vial. After mechanically shaken at 37 °C for 4 h, the solution was discarded. The vial was washed gently with acetonitrile and ultrapure water for 3 times, respectively. Then the above procedure was repeated to increase the thickness of PDA layer. After the formation of a stable PDA film on the inner wall of vial, 0.25 mg mL−1 ODA solution in alcohol was added and mechanically shaken 2 h at 40 °C. Then, the remained solution was poured out, and the vial was washed for 3 times.
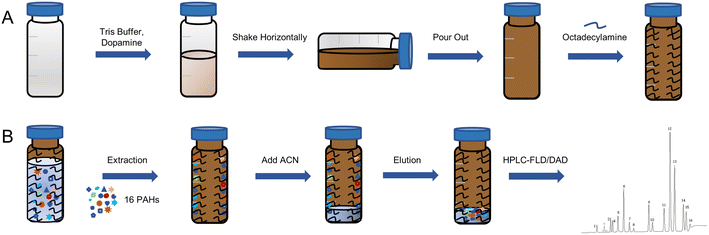 |
| Fig. 2 Schematic illustration of the preparation and extraction procedure of IVSPE. | |
2.4 Characterization
A Quanta 250 FEG electron microscope (Thermo Scientific Inc., USA) was employed to record scanning electronic microscope (SEM) images of coating. The surface chemical component of coating was investigated by an attenuated total reflectance-Fourier transform infrared (ATR-FTIR) spectrometer (Nicolet iS50, Thermo Scientific Inc., USA).
2.5 Extraction procedure of IVSPE
As shown in Fig. 2B, 1.5 mL of the sample solution was added into the prepared vial and shaken at room temperature. When the adsorption process was completed, the solution was poured out. And the vial was washed with 1 mL of ultrapure water for 3 times. Then the target compounds were desorbed from the adsorbent with 300 μL of acetonitrile by shaken at room temperature.
2.6 Preparation of real samples
2.6.1 Preparation of the normal saline. The normal saline was accurately transferred 1.50 mL into the prepared IVSPE vial and shaken for 60 min at room temperature. Then the saline was poured out, and the vial was cleaned three times with ultrapure water. After that, 300 μL of acetonitrile was added and shaken at 200 rpm for 3 h.
2.6.2 Preparation of the powders for injection. Because both powders for injection were water-soluble, the sample solution was prepared with ultrapure water at the concentration of 200 mg mL−1 for penicillin sodium and 20 mg mL−1 for omeprazole sodium, respectively. Then the sample solution was extracted and eluted following the previously described procedure of the normal saline.
3 Results and discussion
It has been proven that DA dissolved in Tris buffer at pH 8.5 could self-polymerize to form PDA on the surfaces of almost various substrates under non-toxic conditions. Moreover, PDA coating is easily to be further modification. Owing to the above advantages, we developed the sample vial coated with PDA and ODA for simple and selective extraction of PAHs.
In order to achieve high efficiency of extraction, the parameters in IVSPE process were designed and optimized by control variable method, including the preparation conditions of coating and the extraction conditions of PAHs.
3.1 Optimization of the preparation of PDA coating
3.1.1 Effect of the concentration of DA. The thickness of PDA film is closely related to the amount of DA.50 Thus, it is necessary to investigate the effect of concentration of DA on the extraction efficiency. As shown in Fig. 3, the recoveries of most PAHs became larger with the increase in the concentration of DA to 5 mg mL−1. When the concentration was further increased to 6 mg mL−1, the recoveries were remained at the same level or even decreased. Therefore, 5 mg mL−1 DA solution was chosen for the preparation of PDA coating in sample vial.
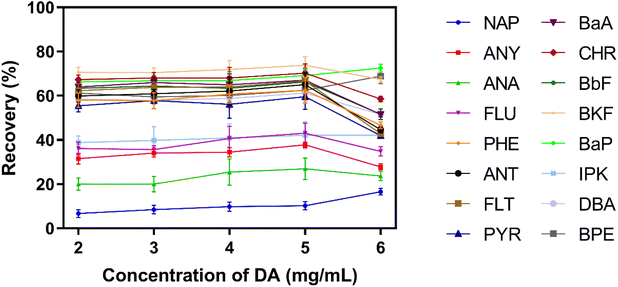 |
| Fig. 3 Recoveries of 16 PAHs with different concentration of DA solution (n = 6). | |
3.1.2 Effect of the number of PDA layers. As the core of IVSPE, the amount of solid adsorbent could be manually controlled by changing the deposition times of PDA in sample vial. To obtain a stable and uniform coating, the DA solution was exchanged every 4 h. It could be observed that the color of vial became darker and darker as the number of PDA layer increased from one to nine (Fig. S1†). The morphologies of PDA-modified vials were investigated by SEM characterization. As shown in Fig. S2A,† the inner surface of bare vial was smooth and clean. After modified layer by layer (Fig. S2B–G†), the inner surface was covered with more and more visible aggregates, which indicated the successful immobilization of each layer of PDA. Meanwhile, it also suggested the positive correlation between the increase in the deposited amounts and the number of coatings. According to the cross-section SEM images of the vials (Fig. S2H and I†), the PDA coating was clearly discerned on the inner wall of the vial. The extraction effect of different layers was illustrated in Fig. 4. The recoveries of most PAHs were in the range of 71.89–89.00% with eight repetitive coatings, except for NAP. It was probably because the more layers, the greater the adsorbent amount and the larger the extraction capacity.
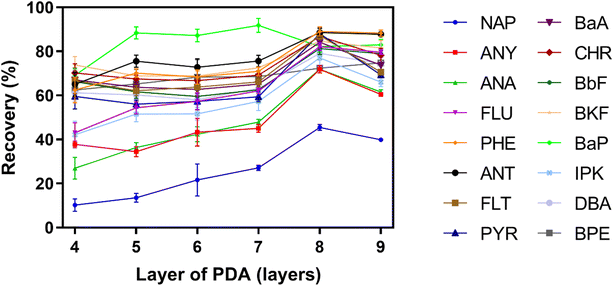 |
| Fig. 4 Recoveries of 16 PAHs with different numbers of PDA layer (n = 6). | |
3.2 Modification of PDA coating
According to the above results, the recovery of NAP was below 50% even after optimizing the preparation conditions of PDA coating. The reason is probably due to there are only two aromatic rings in the structure of NAP, and the π–π stacking interaction between PDA coating and NAP is not sufficiently strong. In order to improve the extraction of NAP, ODA containing long alkyl chain was used to further modify the surface of PDA coating via Schiff base reaction. After the graft of ODA, the hydrophobicity of PDA coating was increased. It was benefit for the adsorption of hydrophobic PAHs, especially for NAP.
In order to prove the post-modification of ODA, ATR-FTIR was used to characterize the surface chemical component of the coating. As shown in Fig. S3,† two new absorption peaks located at around 2910 cm−1 and 2840 cm−1 appeared after ODA was introduced, which were related to the stretching vibration of C–H in ODA molecules. The concentration and the reaction time of ODA could affect the surface properties of coating, and further impact the extraction efficiency of IVSPE on different PAHs. According to Fig. 5A, the recoveries of all PAHs were increased gradually when the concentration of ODA was varied from 0.05 to 0.25 mg mL−1. In particularly, the recovery of NAP could reach 65.49%, which is significantly higher than that of using PDA coating alone. With the further increase of concentration, no improvement of recovery was observed, and the recoveries of high-ring PAHs even decreased obviously. As mentioned above, excess modification with ODA might inhibit the adhesion between PDA coating and high-ring PAHs through π–π stacking interaction. Clearly, there is a compromise of the recovery between low-ring and high-ring PAHs.51–53 Considering the recoveries of all 16 PAHs within an acceptable range, 0.25 mg mL−1 ODA was selected for further optimization. Next, we investigated the effect of reaction time on the recoveries of PAHs as the time was varied from 0.5 h to 4 h. As shown in Fig. 5B, 2 h was sufficient to achieve satisfactory extraction efficiency for all PAHs.
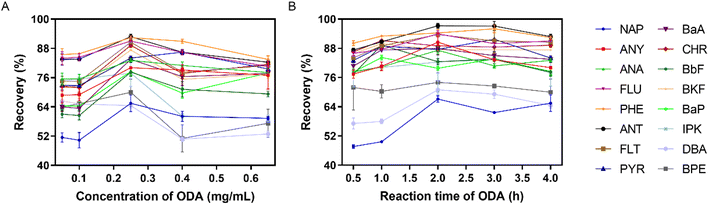 |
| Fig. 5 Recovery of 16 PAHs with the modification of ODA at different concentrations (A) and for different reaction time (B) (n = 6). | |
3.3 Optimization of extraction conditions
The extraction of PAHs with good reproducibility by the established IVSPE mode needs enough contact time between the targets and adsorbent for reaching the equilibrium. Therefore, different extraction time ranging from 30 min to 90 min were studied. The result in Fig. 6A showed that 60 min was sufficient for all the PAHs to achieve satisfactory extraction efficiency. The elution time can also affect the extraction efficiency significantly. Fig. 6B illustrated the effect of elution time on the recovery of 16 PAHs in the range of 2–5 h. The recovery was not greatly increased after 3 h.
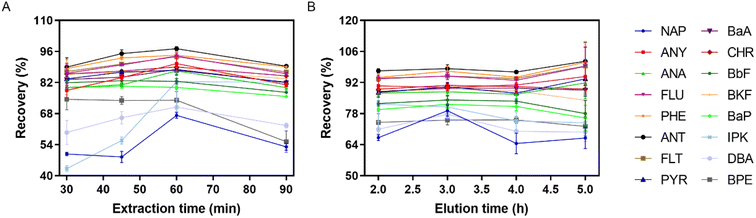 |
| Fig. 6 Recovery of 16 PAHs at different extraction time (A) and at different elution time (B) (n = 6). | |
3.4 Method validation
The established method of IVSPE coupled with HPLC-FLD/DAD was validated for the determination of PAHs. The limit of detection (LOD) and the limit of quantification (LOQ) were calculated based on the signal-to-noise ratio (S/N) of 3
:
1 and 10
:
1, respectively. The LODs of 16 PAHs were in the range of 0.002–0.60 ng mL−1, and LOQs were determined to be 0.007–2.00 ng mL−1. In addition, good linearities were exhibited for PAHs, and all of the correlation coefficients (r) were more than 0.999. The relative standard deviations of six replicate injections (RSDs, n = 6) showed good precision with the range of 0.06–5.40%. Therefore, the established IVSPE-HPLC method is effective and sensitive for the detection of 16 PAHs. These validation results mentioned above were summarized in Table 2. Moreover, we investigated the stability of the prepared vials by testing their extraction ability to PAHs after the vials were stored for one month. Six vials were chosen at random, and the recoveries of 16 kinds of PAHs were in the range of 60–95% with the reproducibility lower than 10%. These results suggested good stability of the SPE vials.
Table 2 Validation data of the proposed method for the determination of 16 PAHs
PAHs |
Linearity range (ng mL−1) |
LOD (ng mL−1) |
LOQ (ng mL−1) |
RSD (%) (n = 6) |
NAP |
0.10–50.0 |
0.020 |
0.08 |
0.22 |
ANY |
2.00–50.0 |
0.60 |
2.00 |
1.51 |
ANA |
0.02–50.0 |
0.007 |
0.02 |
0.20 |
FLU |
0.02–50.0 |
0.007 |
0.02 |
0.31 |
PHE |
0.10–40.0 |
0.020 |
0.08 |
0.31 |
ANT |
0.01–40.0 |
0.002 |
0.007 |
0.24 |
FLT |
0.02–50.0 |
0.007 |
0.02 |
0.27 |
PYR |
0.10–50.0 |
0.020 |
0.08 |
0.54 |
BaA |
0.02–50.0 |
0.007 |
0.02 |
1.15 |
CHR |
0.01–50.0 |
0.002 |
0.007 |
0.79 |
BbF |
0.01–50.0 |
0.002 |
0.007 |
0.06 |
BkF |
0.01–50.0 |
0.002 |
0.007 |
0.06 |
BaP |
0.01–50.0 |
0.002 |
0.007 |
0.09 |
IPY |
0.01–50.0 |
0.002 |
0.007 |
0.19 |
DBA |
0.02–50.0 |
0.007 |
0.02 |
0.18 |
BPE |
2.00–50.0 |
0.60 |
2.00 |
5.40 |
3.5 Application to real samples
To further demonstrate the feasibility of the proposed analytical methodology, it was used for the determination of PAHs in real samples, including normal saline, omeprazole sodium and benzylpenicillin sodium powders for injection. Fig. 7 showed the chromatograms of PAHs in three real samples and 10 ng mL−1 PAHs standard solution. No PAHs were detected in the selected drug formulations. In addition, the recoveries of PAHs in normal saline were measured at three spiked levels in triplicate. The recoveries and RSDs were used to evaluate the accuracy and precision of this method for a real sample. The results in Table 3 showed the average recoveries of PAHs were in the range of 62.70–106.90% with RSDs below 6.78%. These results implied that the developed method could be applied to determine PAHs in drug matrix.
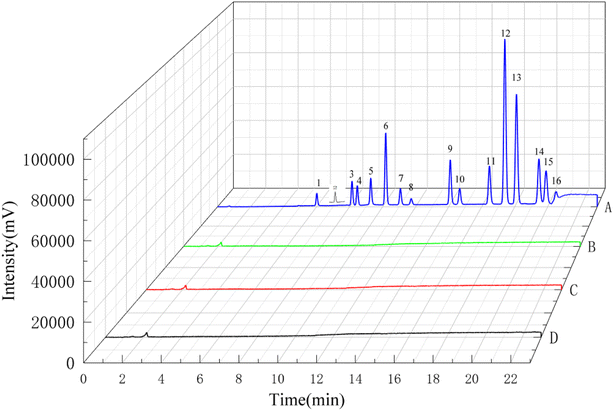 |
| Fig. 7 HPLC chromatograms of (A) 10 ng mL−1 PAHs standard solution (B) normal saline (C) 20 mg mL−1 omeprazole sodium, and (D) 200 mg mL−1 benzylpenicillin sodium. Peak identities: 1, NAP; 2, ANY; 3, ANA; 4, FLU; 5, PHE; 6, ANT; 7, FLT; 8, PYR; 9, BaA; 10, CHR; 11, BbF; 12, BkF; 13, BaP; 14, IPY; 15, DBA; 16, BPE. | |
Table 3 Recoveries of PAHs in normal saline using the proposed method (n = 3)
Analytes |
Spiked sample |
2 ng mL−1 |
6 ng mL−1 |
10 ng mL−1 |
Recovery% |
RSD% |
Recovery% |
RSD% |
Recovery% |
RSD% |
NAP |
76.01 ± 2.83 |
3.72 |
71.27 ± 2.17 |
3.05 |
67.20 ± 2.60 |
3.87 |
ANY |
100.86 ± 3.23 |
3.21 |
97.27 ± 2.87 |
2.95 |
95.97 ± 2.40 |
2.50 |
ANA |
100.46 ± 2.43 |
2.42 |
96.39 ± 1.94 |
2.01 |
94.82 ± 1.57 |
1.66 |
FLU |
104.61 ± 1.56 |
1.49 |
101.26 ± 1.10 |
1.08 |
98.88 ± 1.22 |
1.23 |
PHE |
106.90 ± 1.44 |
1.35 |
98.52 ± 0.57 |
0.58 |
96.30 ± 1.76 |
1.83 |
ANT |
105.88 ± 1.70 |
1.61 |
100.87 ± 1.52 |
1.51 |
97.85 ± 1.56 |
1.59 |
FLT |
100.42 ± 1.59 |
1.58 |
94.37 ± 1.48 |
1.57 |
89.05 ± 1.60 |
1.80 |
PYR |
97.58 ± 1.20 |
1.23 |
95.13 ± 1.51 |
1.59 |
86.24 ± 1.65 |
1.92 |
BaA |
88.04 ± 1.41 |
1.60 |
94.69 ± 2.73 |
2.88 |
91.82 ± 1.72 |
1.87 |
CHR |
91.17 ± 1.92 |
2.10 |
97.69 ± 1.88 |
1.92 |
96.07 ± 1.64 |
1.71 |
BbF |
74.81 ± 2.36 |
3.16 |
87.03 ± 3.73 |
4.29 |
83.83 ± 2.68 |
3.20 |
BkF |
85.96 ± 1.56 |
1.82 |
92.31 ± 2.46 |
2.67 |
92.71 ± 1.89 |
2.04 |
BaP |
76.32 ± 1.61 |
2.11 |
85.18 ± 3.51 |
4.12 |
104.89 ± 4.29 |
4.09 |
IPY |
84.42 ± 1.75 |
2.07 |
86.44 ± 4.95 |
5.73 |
86.36 ± 2.35 |
2.72 |
DBA |
62.72 ± 2.25 |
6.78 |
65.99 ± 0.55 |
0.83 |
74.43 ± 1.37 |
1.85 |
BPE |
73.78 ± 2.96 |
4.02 |
73.22 ± 4.86 |
6.64 |
71.63 ± 3.82 |
5.34 |
There are few studies on the determination of PAHs in drug formulations. Through literature review, the parameters in this work were compared with others, such as LOD, LOQ and recovery. As shown in Table 4, 16 PAHs could be simultaneously determined by our established method with higher sensitivity and acceptable recovery. It is indicated that IVSPE combined with HPLC-FLD/DAD has a promising application in the analysis of PAHs in pharmaceutical preparations.
Table 4 Comparison of different methods for the determination of PAHs in drug formulations
Kinds of PAHs |
Sample pretreatment |
Analytical method |
LOD (ng mL−1) |
LOQ (ng mL−1) |
Recovery (%) |
Ref. |
The unit is ng/inhaler. |
16 |
Cold filtration |
GC-MS |
0.7–6.0a |
4–30a |
— |
29 |
12 |
SPE |
HPLC-UVD/FLD |
10–110 |
40–330 |
85.6–122.8 |
30 |
16 |
SPE |
HPLC-DAD |
1–60 |
3–167 |
75–120 |
31 |
16 |
IVSPE |
HPLC-FLD/DAD |
0.002–0.6 |
0.007–2.00 |
62.72–106.90 |
This work |
4 Conclusion
In this study, a simple, facile and environmentally-friendly pretreatment method, named IVSPE, was established based on the self-polymerization of DA and the post-modification with ODA. The sample vial was coated with PDA film on the inner surface, and then the ODA was grafted on PDA via Schiff base reaction. After optimizing the parameters of PDA coating, modification of ODA and extraction conditions, the prepared vials exhibited satisfactory extraction efficiency for 16 PAHs. In this IVSPE method, no special instruments are required. The operation steps are simplified, and there is no need to transfer the sample several times. This is very helpful to reduce sample loss and the risk of contamination. Moreover, the extraction and desorption in different vials can be operated at the same time, which will save time for sample pretreatment. Coupled with the detection of HPLC-FLD/DAD, the determination results of 16 PAHs showed excellent analytical performance. The LODs and LOQs were in the range of 0.002–0.60 ng mL−1 and 0.007–2.00 ng mL−1, respectively. And the linear relationship was good for all PAHs within the concentration range between 0.01 and 50 ng mL−1. The spiked recovery of normal saline at three concentration levels was 62.72–106.90% with high precision. Finally, the developed method was successfully applied for the determination of PAHs in drug formulations stored in packaging containing rubber.
Conflicts of interest
The authors declare that there are no conflicts of interest.
Acknowledgements
This work was supported by grants from the National Key Research and Development Program of China (No. 2019YFC1605400), and the Natural Science Foundation of Jiangsu Province (No. BK20200567).
References
- W. Deng, A. Huang, Q. Zheng, L. Yu, X. Li, H. Hu and Y. Xiao, Food Chem., 2021, 352, 129331 CrossRef CAS.
- G. R. Sampaio, G. M. Guizellini, S. A. da Silva, A. P. de Almeida, A. C. C. Pinaffi-Langley, M. M. Rogero, A. C. de Camargo and E. Torres, Int. J. Mol. Sci., 2021, 22, 6010 CrossRef CAS.
- H. I. Abdel-Shafy and M. S. M. Mansour, Egypt. J. Pet., 2016, 25, 107–123 CrossRef.
- A. K. Haritash and C. P. Kaushik, J. Hazard. Mater., 2009, 169, 1–15 CrossRef CAS PubMed.
- M. Lv, X. Luan, C. Liao, D. Wang, D. Liu, G. Zhang, G. Jiang and L. Chen, Nat. Sustain., 2020, 3, 878–884 CrossRef.
- W. Xue and D. Warshawsky, Toxicol. Appl. Pharmacol., 2005, 206, 73–93 CrossRef CAS PubMed.
- S. Amirdivani, N. Khorshidian, M. Ghobadi Dana, R. Mohammadi, A. M. Mortazavian, S. L. Quiterio de Souza, H. Barbosa Rocha and R. Raices, Int. J. Dairy Technol., 2019, 72, 120–131 CrossRef CAS.
- A. M. Girelli, D. Sperati and A. M. Tarola, Food Addit. Contam., Part A, 2014, 31, 703–710 CrossRef CAS PubMed.
- R. Stading, G. Gastelum, C. Chu, W. Jiang and B. Moorthy, Semin. Cancer Biol., 2021, 76, 3–16 CrossRef CAS PubMed.
- V. Bansal and K. H. Kim, Environ. Int., 2015, 84, 26–38 CrossRef CAS PubMed.
- Y. Sun, K. Yan, S. Wu and G. Gong, Food Control, 2020, 113, 107197 CrossRef CAS.
- M. C. R. Sola, A. G. Santos, S. T. Martinez, M. M. Nascimento, G. O. da Rocha and J. B. de Andrade, Sci. Rep., 2020, 10, 3465 CrossRef CAS PubMed.
- N. D. Dat and M. B. Chang, Sci. Total Environ., 2017, 609, 682–693 CrossRef CAS PubMed.
- A. G. Santos, A. C. Regis, G. O. da Rocha, A. Bezerra Mde, R. M. de Jesus and J. B. de Andrade, J. Chromatogr. A, 2016, 1435, 6–17 CrossRef CAS PubMed.
- G. L. Stroher, N. R. Poppi, J. L. Raposo and J. B. Gomes de Souza, Microchem. J., 2007, 86, 112–118 CrossRef.
- S. W. Wang, K. H. Hsu, S. C. Huang, S. H. Tseng, D. Y. Wang and H. F. Cheng, J. Food Drug Anal., 2019, 27, 815–824 CrossRef CAS.
- A. G. Gorshkov, O. N. Izosimova and O. V. Kustova, J. Anal. Chem., 2019, 74, 771–777 CrossRef CAS.
- S. Y. Lee, J. Y. Lee and H. S. Shin, Toxicol. Res., 2015, 31, 265–271 CrossRef CAS PubMed.
- V. A. Garcia Londono, C. M. Reynoso and S. Resnik, Food Addit. Contam., Part B, 2017, 10, 284–291 CAS.
- D. Bohrer, C. Viana, M. M. Barichello, J. F. de Moura, L. M. de Carvalho and P. C. Nascimento, JPEN, J. Parenter. Enteral Nutr., 2017, 41, 1037–1044 CrossRef CAS PubMed.
- M. M. Barichello, D. Bohrer, C. Viana, L. M. Carvalho and P. C. Nascimento, J. AOAC Int., 2017, 100, 1070–1076 CrossRef CAS PubMed.
- X. Song, J. Li, S. Xu, R. Ying, J. Ma, C. Liao, D. Liu, J. Yu and L. Chen, Talanta, 2012, 99, 75–82 CrossRef CAS PubMed.
- J. Pang, D. Yuan and X. Huang, J. Chromatogr. A, 2018, 1571, 29–37 CrossRef CAS PubMed.
- L. Han, Y. Sapozhnikova and S. J. Lehotay, Anal. Chim. Acta, 2014, 827, 40–46 CrossRef CAS PubMed.
- D. L. Norwood, D. Prime, B. P. Downey, J. Creasey, S. K. Sethi and P. Haywood, J. Pharm. Biomed. Anal., 1995, 13, 293–304 CrossRef CAS PubMed.
- S. Praveen, P. K. Chattopadhyay, P. Albert, V. G. Dalvi, B. C. Chakraborty and S. Chattopadhyay, Composites, Part A, 2009, 40, 309–316 CrossRef.
- Y. Qi, W. Fu, J. Tian, C. Luo, S. Shan, S. Sun, P. Ren, H. Zhang, J. Liu, X. Zhang and X. Wang, Nat. Commun., 2020, 11, 5051 CrossRef CAS PubMed.
- A. V. Ramanakumar, M. E. Parent, B. Latreille and J. Siemiatycki, Int. J. Cancer, 2008, 122, 183–189 CrossRef CAS PubMed.
- D. L. Norwood, D. Prime, B. P. Downey, J. Creasey, S. K. Sethi and P. Haywood, J. Pharm. Biomed. Anal., 1995, 13, 293–304 CrossRef CAS PubMed.
- D. Bohrer, C. Viana, M. M. Barichello, J. F. de Moura, L. M. de Carvalho and P. C. Nascimento, JPEN, J. Parenter. Enteral Nutr., 2017, 41, 1037–1044 CrossRef CAS PubMed.
- M. M. Barichello, D. Bohrer, C. Viana, L. M. Carvalho and P. C. Nascimento, J. AOAC Int., 2017, 100, 1070–1076 CrossRef CAS PubMed.
- L. R. Bordajandi, G. Gomez, E. Abad, J. Rivera, M. D. Fernandez-Baston, J. Blasco and M. J. Gonzalez, J. Agric. Food Chem., 2004, 52, 992–1001 CrossRef CAS.
- B. M. Lee and G. A. Shim, J. Toxicol. Environ. Health, Part A, 2007, 70, 1391–1394 CrossRef CAS PubMed.
- H. Kataoka, A. Ishizaki and K. Saito, Chim. Oggi – Chem. Today, 2010, 28, 21–24 CAS.
- T. Pena, L. Pensado, C. Casais, C. Mejuto, R. Phan-Tan-Luu and R. Cela, J. Chromatogr. A, 2006, 1121, 163–169 CrossRef CAS.
- I. A. Titaley, U. Eriksson and M. Larsson, J. Chromatogr. A, 2020, 1618, 460896 CrossRef CAS PubMed.
- A. Filipkowska, L. Lubecki and G. Kowalewska, Anal. Chim. Acta, 2005, 547, 243–254 CrossRef CAS.
- Y. Tian, M. Sun, X. Wang, C. Luo and J. Feng, Chromatographia, 2018, 81, 1053–1061 CrossRef CAS.
- M. D. Guillen and P. Sopelana, J. Dairy Sci., 2005, 88, 13–20 CrossRef CAS PubMed.
- J. Feng, M. Sun, X. Wang, Y. Tian, C. Luo and J. Feng, Chromatographia, 2018, 81, 1287–1292 CrossRef CAS.
- N. Yazdanfar, M. Shamsipur, M. Ghambarian and A. Esrafili, Chromatographia, 2018, 81, 487–499 CrossRef CAS.
- X. Fan, Z. Yuan, C. Shou, G. Fan, H. Wang, F. Gao, Y. Rui, K. Xu and P. Yin, Int. J. Nanomed., 2019, 14, 9631–9645 CrossRef CAS.
- H. Lee, S. M. Dellatore, W. M. Miller and P. B. Messersmith, Science, 2007, 318, 426–430 CrossRef CAS PubMed.
- J. Ran, M. He, W. Li, D. Cheng and X. Wang, Polymers, 2018, 10, 495 CrossRef PubMed.
- P. G. Ingole, W. Choi, K. H. Kim, C. H. Park, W. K. Choi and H. K. Lee, Chem. Eng. J., 2014, 243, 137–146 CrossRef CAS.
- E. Faure, C. Falentin-Daudre, C. Jerome, J. Lyskawa, D. Fournier, P. Woisel and C. Detrembleur, Prog. Polym. Sci., 2013, 38, 236–270 CrossRef CAS.
- Y. Wang, S. Wang, H. Niu, Y. Ma, T. Zeng, Y. Cai and Z. Meng, J. Chromatogr. A, 2013, 1283, 20–26 CrossRef CAS PubMed.
- Y. R. Ma, X. L. Zhang, T. Zeng, D. Cao, Z. Zhou, W. H. Li, H. Niu and Y. Q. Cai, ACS Appl. Mater. Interfaces, 2013, 5, 1024–1030 CrossRef CAS.
- Y. Cai, Z. Yan, M. Yang, X. Huang, W. Min, L. Wang and Q. Cai, J. Chromatogr. A, 2016, 1478, 2–9 CrossRef CAS.
- V. Ball, D. D. Frari, V. Toniazzo and D. Ruch, J. Colloid Interface Sci., 2012, 386, 366–372 CrossRef CAS.
- S. Nagamine, Y. Mizuno, Y. Hikima, K. Okada, L. Wang and M. Ohshima, J. Appl. Polym. Sci., 2021, 138, 49851 CrossRef CAS.
- G. K. Gaurav, T. Mehmood, M. Kumar, L. Cheng, K. Sathishkumar, A. Kumar and D. Yadav, J. Contam. Hydrol., 2021, 236, 103715 CrossRef CAS.
- H. Y. Li, P. P. Gao and H. G. Ni, Sci. Total Environ., 2019, 665, 11–17 CrossRef CAS PubMed.
|
This journal is © The Royal Society of Chemistry 2023 |
Click here to see how this site uses Cookies. View our privacy policy here.