DOI:
10.1039/D2RA07636E
(Paper)
RSC Adv., 2023,
13, 2852-2859
A novel double metal-dithizone functionalized polyurethane electrospun nanofiber and film for colorimetric determination of hexavalent chromium†
Received
30th November 2022
, Accepted 8th January 2023
First published on 18th January 2023
Abstract
This work proposes a highly specific method of Cr6+ determination based on the double reactions of two metals, Co2+ with dithizone to form a (DTZ)-Co2+ complex, and the replacement of Co2+ in the formed complex with Cr6+. The fast degradation of DTZ in solution in wet analysis was overcome by preparing dithizone functionalized polyurethane nanofibers that were electrospun into a membrane (DTZ/PU-NF) and a microwell plate film (DTZ/PU-MPF). For comparison, the performance of diphenylcarbazide (DPC), a currently used complexing agent for Cr6+, was also investigated. Colour changes were detected as red-green-blue values. The DTZ/PU-NF was smooth, with an average diameter of 384.09 nm and no bead appeared. A dense network structure was formed. The best formulation of DTZ, PU and Co2+ was also applied as a microwell plate film. In the presence of Cr6+, the colour of DTZ-Co2+ changed from red to magenta. Among the three studied methods, the colorimetric DTZ-Co2+/PU-NF presented the best results. Its linearity range was 0.001–1.0 mg L−1, with a regression equation of Cr6+ = −0.189 + (0.0056 × red) + (0.0086 × green) − (0.0129 × blue), R2 of 0.990. The limit of detection was 0.001 mg L−1 and the precision was 1.7%. The applicability of DTZ/PU-NF was validated for Cr6+ in vegetable oils with recoveries of 89.5–116.8%. The sensitivity of DTZ/PU-NF was ten times higher than that of DTZ/PU-MPF. The methods based on DTZ-Co2+/PU-NF and DTZ-Co2+/PU-MPF proved to be highly selective, rapid, user-friendly, simple and reliable.
1. Introduction
The detection of heavy metals is of great importance in the field of environmental, water, and food monitoring. Chromium is one of the most toxic heavy metals. It is highly carcinogenic, mutagenic, and allergenic and has genetic effects that can be inherited.1,2 Among chromium ions, hexavalent chromium (Cr6+) is of most concern since it is more toxic than other chromium ion forms. The United States Environmental Protection Agency (US EPA) has set the maximum contaminant level of total chromium in drinking water at only 0.1 mg L−1, while the maximum permissible concentration of Cr6+ in vegetable oils has not been mentioned in Codex standard unlike lead and arsenic which were set at 0.1 mg kg−1.3,4
Several analytical methods have been developed for Cr6+ determination. The most used methods are based on atomic absorption and emission, as well as inductively coupled plasma spectrometry.5 Visible spectrophotometry, the less used technique, was attempted in the determination of total chromium with limits of detection (LOD) ranging from mg L−1 to μg L−1, depending on the preconcentration technique, but with a necessary pretreatment step to ensure selectivity toward the studied species. Cr6+ determination by colorimetry with the reagent diphenylcarbazide (DPC) gave the LOD of 0.023 mg L−1,6 and determination with special 2,2-azino-bis(3-ethylbenzo-thiazoline-6-sulfonic acid) diammonium salt achieved the LOD of 7.87 μg L−1.7 Another colorimetry for Cr6+ determination in water and soil samples which based on an oxidative coupling reaction between 3-aminophenol (3AP) and 4-aminoantipyrine (4-AAP) as a chromogen in the presence of peroxidase mimetic Fe2O3 nanoparticles (IONPs) as a catalyst. This method's limits of quantification and detection are 0.003 mg mL−1 and 0.0009 mg mL−1,8 respectively. Despite the advantages of reported techniques, some limitations must be acknowledged. The use of non-specific reagents and multi-step processes, the required laboratory-based analysis, sophisticated equipment, and skilled personnel can add costs and time. Hence, it is necessary to develop a highly selective, reliable, simple, rapid, and portable method of determining Cr6+.
Colorimetry is a promising technique that enables qualitative and quantitative visual detection of analytes based on visible colour changes detected without complicated equipment. Previous studies of Cr6+ determination have mostly focused on the use of gold (Au) and silver (Ag) nanoparticles (NPs) due to their property of surface plasmon resonance.9 For example, gallic acid-modified AuNPs with a 2 μM LOD,2 meso-2,3-dimercaptosuccinic acid capped AuNPs with a 0.01 μM LOD by naked eye11 and biosynthesized AgNPs with a 0.1 mg L−1 LOD.10 A study that did not use metal NPs found that a DPC-coated cellulose strip could detect Cr6+ at a concentration of 1.43 μg L−1.12 However, the use of polymer nanofiber (NF) for colorimetric Cr6+ determination is limited and a more selective method with complexing reactions would also be needed to overcome interferences in complex sample matrices.
To provide good support for redox and complexing reactions, an electrospun NF membrane has several advantages. Using electrostatic force, the electrospinning technique produces high tensile strength membranes with easily-modified surfaces that present a large area with pore diameters between 300 nm and 5 μm.13 To design an NF-based colorimetric sensor, target-specific molecules can either be blended in a polymeric solution prior to electrospinning or immobilized on the polymer NF. Some previous works reported the use of nylon 6/polyvinylidene fluoride combined with AuNPs (naked-eye LOD: 100 μg L−1) for Pb2+,14 2-(2′-pyridyl)imidazole/poly(vinylbenzyl chloride) for Fe2+ (LOD: 2 mg L−1),15 and zein for Fe3+ (LOD: 0.4 mg L−1).16 Moreover, many types of NF have been used for the adsorption or removal of Cr6+. NF has been produced from chitosan, polyaniline, polypyrrole-polyaniline, polyacrylonitrile/polypyrrole, and hollow carbon nanofibers17–21 but, to the best of our knowledge, there has been no study regarding NF for Cr6+ detection.
Polyurethane (PU) is a polymer with great potential for various applications. It consists of hard segments from urethane bonds and aromatic rings, and soft segments from polyol units22 and can therefore be hard like plastics or soft like rubber, depending on the monomer used. It is also known that PU has good mechanical properties, is highly resistant to water, oil, chemicals, and extreme temperature, and can thus maintain its stability.23 PU nanofibers with diameters ranging from 37 to 300 nm have been fabricated by electrospinning.22,24–26 With these benefits in mind, we chose PU-NF as the ESI† for the Cr6+ colorimetric reaction.
We fabricated a metal chelator-functionalized PU-NF for Cr6+ colorimetric determination by electrospinning. An indicator was needed to make the PU-NF specific for Cr6+. We selected dithizone (DTZ), also known as 1,5-diphenylthiocarbazone, a universal metal chelator with a high-density colour. The electrospun PU-NF was functionalized with DTZ to obtain DTZ/PU-NF. To increase the selectivity of the method, DTZ was first complexed with Co2+ which would be replaced with Cr6+ in the oxidoreduction and colorimetric reactions. The DTZ-Co2+/PU-NF was successfully developed and its performance in the presence of Cr6+ was investigated. The linearity range, detection limit, accuracy, and precision were determined. The colour changes in the presence of different concentrations of Cr6+ were recorded as digital images. The Red-Green-Blue (RGB) data from the captured images was analysed using ColorValue version 1.0.0. Calibration curve processing was performed by SigmaPlot software version 14.0 (Systat Software Inc., USA). For comparison with the DTZ-Co2+/PU microwell plate film (DTZ-Co2+/PU-MPF), diphenylcarbazide (DPC), the complexing agent employed in the US EPA 7196A method,27 was also investigated. The quantitative sensing of Cr6+ in vegetable oil was investigated based on the DTZ-Co2+/PU-NF and DTZ-Co2+/PU-MPF systems.
2. Experimental
2.1 Chemicals
Polyurethane (PU), dithizone (DTZ) and nitric acid (HNO3) were purchased from Merck (Germany). Diphenylcarbazide (DPC) and hexane were from LOBA Chemie (India). Dimethylformamide (DMF) was purchased from JT Baker (USA). Cobalt(II) nitrate hexahydrate and potassium dichromate (K2Cr2O7) were purchased from Sigma-Aldrich (Germany). The standard stock solution of 1000 mg L−1 Cr6+ was prepared from dried K2Cr2O7.
2.2 Preparation of DTZ/PU-NF and DPC/PU-NF
The NF was spun using a lab-made electrospinning instrument comprising a 25 kV high-voltage power supply (PHYWE, Germany), a syringe pump (NewEra, USA), and a flat plate collector covered with aluminium foil. To optimize the electrospinning conditions of PU-NF, PU solutions were prepared at concentrations of 10, 15, 20, and 25%. Briefly, PU pellets were dissolved in DMF, and the solution was mixed manually with vortex mixer and sonicated for 30 min. The solution was then mixed again and kept for 24 h to allow PU to be completely dissolved in DMF. This solution was loaded into a 25 mL plastic syringe connected to a stainless-steel needle with an inner tip diameter of 0.6 mm and electrospinning using a 15 kV voltage, at a flow rate of 0.3 mL h−1, a 15 cm tip-collector distance and a spinning time of 1 h. The electrospinning instrument was set up horizontally. The obtained PU-NF was collected at the grounded surface of an aluminium collector and dried at ambient temperature for 12 h. Apart from PU concentration, three more key parameters were investigated during electrospinning for 1 h. The parameters included the applied voltage (15 and 20 kV), flow rate (0.3 and 0.5 mL h−1), and the distance between the two electrodes, or tip-collector distance (10, 15, and 20 cm).
To prepare DTZ/PU-NF and DPC/PU-NF, electrospinning solutions of DTZ or DPC and PU were prepared by mixing 15% PU with 2.5, 5.0, and 7.5% DTZ or DPC. Briefly, after preparing the PU solution, DTZ or DPC was added based on the weight of PU solution and the mixture was magnetically stirred for 1 h. This solution was electrospun in the optimum electrospinning condition.
2.3 DTZ-first metal (Co2+) functionalized PU membrane (DTZ-Co2+/PU-NF)
To establish a highly selective system for Cr6+ determination, the double metal-complex reaction was investigated. DTZ was complexed with Co2+ and Cr6+ was added to replace Co2+ in the DTZ-Co2+ complex as successfully reported in a wet analysis.28 To prepare the DTZ-Co2+ complex, 20 μL of Co2+, as cobalt nitrate at 25, 50, and 100 mg L−1, was dropped onto the surface of the electrospun DTZ/PU-NF membrane. The membrane was dried in a dark compartment, and 20 μL of Cr6+ standard solution was dropped onto the dried membrane at the studied concentrations. The colour changes were recorded with a camera and the RGB values at three different points were evaluated and statistically analysed.
2.4 DTZ-Co2+/PU microwell plate film (DTZ-Co2+/PU-MPF)
The specific detection of Cr6+ using DTZ-Co2+ immobilized in PU-NF was successful developed. Strong colours were produced in few seconds. The oxidation of Co2+ in DTZ-Co2+/PU-MPF by Cr6+ was confirmed by visible light absorption measurement (UV-visible spectrophotometer; Shimadzu, model 2600i). The production of the materials was developed to lower costs and shorten the time required for analysis. This was achieved by using the same double metal reactions in a microwell plate film (MPF) system. Since the colour of DTZ was easier to detect on the PU-MPF than on the PU-NF, lower concentrations of 0.0625, 0.125, 0.25, 0.5, and 1% of DTZ in 10% PU-MPF could be studied. The RGB colour spaces of pure DTZ, DTZ-Co2+, and DTZ-Co2+ with Cr6+ were investigated using a cubic experimental design.
2.5 Analytical method validation
Prior to each test, a series of Cr6+ standard solutions were freshly prepared in 1% HNO3 at concentrations of 0.001, 0.005, 0.01, 0.05, 0.1, 0.5, 1, 5, and 10 mg L−1. Vegetable oil samples were diluted with hexane before testing. The tests were performed as follows; firstly, the dried DTZ/PU-NF and DTZ/PU-MPF were functionalized with 20 μL of 50 mg L−1 Co2+ in 1% HNO3 dropped onto the surface. The responses of the systems to the concentration of Cr6+ in standard and sample solution were then tested. For the standard solution, 10 μL of each Cr6+ standard concentration were dropped onto the DTZ/PU-NF or DTZ/PU-MPF surface, followed by 10 μL of hexane. For the oil sample, 10 μL of 2% HNO3 and 10 μL of diluted oil sample were dropped, respectively. The colour change was determined with the naked eye and photographed. RGB values were processed statistically using SigmaPlot. The sensitivity of the method was determined by the limit of detection and limit of quantitation (LOQ) based on a signal-to-noise ratio of 3 and 10, respectively.29 Precision and repeatability was expressed as relative standard deviation (RSD) (n = 5) and the accuracy of both systems was reported as recoveries after spiking samples with 10 μL of 10 mg L−1 Cr6+. An interference study was carried out involving metals reported in a study of palm oil.30 Thirteen metal ions, Ca2+, Mg2+, Zn2+, Cu2+, Fe3+, Fe2+, Mn2+, Ni2+, Pb2+, As3+, Se2+, Cd2+ and Hg2+, were investigated at a concentration of 10 mg L−1.
2.6 Preparation of vegetable oil samples
Eight different vegetable oils were purchased from supermarkets located in Songkhla and Pattani Provinces, Thailand. A 1 mL aliquot of each sample was transferred to the test tube and diluted with hexane. Before the colorimetric assay, the prepared samples were stored at ambient temperature. The concentrations of Cr6+ in the same set of samples were determined with the DTZ-Co2+/PU-NF and DTZ-Co2+/PU-MPF systems and confirmed by inductively coupled plasma-mass spectrometry (ICP-MS, NexION2000, PerkinElmer, USA).
2.7 Sensing of Cr6+ in samples
To measure Cr6+ in the DTZ/PU-NF system, 20 μL of 50 mg L−1 reducing agent (Co2+) were dropped onto the surface of the membrane and incubated in a dark compartment for 10 min. The functionalised membrane was removed from the compartment and 10 μL of 2% HNO3 and diluted oil sample were dropped onto the membrane. The colour of the membrane was assessed and RGB values were analysed. To functionalise the DTZ/PU-MPF system, 40 μL of optimum DTZ-Co2+/PU were dropped onto the microwell plate and allowed to dry for 10 min before HNO3 and diluted samples were added. To obtain the Cr6+ concentration, RGB values were calculated from the standard curve equation plotted with SigmaPlot (Fig. S1†).
3. Results and discussion
3.1 Optimization of electrospinning conditions for PU-NF
The electrospinning technique has gained attention since it is useful for fabricating sub-micron-sized polymer fibers that can be applied as a sensing material in tissue engineering, drug delivery, and enzyme immobilization. To achieve the desired membrane, the parameters influencing the electrospinning of PU-NF were investigated, including PU concentration, applied voltage, flow rate, and tip-collector distance. The respective data and figures are discussed in the section titled ESI† (Fig. S2–S4). The optimum parameters were as follows: (a) PU concentration of 15%, (b) applied voltage of 20 kV, (c) flow rate of 0.3 mL h−1, and (d) tip-collector distance of 20 cm.
Based on SEM analysis of the microstructure of PU-NF, the fibers of PU-NF produced with 20% PU were bigger than the fibers of PU-NF produced with 15% PU (Fig. 1A and B). The corresponding diameter distributions are shown in Fig. S3.† The 15% PU-NF (758.33 ± 268.19 nm) contained a bandwidth distribution of diameter and had a maximum intensity frequency in the range of 601–700 nm. For the 20% PU-NF (872.84 ± 481.56 nm), it is clearly seen that 20% PU-NF has non-uniformity in terms of width containing the first width locates about 301–500 nm and the second about >900 nm.
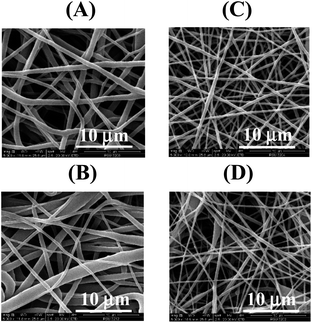 |
| Fig. 1 SEM images of PU-NF produced at (A) 15% PU and (B) 20% PU. The electrospinning conditions were: 20 kV, 0.3 mL h−1, 20 cm and 1 h. SEM images of (C) 2.5% DTZ/PU-NF and (D) 2.5% DPC/PU-NF. The electrospinning conditions were: 20 kV, 0.3 mL h−1, 20 cm and 1.5 h. Magnification of 5000×. | |
3.2 Comparison of DTZ/PU-NF and DPC/PU-NF
DTZ is an organic reagent that has been applied for qualitative and quantitative analysis of many heavy metals, while DPC is a complexing agent used as a reagent for Cr6+ in the US EPA method27 and has been coated on cellulose membrane to determine Cr6+ in plated-metallic sheets.11 In the presence of metal complexes, DTZ produces a wider colour range with higher intensity than DPC. It is believed that the connection between divalent metal and DTZ is caused by the formation of a penta-heterocycles chelating complex between metal and the dithizone molecule. In this complex, nitrogen and sulphur atoms act as donors.31
In the present study, DTZ and DPC were used as complexing agents for comparative purposes. Each was mixed with PU to obtain DTZ/PU-NF and DPC/PU-NF by electrospinning. Initially, 2.5% DTZ or DPC mixed with 15% PU was tried, and it was discovered that the DTZ/PU-NF maintained its dark green hue and dense network of smooth threads (Fig. 1C). Meanwhile, the DPC/PU-NF was light grey, with beaded fibers (Fig. 1D). Because the complexing agent was extremely sensitive to light, moisture, and air, the functionalized NF had to be stored in dry and dark container to avoid oxidation.
The thermal behaviours of the PU-NF, 5% DTZ/PU-NF and 5% DPC/PU-NF were characterized by thermogravimetric analysis (Fig. 2A and Table S1†). All three NF curves showed one step of decomposition. At 300 °C, the PU-NF had lost practically no weight, however, from 350 °C weight loss was fast, reaching trace residue at 600 °C. Similar to the thermal degradation of PU-NF, both the 5% DTZ/PU-NF and 5% DPC/PU-NF initially decomposed at ∼300 °C and persisted as traces of residue after 500 °C. The 5% DTZ/PU-NF had the lowest degradation peak temperature, followed by 5% DPC/PU-NF and PU-NF, meaning that 5% DTZ/PU-NF showed the best resistance towards high temperature. Also, the main diameter of 5% DTZ/PU-NF had a similar size (384.09 ± 110.73 nm) to 5% DPC/PU-NF (351.63 ± 183.61 nm). However, the formation of beads in the fibers was found in 5% DPC/PU-NF.
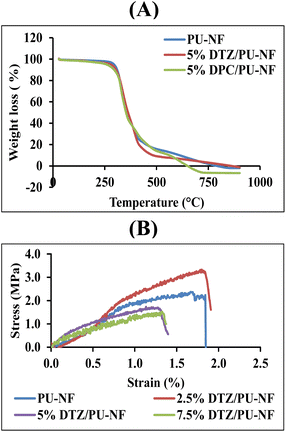 |
| Fig. 2 (A) Thermogravimetric curves of PU-NF, 5% DTZ/PU-NF and 5% DPC/PU-NF. (B) Stress versus strain curve of PU-NF and DTZ/PU-NF at various concentrations of DTZ. The 15% PU solution was used for all studies. | |
The mechanical properties of DTZ/PU-NF were evaluated in terms of the stress–strain curve and elongation at break. 2.5, 5.0, and 7.5% DTZ/PU-NF exhibited similar linear elastic behaviour to PU-NF, which is the inherent characteristic behaviour of thermoplastic PU polymer. Tensile strength significantly decreased with increments of DTZ loading (Fig. 2B). It was found that the elongation at the break of 2.5% DTZ/PU-NF (178.2 ± 50.0%) was the same as that of PU-NF (166.8 ± 25.1%). The addition of one component to an electrospinning solution was reported to decrease membrane tensile strength.32 All of the NFs examined produced greater than 100% elongation at break, while DTZ loadings greater than 2.5% significantly inhibited DTZ/PU-NF elongation at break. However, the mechanical properties were not taken into account for the purposes of Cr6+ colorimetric detection.
3.3 DTZ-Co2+/PU-NF for Cr6+ detection
It has been reported that among several metals which can form a coloured complex with DTZ, Co2+ and Zn2+ gave the strongest colours; red and purple, respectively. However, unlike Zn2+, the presence of Co2+ has hardly been mentioned in natural samples.33,34 To make the DTZ/PU-NF more selective, we chose Co2+ to react with DTZ in the first stage of this investigation. The colour changed from red to magenta after Cr6+ was introduced to the testing area holding the DTZ-Co2+ combination, which could not be seen when tested with other common metals prevalent in fruit juice as described in a wet analysis.28 Fig. 3 shows the mechanism of detection based on DTZ-Co2+/PU-NF. DTZ can be either immobilized on the fibers by the conjugation of the benzene ring of DTZ and polystyrene or deposited on the surface of the membrane.35 The reaction between DTZ and Co2+ has been discussed previously.28,35 In the reaction, DTZ can coordinate with Co2+ via nitrogen and sulphur atoms as donors to form a penta-heterocycles complex. The red DTZ-Co2+/PU-NF becomes magenta after adding Cr6+. It should be noted that if the redox reaction is involved in this colour shift, standard Cr6+ (Cr2O72−, E0red = +1.33 V) electrode potential values might autonomically oxidize Co2+ (E0red = −0.28 V) in DTZ-Co2+, creating Cr3+ in the system.
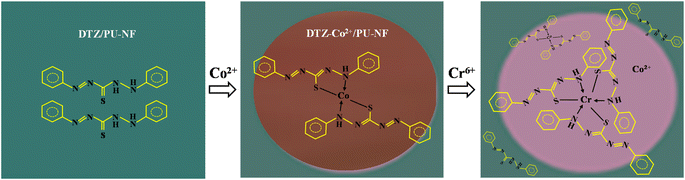 |
| Fig. 3 Proposed mechanism of Cr6+ detection based on DTZ-Co2+/PU-NF. | |
To find the concentration of Co2+ that will bind optimally to DTZ, Co2+ concentrations of 25, 50, and 100 mg L−1 were studied (Fig. 4A). Higher concentrations of Co2+ gave significantly higher red values (p < 0.05). The red colour is preferable here due to its contrast with green DTZ, and it can persist longer. The concentration of Co2+ at 100 mg L−1 was, therefore, considered optimal.
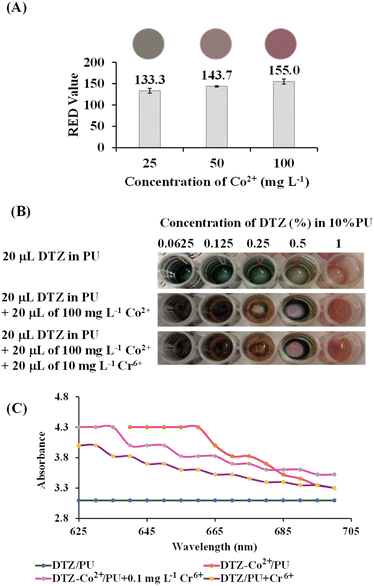 |
| Fig. 4 (A) Effect of Co2+ concentrations in the DTZ/PU-NF system. (B) Photograph of the PU-MPF system with various concentrations of DTZ in the presence of Co2+ and Cr6+. (C) UV-visible spectra of DTZ/PU-MPF without and with Cr6+ in DTZ-Co2+/PU-MPF. | |
To optimize the DTZ concentration for the PU-MPF system, concentrations of DTZ were studied ranging from 0.0625 to 1% (Fig. 4B). After the addition of Co2+ and Cr6+, DTZ at 0.5% was found to be the optimal concentration as the difference between film colour and visible light absorption (Fig. 4C) without and with Cr6+ could be differentiated and therefore the PU-MPF system was fabricated with 0.5% DTZ-Co2+.
3.4 Method performances
The colour chart of the 5% DTZ-Co2+/PU-NF system was established at Cr6+ concentrations from 0.001 to 10 mg L−1. The resulting colour changes were compared with the colour changes produced by the same Cr6+ concentrations in the 7.5% DPC/PU-NF system (Fig. 5A and B). In the DTZ-Co2+/PU-NF system, a colour change could be detected by the naked eye at a Cr6+ concentration of 0.001 mg L−1 while in the DPC/PU-NF system, a colour change could only be detected at 0.1 mg L−1 Cr6+. This demonstrated that 5% DTZ-Co2+/PU-NF gave a better sensitivity than 7.5% DPC/PU-NF, although DPC/PU-NF worked better in a higher range.
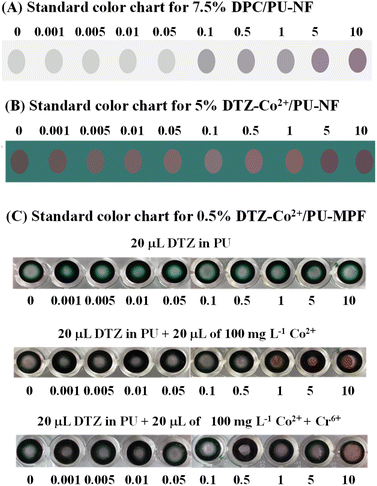 |
| Fig. 5 Colour charts are for Cr6+ concentrations ranging from 0.001 to 10 mg L−1 detected by (A) DPC/PU-NF, (B) DTZ-Co2+/PU-NF, and (C) 0.5% DTZ-Co2+/PU-MPF. A 10% PU solution was used. | |
Moreover, DTZ-Co2+/PU-NF produced a wider shading of colour or linearity (0.001–10 mg L−1 of Cr6+) than DPC/PU-NF (0.1–10 mg L−1 of Cr6+). The DTZ-Co2+/PU-MPF system produced colour changes from red to magenta with increments of Cr6+ concentration from 0.01–5.0 mg L−1 (Fig. 5C).
The overall results of the method validation are summarized in Table 1 and in comparison, with other methods in Table S2.† Among the three studied methods, the DTZ-Co2+/PU-NF system exhibited the lowest LOD and LOQ values, followed by DPC/PU-NF and DTZ-Co2+/PU-MPF. The LODs achieved by these methods were also lower than the US EPA limit of total chromium in drinking water (0.1 mg L−1).3 Even though the DTZ-Co2+/PU-MPF system could not detect low concentrations of Cr6+, it has advantages in terms of lower analysis costs and shorter production time. Thus, the DTZ-Co2+/PU-NF and DTZ-Co2+/PU-MPF systems were further studied for recovery and precision. The DTZ-Co2+/PU-NF system achieved recoveries from 89.5 to 116.8%, with a RSD of 1.7%, while the DTZ-Co2+/PU-MPF system achieved recoveries from 80.0 to 137.5%, with a RSD of 4.7%.
Table 1 Method validation results of DTZ-Co2+/PU-NF, DPC/PU-NF and DTZ-Co2+/PU-MPF
Parameter |
DTZ-Co2+/PU-NF |
DPC/PU-NF |
DTZ-Co2+/PU-MPF |
Linearity range (mg L−1) |
0.001–1.0 |
0.1–10.0 |
0.01–5.0 |
Equation |
Cr6+ (mg L−1) = −0.189 + (0.0056 × red) + (0.0086 × green) − (0.0129 × blue) |
Cr6+ (mg L−1) = −12.159 − (0.0094 × red) − (0.4980 × green) + (0.5710 × blue) |
Cr6+ (mg L−1) = −4.280 + (0.1194 × red) − (0.3290 × green) + (0.2334 × blue) |
R2 |
0.990 |
0.990 |
0.964 |
Colour shades |
Dark to light reddish brown |
Grey to magenta |
Red to magenta |
LOD (mg L−1) |
0.001 |
0.010 |
0.018 |
LOQ (mg L−1) |
0.007 |
0.030 |
0.060 |
3.5 Interference investigation
The interference study was based on Cr6+ detection by the DTZ-Co2+/PU-NF system (Fig. 6A). Some metal cations mentioned in a previous work31 were investigated, including Ca2+, Mg2+, Zn2+, Cu2+, Fe3+, Fe2+, Mn2+, Ni2+, Pb2+, As3+, Se2+, Cd2+, and Hg2+, with each at a high concentration of 10 mg L−1. The colour change observed by the naked eye showed that no interfering cation affected the colour response to Cr6+, indicating that the double reaction on DTZ/PU-NF is selective toward Cr6+.
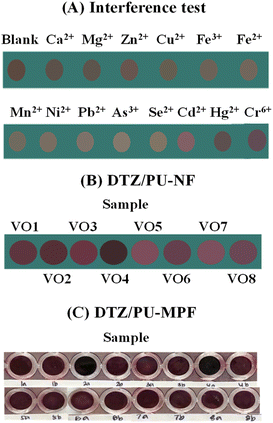 |
| Fig. 6 (A) The interference study for Cr6+ was based on the DTZ-Co2+/PU-NF system (each at 10 mg L−1). Detection of Cr6+ in non-spiked and spiked vegetable oil samples was based on (B) the DTZ-Co2+/PU-NF system and (C) the DTZ-Co2+/PU-MPF system. | |
3.6 Sample applications
The DTZ-Co2+/PU-NF and DTZ-Co2+/PU-MPF systems were applied as sensors for Cr6+ determination in vegetable oil samples. As seen in Fig. 6B, the naked eye could detect different colours on the DTZ-Co2+/PU-NF membrane. These could be compared with the standard colour chart of Cr6+ (Fig. 5B).
The RGB values from digital images were analysed based on the standard equation of Cr6+ for DTZ-Co2+/PU-NF (Table 1). While the DTZ-Co2+/PU-MPF system was able to find the detectable concentration level (1 mg L−1 Cr6+) in spiked samples (Fig. 6C), both the naked eye and RGB analysis were able to differentiate the colour. However, the results showed that there was no detectable Cr6+ in any non-spiked samples which agreed well with the results of the DTZ-Co2+/PU-MPF system and the ICP-MS method. Therefore, the concentration of Cr6+ in all samples did not exceed the USP Oral Component Limit (OCL) for chromium (25 mg g−1).35
4. Conclusions
In this work, we successfully developed a novel polyurethane nanofiber (PU-NF) with selective reactions of a complexing agent (DTZ) and double metal reactions, Co2+ and Cr6+. The nanofiber was electrospun into a membrane for Cr6+ detection. The high porosity of the smooth, multi-layered nanofiber increased reagent adsorption. The electrospun membrane exhibited good stability and ease of use. In addition, we reported the first use of double reactions for Cr6+, making the method more specific without any interference from metals commonly found in vegetable oils. This novel membrane showed promise for application in food quality control. Moreover, a microplate film with the same double reactions was developed. The complex reaction on the developed materials makes the detection of Cr6+ easier and more convenient. There is no need for sensitive reagent preparation. The produced colours were dense and could be identified easily with the naked eye, or with RGB data for quantitative analysis. The DTZ-functionalized nanofiber with Co2+ showed very good sensitivity with an LOD of 0.001 mg L−1 and gave a wide linear range (0.001–1.0 mg L−1). The DTZ-functionalized microwell plate film with Co2+ showed a higher LOD (0.018 mg L−1), with a narrower linearity range of 0.01–5.0 mg L−1 but it was very easy to prepare and apply. These highly sensitive and reliable new systems could be used with a variety of sample types, including environmental samples.
Author contributions
Indiah Ratna Dewi: writing – original draft, investigation, data curation, validation. Thitima Rujiralai: writing – original draft, writing – review & editing, investigation, validation, supervision. Chatchai Putson: methodology, investigation, and supervision. Wilairat Cheewasedtham: writing – original draft, investigation, writing – review & editing, project administration, validation, supervision, conceptualization, resources.
Conflicts of interest
There are no conflicts to declare.
Acknowledgements
This work was supported by Prince of Songkla University (PSU); a Graduate Study grant from Thailand's Education Hub for the Southern Region of ASEAN Countries, the Project Office of the Higher Education Commission (TEH-AC); the Faculty of Science and Technology, PSU; Faculty of Science, PSU; the Graduate School, PSU; and Lin Shing Enterprise (Thailand) Co., Ltd. We acknowledge the Center for Leather, Rubber, and Plastics, Ministry of Industry, Republic of Indonesia for staff support. We also thank Mr Thomas Duncan Coyne and Mrs Anna Chatthong for English editing.
References
- T. S. Najim and A. J. Salim, Arabian J. Chem., 2014, 10, S3459–S3467 CrossRef.
- C. Dong, G. Wu, Z. Wang, W. Ren, Y. Zhang, Z. Shen, T. Li and A. Wu, Dalton Trans., 2016, 45, 8347–8354 RSC.
- United States Environmental Protection Agency, Chromium in Drinking Water, 2021 Search PubMed.
- Codex Standard for Named Vegetable Oils (CODEX-STAN 210 – 1999), https://www.fao.org/3/y2774e/y2774e04.htm#bm4.1, accessed 2, 11, 2022 Search PubMed.
- V. Gómez and M. P. Callao, Trends Anal. Chem., 2006, 25(10), 1006–1015 CrossRef.
- A. Lace, D. Ryan, M. Bowkett and J. Cleary, Int. J. Environ. Res. Public Health, 2019, 16(10), 1803 CrossRef CAS PubMed.
- J. Li, H. Wei, S. Guo and E. Wang, Anal. Chim. Acta, 2008, 630, 181–185 CrossRef CAS PubMed.
- N. A. Chamaraja, B. Mahesh and C. B. Praveen Kumar, Environ. Nanotechnol., Monit. Manage., 2021, 16, 100471 Search PubMed.
- V. S. A. Piriya, P. Joseph, S. C. G. K. Daniel, S. Lakshmanan, T. Kinoshita and S. Muthusamy, Mater. Sci. Eng., C, 2017, 78, 1231–1245 CrossRef PubMed.
- W. Chen, F. Cao, W. Zheng, Y. Tian, Y. Xianyu, P. Xu, W. Zhang, Z. Wang, K. Deng and X. Jiang, Nanoscale, 2015, 7, 2042–2049 RSC.
- M. Ismail, M. I. Khan, K. Akhtar, M. Ali, A. M. Asiri and S. Bahadar, Phys. E, 2018, 103, 367–376 CrossRef CAS.
- S. Danwittayakul and A. Thanaboonsombut, Nucl. Sci. J., 2009, 6(S1), 64–72 Search PubMed.
- S. Thenmozhi, N. Dharmaraj, K. Kadirvelu and H. Y. Kim, Mater. Sci. Eng. Carbon, 2017, 217, 36–48 CrossRef CAS.
- Y. Li, B. Ding, G. Sun, T. Ke, J. Chen, S. S. Al-Deyab and J. Yu, Sens. Actuators, B, 2014, 204, 673–681 CrossRef CAS.
- D. A. Ondigo, Z. R. Tshentu and N. Torto, Anal. Chim. Acta, 2013, 804, 228–234 CrossRef CAS PubMed.
- A. Saithongdee, N. Praphairaksit and A. Imyim, Sens. Actuators, B, 2014, 202, 935–940 CrossRef CAS.
- M. Jiang, T. Han, J. Wang, L. Shao, C. Qi, X. M. Zhang, C. Liu and X. Liu, Int. J. Biol. Macromol., 2018, 120, 213–221 CrossRef CAS PubMed.
- T. Li, Z. Qin, Y. Shen, X. Xu, N. Liu and Y. Zhang, Mater. Lett., 2019, 252, 130–133 CrossRef CAS.
- M. Bhaumik, A. Maity, V. V. Srinivasu and M. S. Onyango, Chem. Eng. J., 2012, 181–182, 323–333 CrossRef CAS.
- J. Wang, K. Pan, Q. He and B. Cao, J. Hazard. Mater., 2013, 244–245, 121–129 CrossRef CAS PubMed.
- S. Mishra and N. Verma, J. Ind. Eng. Chem., 2016, 36, 346–354 CrossRef CAS.
- Y. Morita, G. Koizumi, H. Sakamoto and S.-I. Suye, Mater. Chem. Phys., 2017, 187, 1–4 CrossRef CAS.
- J. O. Akindoyo, M. D. H. Beg, S. Ghazali, M. R. Islam, N. Jeyaratnam and A. R. Yuvaraj, RSC Adv., 2016, 6, 114453–114482 RSC.
- M.-S. Khil, D.-l. Cha, H.-Y. Kim, I.-S. Kim and N. Bhattarai, J. Biomed. Mater. Res., 2003, 67B(2), 675–679 CrossRef CAS PubMed.
- H.-Y. Mi, Y. Jiang, X. Jing, E. Enriquez, H. Li, Q. Li and L.-S. Turng, Mater. Sci. Eng., C, 2019, 98, 241–249 CrossRef CAS PubMed.
- W. Yang, R. Li, C. Fang and W. Hao, Prog. Org. Coat., 2019, 131, 67–72 CrossRef CAS.
- United States Environmental Protection Agency, SW-846 Test Method 7196A: Chromium, Hexavalent (Colourimetric), 1992 Search PubMed.
- I. R. Dewi, C. Putson, T. Rujiralai and W. Cheewasedtham, The 45th Congress on Science and Technology of Thailand (STT 45), Mae Fah Luang University, Chiang Rai, Thailand, 2019, pp. 271–281 Search PubMed.
- J. N. Miller and J. C. Miller, Statistics and chemometrics for analytical chemistry, Pearson Education Limited, Essex, England, 2005, 5th edn Search PubMed.
- O. B. Olafisoye, O. S. Fatoki, O. O. Oguntibeju and O. A. Osibote, Toxicol. Rep., 2020, 7, 324–334 CrossRef CAS PubMed.
- H. Tavallali, G. Deilamy-Rad, A. Parhami and S. Z. Mousavi, J. Photochem. Photobiol., B, 2013, 125, 121–130 CrossRef CAS PubMed.
- M. A. Al-Baadani, K. H. R. Yie, A. M. Al-Bishari, B. A. Alshobi, Z. Zhou, K. Fang, B. Dai, Y. Shen, J. Ma, J. Li and X. Shen, Mater. Des., 2021, 209, 109962 CrossRef CAS.
- Y. Pourshojaei and A. Nasiri, Anal. Methods Environ. Chem. J., 2021, 4(3), 21–32 CrossRef CAS.
- A. A. Adepoju-Bello, S. A. Osagiede and O. O. Oguntibeju, J. Bioanal. Biomed., 2012, 4(5), 092–095 CAS.
- J. Deng, X. Kang, L. Chen, Y. Wang, Z. Gu and Z. Lu, J. Hazard. Mater., 2011, 196, 187–193 CrossRef CAS PubMed.
|
This journal is © The Royal Society of Chemistry 2023 |
Click here to see how this site uses Cookies. View our privacy policy here.