DOI:
10.1039/D2RA07919D
(Paper)
RSC Adv., 2023,
13, 3329-3332
Boron-catalysed transition-metal-free arylation and alkenylation of allylic alcohols with boronic acids†
Received
12th December 2022
, Accepted 15th January 2023
First published on 23rd January 2023
Abstract
The development of efficient catalytic reactions with excellent atom and step economy employing sustainable catalysts is highly sought-after in chemical synthesis to reduce the negative effects on the environment. The most commonly-used strategy to construct allylic compounds relies on the transition-metal-catalysed nucleophilic substitution reaction of allylic alcohol derivatives. These syntheses exhibit good yield and selectivity, albeit at the expense of toxic and expensive catalysts and extra steps. In this paper, we report a transition-metal-free arylation and alkenylation reaction between unprotected allylic alcohols and boronic acids. The reactions were performed with B(C6F5)3 as the catalyst in toluene, and corresponding products were obtained in 23–92% yields. The reaction has mild conditions, scalability, excellent atom and step economy.
Allylic alkylation and alkenylation of allylic reagents such as allylic halides, carboxylates, carbonates, phosphates, and related compounds are some of the most important textbook reactions for carbon–carbon bond-forming, and have been widely applied in the syntheses of a broad scope of complex molecules.1 Among these dozens of building blocks, the direct transformation of allylic alcohols would be highly beneficial from the viewpoint of sustainable chemistry:1h,2 (a) the substrate could be used without the pre-activation of the hydroxyl group; (b) avoidance of other undesired by-products other than water. Regarding the other reaction partner, boronic acids3 are a promising choice compared with halides, grignard reagents and other feedstocks due to their stability, operational convenience, negligible toxicity, and broad functional group compatibility. A number of transition-metal-catalysed cross-coupling reactions between allylic alcohols and boronic acids have been established based on π-allyl metal complexes with outstanding efficacy and selectivity in a long-range.4 However, these cases bear the intrinsic limitations of the transition-metal-catalysts, which are widely regarded as expensive, hard to prepare, oxygen and moisture sensitive. As an alternative, several Brønsted and Lewis acids catalysed substitution reaction of allylic alcohols with different nucleophiles have been explored as the environmental benign approaches to obtain allylic compounds.5 In 2015, Gandon6 group reported a alkenylation of alcohols with vinylboronic acids employing air stable calcium(II) complex as the catalyst. Despite of these progresses, the green and sustainable catalytic arylation protocol between alcohols and boronic acids remain underdeveloped. In the last decade, B(C6F5)3 has been realized to be capable of initiating a wide range of chemical transformations with remarkable performance.7 In particular, the cross-coupling reaction of alcohols to form C–C and C–P bond have been achieved with B(C6F5)3 by Xie8 et al. very recently. Herein, as our longstanding interest in the development of environmental-friendly chemical transformations, we envisaged to explore the efficacy of B(C6F5)3 in the arylation and alkenylation reaction of allylic alcohols (Scheme 1).
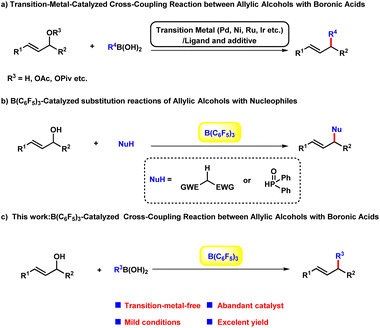 |
| Scheme 1 Catalytic cross-coupling reaction between boronic acids and allylic alcohol derivatives. | |
Initially, the (E)-1,3-diphenylprop-2-en-1-ol 1a and 4-methoxyphenylboronic acid 2a were chosen as the model substrates to optimize the reaction conditions. The reaction of 1a and 2a was carried out in the presence of B(C6F5)3 (20 mol%) at 80 °C in MeCN, affording compound 3a in 57% yield (Table 1, entry 1). Delighted by this initial result, we investigated the reaction conditions comprehensively. The screening of the solvent indicated that the yield could be promoted to 71% in nonpolar methylbenzene (Table 1, entry 2–7). Lewis acid catalysts such as Cu(OTf)2, Zn(OTf)2, and Sc(OTf)2 were evaluated to offer the desired product only in moderate yield (Table 1, entries 8–10). Moreover, other boron containing catalysts including B(OH)3 and BF3·OEt2 only offered diminished yields as low as 32% (Table 1, entries 11–12). In consideration of that the unstability of alcohols in presence of the strong Lewis acid catalyst, we increased 1a to 1.2 equivalent and more, up to 96% yield was obtained with 2.0 equivalent of 1a (Table 1, entries 13–15). The desired product could also be obtained in 93% yield with a reduced amount of B(C6F5)3 of 10% (Table 1, entries 16–17). Then, we conducted the reaction at lower temperature, and the results indicated that the reaction performed smoothly at ambient temperature giving 91% yield (Table 1, entries 18–19).
Table 1 The optimization of the conditionsa
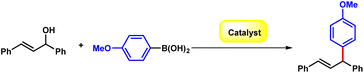
|
Entry |
Catalyst |
Solvent |
Temperature (°C) |
Yieldb |
Reaction conditions: 1a (0.1 mmol), 2a (0.2 mmol), catalyst (20 mol%), solvent (2 mL), 6 hours, under air atmosphere. Isolated yield. 1.2 equiv. of 1a was added. 1.5 equiv. of 1a was added. 2.0 equiv. of 1a was added. 10 mol% of B(C6F5)3 was used. 5 mol% of B(C6F5)3 was used. The reaction time is 36 hours. |
1 |
B(C6F5)3 |
CH3CN |
80 |
57% |
2 |
B(C6F5)3 |
DMF |
80 |
38% |
3 |
B(C6F5)3 |
DMSO |
80 |
25% |
4 |
B(C6F5)3 |
THF |
80 |
18% |
5 |
B(C6F5)3 |
DCE |
80 |
62% |
6 |
B(C6F5)3 |
EA |
80 |
12% |
7 |
B(C6F5)3 |
Toluene |
80 |
71% |
8 |
Cu(OTf)2 |
Toluene |
80 |
55% |
9 |
Zn(OTf)2 |
Toluene |
80 |
52% |
10 |
Sc(OTf)2 |
Toluene |
80 |
63% |
11 |
B(OH)3 |
Toluene |
80 |
36% |
12 |
BF3·OEt2 |
Toluene |
80 |
32% |
13c |
B(C6F5)3 |
Toluene |
80 |
88% |
14d |
B(C6F5)3 |
Toluene |
80 |
91% |
15e |
B(C6F5)3 |
Toluene |
80 |
96% |
16f |
B(C6F5)3 |
Toluene |
80 |
93% |
17g |
B(C6F5)3 |
Toluene |
80 |
85% |
18 |
B(C6F5)3 |
Toluene |
50 |
90% |
19h |
B(C6F5)3 |
Toluene |
25 |
91% |
With improved reaction conditions in hand, the scope of the boron-catalysed cross coupling reaction was explored between a set of di-aryl allylic alcohols and boronic acids. At first, the substituent effects on the benzene ring of 1 was evaluated. Delightfully, both electric-donating and -withdrawing groups were well tolerated with the conditions. When methyl substituted allylic alcohol on the para-position was tested, 58% yield was obtained (3b). Products with halogen groups such as fluorine, chlorine and bromine were also generated in the yields up to 90% (3c–3e). Methyl, methoxyl and chlorine groups on meta and ortho positions of the benzene ring were also appropriate under standard conditions offering 3f to 3i in the yields of 23–78%. When the benzene ring was replaced with 2-naphthalene, the corresponding product was prepared in 45% yield (3j). Next, the scope of boronic acids were also explored. Apart from 2a, phenylboronic acid bearing a series of electron-rich groups such as alkoxyl, methylthio and dimethylamino groups were compatible with this protocol affording corresponding products 3k–3o in the yields of 45–90%. Further evaluation employing alkyl substituent allylic alcohol such as (E)pent-3-en-2-ol failed to yield the desired product. Later, several aryboronic acids including (4-methoxynaphthalen-1-yl) boronic acid, indole-5-boronic acid, benzofuran-3-boronic acid and benzofuran-2-boronic acid provided the desired products 3p–3s in 58–92% yields. Moreover, alkenyl boric acid was also evaluated offering 3t in the yield of 84%. It is always challenging to control the regio-selectivity of the nucleophilic substitution for unsymmetrical allylic alcohols.9 When electron deficient boronic acids such as (perfluorophenyl)boronic acid and pyridine heterocycle boronic acid were tested under the optimized conditions, the reaction did not occur with the boronic acids recovered. The regio-selectivity of this kind of reaction is later, to illustrate the regio-selectivity of the protocol further, the unsymmetrical allylic alcohol 1u was loaded to the standard conditions, and 3u was obtained as the only products in 85% yield, which emphasize the excellent selectivity of the reaction (Table 2).
Table 2 Scope of the reactiona
Reaction conditions: 1 (0.1 mmol), 2 (0.2 mmol), B(C6F5)3 (10 mol%), toluene (2 mL), 36 hours, under air atmosphere. |
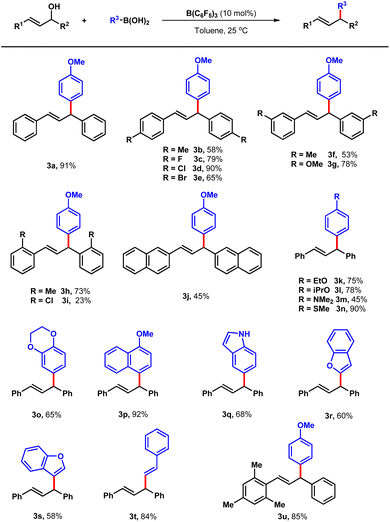 |
Based on previous reports,8 the reaction mechanism was proposed as shown in Scheme 2. At first, the coordination of the B(C6F5)3 catalyst with the alcohol generated the intermediate a (Int a), which tend to offer the carbocation intermediate b (Int b) after the cleavage of C–O bond. Later, the migration of R3 to C1 or C3 position occurred to generate the desired product 3 and boric acid with the B(C6F5)3 catalyst recovered. The selectivity between the C1 or C3 arylation mainly depends on the steric effect of R1 and R2.
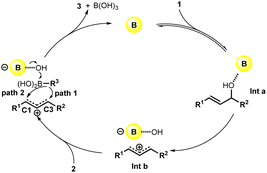 |
| Scheme 2 Proposed reaction pathway. | |
Conclusions
In conclusion, we have established an efficient and convenient cross-coupling reaction between allylic alcohols and boronic acids under transition-metal-free conditions. This boronic-catalysed protocol exhibited excellent selectivity, mild conditions and broad scope.
Conflicts of interest
There are no conflicts to declare.
Acknowledgements
Generous financial support from the National Natural Science Foundation of China (21702235, 82141001), the Fundamental Research Funds for the Central Public Welfare Research Institutes (ZZ13-YQ-098, ZZ14-FL-010).
Notes and references
-
(a) Y. L. Lai and J. M. Huang, Org. Lett., 2017, 19, 2022–2025 CrossRef CAS PubMed;
(b) J. A. Marshall, Chem. Rev., 2000, 100, 3163–3186 CrossRef CAS PubMed;
(c) K. Aikawa, K. Ishii, Y. Endo and K. Mikami, J. Fluorine Chem., 2017, 203, 122–129 CrossRef CAS;
(d) H. Chen, X. Jia, Y. Yu, Q. Qian and H. Gong, Angew. Chem., Int. Ed., 2017, 56, 13103–13106 CrossRef CAS PubMed;
(e) Y. Shi, H. Wu and G. Huang, Org. Chem. Front., 2021, 8, 3320–3331 RSC;
(f) N. Kranidiotis-Hisatomi and M. Oestreich, Org. Lett., 2022, 24, 4987–4991 CrossRef CAS PubMed;
(g) W. B. Xu, M. Sun, M. Shu and C. Li, J. Am. Chem. Soc., 2021, 143, 8255–8260 CrossRef CAS PubMed;
(h) S. Tang, P. Zhang, C. Wang, Y. Shao and J. Sun, Chem. Commun., 2021, 57, 11080–11083 RSC;
(i) T. Shinozawa, S. Terasaki, S. Mizuno and M. Kawatsura, J. Org. Chem., 2016, 81, 5766–5774 CrossRef CAS PubMed;
(j) C. Chevrin, J. Le Bras, F. Hénin and J. Muzart, Tetrahedron Lett., 2003, 44, 8099–8102 CrossRef CAS.
-
(a) P. Trillo, A. Baeza and C. Najera, J. Org. Chem., 2012, 77, 7344–7354 CrossRef CAS PubMed;
(b) C. Y. Meng, X. Liang, K. Wei and Y. R. Yang, Org. Lett., 2019, 21, 840–843 CrossRef CAS PubMed;
(c) B. Yang and Z. X. Wang, J. Org. Chem., 2020, 85, 4772–4784 CrossRef CAS PubMed;
(d) P. Xie, Z. Sun, S. Li, L. Zhang, X. Cai, W. Fu, X. Yang, Y. Liu, X. Wo and T. P. Loh, Org. Lett., 2020, 22, 1599–1604 CrossRef CAS PubMed;
(e) S. B. Tang, X. Zhang, H. F. Tu and S. L. You, J. Am. Chem. Soc., 2018, 140, 7737–7742 CrossRef CAS PubMed;
(f) Y.-C. T. Shyh-Chyun Yang and Y.-J. Shue, Organometallics, 2001, 20, 5326–5330 CrossRef;
(g) F. P. Wu, J. B. Peng, L. Y. Fu, X. Qi and X. F. Wu, Org. Lett., 2017, 19, 5474–5477 CrossRef CAS PubMed;
(h) W. Ahmed, S. Zhang, X. Yu, X. Feng, Y. Yamamoto and M. Bao, Angew. Chem., Int. Ed., 2019, 58, 2495–2499 CrossRef CAS PubMed;
(i) R. Xu, K. Li, J. Wang, J. Lu, L. Pan, X. Zeng and G. Zhong, Chem. Commun., 2020, 56, 8404–8407 RSC;
(j) J. S. Foot, H. Kanno, G. M. P. Giblin and R. J. K. Taylor, Synlett, 2002, 1293–1295 CAS;
(k) P. Trillo, A. Baeza and C. Nájera, ChemCatChem, 2013, 5, 1538–1542 CrossRef CAS;
(l) P. Trillo and I. M. Pastor, Adv. Synth. Catal., 2016, 358, 2929–2939 CrossRef CAS;
(m) B. G. Das, R. Nallagonda and P. Ghorai, J. Org. Chem., 2012, 77, 5577–5583 CrossRef CAS PubMed;
(n) A. Ohtsuki, S. Aoki, R. Nishida, S. Morita, T. Fujii and K. Okumura, Eur. J. Org.
Chem., 2020, 2020, 4309–4318 CrossRef CAS.
-
(a) S. Roscales and A. G. Csaky, Chem. Soc. Rev., 2020, 49, 5159–5177 RSC;
(b) H. Xu, M. Ye, K. Yang and Q. Song, Org. Lett., 2021, 23, 7776–7780 CrossRef CAS PubMed;
(c) L. Bering and A. P. Antonchick, Org. Lett., 2015, 17, 3134–3137 CrossRef CAS PubMed;
(d) M. Raducan, R. Alam and K. J. Szabo, Angew. Chem., Int. Ed., 2012, 51, 13050–13053 CrossRef CAS PubMed;
(e) X.-D. Li, L.-J. Xie, D.-L. Kong, L. Liu and L. Cheng, Tetrahedron, 2016, 72, 1873–1880 CrossRef CAS.
-
(a) L. Mao, K. J. Szabo and T. B. Marder, Org. Lett., 2017, 19, 1204–1207 CrossRef CAS PubMed;
(b) C. P. Donald, A. Boylan, T. N. Nguyen, P. A. Chen and J. A. May, Org. Lett., 2022, 24, 6767–6771 CrossRef CAS PubMed;
(c) N. J. Green, A. C. Willis and M. S. Sherburn, Angew. Chem., Int. Ed., 2016, 55, 9244–9248 CrossRef CAS PubMed;
(d) J. Ye, J. Zhao, J. Xu, Y. Mao and Y. J. Zhang, Chem. Commun., 2013, 49, 9761–9763 RSC;
(e) G. Wang, Y. Gan and Y. Liu, Chin. J. Chem., 2018, 36, 916–920 CrossRef CAS;
(f) V. Poláčková, Š. Toma and C. Oliver Kappe, Tetrahedron, 2007, 63, 8742–8745 CrossRef;
(g) G. W. Kabalka, G. Dong and B. Venkataiah, Org. Lett., 2003, 5, 893–895 CrossRef CAS PubMed;
(h) H. Tsukamoto, T. Uchiyama, T. Suzuki and Y. Kondo, Org. Biomol. Chem., 2008, 6, 3005–3013 RSC.
-
(a) H. S. Rao and A. V. Rao, Beilstein J. Org. Chem., 2016, 12, 496–504 CrossRef CAS PubMed;
(b) G.-P. Fan, Z. Liu and G.-W. Wang, Green Chem., 2013, 15 Search PubMed;
(c) M. Zhuang and H. Du, Org. Biomol. Chem., 2014, 12, 4590–4593 RSC;
(d) J. L. Bras and J. Muzart, Tetrahedron, 2007, 63, 7942–7948 CrossRef;
(e) W. Wu, W. Rao, Y. Q. Er, J. K. Loh, C. Y. Poh and P. W. H. Chan, Tetrahedron Lett., 2008, 49, 2620–2624 CrossRef CAS;
(f) M. Rueping, U. Uria, M. Y. Lin and I. Atodiresei, J. Am. Chem. Soc., 2011, 133, 3732–3735 CrossRef CAS PubMed;
(g) R. Sanz, A. Martínez, D. Miguel, J. M. Álvarez-Gutiérrez and F. Rodríguez, Adv. Synth. Catal., 2006, 348, 1841–1845 CrossRef CAS.
- D. Leboeuf, M. Presset, B. Michelet, C. Bour, S. Bezzenine-Lafollee and V. Gandon, Chem.–Eur. J., 2015, 21, 11001–11005 CrossRef CAS PubMed.
-
(a) G. Kumar, S. Roy and I. Chatterjee, Org. Biomol. Chem., 2021, 19, 1230–1267 RSC;
(b) J. N. Bentley and C. B. Caputo, Organometallics, 2018, 37, 3654–3658 CrossRef CAS;
(c) W. Li and T. Werner, Org. Lett., 2017, 19, 2568–2571 CrossRef CAS PubMed;
(d) Y. L. Li, J. Y. Pang, J. C. Lou, W. W. Sun, J. K. Liu and B. Wu, Asian J. Org. Chem., 2021, 10, 1424–1427 CrossRef CAS;
(e) K. Chulsky and R. Dobrovetsky, Angew. Chem., Int. Ed., 2017, 56, 4744–4748 CrossRef CAS PubMed;
(f) M. Oestreich, J. Hermeke and J. Mohr, Chem. Soc. Rev., 2015, 44, 2202–2220 RSC;
(g) H. Fang, G. Wang and M. Oestreich, Org. Chem. Front., 2021, 8, 3280–3285 RSC;
(h) T. Hackel and N. A. McGrath, Molecules, 2019, 24, 432 CrossRef PubMed;
(i) C.-C. Ma, X.-X. Zhu, L. Liu, J.-J. Dai, J. Xu and H.-J. Xu, Tetrahedron Lett., 2021, 82, 153387 CrossRef CAS.
- X. Yang, B. Li, H. Xing, J. Qiu, T.-P. Loh and P. Xie, Green Chem., 2021, 23, 1633–1637 RSC.
-
(a) G. Hirata, H. Satomura, H. Kumagae, A. Shimizu, G. Onodera and M. Kimura, Org. Lett., 2017, 19, 6148–6151 CrossRef CAS PubMed;
(b) S. Tang, Z. Li, Y. Shao and J. Sun, Org. Lett., 2019, 21, 7228–7232 CrossRef CAS PubMed.
|
This journal is © The Royal Society of Chemistry 2023 |
Click here to see how this site uses Cookies. View our privacy policy here.