DOI:
10.1039/D2RA08317E
(Paper)
RSC Adv., 2023,
13, 3715-3722
Thiourea fused γ-amino alcohol organocatalysts for asymmetric Mannich reaction of β-keto active methylene compounds with imines†
Received
30th December 2022
, Accepted 18th January 2023
First published on 26th January 2023
1. Introduction
The development of new optically active organocatalysts and their application in asymmetric synthesis has attracted considerable interest in the scientific community over the past 20 years.1 Excellent covalent and non-covalent organocatalysts have been developed and applied over a wide range of reactions.2 In recent years, we have also developed various β-amino alcohols X, which were utilized effectively as organocatalyst in different enantioselective reactions.3 Most recently, we have also reported the effectiveness of di-amino alcohol organocatalyst Y in aldol reactions.4 Based on these backgrounds, we have designed a new thiourea fused γ-amino alcohol organocatalyst Z (Scheme 1) that contains thiourea and hydroxy groups acting as hydrogen bonding sites, and a tert-amino group acting as a basic and steric influence site. Furthermore, diaryl groups may work as steric influence site for controlling the stereoselective reaction course. A large number of bifunctional tertiary amine-thioureas have emerged as efficient organocatalysts and were explored in a variety of asymmetric transformations.5 The key to the great success of these thiourea-based catalysts is the functionality of the thiourea moiety as a hydrogen bond donor, which is a general structural unit in the design of bifunctional catalysts. As a reaction for exploring the catalytic ability of catalysts X, Y and designed Z, we have selected the Mannich reaction of β-keto active methylene compounds with imines, which is a versatile reaction for affording chiral Mannich products W,β-amino keto carbonyl compounds containing continuous chiral quaternary carbon center, which are useful intermediates for synthesis of various biologically active compounds and pharmaceuticals.6 Although thioureas fused amine organocatalysts such as bifunctional catalysts V (ref. 6a), W (ref. 6f) and others are commonly used in this reaction, to the best of our knowledge, there have been no references using amino alcohol X, di amino alcohol Y and thiourea fused γ-amino alcohol Z organocatalysts deriving from various low-cost amino acids (Scheme 1). In particular, the newly designed trifunctional catalyst Z has a good tertiary basic site, two strong hydrogen bonding sites (thiourea and hydroxy groups), and steric control sites (diphenyl groups at α-position, tertiary amino group at γ-position and aryl group on thiourea group) in its molecule, as well as molecular flexibility according to substrate structure. Therefore, it is expected to activate substrates strongly and to be able to coordinate two substrates to one catalyst, effectively controlling the enantioselective reaction direction and giving Mannich products W with good chemical yields and stereoselectivities.
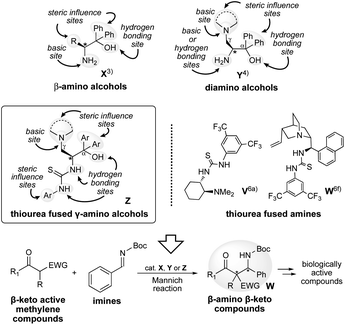 |
| Scheme 1 Plan of catalyst design. | |
We herein describe the functionality of amino alcohol X, di-amino alcohol Y, and newly designed thiourea fused γ-amino alcohols Z in the asymmetric Mannich reaction of β-keto active methylene compounds with imines. In this study, catalyst Z showed superior activity and the desired Mannich products W were obtained in satisfactory chemical yields and stereoselectivities (up to 88%, up to syn
:
anti/93
:
7 dr, up to 99% ee).
2. Results and discussion
2.1. Synthesis of organocatalysts
Amino alcohols X1–9 (ref. 3e) and Y1–5 (ref. 4) were prepared according to our previous reported methods3e,4 (Scheme 2). Thiourea fused γ-amino alcohols Z1–9 were derived from L-serine 1 based on the synthetic route of di-amino alcohols Y (Scheme 2). The deprotections of 6a–e afforded the corresponding di-amino alcohols Y1–5 in good yields, followed by the reactions of the obtained Y1–5 with 3,5-di(trifluoromethyl)phenyl isocyanate afforded the desired corresponding catalyst Z1–5 having different tert-amino groups at β-position, respectively. Thiourea fused catalysts Z6–9 with different di-aryl groups at α-position were also prepared by the reactions of 5b–e with pyrrolidine, the deprotections of the obtained 6f–i, followed by the reaction of 7b–e with isothiocyanate, respectively. Furthermore, catalysts Z10–15 having different thiourea groups were also prepared by the reactions of Y3 with isothiocyanates at good yields. Also, catalyst Z16 (ref. 7) with no amino group at γ-position was prepared by the reaction of X4 with isothiocyanates for the confirmation of the functionality of amino group as a base. In addition, amino silyl ether Z17 that masked the hydroxyl group was also prepared by the reaction of Z3 with TMSCl (Scheme 2) for the confirmation of the functionality of hydroxy group as a hydrogen bonding site. Furthermore, Z18 (ref. 8) were prepared according to previous reported method.8
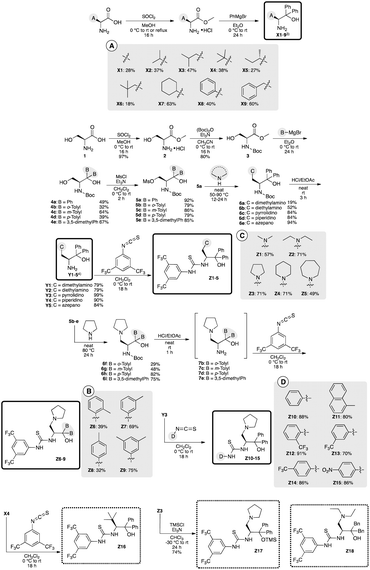 |
| Scheme 2 Preparation of organocatalysts. | |
2.2. Catalytic efficiency in Mannich reaction
We examined the catalytic activity of the prepared organocatalysts X1–9, Y1–5, and Z1–19 in the asymmetric Mannich reactions of β-keto ester 10a with imine 11a. First, the catalytic activity of β-amino alcohols X1–9 and di-amino alcohols Y1–5 were examined, respectively (Table 1). The reaction of 10a with 11a (ref. 9) was carried out in the presence of 15 mol% of catalysts in toluene at −60 °C for 14 h. Although every catalyst showed catalytic activities and afforded the Mannich product 12 (12a-1, 12b-1) in moderate to good chemical yields, good to excellent diastereoselectivities, enough enantioselectivity were not obtained in this reaction condition (entries 1–9). In the uses of every X1–9, only β-amino alcohol catalyst X4 with tert-butyl group at β-position afforded 12a-1 as a major product in moderate enantioselectivity (62% ee) with good chemical yield and diastereoselectivity (entry 4). On the other hand, all di-amino alcohol catalysts Y1–5 did not show satisfactory catalytic activity similarly to X (entries 10–14). Secondly, newly synthesized thiourea fused γ-amino alcohols Z1–19 were examined in the same reaction condition of the case of catalysts X and Y (10a: 1.2 eq., solvent: toluene, catalyst: 15 mol%, reaction temperature: −60 °C) (Table 2). First, catalytic activity of catalysts Z1–5 with acyclic or cyclic amino substituents at γ-position and diphenyl groups at α-position were examined (entries 1–5). Every catalyst showed good catalytic activities and afforded the chiral Mannich product 12′-1 (ref. 6a) as a major product. Especially, catalyst Z3 with pyrrolidine ring at γ-position afforded totally good results for chemical yield, diastereoselectivity and enantioselectivity (74%, 12a′-1/12b-1: 83/17 dr, 97% ee) (entry 3). Next, catalysts Z6–9 with pyrrolidine ring at β-position and several substituted diphenyl groups at β-position were applied in this reaction (entries 6–9). Catalysts Z6–9 also showed good catalytic activity to afford 12b as a major product similarly to Z1–5. Furthermore, the catalytic activity of catalysts Z10–15 with pyrrolidine ring, diphenyl groups and several thiourea groups at β-position also screened (entries 10–15) and these catalysts also showed good catalytic activity, but enantioselectivity widely varied by the substitution of thiourea group having aryl group on nitrogen atom. From the result, it was appeared that catalyst Z3 with pyrrolidine ring at β-position and diphenyl groups at α-position and thiourea group having di-trifluoromethyl phenyl group on nitrogen atom works as best catalyst in this reaction (entry 3). In order to predict the functionalities of tert-amino group at γ-position acting as basic site and hydroxy group at α-position acting as hydrogen bonding site of catalysts, the reactions using catalysts Z16 with no amino group and Z17 that protected hydroxy group by TMS group were attempt (entries 16 and 17). The use of Z16 was significantly decreased chemical yield and stereoselectivity (entry 16). From this fact, it is indicated that tert-amino group acts as basic site for proceeding the reaction. On the other hand, Z17 showed satisfactory catalytic activity for proceeding the reaction, but diastereoselectivity and enantioselectivity were decreased (entry 17). It may be indicated that hydroxy group at α-position may participate to the control of stereoselectivity as hydrogen bonding site in the reaction. The known catalyst Z18 also showed good catalytic activity similar to Z3, but caused a significant decrease in chemical yield (entry 18).
Table 1 Mannich reaction of β-keto ester 10a with imino ester 11a using catalysts X1–9, Y1–5
Table 2 Mannich reaction of β-keto ester 10a with imino ester 11a using catalysts Z1–19
With these results in hand, we tried to optimize the reaction conditions using superior catalyst Z3 improving the chemical yield and stereoselectivities (Table 3). An extensive screening of the reaction were further carried out by varying different parameters such as solvent, catalyst and substrate loading, and reaction temperature. First, the reaction of three kinds of β-keto esters 10a–c (ref. 10) with imine 11a were examined in toluene using 15 mol% of superior catalyst Z3 under −60 °C at 14 h (entries 1–3). As a result, the use of β-keto ester 10a with ethoxy carbonyl group afforded 12a′-1 in the best results for chemical yield and stereoselectivities (83%, syn
:
anti/83
:
17 dr, 97% ee) (entry 1). The differences of chemical yields and stereoselectivities might be due to the steric interaction between catalyst and of the interaction between catalyst and substrates with the different alkyl groups on carboxyl group of 10a–c in the corresponding transition states, respectively. Next, the reaction temperature (25, −25 and −78 °C) were also examined in the presence of 15 mol% of catalyst Z3 in toluene for 14 h (entries 4–6). Although the reactions under these temperatures (entries 4–6) afforded 12a′-1 in good chemical yield and stereoselectivities, −60 °C gave better overall results with respect to chemical yield and stereoselectivities (entry 1). Although it is not clear, the thermal energy imparted by the temperature change might have changed the state of the catalyst–substrate interaction in the transition state. Moreover, the ratio of substrate used among 10a and 11a (entries 7 and 8) was also examined and both equivalents are effective to afford the product 12a′-1 in satisfactory chemical yield and stereoselectivities. However, the use of 1.2 equivalent of 10a was totally effective (entry 1). We examined the effect of catalyst loading by varying from 20, 10, 5 mol%, 2.5 and 1 mol% under superior reaction condition (entries 9–13). Good result (81%, syn
:
anti/84
:
16 dr, 98% ee) was obtained in the presence of 20 mol% of catalyst Z3 (entry 9). The use of 10 mol% of Z3 also afforded good chemical yield and stereoselectivities similar to the use of 20 mol% of Z3 (entry 10). When the reaction was carried out in the presence of 5 mol% of Z3, chemical yield and diastereoselectivity were maintained, but enantioselectivity was slightly decreased to 87% ee (entries 11). Furthermore, the uses of both 2.5 mol% and 1 mol% of Z3 brought about the small decrease of chemical yields and stereoselectivities (2.5 mol%
:
72%, syn
:
anti/78
:
22 dr, 81% ee, 1 mol%
:
72%, syn
:
anti/72
:
28 dr, 75% ee) (entries 12 and 13). These results indicate that overall the use of 15 mol% of Z3 was favourable (entry 1). Solvent screening was performed with ethereal (entry 14), non-polar aliphatic (entry 15), chlorinated (entries 16 and 17), protic and aprotic polar (entries 18 and 19) solvents. Catalyst Z3 showed catalytic activity in all solvents to afford the Mannich product 12a′-1. However, the uses of protic polar EtOH and aprotic polar DMF brought about the decrease in enantioselectivity (entries 18 and 19). From these results, toluene was observed to be superior solvent to this reaction using catalyst Z3. The reactions at both 1 h and 3 h brought about decrease in chemical yields, although stereoselectivity were kept to good values (entries 20 and 21). On the other hand, the catalytic activity of catalyst Z3 did not change, when this reaction was carried out at 7 h (entry 22). From these screening, catalyst Z3 showed best catalytic activity when the reaction was carried out using β-keto ester 10a and imine 11a under the reaction condition (10a: 1.2 eq., solvent: toluene, catalyst: 15 mol%, reaction temperature: −60 °C) as shown at entry 1.
Table 3 Optimization of the reaction conditions using catalyst Z3
2.3. Substrate scope
After optimization of the reaction conditions, we examined the generality of superior catalyst Z3 in the reactions of β-keto active methylene compounds 10a,d–h with imines 11a,b, respectively (Table 4). The reaction of cyclohexyl 10d with 11a afforded Mannich product 13 (ref. 6i) in moderate chemical yield (37%) and good diastereoselectivity (syn
:
anti/93
:
7 dr), although enantioselectivity was low (22% ee (syn)). Similarly, this reaction using heterocyclic β-keto ester 10e with 11a also afforded the corresponding Mannich product 14 in moderate chemical yield (42%) and good stereoselectivities (syn
:
anti/85
:
15 dr, 71% ee (syn)). It might be for the steric influence of ring size of cyclohexyl group (entry 1) in β-keto ester 10d and for the electronic influence of oxygen atom in hydrofuran ring of β-keto ketone 10e (entry 2). They might have caused a change of the interaction between catalyst and substrates in the transition state and affected the chemical yield and stereoselectivity, although it is not clear. In addition, the reaction using β-keto nitrile 10f11 with 11a also smoothly proceed and afforded 15 in satisfactory chemical yield and stereoselectivities (79%, syn
:
anti/80
:
20 dr, 85% ee (syn)). Furthermore, the use of dicyclic 10g (ref. 12) with 11a also afforded 16 in satisfactory chemical yield and enantioselectivity (87%, 99% ee), although diastereoselectivity was low (syn
:
anti/55
:
45 dr). The uses of acyclic 10h (ref. 6i) and 11a also afforded 17 (ref. 6i) in satisfactory chemical yield and enantioselectivity, although diastereoselectivity was low (74%, syn
:
anti/57
:
43 dr, 91% ee (syn)). Moreover, reaction of 10a with thiophene based imine 11b (ref. 13) also afforded 18 in moderate chemical yield and good stereoselectivities (61%, syn
:
anti/91
:
9 dr, 94% ee (syn)) from these results, it was indicated that catalyst Z3 is effective for a variety of substrates.
Table 4 Substrate scope using catalyst Z3
2.4. Reaction mechanism
The use of catalyst Z3 afforded Mannich product 12a′-1 with best chemical yield and stereoselectivities in the reaction of 10a and 11a (Tables 2 and 3). Based on both the good enantioselectivity (97% ee) of the obtained chiral Mannich product [2S,3R]-12a′-1 and the results of its calculation studies (Fig. 1 and 2), an enantioselective reaction course is proposed as follows (Scheme 3). Thus, the stable conformations of both β-keto ester 10a and imine 11a were indicated by the scan of total energy and the DFT optimization calculations of structures (Fig. 1).
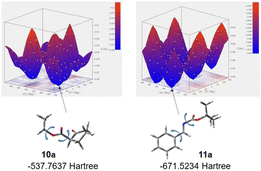 |
| Fig. 1 Scan of total energies (up) and DFT optimized structures (down, at the B3LYP/6-31G(d) level of theory) of compounds 10a and 11a generated by varying the torsion angles (the dihedral scans showed with u-shaped arrows). | |
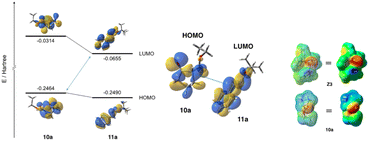 |
| Fig. 2 Frontier orbital calculation and electrostatic potential calculation. | |
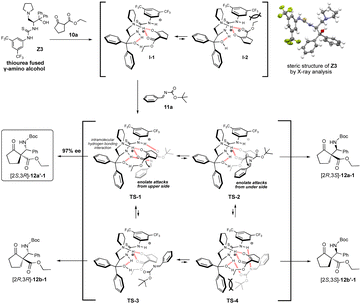 |
| Scheme 3 Plausible reaction course. | |
Furthermore, the conformation of catalyst Z3 was also estimated by X-ray analysis (Scheme 3), the scan of total energy and the DFT optimization calculations (Fig. 2). Moreover, the regioselectivity of the interaction between 10a and 11a was estimated by the calculations of the energies and coefficients of their frontier orbitals (Fig. 2). The energy levels of the orbitals calculation clearly showed the interaction between the LUMO of 10a and the HOMO of 11a, and their orbital phase clearly demonstrated a matching in favor of overlapping to afford the observed configuration of major Mannich product 12a′-1 (Fig. 2). In addition, the direction of coordination of Z3 and imine 11a was indicated by the electrostatic potential calculation (Fig. 2). Based on these results, firstly, catalyst Z3 acts as a base to 10a and generate an enolate species of 10a and then the species is fixed with ammonium site of catalyst by two points of hydrogen bonding interactions to form intermediates I-1 or I-2. In I-1 or I-2, I-1 has less steric interaction of between catalyst and enolate species than that of intermediate I-2 that has high steric interaction of between di-trifluorophenyl group on catalyst species and enolate (Scheme 3). Then, after adding imino ester 11a might be proceed through any proposed Ts-1-4 in which the amino hydrogen atom on thiourea part fixes by the intramolecular hydrogen bonding interaction with hydroxy group at α-position on the ammonium catalyst species. In the proposed Ts-1-4, the reaction might be proceeded through Ts-1 that has smaller steric interactions between substrates 10a, 11a and the ammonium catalyst species than those of Ts-2-4 that have a larger steric interaction between substrates 10a, 11a and the ammonium catalyst species. In this reaction, thiourea fused catalyst Z3 showed a great difference of the catalytic activities compared to amino alcohol catalyst X4, namely, the reaction using catalyst Z3 afforded the chiral Mannich product [2S,3R]-12a′-1 in excellent enantioselectivity (97% ee, entry 1, Table 2 and entry 1, Table 3) and the use of catalyst X4 afforded enantiomeric [2S,3R]-12a-1 (62% ee, entry 9, Table 1). It might be reasons of that Z3 could highly immobilize both substrates 10a and 11a and for the steric influences of substituents on catalyst Z3 (Scheme 4). Catalyst Z3 can immobilize two substrates and may be able to control the direction of attack of 16a to a high degree of steric control through the influence of substituent to afford 12a′-1. On the other hand, catalyst X4 can immobilize only substrate 10a and may not be sufficiently influenced by substituents to provide an enough degree of steric control over the direction of attack of 11a to afford 12b-1.
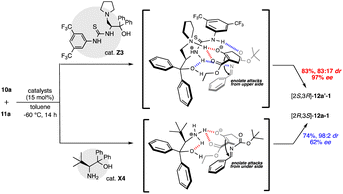 |
| Scheme 4 Differences of enantioselectivities by catalysts Z3 and X4. | |
3. Conclusions
A new thiourea fused γ-amino alcohol organocatalysts Z were explored based on a new catalyst design concept and their catalytic activity were examined in asymmetric Mannich reaction of various β-keto active methylene compounds with imines. In the catalyst Z, Z3 showed best catalytic performance and afforded the corresponding chiral Mannich products with good to excellent chemical yields, diastereoselectivities and enantioselectivities (up to 88%, up to syn
:
anti/93
:
7, up to 99% ee). Furthermore, the plausible stereoselective reaction course of Mannich reaction was predicted with the support of Frontier orbital and DFT calculations. The γ-amino alcohol organocatalysts Z designs modifications, further substrate scope of catalyst Z and the detailed mechanistic studies are in progress.
4. Experimental
4.1. General information
All reagents and dry solvents were purchased from commercial vendors and used directly without further purification. All reactions were placed in dried sample vials inserted with magnetic beads. 1H and 13C NMR spectroscopic data were recorded using a JEOL JNM-ECA500 instrument with tetramethylsilane as the internal standard. Thin-layer chromatography (TLC) was performed on Merck silica gel 60 F254 plates and the analytes were identified under UV light. Flash column chromatography was performed using silica gel pore size 60 N (40–100 μm). Infrared (IR) spectra were measured with a JASCO FT/IR-4100 spectrophotometer. HPLC data were collected using the TOSOH instrument equipped with (UV-8020, DP-8020, and SD-8022) detectors using Daicel CHIRALPAK AD-H OD-H column, CHIRALPAK IC column. Optical rotations were measured with a JASCO-DIP-370 digital polarimeter. MS were taken on a JEOL-JMS-700 V spectrometers. The data for the X-ray crystallographic structure of Z3 is available free of charge from the Cambridge Crystallographic Data Centre under accession number CCDC 2179025.
4.2. General procedure for the catalytic asymmetric Mannich reaction of β-keto carbonyl compounds 10a,d–h with NBoc imines 11a,b
To a solution of the corresponding catalyst (15 mol%) in solvent (1.0 mL) were added NBoc imine (0.10 mmol) and β-keto active methylene compounds (0.12 mmol) at −60 °C under an argon atmosphere and the solution was stirred at same temperature. After 14 h, the solvent was removed under reduced pressure and the residue was purified by preparative thin-layer chromatography. (n-hexane
:
EtOAc/25
:
1 to 8
:
1 to 6
:
1) to give the chiral Mannich products 12–18. Compounds 12–18 are the known compounds and the structures were identified by spectral datas which were in good agreement with those reported. The enantiomeric ratio and diastereomeric ratio of the products were determined by using chiral HPLC analysis.6i
Conflicts of interest
There are no conflicts to declare.
Notes and references
-
(a) B. List, R. A. Lerner and C. F. Barbas III, J. Am. Chem. Soc., 2000, 122, 2395 CrossRef CAS;
(b) P. I. Dalko and L. Moisan, Angew. Chem., Int. Ed., 2001, 40, 3726 CrossRef CAS;
(c) C. Bolm and J. A. Gladysz, Chem. Rev., 2003, 103, 2761 CrossRef CAS;
(d) Z. Tang, F. Jiang, L. T. Yu, X. Cui, L. Z. Gong, A. Q. Mi, Y. Z. Jiang and Y. D. Wu, J. Am. Chem. Soc., 2003, 125, 5262 CrossRef CAS PubMed;
(e) Y. Chi and S. H. Gellman, Org. Lett., 2005, 7, 4253 CrossRef CAS PubMed;
(f) A. Lattanzi, Org. Lett., 2005, 7, 2579 CrossRef CAS PubMed;
(g) D. Enders, C. Grondal and R. M. Matthias, Angew. Chem., Int. Ed., 2007, 46, 1570 CrossRef CAS PubMed;
(h) D. W. C. MacMillan, Nature, 2008, 455, 304 CrossRef CAS PubMed;
(i) S. Bertelsen and K. A. Jorgensen, Chem. Soc. Rev., 2009, 38, 2178 RSC;
(j) S. Kobayashi, Y. Mori, J. S. Fossey and M. M. Salte, Chem. Rev., 2011, 111, 2626 CrossRef CAS PubMed;
(k) A. Moyano and R. Rios, Chem. Rev., 2011, 111, 4703 CrossRef CAS PubMed;
(l) P. Kasaplar, C. R. Escrich and M. A. Pericas, Org. Lett., 2013, 15, 3498 CrossRef CAS PubMed;
(m) D. L. SIlverio, S. Torker, T. Pilyugina, E. M. Vieria, M. L. Snapper, F. Haeffener and A. H. Hoyeda, Nature, 2013, 494, 7436 CrossRef PubMed;
(n) S. Saravanan, N. H. Khan, R. I. Kureshy, S. H. Abdi and H. C. Bajaj, ACS Catal., 2013, 3, 2873 CrossRef CAS;
(o) D. F. Chen, Z. Y. Han, X. L. Zhou and L. Z. Gong, Acc. Chem. Res., 2014, 47, 2365 CrossRef CAS PubMed;
(p) C. M. R. Volla, I. Atodiresei and M. Rueping, Chem. Rev., 2014, 114, 2390 CrossRef CAS PubMed;
(q) X. Fang and C. J. Wang, Chem. Commun., 2015, 51, 1185 RSC;
(r) L. Xu, J. Huang, Y. Liu, Y. Wang, B. Xu, K. Ding, Y. Ding, Q. Xu, L. Yu and Y. Fan, RSC Adv., 2015, 5, 42178 RSC;
(s) T. Sekikawa, T. Kitaguchi, H. Kitaura, T. Minami and Y. Hatanaka, Org. Lett., 2016, 18, 646 CrossRef CAS PubMed;
(t) U. Varal, M. Durmaz and A. Sirit, Org. Chem. Front., 2016, 3, 730 RSC;
(u) H. Zhang, M. Han, T. Chen, L. Xu and L. Yu, RSC Adv., 2017, 7, 48214 RSC;
(v) Y. Zheng, A. Wu, Y. Ke, H. Cao and L. Yu, Chin. Chem. Lett., 2019, 30, 937 CrossRef CAS;
(w) S. H. Xiang and B. Tan, Nat. Commun., 2020, 11, 3786 CrossRef CAS PubMed.
-
(a) S. Mukherjee, J. W. Yang, S. Hoffmann and B. List, Chem. Rev., 2007, 107, 5471 CrossRef CAS PubMed;
(b) W. Notz, F. Tanaka and C. F. Barbas III, Acc. Chem. Res., 2004, 37, 580 CrossRef CAS PubMed;
(c) S. L. Zhang, H. X. Xie, J. Zhu, H. Li, X. S. Zhang, J. Li and W. Wang, Nat. Commun., 2011, 211(2), 211–217 CrossRef PubMed;
(d) Y. Q. Zou, F. M. Hormann and T. Bach, Chem. Soc. Rev., 2018, 47, 278 RSC;
(e) X. Fang and C. J. Wang, Chem. Commun., 2015, 51, 1185 RSC;
(f) X. Yu and W. Wang, Chem.–Asian J., 2008, 3, 516 CrossRef CAS PubMed;
(g) H. Y. Bae, S. Some, J. Soh, Y. S. Lee and C. E. Song, Chem. Commun., 2011, 47, 9621 RSC.
-
(a) J. Kumagai, Y. Kohari, C. Seki, K. Uwai and Y. Okuyama, Heterocycles, 2014, 90, 1124 Search PubMed;
(b) Y. Kohari, Y. Okuyama, E. Kwon, T. Furuyama, N. Kobayashi, T. Otuki, J. Kumagai, C. Seki, K. Uwai, G. Dai, T. Iwasa and H. Nakano, J. Org. Chem., 2014, 79, 9500 CrossRef CAS PubMed;
(c) H. Nakano, I. A. Owolabi, M. Chennapuram, Y. Okuyama, E. Kwon, C. Seki, M. Tokiwa and M. Takeshita, Heterocycles, 2018, 97, 647 CrossRef CAS PubMed;
(d) D. Ganesan, M. Chennapuram, Z. Begum, C. Seki, K. Uwai, Y. Okuyama, E. Kwon, M. Tokiwa, M. Takeshita and H. Nakano, Heterocycles, 2019, 98, 1536 CrossRef CAS;
(e) P. Parasuraman, Z. Begum, M. Chennapuram, C. Seki, K. Uwai, Y. Okuyama, E. Kwon, M. Tokiwa, M. Takeshita and H. Nakano, RSC Adv., 2020, 10, 17486 RSC;
(f) Z. Begum, H. Sannabe, C. Seki, K. Uwai, Y. Okuyama, E. Kwon, M. Tokiwa, M. Takeshita and H. Nakano, RSC Adv., 2021, 11, 203 RSC;
(g) D. Ganesan, P. Parasuraman, Z. Begum, R. Thiyagarajan, C. Seki, K. Uwai, Y. Okuyama, E. Kwon, M. Tokiwa, M. Takeshita and H. Nakano, Heterocycles, 2022, 105, 369 CrossRef CAS PubMed;
(h) Z. Begum, C. Seki, K. Uwai, Y. Okuyama, E. Kwon, M. Tokiwa, M. Takeshita and H. Nakano, RSC Adv., 2023, 13, 888 RSC.
- U. V. Subba Reddy, M. Chennapuram, K. Seki, C. Seki, B. Anusha, E. Kwon, Y. Okuyama, K. Uwai, M. Tokiwa, M. Takeshita and H. Nakano, Eur. J. Org. Chem., 2017, 26, 3874 CrossRef.
-
(a) M. S. Taylor and E. N. Jacobsen, Angew. Chem., Int. Ed., 2006, 45, 1520 CrossRef CAS PubMed;
(b) E. A. Peterson and E. N. Jacobsen, Angew. Chem., Int. Ed., 2009, 48, 6328 CrossRef CAS PubMed;
(c) W.-Y. Siau and J. Wang, Catal. Sci. Technol., 2011, 1, 1298 RSC;
(d) O. V. Serdyuk, C. M. Heckel and S. B. Tsogoeva, Org. Biomol. Chem., 2013, 11, 7051 RSC;
(e) Y. Wang, H. Lu and P.-F. Xu, Acc. Chem. Res., 2015, 48, 1832 CrossRef CAS PubMed.
-
(a) X. Jiang, D. Fu, G. Zhang, Y. Cao, L. Liu, J. Song and R. Wang, Chem. Commun., 2010, 46, 4294–4296 RSC;
(b) Y. P. Lou, C. W. Zheng, R. M. Pan, Q. W. Jin, G. Zhao and Z. Li, Org. Lett., 2015, 17, 688 CrossRef CAS PubMed;
(c) Y. Yamada, H. Miyabe, Y. Yasui and Y. Takemoto, Synthesis, 2007, 16, 2571 Search PubMed;
(d) A. J. Neuvonen and P. M. Pihko, Org. Lett., 2014, 16, 5152 CrossRef CAS PubMed;
(e) M. Hatano and K. Ishihatra, Synthesis, 2010, 22, 3785 Search PubMed;
(f) A. L. Tillman, J. Ye and D. J. Dixon, Chem. Commun., 2006, 11, 1191 RSC;
(g) Y. K. Kang and D. Y. Kim, J. Org. Chem., 2009, 74, 5734 CrossRef CAS PubMed;
(h) M. Hatano, K. Moriyama, T. Maki and K. Ishihara, Angew. Chem., Int. Ed., 2010, 49, 3823 CrossRef CAS PubMed;
(i) M. Hatano, T. Horibe and K. Ishihara, J. Am. Chem. Soc., 2010, 132, 56–57 CrossRef CAS PubMed;
(j) Y. Hamashima, N. Sasamoto, D. Hotta, H. Somei, N. Umebayashi and M. Sodeoka, Angew. Chem., Int. Ed., 2005, 44, 1525 CrossRef CAS PubMed;
(k) O. Marianacci, G. Michelettin, L. Bernardi, F. Fini, M. Fochi, D. Pettersen, V. Sgarzani and A. Ricci, Chem.–Eur. J., 2007, 13, 8338 CrossRef CAS PubMed;
(l) S. Lou, P. Dai and S. E. Schaus, J. Org. Chem., 2007, 72, 9998 CrossRef CAS PubMed;
(m) T. B. Poulsen, C. Alemparte, S. Saaby, M. Bella and K. A. Jørgensen, Angew. Chem., Int. Ed., 2005, 44, 2896 CrossRef CAS PubMed;
(n) A. Ting, S. Lou and S. E. Schaus, Org. Lett., 2006, 10, 2003–2006 CrossRef PubMed;
(o) N. H. Nguyen, A. B. Hughes and B. E. Sleebs, Curr. Org. Chem., 2014, 18, 260 CrossRef CAS;
(p) J. Vesely and R. Rios, Chem. Soc. Rev., 2014, 43, 611 RSC.
- K. Moria, T. Yamauchia, J. Maddalunob, K. Nakanoa, Y. Ichikawaa and H. Kotsuki, Synlett, 2011, 14, 2080 Search PubMed.
- T. Tatsumi, T. Misaki and T. Sugimura, Chem.–Eur. J., 2015, 21, 18971 CrossRef CAS PubMed.
- R. Pedrosa, J. M. Andrés, D. P. Ávila, M. Ceballosa and R. Pindadoa, Green Chem., 2015, 17, 2217 RSC.
- X. Gu, Y. Zhang, Z. Xu and C. Che, Chem. Commun., 2014, 50, 7870 RSC.
-
(a) F. F. Fleming, V. A. Vu, B. C. Shook, M. Rahman and O. W. Steward, J. Org. Chem., 2007, 72, 1431 CrossRef CAS PubMed;
(b) Z. Jia, E. Gálvez, R. M. Sebastián, R. Pleixats, Á. Álvarez-Larena, E. Martin, A. Vallribera and A. Shafir, Angew. Chem., Int. Ed., 2014, 53, 1 CrossRef.
- Y. Duan, L. Cui, L. Zuo and C. Zhang, Chem.–Eur. J., 2015, 21, 13052 CrossRef CAS PubMed.
- A. G. Wenzel and E. N. Jacobsen, J. Am. Chem. Soc., 2002, 124, 12964 CrossRef CAS PubMed.
|
This journal is © The Royal Society of Chemistry 2023 |