DOI:
10.1039/D3RA00164D
(Paper)
RSC Adv., 2023,
13, 9347-9352
Homogeneous base catalyst with high activity and stability for synthesis of dimethyl carbonate by transesterification†
Received
9th January 2023
, Accepted 12th March 2023
First published on 21st March 2023
Abstract
In the synthesis of dimethyl carbonate (DMC) by transesterification, CH3ONa has been commonly applied as a homogeneous catalyst due to its high catalytic activity, but its stability is unsatisfactory. Here, by studying the influence of ionic liquid base strength on transesterification, we prepared an organic base catalyst, potassium imidazole (KIm), with high catalytic activity and stability, which solved the problem of catalyst deactivation in transesterification. The results showed that when KIm was used in the synthesis of DMC from propylene carbonate (PC) and methanol (MeOH), the chemical equilibrium could be reached within 3 minutes and the yield of DMC reached 73.03%, indicating that KIm performed better in transesterification than the majority of previously reported catalysts. In addition, the activity of the catalyst had hardly decreased after ten cycles of reaction, which can well meet the requirements of industrial production.
1 Introduction
Dimethyl carbonate (DMC) is a crucial intermediate in organic synthesis with excellent environmental performance and low toxicity.1–3 DMC has been utilized extensively as a methylation or carbonylation reagent in medicine, pesticides, synthetic materials, and other fields.4,5 DMC is an electrolyte for Li-ion batteries because of its high dielectric constant.5–9 Therefore, the synthesis of DMC and its applications have attracted more and more attention from researchers. There are various synthetic methods for DMC, including methanol oxidative carbonylation, methyl nitrite carbonylation, and transesterification.10 For the methanol oxidative carbonylation, the existence of oxygen in the reaction system raises the risk of explosion.11–13 Methyl nitrite carbonylation reduces the possibility of explosion by introducing NO, but the use of toxic raw materials and lengthy process make the method non-preferred.14–16 Transesterification has the advantages of mild reaction conditions, environmental friendliness, simple process, small investment, and high added value of co-produced ethylene glycol (EG) or propylene glycol (PG).17–19
At present, the industrial-grade catalyst for transesterification is CH3ONa.20,21 Although CH3ONa has great activity and efficiency, it is sensitive to water and methanol, readily deactivated, and causes a severe “three wastes” issue. Therefore, the deactivation of catalysts is the most significant barrier to the further development of the transesterification process.22,23
Ionic liquid is favored in the field of catalysis because of their advantages of strong solubility, high boiling point and recyclability, and is a superior substitute to traditional catalysts.18,24–26 Ju et al. reported that [EMIm]Cl was used as the catalyst in the synthesis of DMC. The results showed that the conversion of PC was 35.7% and the selectivity of DMC was 43.2% at 140 °C for 6 h.27 Jeong et al. found that TDodAC has good activity in the synthesis of DMC. The conversion of PC was 61.6% and the selectivity of DMC was 67.6% at 140 °C for 6 h.28 Even though some ionic liquids achieve definite activity in the synthesis of DMC, they suffer from relatively low activity, long reaction times, and high reaction temperatures when compared to CH3ONa. Song et al. utilized [DBUH][Im] to synthesize DMC by transesterification, 97% conversion of EC was obtained at 70 °C for 2 h; however, the reusability of [DBUH][Im] need to be further improved.7 Consequently, it is essential to find a highly active and stable catalyst to replace CH3ONa.
In this paper, analyze and discuss the mechanism of ionic liquid on EC or PC and MeOH based on the investigation of the reaction mechanism of CH3ONa. The conclusion is that it is difficult for the existing basic ionic liquid to match the high activity and stability requirements of transesterification. Efficient and stable synthesis of DMC can be achieved by using strongly basic KIm as catalyst, the DMC yield reached 73.03% at 30 °C for 3 minutes. The catalytic activity was not only comparable to that of CH3ONa, but also did not decrease significantly after multiple cycles. The high stability of KIm can solve the problem of catalytic deactivation in transesterification and is well suited to industrial production requirements.
2 Experimental
2.1 Materials
Imidazole (99%), 1-allyl-3-methylimidazolium bromide ([AmIm]Br, 98%), 1-allyl-3-methylimidazolium chloride ([AmIm]Cl, 97%), sodium methoxide (CH3ONa, 97%), 1-butyl-3-methylimidazolium chloride ([BmIm]Cl, 97%), 1-butyl-3-methylimidazolium acetate ([BmIm] [AcO], 95%), 1-butyl-3-methylimidazolium bromide ([BmIm]Br, 97%), propylene carbonate (PC, 99.5%), 1-hexyl-3-methylimidazolium chloride ([HmIm]Cl, 98%), 1-vinyl-3-butylimidazolium bromide ([VmIm]Br, 98%), bromothymol blue (99%), phenolphthalein (99%), 4-chloro-2-nitroaniline (99%), 2,4-dinitroaniline (99%) and 1-ethyl-3-methylimidazolium bromide ([EmIm]Br, 98%) were obtained from Aladdin. Anhydrous methanol (MeOH, 99.5%) was obtained from Shaanxi Yulin Energy and Chemical Co., Ltd. Ethanol (99.5%) was obtained from Shanghai Lingfeng Chemical Reagent Co., Ltd. Potassium hydroxide (99.9%), sodium hydroxide (99.9%) were obtained from Pharmaceutical Group Chemical Reagent Co., Ltd.
2.2 Catalyst preparation
In 20 mL of KOH anhydrous ethanol solution (c(KOH) = 0.5 mol L−1), 0.01 mol imidazole was added. After vigorous stirring at room temperature for 30 min, an ethanolic solution of KIm was obtained. The crude product of KIm was obtained by reduced pressure distillation followed by repeatedly washing with methanol and distilled under reduced pressure. The obtained solid was dried in a desiccator at 120 °C for 4 h to obtain KIm catalyst.
2.3 Catalytic tests
Different molar ratios (2, 5, 7, 10, 15) of MeOH and PC was added to the three-necked flask. When the reaction mixture was heated and stirred to the set temperature, catalyst (0.2, 0.5, 1, 1.5, 2 wt% to the total mass of the raw materials) was added to reactor. After reacting for a certain time (3, 5, 10, 30, 60 min) at the set temperature, the temperature of the reaction solution was rapidly reduced by means of an ice bath, and the reaction solution was then analyzed by gas chromatography.
The PC conversion, selectivity and DMC yield were defined as:
Here,
XPC is the PC conversion, %;
SDMC is the DMC selectivity, %;
YDMC is the yield of DMC, %;
MPC is the molar mass of PC, g mol
−1;
mPC1 is the mass of PC in the feed before reaction,
g;
mPC2 is the mass of PC in the product after reaction,
g;
MDMC is the molar mass of DMC, g mol
−1;
mDMC is the mass of DMC in the product after reaction,
g.
3 Results and discussion
3.1 The effect of different homogeneous catalysts on the transesterification of PC
As can be seen from Table 1 below, no DMC was generated after 5 minutes of reaction when no catalyst was added. This is owing to the fact that in the absence of a catalyst, only a tiny number of MeOH molecules were activated, and the amount of MeO− formed was negligible, resulting in low catalytic activity. Some base ionic liquids were selected to obtain the activity comparable to that of CH3ONa, as basic catalysts can effectively activate MeOH molecules.
Table 1 The effect of different homogeneous catalysts on the transesterificationa
Reactions |
Catalyst |
Catalyst amount/mol% |
Molar ratio |
Temperature/°C |
Time/min |
PC/EC conversion/% |
DMC yield/% |
DMC selectivity/% |
Reference |
Note: catalyst amount is relative to the amount of PC/EC. |
PC + MeOH |
No catalyst |
0 |
5 : 1 |
50 |
5 |
6.6 |
0.0 |
0.0 |
This work |
[BmIm]Cl |
0.5 |
5 : 1 |
50 |
5 |
11.4 |
8.0 |
70.4 |
This work |
[HmIm]Cl |
0.5 |
5 : 1 |
50 |
5 |
9.3 |
0.3 |
3.3 |
This work |
[AmIm]Cl |
0.5 |
5 : 1 |
50 |
5 |
13.3 |
4.5 |
33.8 |
This work |
[EmIm]Br |
0.5 |
5 : 1 |
50 |
5 |
26.0 |
9.3 |
35.8 |
This work |
[AmIm]Br |
0.5 |
5 : 1 |
50 |
5 |
21.6 |
9.5 |
43.8 |
This work |
[VbIm]Br |
0.5 |
5 : 1 |
50 |
5 |
23.1 |
1.3 |
5.6 |
This work |
[BmIm]Br |
0.5 |
5 : 1 |
50 |
5 |
19.6 |
4.7 |
23.8 |
This work |
[BmIm][AcO] |
0.5 |
5 : 1 |
50 |
5 |
12.1 |
5.3 |
44.1 |
This work |
CH3ONa |
0.5 |
5 : 1 |
50 |
5 |
46.8 |
43.9 |
93.9 |
This work |
NaIm |
0.5 |
5 : 1 |
50 |
5 |
41.9 |
35.5 |
84.7 |
This work |
KIm |
0.5 |
5 : 1 |
50 |
5 |
51.1 |
50.0 |
97.9 |
This work |
EMImCl |
8.0 |
8 : 1 |
140 |
360 |
35.7 |
15.4 |
43.2 |
27 |
OMImCl |
8.0 |
8 : 1 |
140 |
360 |
20.8 |
5.3 |
25.6 |
27 |
[EMIm][BF4] |
8.0 |
8 : 1 |
140 |
360 |
25.2 |
8.0 |
31.9 |
27 |
[EMIm][PF6] |
8.0 |
8 : 1 |
140 |
360 |
22.7 |
4.9 |
21.4 |
27 |
TDodAC |
8.0 |
8 : 1 |
140 |
360 |
61.6 |
41.6 |
67.6 |
28 |
EC + MeOH |
KIm |
1.0 |
10 : 1 |
50 |
5 |
91.7 |
88.2 |
96.7 |
This work |
Imidazole |
1.0 |
15 : 1 |
70 |
360 |
28.0 |
3.0 |
10.7 |
7 |
[SmIm]Cl |
1.6 |
10 : 1 |
65 |
300 |
43.6 |
31.1 |
71.3 |
24 |
[SmIm]OH |
1.7 |
10 : 1 |
65 |
300 |
89.4 |
86.1 |
96.3 |
24 |
DMIC |
1.0 |
10 : 1 |
110 |
80 |
82.0 |
81.0 |
98.7 |
30 |
DABCO |
1.0 |
10 : 1 |
70 |
240 |
95.0 |
84.0 |
88.4 |
31 |
BrTBDPEG150TBDBr |
1.0 |
20 : 1 |
70 |
240 |
96.0 |
85.0 |
88.5 |
32 |
DBU |
1.0 |
15 : 1 |
70 |
360 |
97.0 |
90.0 |
92.8 |
7 |
[DBUH][Im] |
1.0 |
15 : 1 |
70 |
360 |
97.0 |
91.0 |
91.9 |
7 |
The base strength of the catalyst listed in Table S2† was evaluated using the Hammert indicator method, found that the selected ionic liquids had a weak basic strength of 7.2 < H_ < 8.2; CH3ONa, NaIm and KIm had a strong basic strength of 15.0 < H_ < 18.4. According to Table S2,† despite the fact that the chosen ionic liquid exhibited definite activity and selectivity in the transesterification, its activity was clearly inferior to that of CH3ONa, NaIm and KIm. Therefore, we can conclude that the stronger the basicity of the catalyst, the higher the transesterification activity, as demonstrated by the superior catalytic activity of KIm compared to NaIm. On the other hand, contrasting the catalytic performance of different ionic liquids, it is found that when the anions were identical, the longer the carbon chain of the substituent of the imidazolium cation, the poorer the catalytic activity. This is due to the high steric hindrance caused by the long carbon chain of the substituent, which raises the difficulty of activating MeOH.29 Among the selected catalysts, only KIm had achieved catalytic activity comparable to that of CH3ONa, and the high catalytic activity is crucial for realizing industrial applications.
3.2 The effect of reaction conditions on the yield of DMC
The effects of different reaction time, reaction temperature, catalyst amount and MeOH/PC molar ratio were investigated.
3.2.1 Effect of reaction time. Fig. 1(a) depicts the effect of different reaction time on the yield of DMC. Under the same reaction conditions, the yield of DMC reached 53.2% within 3 minutes. Although the reaction time continued to rise, the yield of DMC did not increase significantly, indicating that equilibrium can be reached within 3 minutes, and also demonstrating that the catalytic activity of KIm is extremely high.
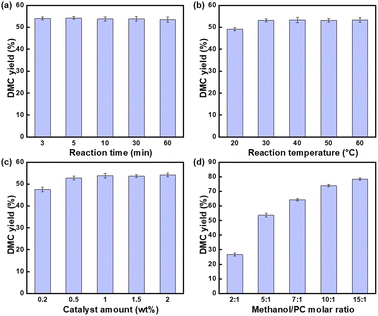 |
| Fig. 1 (a) The effect of reaction time on the yield of DMC. Reaction conditions: the reaction temperature was 50 °C, catalyst amount was 0.5% of the total mass of the raw materials, and n(MeOH) : n(PC) = 5 : 1. (b) The effect of reaction temperature on the yield of DMC. Reaction conditions: the reaction time was 5 min, catalyst amount was 0.5% of the total mass of the raw materials, and n(MeOH) : n(PC) = 5 : 1. (c) Effect of catalyst amount on the yield of DMC. Reaction conditions: reaction time 5 min, reaction temperature 50 °C, and n(MeOH) : n(PC) = 5 : 1. (d) Effect of MeOH/PC molar ratio on the yield of DMC. Reaction conditions: the reaction time was 5 min, the reaction temperature was 50 °C, and catalyst amount was 0.5% of the total mass of the raw materials. | |
3.2.2 Effect of reaction temperature. It was investigated how different reaction temperatures affected DMC yield. The yield of DMC improved as the reaction temperature rised from 20 °C to 30 °C under the same conditions as illustrated in Fig. 1(b). However, when the temperature reached 30 °C, the increase in temperature did not contribute to the increase in DMC yield. So KIm has outstanding catalytic activity at low temperatures. The exceptional low-temperature catalytic activity of KIm can not only considerably reduce the amount of energy required for transesterification, but also simplify the industrial manufacturing process and cut production costs.
3.2.3 Effect of catalyst amount. The effect of catalyst amount on the yield of DMC is displayed in Fig. 1(c). The yield of DMC enhanced as the amount of the catalyst rose from 0.2% to 0.5%, because a higher dose of the catalyst accelerated methanol activation. When the catalyst amount exceeded 0.5%, the increase in catalyst amount had no obvious influence on transesterification. This suggests that the catalyst amount 0.5% of the total mass of the raw materials achieved the most effective catalytic effect.
3.3 The stability test of KIm
3.3.1 Stability of catalytic activity. In addition to examining the catalytic activity and DMC selectivity of KIm in transesterification, the stability of KIm is a crucial element in evaluating its catalytic performance. In order to assess the reusability of KIm, it was recycled following the reaction of PC and MeOH. The catalyst was recovered from the mixture after reaction by vacuum distillation for the next reaction. As demonstrated in Fig. 2, KIm could be reused 10 times. Under the condition that the catalyst amount was only 0.5%, the DMC yield had been maintained between 69% and 72%, and no evident deactivation phenomena had been observed, revealing great catalytic stability. Problems such as rapid deactivation and limited reusability make catalysts difficult to achieve large-scale industrial applications. As a result, the high stability of KIm has good prospects for industrial applications.
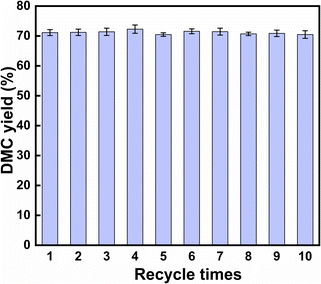 |
| Fig. 2 The effect of catalyst cycle times on the yield of DMC. Reaction conditions: the reaction time was 3 min, the reaction temperature was 30 °C, catalyst amount was 0.5% of the total mass of raw materials, and n(MeOH) : n(PC) = 10 : 1. | |
3.3.2 Thermal stability. Besides the active stability of KIm, the thermal stability of KIm was also explored. The TG-DTG data acquired from KIm is presented in Fig. 3. As is depicted in Fig. 3, the catalyst mainly underwent two stages of mass loss. The first mass loss of 6.7% was mainly resulted from physical absorption of water, as KIm absorbs water easily. The mass of KIm remained basically unchanged from 275 °C to 510 °C. The second mass loss started from 554 °C, when the structure of imidazole was destroyed, accompanied by the release of CO2. As can be seen from the above, KIm has a high thermal stability, which is also advantageous for industrial applications.
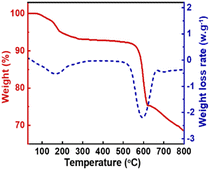 |
| Fig. 3 TG-DTG curves of KIm. | |
3.4 Mechanism of PC and MeOH transesterification
The reaction mechanism of CH3ONa and basic ionic liquid in the transesterification reaction is as follows: first, the basic site of CH3ONa or the anion of the ionic liquid activates the MeOH molecule and captures the proton of MeOH to form MeO−. This step is a rate-controlling step in transesterification. The carbonyl group in EC or PC is then attacked by MeO−, leading to the formation of the active intermediate HEMC (HPMC). MeO− continues to attack the carbonyl group in active intermediate HEMC (HPMC), and finally generating the target product DMC and by-product ethylene glycol (propylene glycol).33,34
MeO− in CH3ONa has strong basicity and nucleophilicity, which is favorable for MeO− to attack carbonyl groups in EC or PC to form intermediates. Furthermore, the radius of Na+ is tiny and its binding ability to MeO− is very limited, resulting in substantial transesterification catalytic activity. For basic ionic liquids, it is challenging for anions to display strong basicity and nucleophilicity like MeO−, and when the anions are the same, the longer the carbon chain of the imidazolium cationic substituent, the greater the steric hindrance, and the more hard it is to activate MeOH to form MeO−.27,29 However, because the ionic liquid cannot be formed if there is no substituent on the imidazolium cation, the conventional ionic liquid cannot satisfy the requirement of high activity of transesterification.
Imidazole is both acidic and basic. Because the basicity of imidazole is not strong enough to activate methanol, the catalytic activity of imidazole in transesterification is unsatisfactory. However, imidazole can generate organic salts with strong bases. The surface electrostatic potential of KIm was calculated. The results is illustrated in Fig. 4, the electrostatic potential value of the blue area is negative, which is easier to give electrons than other areas and is more nucleophilic. It can be inferred that the imidazole anion has strong nucleophilicity, and the surface electrostatic potential of the nitrogen atom on the imidazole ring is the highest, it can produce strong hydrogen bond with MeOH molecule, efficiently extract H+ in MeOH molecule, and activate MeOH molecule to MeO−. K+ has a weak binding to imidazole anion, which is conducive to the abstraction of imidazole anion protons form MeO−. Thus, the catalytic activity of KIm is comparable to that of CH3ONa.
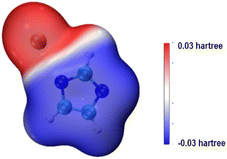 |
| Fig. 4 Electrostatic potential distribution on the surface of KIm molecule. | |
From the above, the reaction mechanism of KIm in PC and MeOH to synthesize DMC is depicted in Fig. 5. In the first step, the imidazolium anion reacts with methanol to take away the hydrogen atom of methanol to generate MeO−. In the second step, MeO− attacks PC as a nucleophilic reagent, breaking the C
O bond and forming intermediate. In the third step, the unstable intermediate breaks the C–O bonds, forming two intermediates a1 and a2. In the fourth step, the nucleophilic addition of intermediates and H+ in the system generates relatively stable and uncharged intermediates HMC and HPMC. In the fifth step, MeO− subsequently nucleophilically attacked HMC and HPMC to form new intermediates; in the sixth step, due to the instability of new intermediates, the C–O bond was destroyed to form the target products DMC and PG−; in the last step, PG− captures or combines with H+ in the system to generate the final product PG.34,35
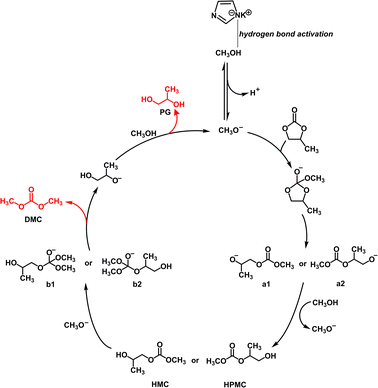 |
| Fig. 5 Schematic diagram of PC transesterification reaction mechanism on KIm catalyst. | |
4 Conclusions
In conclusion, a novel type of homogeneous catalyst KIm was developed and applied to the reaction of PC and MeOH to synthesize DMC. When n(PC)
:
n(MeOH) = 1
:
10, the catalyst amount was 0.5% of the total mass of the raw materials and the chemical equilibrium could reach at 30 °C for 3 minutes, and the DMC yield reached 73.03%. Even at room temperature, KIm exhibited an exceptionally high level of catalytic activity. By studying the mechanism of the transesterification catalyzed by KIm, it was determined that the catalytic active center of KIm was the nitrogen atom on the imidazole ring, which formed a strong hydrogen bond interaction with the reactant and facilitated the activation of methanol. The catalyst can be recycled 10 times, the yield of DMC has been maintained between 69% and 72%, there was no noticeable deactivation phenomena, and the catalyst has superb thermal stability, so it is expected to replace CH3ONa for wider industrial applications.
Author contributions
Yu-Fen Bao: resources, investigation, formal analysis, data curation, writing – original draft. Yi-Jie Wang: formal analysis, writing – review & editing. Yu-Chen Wang: resources, investigation. Ding-Hua Liu: writing – review & editing, funding acquisition, conceptualization, methodology.
Conflicts of interest
The authors declare that they have no conflict of interest.
Acknowledgements
This work was supported by the Project of Priority Academic Program Development of Jiangsu Higher Education Institutions.
References
- S. Ji, Y. Chen, G. Zhao, Y. Wang, W. Sun, Z. Zhang, Y. Lu and D. Wang, Appl. Catal. B, 2022, 304 Search PubMed.
- A. H. Tamboli, A. A. Chaugule and H. Kim, Chem. Eng. J., 2017, 323, 530–544 CrossRef CAS.
- B. J. V. Davies, M. Šarić, M. C. Figueiredo, N. C. Schjødt, S. Dahl, P. G. Moses, M. Escudero-Escribano, M. Arenz and J. Rossmeisl, ACS Catal., 2019, 9, 859–866 CrossRef CAS.
- P. Tundo, M. Musolino and F. Aricò, Green Chem., 2018, 20, 28–85 RSC.
- K. M. Lee, J. H. Jang, M. Balamurugan, J. E. Kim, Y. I. Jo and K. T. Nam, Nat. Energy, 2021, 6, 733–741 CrossRef CAS.
- J. Xie and Y. C. Lu, Nat. Commun., 2020, 11, 2499 CrossRef CAS PubMed.
- Y. Song, X. He, B. Yu, H.-R. Li and L.-N. He, Chin. Chem. Lett., 2020, 31, 667–672 CrossRef CAS.
- W. Liu, Y. Wang, J. Zhang and Q. Shan, Fuel, 2022, 324 Search PubMed.
- H.-Z. Tan, Z.-Q. Wang, Z.-N. Xu, J. Sun, Y.-P. Xu, Q.-S. Chen, Y. Chen and G.-C. Guo, Catal. Today, 2018, 316, 2–12 CrossRef CAS.
- P. Kumar, V. C. Srivastava, U. L. Štangar, B. Mušič, I. M. Mishra and Y. Meng, Catal. Rev., 2019, 63, 363–421 CrossRef.
- W.-S. Dong, X. Zhou, C. Xin, C. Liu and Z. Liu, Appl. Catal., A, 2008, 334, 100–105 CrossRef CAS.
- C. Wang, W. Xu, Z. Qin, H. Guo, X. Liu and S. Mintova, J. Energy Chem., 2021, 52, 191–201 CrossRef CAS.
- J. Xu, K.-Z. Long, T. Chen, B. Xue, Y.-X. Li and Y. Cao, Catal. Sci. Technol., 2013, 3, 1–9 RSC.
- H.-Y. Wu, Y.-Y. Qin, Y.-H. Xiao, J.-S. Chen, R. Guo, S.-Q. Wu, L. Zhang, J. Zhang and Y.-G. Yao, Inorg. Chem. Front., 2022, 9, 2379–2388 RSC.
- D. Delledonne, F. Rivetti and U. Romano, Appl. Catal., A, 2001, 221, 241–251 CrossRef CAS.
- R. Guo, Z. Hou, J. Chen, Y. Qin, G. Chai and Y. Yao, Fuel, 2022, 330 Search PubMed.
- J. Hur, J. Park, R. S. Landon and I. Moon, Ind. Eng. Chem. Res., 2019, 58, 17898–17905 CrossRef CAS.
- Y. Shen, Z. Su, Q. Zhao, R. Shan, Z. Zhu, P. Cui and Y. Wang, Process Saf. Environ. Prot., 2022, 158, 181–188 CrossRef CAS.
- Z. Song, B. Subramaniam and R. V. Chaudhari, ACS Sustainable Chem. Eng., 2019, 7, 5698–5710 CrossRef CAS.
- Z. Song, B. Subramaniam and R. V. Chaudhari, React. Chem. Eng., 2020, 5, 101–111 RSC.
- J. Holtbruegge, M. Leimbrink, P. Lutze and A. Górak, Chem. Eng. Sci., 2013, 104, 347–360 CrossRef CAS.
- S. KoohiKamali, C. P. Tan and T. C. Ling, Sci. World J., 2012, 2012, 475027 Search PubMed.
- W. Deng, J. Yao and L. Shi, Fuel, 2022, 330 Search PubMed.
- J. Xu, H.-T. Wu, C.-M. Ma, B. Xue, Y.-X. Li and Y. Cao, Appl. Catal., A, 2013, 464–465, 357–363 CrossRef CAS.
- H. Y. Ju, M. D. Manju, D. W. Park, Y. Choe and S. W. Park, React. Kinet. Catal. Lett., 2007, 90, 3–9 CrossRef CAS.
- C. Hardacre and V. I. Pârvulescu, Chem. Rev., 2007, 107, 2615–2665 CrossRef PubMed.
- H.-Y. Ju, M. D. Manju, K.-H. Kim, S.-W. Park and D.-W. Park, Korean J. Chem. Eng., 2007, 24, 917–919 CrossRef CAS.
- E.-S. Jeong, K.-H. Kim, D.-W. Park, S.-W. Park and J.-W. Lee, React. Kinet. Catal. Lett., 2005, 86, 241–248 CrossRef CAS.
- J.-Q. Wang, K. Dong, W.-G. Cheng, J. Sun and S.-J. Zhang, Catal. Sci. Technol., 2012, 2, 1480–1484 RSC.
- J.-Q. Wang, J. Sun, W.-G. Cheng, C.-Y. Shi, K. Dong, X.-P. Zhang and S.-J. Zhang, Catal. Sci. Technol., 2012, 2, 600–605 RSC.
- Z.-Z. Yang, L.-N. He, X.-Y. Dou and S. Chanfreau, Tetrahedron Lett., 2010, 51, 2931–2934 CrossRef CAS.
- Z.-Z. Yang, Y.-N. Zhao, L.-N. He, J. Gao and Z.-S. Yin, Green Chem., 2012, 14 Search PubMed.
- Z. Song, X. Jin, Y. Hu, B. Subramaniam and R. V. Chaudhari, ACS Sustainable Chem. Eng., 2017, 5, 4718–4729 CrossRef CAS.
- K. Liu and C. Liu, ACS Omega, 2021, 6, 13839–13846 CrossRef CAS PubMed.
- W. Deng, J. Yao, L. Shi, W. Wei, F. Chen and G. Xu, Chem. Eng. J., 2022, 427 Search PubMed.
|
This journal is © The Royal Society of Chemistry 2023 |
Click here to see how this site uses Cookies. View our privacy policy here.