DOI:
10.1039/D3RA00320E
(Paper)
RSC Adv., 2023,
13, 15772-15782
A pretreatment method combined matrix solid-phase dispersion with dispersive liquid–liquid micro-extraction for polybrominated diphenyl ethers in vegetables through quantitation of gas chromatography-tandem mass spectrometry (GC-MS)†
Received
16th January 2023
, Accepted 18th May 2023
First published on 25th May 2023
Abstract
Herein, a novel pretreatment method for extraction of polybrominated diphenyl ethers (PBDEs) using matrix solid phase dispersion (MSPD) and depth purification using dispersive liquid–liquid micro-extraction (DLLME) from vegetables was designed. The vegetables included three leafy vegetables (Brassica chinensis, Brassica rapa var. glabra Regel and Brassica rapa L.), two root vegetables (Daucus carota and Ipomoea batatas (L.) Lam.), and Solanum melongena L. First, the freeze-dried powders of vegetables and sorbents were evenly ground to a mixture, which was then loaded into a solid phase column containing two molecular sieve spacers, one positioned at the top and the other at the bottom. The PBDEs were eluted with a small amount of solvent, concentrated, redissolved in acetonitrile, and then mixed with extractant. Next, 5 mL water was added to form an emulsion and centrifuged. Finally, the sedimentary phase was collected and injected into a gas chromatography-tandem mass spectrometry (GC-MS) system. The main factors such as the type of adsorbent, ratio of sample mass and adsorbents, volume of elution solvent used in the MSPD process, as well as the types and volume of dispersant and the, extractant used in DLLME were all evaluated using the single factor method. Under optimal conditions, the proposed method showed good linearity (R2 > 0.999) within the range of 1 to 1000 g kg−1 for all PBDEs and satisfactory recoveries of spiked samples (82.9–113.8%, except for BDE-183 (58.5–82.5%)) and matrix effects (−3.3–18.2%). The limits of detection and the limits of quantification were in the range of 1.9–75.1 g kg−1 and 5.7–25.3 g kg−1, respectively. Moreover, the total pretreatment and detection time was within 30 min. This method proved to be a promising alternative to other high-cost and time-consuming and multi-stage procedures for determination of PBDEs in vegetables.
1. Introduction
Polybrominated diphenyl ethers (PBDEs) are extensively used as flame retardants in plastics, textiles, and electronic products to enhance their resistance to fire. Because of their high lipophilicity, chemical persistence, bioaccumulation, and potential adverse effects on wildlife and humans, PBDEs were classified as persistent organic contaminants (POPs) by the Stockholm convention in 2009.1 High concentrations of PBDE residues have been found in various environments, especially in the soil close to landfill sites, sewage treatment facilities, and electronic waste recycling sites.2,3 PBDEs can accumulate in leafy vegetables4 or crops planted on PBDE-contaminated soils,5,6 and ultimately exert adverse effects on local ecosystems and humans via the food chain.7 Furthermore, PBDEs have been demonstrated to exert adverse effects on individuals and their offspring due to disruption in reproductive development8,9 and the endocrine system.10,11 Therefore, research on environmental behavior and ecological risk of PBDEs has gradually attracted the attention of scientific researchers. In addition, effective and sensitive analytical methods for detecting PBDEs in vegetable samples have to be developed to further understand the ecological risk and dietary exposure to PBDEs.
The main sample pretreatment methods for PBDE analysis include solid-phase extraction (SPE),12,13 ultrasonic-assisted extraction (UAE),14 microwave-assisted extraction (MAE),15 and accelerated solvent extraction (ASE),16 followed by purification using multi-layer solid-phase extraction (SPE),17 gel chromatography column (GCC),18 and so on. However, the high cost and time-consuming and multi-stage procedures associated with these techniques underscore the need for developing a new, more readily available, and eco-friendly method. The new sample preparation methods for PBDE analysis include solid-phase microextraction (SPME),19 matrix solid-phase dispersion (MSPD), and dispersive liquid–liquid microextraction (DLLME).20 However, SPME requires specialized apparatus, and the SPME fibers are expensive and their lifespan is limited. In addition, SPME has been reported to be associated with sample carry-over between runs.21
To reduce the consumption of organic solvents, matrix solid-phase dispersion (MSPD) which combines extraction, purification, and concentration, has been promoted for extraction of various organic pollutants from environmental22 and food sample.19 The extraction effectiveness of organic pollutants based on MSPD depends on their properties, such as wide-range hydrophobicity23 of PBDEs (log
Kow values of BDE-28 to BDE-183 is 5.96–8.35) and matrix composition. The vegetable pigments are mainly influenced by the type of adsorbent and extraction conditions. Silicone bonded C18 (octadecyl silyl, ODS), and propyl sulfonic acid (PSA) are two typical chromatographic fillers with completely different composition and properties, which can be used as adsorbents to extract various organic pollutants with different properties.24,25 However, studies on the various extraction conditions of PBDE-contaminated vegetables using the two types of adsorbents are lacking. The extraction effectiveness of PBDEs, such as adsorbent content, reaction time, and adsorbent type, was designed using single factor analysis or response surface method.26 Owing to the vapor pressure and polarity of PBDEs, gas chromatography (GC) is the most widely used technique for analyzing various homologues12,13 using electron ionization-mass spectrometry (EI-MS) for detection.27 GC-EI-MS provides better structural information than GC-electron capture detection.
Vegetables are major sources of vitamins and minerals for the human body.28 Although many reports have suggested that the levels of PBDEs in most foods,29 especially vegetables, are low, the risk of eating PBDE-contaminated vegetables is high because of the high daily intake rate of vegetables. Therefore, detecting the PBDE content in vegetables with precision using combined or emerging techniques for in-depth evaluation of food quality and safety is critical. The present study aimed to develop and validate an effective, facile, and rapid method for the simultaneous determination of seven PBDEs in certain vegetables using combined MSPD based on complex adsorbents and DLLME. Moreover, the main influencing parameters such as the ratio of sample mass and adsorbent, the types of adsorbents, type and volume of elution solvents, and reaction time in MSPD, as well as the type and volume of the extractant and dispersant in DLLME were evaluated systematically using single factor method. The performance of the developed method was also validated using spiked samples, blank produce, and matrix effects, and the presence of PBDEs in real vegetables was evaluated to assess the risk of PBDEs exposure in edible vegetables.
2. Materials and methods
2.1 Chemicals and materials
A mixture of standard solutions (BDE-CSM7) of 20 mg L−1 PBDEs was dissolved in isooctane (AccuStandard, USA), which included 2,4,4′-tribrominated diphenyl (BDE-28), 2,2′,4,4′-tetrabrominateddiphenyl ether (BDE-47), 2,2′,4,4′,5-pentabrominated diphenyl ether (BDE-99), 2,2′,4,4′,6-pentabrominated diphenyl ether (BDE-100), 2,2′,4,4′,5,5′-hexabrominateddiphenyl ether (BDE-153), 2,2′,4,4′,5,6′-hexabrominated diphenyl ether (BDE-154), and 2,2′,3,4,4′,5′,6-heptabrominated diphenyl ether (BDE-183). Isooctane, acetonitrile (ACN), n-hexane, dichloromethane (DCM), acetone (AC), dichlorobenzene (DCB), chlorobenzene (CB), trichloroethane (TCA), carbon tetrachloride (CTC), ethanol and methanol (MeOH) were all of HPLC grade (purity > 98%), and were purchased from ANPEL Laboratory Technologies Inc., (CNW, Shanghai, China).
Octadecyl silyl (C18, 100–200 mesh, CNW), PSA (100–200 mesh, CNW) and alumina (100–200 mesh, CNW) were obtained from ANPEL Laboratory Technologies Inc (Shanghai, China), florisil (30–60 mesh, Sigma-Aldrich) was purchased from Merck (St Louis, USA). Sodium sulfate were all muffled at 450 °C for 8 hours. Six vegetables, including Brassica chinensis (Brassica rapa L.), baby cabbage (Brassica rapa var. glabra Regel), Chinese cabbage (Brassica chinensis L.), carrot (Daucus carota var. sativa Hoffm.), sweet potato (Ipomoea batatas (L.) Lam.), and eggplant (Solanum melongena L.) were purchased from a local market in Suzhou, China. The vegetables were freeze-dried, ground to a fine powder, and then stored at −20 °C until analysis.
2.2 MSPD-DLLME procedures
The MSPD process was modified slightly according to previous methods.27 Accurately weighed spiked vegetable samples (0.20 g), adsorbents C18 (0.80 g), and additional alumina (0.20 g) for purification were transferred to a glass mortar for grinding and mixing for 3 min, and then transferred to a home-made column. The column was compacted with molecular sieve plate, anhydrous sodium sulfate, sample mixture, anhydrous sodium sulfate, and molecular sieve plate from the bottom to top (see Fig. 2f). The samples were eluted using 8 mL hexane/dichloromethane (1
:
1, v/v). The eluates were collected, evaporated using a stream of N2, and re-dissolved in 500 μL acetonitrile. For DLLME, 35 μL carbon tetrachloride was rapidly mixed in 5 mL ultra-pure water. After the solution turned cloudy, it was centrifuged at 4000 rpm for 5 min. The sedimentary facies at the bottom of the centrifuge tube were collected and then evaporated using a stream of N2 and re-dissolved in isooctane (500 μL). Every fresh vegetable sample was pretreated in three replicates. Finally, the PBDEs were sterilized via filtration using 0.22 μm filters and stored in 2 mL brown injection vials for detection using gas chromatography-tandem mass spectrometry (GC-MS/MS) (Fig. 1).
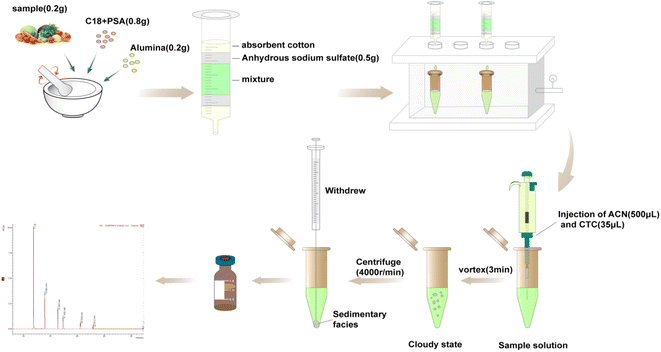 |
| Fig. 1 Step of the detection of PBDEs in vegetables by MSPD-DLLME-GC-MS/MS. | |
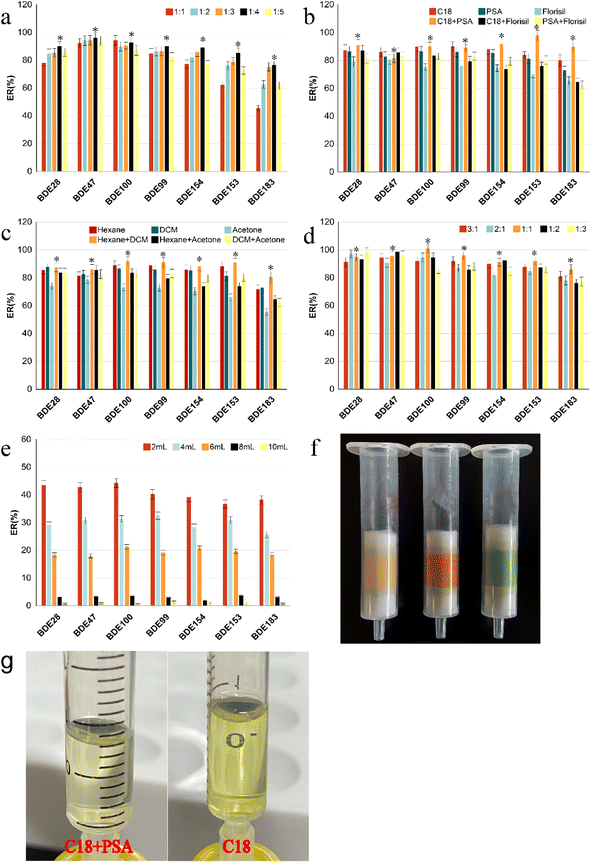 |
| Fig. 2 Optimization of MSPD process (a) the effect of the ratio of sample amount to the adsorbent mass on recoveries; (b) the type of dispersant; (c) the type of elution solvents; (d) the ratio of n-hexane to methylene chloride; (e) volume of elution solvent, (f) the physical diagram of home-made column of MSPD. The spiked concentration was 1000 ng g−1, (g) comparison of transparency of eluents based different adsorbents. Note: * indicates p < 0.01. | |
2.3 Instruments
The PBDEs were analyzed using a gas chromatography-tandem triple quadrupole mass spectrum (SCION456GC-TQ, Bruker, Germany) with a 30 m capillary column (DB-5MS, 30 m × 0.25 μm × 0.25 mm, Bruker, Germany). High purity helium was used as the carrier gas (purity 99.999%), the injection temperature was set at 280 °C, a non-split mode with a constant column flow rate (1.0 mL min−1) was set, and the injection volume was 2.0 μL. The initial temperature of the oven was set at 90 °C with holding for 1 min, and then increased at the rate of 30 °C min−1 to 220 °C, held for 1 min, then increased at the rate of 8 °C min−1 to 270 °C, and held for 3 min. The ion source was electron impact source, and the electron energy was 70 eV. The multiple reaction monitoring mode was used for detection. The collision pressure was set as 2.0 m Torr. The temperatures of ion source and transfer line were set at 260 °C and 280 °C, respectively. The qualitative and quantitative ions pairs and mass of PBDEs are shown in the Table S1.†
2.4 Method validation
To validate this method, the calibration curve range, matrix effect, limit of detection (LOD), limit of quantification (LOQ), accuracy, and precision were evaluated. The linearity of the curve calibration was determined using 1, 10, 20, 100, 500 and 1000 g kg−1 of the concentrations of standard PBDEs, verified by estimating the correlation coefficients (r). Three leafy vegetables, two root vegetables, and one fruiting vegetable, were selected as the blank matrices. The blank samples were spiked with three different concentrations of PBDEs (10, 20, and 100 ng g−1). The accuracy is defined as the average recovery (AR) for PBDEs in spiked matrices, which is acceptable in a range of 80–120% with a relative standard deviation (RSD) of 20%.27 The AR was calculated using the following equation.
where Cf and C0 indicate the concentrations of the analytes in the organic phase and in the initial sample, respectively; Vf and V0 indicate the volumes of the organic phase and sample solvent, respectively.
The matrix effects (ME) were determined as mentioned previously,30 calculated by comparing the slopes of the calibration curves between the blank matrices and pure solvent (isooctane) for all concentrations of the analytes. The MEs were calculated using the following equation, considering the matrix effect to be significant when higher than ±20%.31
The LODs and LOQs were calculated from the values of standard deviations (SDs) associated with the instrument of PBDEs. Seven replicates of each matrix were injected at the lowest concentration level. LOD and LOQ were evaluated statistically as 3.3 and 10 times SD, respectively.
2.5 Statistical analysis
All data are expressed as mean ± SD. The analysis of variance (ANOVA) and Fisher's least significant difference test (LSD) was used to analyze the differences among experimental conditions (p < 0.01).
3. Results and discussion
3.1 MSPD and optimization
The effects of the ratio of sample mass and adsorbent, type of adsorbents, and type and volume of elution solvents on the recoveries of PBDEs were evaluated.
3.1.1 Optimization of the ratio of sample amount to the adsorbent mass (dispersion ratio). According to the reports on adsorbents with better PBDE adsorption capacities,32 0.2 g vegetable was used and, C18 was used as the adsorbent; the adsorbent masses used were 0.2, 0.4, 0.6, 0.8, 1.0 g (i.e., the dispersion ratio was 1
:
1; 1
:
2; 1
:
3; 1
:
4, and 1
:
5, respectively). Then, the PBDEs were eluted using 10 mL n-hexane
:
dichloromethane mixture (1
:
1, v/v). The remain procedure was the same as that used fore MSPD-DLLME. Fig. 2a shows that the extraction recoveries of the targets were first gradually increased with the mass of the adsorbents. A reasonable explanation is that the increase in adsorbent mass can increase the adsorption affinity between PBDEs in vegetables and the adsorbent, resulting in increase in recoveries. However, the recoveries of the PBDEs decreased sharply, at the dispersion ratio exceeding 1
:
4. This is because the additional mass of the adsorbents can adsorb more interfering substances from vegetables,24 causing reduction in the corresponding signal peak of the PBDEs. Therefore, the 1
:
4 ratio between sample mass and adsorbent was selected as the optimum condition, at which the AR of the PBDEs was 76.5–96.2%.
3.1.2 Type of adsorbents. In this study, three commonly used adsorbents (C18, PSA, Florisil) were optimized with sample mass of 0.2 g, dispersion ratio of 1
:
4, and the same conditions as mentioned in 3.1.1. As shown in Fig. 2b, among all adsorbents, the recoveries of all targets using single Florisil as the adsorbent were the lowest, while those of the lower brominated congeners (BDE-28 and -47) using single C18 as the adsorbent were immeasurably higher than those with single PSA. However, the recovery of the higher brominated congener (BDE-183) using single C18 as the adsorbent was significantly higher than that using single PSA. As the hydrophobicity of the PBDE homologs increased with the number of bromine-substituted atoms, the higher brominated congeners showed better sorption capacities on C18 adsorbent than the lower brominated congeners; hence, we also considered the combination effect of PSA and C18 on the extraction recoveries. The recoveries of all targets (except for BDE-47), especially BDE-153 and BDE-183, when using the combination of C18 and PSA were considerably higher than that obtained using single PSA and slightly higher than that obtained using single C18. Although the recoveries of PBDEs did not improve significantly with the addition of a small amount of PSA mixed with C18, the eluent was considerably transparent and clear after the addition of PSA (Fig. 2g). Possibly, the adsorption capacity of PSA for pigment in vegetables is considerably higher than that of the elution solvent, which reduces the desorption of pigments and other interfering substances from the adsorbent into the eluent.24 In general, the recoveries of all PBDEs ranged from 81.6% to 98.2% based on C18 and PSA as the combined adsorbents.
3.1.3 Type of eluent. According to the principle of solubility of substances of similar polarity and high lipid affinity to PBDEs, non-polar or medium polarity organic solvents are generally selected as elution solvents.27 Six common solvents, namely, n-hexane, dichloromethane (DCM), acetone, n-hexane/DCM (1
:
1, v/v), n-hexane/acetone (1
:
1, v/v), and DCM/acetone (1
:
1, v/v), were selected in this study.12,15,32 The results are shown in Fig. 2c. The recoveries of all PBDEs were lowest when acetone was used as the elution solvent, and the elution solution showed dark green and other pigments because of the dissolution of more pigments and interference at the same time, which interfered with the subsequent chromatographic analysis. The recovery of BDE-183 using the mixture of n-hexane/dichloromethane (1
:
1, v/v) as the eluent was significantly higher than that of n-hexane. Good recoveries of PBDEs were observed (86.1–101.1%) when the volume ratio of hexane to dichloromethane was 1
:
1, as shown in Fig. 2d, which is similar to the results of a previous study.27
3.1.4 Volume of elution solvents. A multi-stage collection method was used to optimize the volume of the eluent. For instance, eluent was collected as every 2.0 mL fraction and detected separately. As shown in Fig. 2e, the recoveries of PBDEs in the first 2.0 mL fraction accounted for almost 40% of the recovery. The recoveries of every PBDE congener increased rapidly from 2.0 mL to 6.0 mL, with up to 80% recovery at 6.0 mL and was constant at 8.0 mL. The recovery of each PBDE congener was <1% in the last 2.0 mL fraction. The volume of the eluent was finally selected as 8.0 mL, which was lower than that in a previous study (30 mL).27
3.2 DLLME and optimization
The recoveries were mainly influenced by the type and volume of the extractant, and dispersant in the dispersive liquid–liquid microextraction procedure.
3.2.1 Selection of the type and volume of extractants. In general, the extractant in the DLLME process must be able dissolve the target and be heavier than water to facilitate centrifugal separation and good chromatographic performance for subsequent analysis.20 Furthermore, the volume of the extractant determines the volume of the precipitated phase, and the enrichment coefficient of the target substance. The optimization of four common extractants (including dichlorobenzene, chlorobenzene, trichloroethane, and carbon tetrachloride) and five levels of extractant volume (20, 30, 35, 40, and 50 μL) were performed. As shown in Fig. 3a, carbon tetrachloride has higher extraction performance than the other three extractants for almost all PBDEs. Furthermore, the recoveries of all PBDEs initially increased with the amount of extractant, but slightly decreased when the amount exceeded 35 μL (Fig. 3b). This indicated that excessive amounts of extractants increased the volume of the sedimentary phase, thereby diluting the concentration of the targets. The extractant (35 μL) completely extracted the targets, which is consistent with the result of our previous study.33
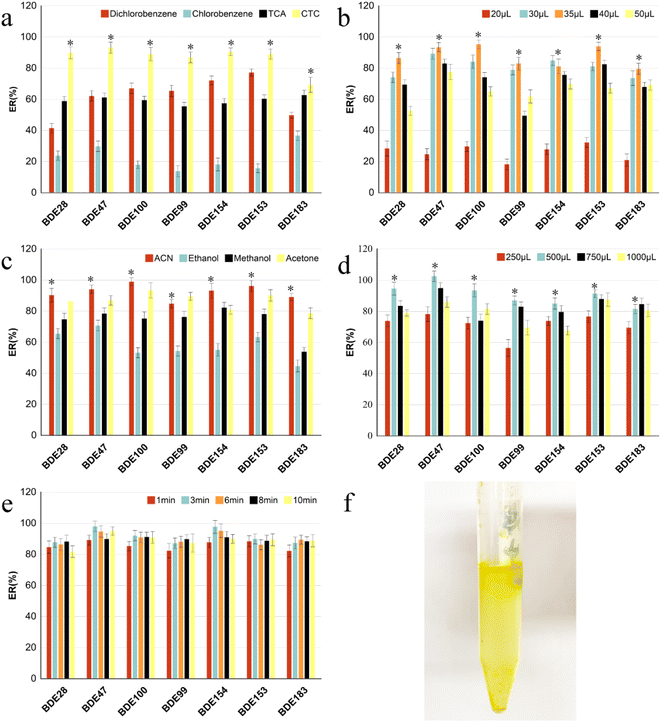 |
| Fig. 3 Optimization of DLLME (a) type of extractant; (b) volume of extractant; (c) type of dispersing solvent; (d) volume of dispersant; (e) extraction time; (f) physical map of forming emulsion. Note: * indicates p < 0.01. | |
3.2.2 Selection of the type and volume of dispersants. Dispersant can increase the contact probability between extractant and target analyte and accelerate the reaction equilibrium during the DLLME process.20 Four typical dispersants, including acetonitrile, ethanol, methanol, and acetone were considered in this study. Fig. 3c shows that the recoveries of all PBDEs, using acetonitrile as the dispersant, were significantly higher than that of methanol and ethanol, and slightly higher than that of acetone. Acetonitrile was finally selected for facile acquisition. The acetonitrile volume (250, 500, 750, 1000 μL) was also optimized. Fig. 3d shows that the recoveries of all PBDEs first increased with dispersant volume and then decreased, with the highest recovery observed when the dispersant volume was 500 μL, except for BDE-183, for which it was 750 μL. However, excessive dispersant resulted in increase in the volume of the sedimentary phase and dilution of target concentration.33 We ultimately selected 500 μL acetonitrile.
3.2.3 Optimization of reaction time. The targets were repeatedly dissolved and volatilized in the water phase and the extractant phase until the equilibrium between the two phases was reached. Therefore, reaction time affects the concentration of the target dissolved in the extractant at equilibrium state (emulsion state).20The effect of reaction time (1, 3, 6, 8, 10 min) on recoveries was investigated (Fig. 3e). The results showed that the extraction recoveries improved continuously with increase in reaction time. The highest was achieved at 3 min, following which it decreased, indicating that the target dissolved in the extractant reached a distributive equilibrium within 3 min. If the reaction time is increased, part of the target would be evaporated from the extractant, causing loss of the targets. Furthermore, it also verified that DLLME had the outstanding advantages of being high efficiency, rapid and simple to operate.
3.3 Method validation using real sample
Calibration curves were established for five different concentration levels in the range of 1–1000 g kg−1. The target compounds in vegetable samples showed good linearity curve calibration, with correlation coefficients exceeding 0.9992. The LODs and LOQs of the seven target compounds were in the range of 0.08–1.0 g kg−1 and 0.24–3.00 g kg−1, respectively, which are shown in Table 1.
Table 1 Linear range, R2, LODs, and LOQs of seven PBDEs congener
Compounds |
Range (ng g−1) |
Calibration linear equations (R2) |
LODs (ng g−1) |
LOQs (ng g−1) |
BDE-28 |
1–1000 |
y = 5233.9478x − 9429.6357 (0.9997) |
0.08 |
0.24 |
BDE-47 |
1–1000 |
y = 3030.3179x − 2620.0439 (0.9996) |
0.13 |
0.39 |
BDE-100 |
1–1000 |
y = 1221.9597x − 2114.1582 (0.9998) |
0.13 |
0.39 |
BDE-99 |
1–1000 |
y = 1364.0752x − 4002.4272 (0.9994) |
0.58 |
1.74 |
BDE-154 |
1–1000 |
y = 2200.2214x − 5104.7711 (0.9994) |
1.00 |
3.00 |
BDE-153 |
1–1000 |
y = 1905.4517x − 1534.7667 (0.9995) |
0.20 |
0.60 |
BDE-183 |
1–1000 |
y = 2515.1306x − 1511.7332 (0.9992) |
0.75 |
2.25 |
The accuracy (average recoveries), precision (intra-day and inter-day), and matrix effects were determined by analyzing vegetable samples spiked with 10, 20, and 100 g kg−1 of standard substances. The results are shown in Table 2. The recoveries of PBDEs ranged from 82.9% to 113.8%, while it ranged from 58.5% to 82.5% for BDE-183. The RSDs for intra-day and inter-day ranged between 0.9% and 4.3%, and between 1.2% and 5.2%, respectively. The matrix effect ranged from −3.3% to −18.2% for all PBDEs. The absence of matrix effect was observed, owing to the good chromatographic peak signal response in six spiked samples shown in Fig. 4.
Table 2 ME (matrix effect), average recovery (AR), intra-day (RSDr) and inter-day (RSDR) (n = 3) of seven PBDEs congeners (%)
Analytes |
Spiked contents (ng g−1) |
Baby cabbage |
Eggplant |
Brassica chinensis |
Carrots |
Chinese cabbage |
Sweet potatoes |
|
|
AR |
RSDr |
RSDR |
ME |
AR |
RSDr |
RSDR |
ME |
AR |
RSDr |
RSDR |
ME |
AR |
RSDr |
RSDR |
ME |
AR |
RSDr |
RSDR |
ME |
AR |
RSDr |
RSDR |
ME |
BDE28 |
10 |
99.6 |
2.0 |
2.1 |
−8.4 |
95.3 |
3.3 |
2.9 |
−9.7 |
82.9 |
2.4 |
3.1 |
−11.2 |
93.2 |
2.0 |
3.2 |
−6.1 |
85.4 |
3.1 |
2.6 |
−11.3 |
104.6 |
2.0 |
2.0 |
−4.2 |
20 |
103.2 |
1.8 |
2.4 |
95.5 |
2.5 |
3.0 |
83.9 |
3.4 |
3.0 |
95.8 |
2.4 |
1.9 |
90.8 |
2.7 |
3.2 |
103.9 |
2.1 |
2.7 |
100 |
98.7 |
1.9 |
1.7 |
97.4 |
2.5 |
2.7 |
83.1 |
2.2 |
2.0 |
96.8 |
1.6 |
2.7 |
90.3 |
2.6 |
1.9 |
101.7 |
1.9 |
3.0 |
BDE47 |
10 |
95.8 |
3.3 |
5.2 |
−5.9 |
94.9 |
2.1 |
3.0 |
−8.1 |
86.5 |
3.3 |
3.5 |
−10.9 |
93.2 |
2.2 |
3.2 |
−5.9 |
93.3 |
4.3 |
2.7 |
−9.3 |
103.2 |
1.7 |
1.6 |
−6.5 |
20 |
101.0 |
3.0 |
2.0 |
102.6 |
2.5 |
1.9 |
86.6 |
3.4 |
1.6 |
95.2 |
2.2 |
1.9 |
87.8 |
3.4 |
3.4 |
98.6 |
1.7 |
2.1 |
100 |
97.2 |
3.1 |
3.5 |
97.8 |
2.4 |
3.0 |
92.9 |
2.7 |
3.2 |
98.9 |
2.4 |
2.1 |
89.9 |
3.2 |
4.0 |
100.7 |
0.9 |
1.3 |
BDE100 |
10 |
99.3 |
1.9 |
2.5 |
−3.3 |
86.6 |
3.0 |
2.7 |
−11.8 |
84.5 |
2.9 |
2.8 |
−7.3 |
105.1 |
2.1 |
3.3 |
−4.9 |
87.5 |
3.6 |
3.0 |
−10.7 |
94.2 |
2.7 |
1.9 |
−8.9 |
20 |
99.0 |
2.0 |
1.6 |
89.7 |
3.7 |
2.2 |
89.4 |
4.1 |
3.2 |
104.6 |
2.2 |
2.9 |
86.3 |
2.6 |
3.5 |
98.2 |
3.6 |
2.1 |
100 |
101.7 |
1.8 |
2.3 |
96.7 |
2.0 |
2.8 |
86.5 |
3.3 |
2.4 |
109.8 |
1.5 |
2.0 |
87.2 |
3.3 |
2.4 |
95.3 |
2.6 |
1.9 |
BDE99 |
10 |
101.2 |
2.0 |
1.5 |
−6.1 |
90.5 |
2.9 |
1.4 |
−10.7 |
88.4 |
3.7 |
3.0 |
−13.8 |
113.8 |
2.6 |
2.2 |
−9.6 |
89.7 |
2.1 |
3.3 |
−11.5 |
111.1 |
2.9 |
2.4 |
−7.8 |
20 |
105.8 |
1.9 |
1.6 |
96.2 |
1.6 |
3.2 |
89.8 |
3.1 |
2.2 |
108.4 |
1.6 |
3.3 |
92.0 |
2.2 |
3.1 |
105.7 |
1.7 |
1.9 |
100 |
108.5 |
2.3 |
2.9 |
102.2 |
1.9 |
2.3 |
92.4 |
2.3 |
3.4 |
111.8 |
1.6 |
1.7 |
93.2 |
1.8 |
3.1 |
111.9 |
2.6 |
1.6 |
BDE154 |
10 |
96.3 |
1.8 |
1.3 |
−7.2 |
89.9 |
3.3 |
1.8 |
−4.8 |
85.8 |
2.9 |
3.1 |
−10.9 |
104.5 |
1.8 |
1.3 |
−8.0 |
85.9 |
3.4 |
1.9 |
−7.3 |
104.6 |
2.3 |
1.2 |
−6.9 |
20 |
99.9 |
3.4 |
1.5 |
89.6 |
2.8 |
2.1 |
86.5 |
2.2 |
1.9 |
102.0 |
3.1 |
2.8 |
90.0 |
2.1 |
3.3 |
105.0 |
3.0 |
2.2 |
100 |
103.7 |
3.0 |
2.4 |
95.6 |
2.2 |
3.2 |
83.9 |
1.6 |
2.9 |
100.9 |
1.7 |
2.3 |
92.4 |
2.5 |
2.9 |
106.6 |
1.0 |
1.7 |
BDE153 |
10 |
104.0 |
1.9 |
2.4 |
−6.5 |
103.0 |
2.0 |
2.8 |
−7.8 |
94.3 |
2.9 |
2.3 |
−8.5 |
108.0 |
1.7 |
1.9 |
−6.8 |
93.4 |
1.4 |
2.1 |
−10.9 |
112.0 |
1.2 |
2.2 |
−5.8 |
20 |
102.2 |
2.6 |
3.1 |
103.8 |
2.4 |
2.7 |
96.6 |
1.8 |
3.1 |
106.6 |
1.1 |
2.1 |
96.7 |
1.9 |
2.4 |
110.2 |
2.2 |
1.2 |
100 |
112.4 |
2.0 |
1.9 |
103.4 |
2.6 |
1.9 |
97.0 |
2.2 |
1.9 |
111.6 |
3.2 |
2.2 |
101.1 |
2.1 |
1.9 |
113.7 |
1.6 |
1.2 |
BDE183 |
10 |
75.6 |
2.4 |
2.6 |
−14.0 |
75.0 |
1.8 |
3.2 |
−17.5 |
58.5 |
2.3 |
3.2 |
−18.2 |
71.4 |
1.8 |
1.7 |
−13.1 |
61.3 |
2.0 |
3.1 |
−16.2 |
78.5 |
2.1 |
3.0 |
−11.9 |
20 |
77.9 |
2.6 |
1.8 |
75.3 |
2.9 |
3.0 |
62.2 |
1.9 |
3.0 |
73.1 |
1.8 |
1.3 |
64.7 |
2.4 |
2.8 |
78.6 |
1.7 |
1.2 |
100 |
77.5 |
2.7 |
1.9 |
76.0 |
3.1 |
1.7 |
65.4 |
2.9 |
2.3 |
78.2 |
1.7 |
1.4 |
66.1 |
2.2 |
3.0 |
82.5 |
1.6 |
1.1 |
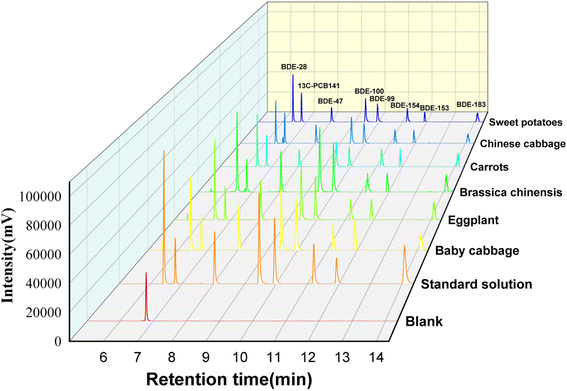 |
| Fig. 4 Chromatographic peak signal response of real spiked sample. | |
To examine the applicability of the proposed method, six types of common vegetables (baby vegetable, eggplant, bracken, carrot, small vegetable, and sweet potato) were purchased from local supermarkets and analyzed using the above developed method under optimal conditions. The results are shown in Table S2.† The total PBDEs concentration ranged from 23.7 to 110.3 g kg−1 in real samples. Furthermore, BDE-28 and BDE-100 were detected in all samples, while BDE-183 was not detected due to its high octanol water partition coefficient (log
Kow = 8.35).23 BDE-47, -100, -153, and -154 were the mainly PBDE congeners in almost all samples.
3.4 Method comparison
To evaluate the performance of the proposed method, methods previously reported for the determination of PBDEs in different matrixes were listed (Table 3). Compared with other methods, the recovery of the PBDEs (82.9–113.8% except for BDE-183) in this study were slightly higher than that of the ionic liquid-based dispersion liquid–liquid microextraction (META-IL-DLLME) (77.3–106.7%).34 The precision (0.9–4.3%) in this study is superior to those of the other methods.34–37 The total preparation and detection time was within 30 min under the premise of satisfactory precision and accuracy. This method uses commonly available commercial adsorbents (C18 and PSA) and does not require complex steps such as the use of synthetic materials and material modification. Only 8.0 mL of the hexane/DCM mixture (1
:
1, v/v) completely eluted the targets. Our method is superior to other methods and small volumes of organic solvent are consumed. In other words, the present method is a low-cost, simple-to operate method that does not require regulation of pH and temperature. This method has the advantages of using little or no solvent and being simple to operation. However, the detection limit for BDE-183, which is highly hydrophobic, is relatively high.
Table 3 The different method for determination of PBDEs
Samples |
Pretreatment |
Detection |
Extraction solvent and its volume |
Method parameters |
Ref. |
Liquid matrix |
META-IL-DLLME |
HPLC-DAD |
Extracted with 10.0 μL C4MIMPF6 and eluted with 1000 μL of ACN |
ER: 77.3–106.7% |
34 |
LODs: 1–170 pg g−1 |
RSDs: 0.6–22% |
Catfish |
QuEChERS |
LP-GC/MS-MS |
Extracted with 10 mL acetonitrile |
ER: 70–119% |
36 |
LOD: 0.5–10 ng g−1 |
RSDs: 3–13% |
Wild bird eggs |
MSPD |
GC-NCI-qMS |
Eluted with 12 mL of hexane/DCM mixture (9 : 1, v/v) |
ER: 83–121% (BDE-209.63%) |
37 |
LOD: 2–16 pg g−1 |
RSDs: 3–13% |
Fish tissues |
MSPD |
GC-QQQ-MS/MS |
Extracted with 20 mL hexane/DCM (1 : 1, v/v) and eluted with 15 mL hexane/DCM mixture (8 : 2, v/v) |
ER: 56.2–119.0% |
35 |
LOD: 0.012–0.078 μg L−1 |
RSDs: 1.32–4.83% |
Vegetables |
MSPD-DLLME |
GC-MS/MS |
Extracted with 8 mL of hexane/DCM mixture (1 : 1, v/v) and 500 μL of ACN |
ER: 82.9–113.8%, BDE-183 (58.5–82.5%) |
This study |
LODs: 1.9–75.1 ng g−1 |
RSDs: 0.9–4.3% |
4. Conclusions
This study established that the pretreatment technique using a combination of MSPD and DLLME is suitable for the extraction and purification of PBDEs from vegetables. MSPD based on two types of absorbents, C18 and PSA, effectively extracted and purified PBDEs from the vegetables. At the same time, DLLME further cleaned-up the PBDEs based on the aqueous solubility of the interference materials in the matrix. This method provides a solution to chromatographic interferences related to some pigments in vegetables using a combination of small amounts of PSA and C18 as adsorbents. Furthermore, the proposed method was successfully applied for detecting PBDE in different vegetables, which resulted in satisfactory and stable recovery of the target PBDEs. This method is a promising alternative of other high-cost, time-consuming, and multi-stage pretreatment procedures. This method offers a new development direction for analyzing PBDEs in vegetables.
Author contributions
S. Xu and X. Deng: experimental scheme design, condition optimization and data processing; J. Wang and Y. Sun: experimental methodology, writing and editing; X. Wang and Z. Zhang: funding acquisition, writing review and editing.
Conflicts of interest
The authors declared no competing interest.
Acknowledgements
This study was supported by the National Natural Science Foundation of China (No. 22076134 and 21876125) and the Graduate Research and Practice Innovation Program of Jiangsu Province (SJCX21_1398).
References
- Bute, W. Chen, T. Li, Y. Liu, H. Wang, P. Zhao, J. Hu and J. Jin, Distributions and biomagnification of polybrominated diphenyl ethers in a grassland ecosystem food chain, Sci. Total Environ., 2020, 747, 141141 CrossRef CAS PubMed.
- Y. Li, S. Niu, R. Hai and M. Li, Concentrations and distribution of polybrominated diphenyl ethers (PBDEs) in soils and plants from a deca-BDE manufacturing factory in China, Environ. Sci. Pollut. Res. Int., 2015, 22(2), 1133–1143 CrossRef CAS PubMed.
- L. Chen, D. Cao, L. X. Li, Y. Zhao, C. M. Xie and Y. H. Zhang, Assessment of Exposure to Polybrominated Dipheny Ethers via Inhalation and Diet in China, Biomed. Environ. Sci., 2014, 27(11), 872–882 Search PubMed.
- C. Y. Yang, S. C. Wu, C. C. Lee and Y. H. Shih, Translocation of polybrominated diphenyl ethers from field-contaminated soils to an edible plant, J. Hazard. Mater., 2018, 351, 215–223 CrossRef CAS PubMed.
- A. R. Fernandes, T. Zwickel and A. Schachtele, Ensuring the reliability of brominated flame retardant data on food and feed occurrence through harmonised analytical criteria and proficiency testing, Chemosphere, 2022, 286(Pt 3), 131921 CrossRef CAS PubMed.
- B. A. Boucher, J. K. Ennis, D. Tsirlin and S. A. Harris, A global database of polybrominated diphenyl ether flame retardant congeners in foods and supplements, J. Food Compos. Anal., 2018, 69, 171–188 CrossRef CAS.
- M. Bedi, N. von Goetz and C. Ng, Estimating polybrominated diphenyl ether (PBDE) exposure through seafood consumption in Switzerland using international food trade data, Environ. Int., 2020, 138, 105652 CrossRef CAS PubMed.
- D. C. Dorman, W. Chiu, B. F. Hales, R. Hauser, K. J. Johnson, E. Mantus, S. Martel, K. A. Robinson, A. A. Rooney, R. Rudel, S. Sathyanarayana, S. L. Schantz and K. M. Waters, Polybrominated diphenyl ether (PBDE) neurotoxicity: a systematic review and meta-analysis of animal evidence, J. Toxicol. Environ. Health B Crit. Rev., 2018, 21(4), 269–289 CrossRef CAS PubMed.
- P. Li, L. Liu, G. Zhou, Z. Tian, C. Luo, T. Xia, J. Chen, Q. Niu, L. Dong, Q. Zhao, A. Wang and S. Zhang, Perigestational exposure to low doses of PBDE-47 induces excessive ER stress, defective autophagy and the resultant apoptosis contributing to maternal thyroid toxicity, Sci. Total Environ., 2018, 645, 363–371 CrossRef CAS PubMed.
- T. Zhang, X. Zhou, A. Xu, Y. Tian, Y. Wang, Y. Zhang, Q. Gu, S. Wang and Z. Wang, Toxicity of polybrominated diphenyl ethers (PBDEs) on rodent male reproductive system: a systematic review and meta-analysis of randomized control studies, Sci. Total Environ., 2020, 720, 137419 CrossRef CAS PubMed.
- L. Xu, Y. Wang, E. Song and Y. Song, Nucleophilic and redox properties of polybrominated diphenyl ether derived-quinone/hydroquinone metabolites are responsible for their neurotoxicity, J. Hazard. Mater., 2021, 420, 126697 CrossRef CAS PubMed.
- M. Smielowska and B. Zabiegala, Determination of polybrominated diphenyl ethers (PBDEs) in dust samples collected in air conditioning filters of different usage – method development, J. Chromatogr., A, 2018, 1565, 57–67 CrossRef CAS PubMed.
- V. C. Fernandes, W. Luts, C. Delerue-Matos and V. F. Domingues, Improved QuEChERS for Analysis of Polybrominated Diphenyl Ethers and Novel Brominated Flame Retardants in Capsicum Cultivars Using Gas Chromatography, J. Agric. Food Chem., 2020, 68(10), 3260–3266 CrossRef CAS PubMed.
- P. Berton, S. B. Mammana, D. A. Locatelli, N. B. Lana, M. B. Hapon, A. B. Camargo and J. C. Altamirano, Determination of polybrominated diphenyl ethers in milk samples. Development of green extraction coupled techniques for sample preparation, Electrophoresis, 2017, 38(3–4), 460–468 CrossRef CAS PubMed.
- K. Jagic, M. Dvorscak, A. Juric, T. Safner and D. Klincic, Optimization and validation of a two-step method for the determination of polybrominated diphenyl ethers in Croatian house dust samples, Anal. Methods, 2021, 13(31), 3504–3510 RSC.
- F. Mercier, E. Gilles, G. Saramito, P. Glorennec and B. Le Bot, A multi-residue method for the simultaneous analysis in indoor dust of several classes of semi-volatile organic compounds by pressurized liquid extraction and gas chromatography/tandem mass spectrometry, J. Chromatogr., A, 2014, 1336, 101–111 CrossRef CAS PubMed.
- Y. Lin, C. Feng, Q. Xu, D. Lu, X. Qiu, Y. E. Jin, G. Wang, D. Wang, J. She and Z. Zhou, A validated method for rapid determination of dibenzo-p-dioxins/furans (PCDD/Fs), polybrominated diphenyl ethers (PBDEs) and polychlorinated biphenyls (PCBs) in human milk: focus on utility of tandem solid phase extraction (SPE) cleanup, Anal. Bioanal. Chem., 2016, 408(18), 4897–4906 CrossRef CAS PubMed.
- M. Lin, J. Tang, S. Ma, Y. Yu, G. Li, B. Mai, R. Fan and T. An, Simultaneous determination of polybrominated diphenyl ethers, polycyclic aromatic hydrocarbons and their hydroxylated metabolites in human hair: a potential methodology to distinguish external from internal exposure, Analyst, 2019, 144(24), 7227–7235 RSC.
- F. Ghasemi, S. Pirsa, M. Alizadeh and F. Mohtarami, Extraction and determination of volatile organic acid concentration in pomegranate, sour cherry, and red grape juices by PPy-Ag nanocomposite fiber for authentication, Sep. Sci. Technol., 2017, 53(1), 117–125 CrossRef.
- S. Pirsa and N. Alizadeh, Rapid determination of pyridine derivatives by dispersive liquid–liquid microextraction coupled with gas chromatography/gas sensor based on nanostructured conducting polypyrrole, Talanta, 2011, 87, 249–254 CrossRef CAS PubMed.
- M. Alizadeh, S. Pirsa and N. Faraji, Determination of Lemon Juice Adulteration by Analysis of Gas Chromatography Profile of Volatile Organic Compounds Extracted with Nano-Sized Polyester-Polyaniline Fiber, Food Anal. Methods, 2016, 10(6), 2092–2101 CrossRef.
- S. v. Gopalan and A. N. Hasanah, Mi-Spe, M-Spe and M-Spd Recent Application on Solid Phase Extraction for Compound Extraction of Complex Matrices, Int. J. Appl. Pharm., 2019, 16–25 CrossRef CAS.
- C. Yue and L. Y. Li, Filling the gap: estimating physicochemical properties of the full array of polybrominated diphenyl ethers (PBDEs), Environ. Pollut., 2013, 180, 312–323 CrossRef CAS PubMed.
- Y. Guo, H. Hu, T. Li, L. Xue, X. Zhang, Z. Zhong, Y. Zhang and Y. Jin, Primary secondary amine as a sorbent material in dispersive solid-phase extraction clean-up for the determination of indicator polychlorinated biphenyls in environmental water samples by gas chromatography with electron capture detection, J. Sep. Sci., 2017, 40(16), 3279–3288 CrossRef CAS PubMed.
- S. Samadi, H. Sereshti and Y. Assadi, Ultra-preconcentration and determination of thirteen organophosphorus pesticides in water samples using solid-phase extraction followed by dispersive liquid–liquid microextraction and gas chromatography with flame photometric detection, J. Chromatogr., A, 2012, 1219, 61–65 CrossRef CAS PubMed.
- S. Pirsa, E. Banafshechin, S. Amiri, A. Rahimirad and J. Ghafarzadeh, Detection of fraud of palm, sunflower, and corn oil in butter using HPLC profile of tocopherols and tocotrienols by response surface method, J. Iran. Chem. Soc., 2020, 18(5), 1167–1177 CrossRef.
- M. Smielowska and B. Zabiegala, Matrix solid-phase dispersion (MSPD) as simple and useful sample preparation technique for determination of polybrominated diphenyl ethers (PBDEs) in dust, Anal. Chim. Acta, 2019, 1084, 33–42 CrossRef CAS PubMed.
- H. Gorska-Warsewicz, K. Rejman, J. Kaczorowska and W. Laskowski, Vegetables, Potatoes and Their Products as Sources of Energy and Nutrients to the Average Diet in Poland, Int. J. Environ. Res. Publ. Health, 2021, 18(6), 3217 CrossRef PubMed.
- W. J. Pietron and P. Malagocki, Quantification of polybrominated diphenyl ethers (PBDEs) in food. A review, Talanta, 2017, 167, 411–427 CrossRef CAS PubMed.
- A. S. Mohammed, G. A. Ramadan, A. I. Abdelkader, S. A. Gadalla, M. M. Ayoub, N. A. Alabdulmalik, W. A. Al Baker and F. Yildiz, Evaluation of method performance
and matrix effect for 57 commonly used herbicides in some vegetable families using LC-MS/MS determination, Cogent Food Agric., 2020, 6(1), 1815287 CrossRef.
- M. Kemmerich, M. Demarco, G. Bernardi, O. D. Prestes, M. B. Adaime and R. Zanella, Balls-in-tube matrix solid phase dispersion (BiT-MSPD): an innovative and simplified technique for multiresidue determination of pesticides in fruit samples, J. Chromatogr., A, 2020, 1612, 460640 CrossRef CAS PubMed.
- B. A. Babalola and A. A. Adeyi, Levels, dietary intake and risk of polybrominated diphenyl ethers (PBDEs) in foods commonly consumed in Nigeria, Food Chem., 2018, 265, 78–84 CrossRef CAS PubMed.
- X. Liu, J. Li, Z. Zhao, W. Zhang, K. Lin, C. Huang and X. Wang, Solid-phase extraction combined with dispersive liquid–liquid microextraction for the determination for polybrominated diphenyl ethers in different environmental matrices, J. Chromatogr., A, 2009, 1216(12), 2220–2226 CrossRef CAS PubMed.
- Y. Li, J. Hu, W. Liu, L. Jin, P. Zhou, Y. Zhang, B. Zhang, R. A. Dahlgren, X. Wang and Y. Zhou, Magnetic effervescent tablet-assisted ionic liquid-based dispersive liquid–liquid microextraction of polybrominated diphenyl ethers in liquid matrix samples, Talanta, 2019, 195, 785–795 CrossRef CAS PubMed.
- P. Labadie, F. Alliot, C. Bourges, A. Desportes and M. Chevreuil, Determination of polybrominated diphenyl ethers in fish tissues by matrix solid-phase dispersion and gas chromatography coupled to triple quadrupole mass spectrometry: case study on European eel (Anguilla anguilla) from Mediterranean coastal lagoons, Anal. Chim. Acta, 2010, 675(2), 97–105 CrossRef CAS PubMed.
- Y. Sapozhnikova and S. J. Lehotay, Multi-class, multi-residue analysis of pesticides, polychlorinated biphenyls, polycyclic aromatic hydrocarbons, polybrominated diphenyl ethers and novel flame retardants in fish using fast, low-pressure gas chromatography-tandem mass spectrometry, Anal. Chim. Acta, 2013, 758, 80–92 CrossRef CAS PubMed.
- J. L. Roscales, A. Vicente, L. Ramos and B. Jimenez, Miniaturised sample preparation method for the multiresidual determination of regulated organohalogenated pollutants and related compounds in wild bird eggs, Anal. Bioanal. Chem., 2017, 409(20), 4905–4913 CrossRef CAS PubMed.
|
This journal is © The Royal Society of Chemistry 2023 |
Click here to see how this site uses Cookies. View our privacy policy here.