DOI:
10.1039/D3RA00636K
(Paper)
RSC Adv., 2023,
13, 7703-7718
Gadolinium-based Sm3+ activated GdSr2AlO5 nanophosphor: synthesis, crystallographic and opto-electronic analysis for warm wLEDs
Received
30th January 2023
, Accepted 24th February 2023
First published on 8th March 2023
Abstract
A material's luminosity characteristics, which in turn dictate its applicability, are critically influenced by its structure. Therefore, it is essential for design and fabrication of optical nanocrystalline materials to comprehend the relationship between structural and luminescence properties. The gel-combustion approach was used to produce a sequence of orange–red light emanating GdSr2AlO5:Sm3+ (GSA:Sm3+) nanophosphors which are used for warm white-light-emitting diodes (w-LEDs). Comprehensive investigation of the structural and optical characteristics of GdSr2AlO5:Sm3+ nanophosphors has been done in a detailed manner. The synthesized powdered nanophosphors are crystallized in a tetragonal phase with I4/mcm (140) space group, affirmed through Rietveld refining method. The nano size with an aggregated, spherical form of the particles in the powdered nanocrystalline material was revealed by TEM analysis. These orange–red emitting phosphors Gd1−xSr2AlO5:xSm3+ (x = 1–7 mol%) were shown to possess photoluminosity (PL) properties that demonstrated the presence of most intense emission peaks at 603 nm that were caused by 4G5/2 → 6H7/2 transitions of the Sm3+ ion under 273 nm excitation. Considering its long decay lifespan and PL emission, it can be concluded that the GdSr2AlO5:Sm3+ phosphor is a potential single element for the fabrication of warm white light-emitting devices.
1 Introduction
Due to an overpopulation problem and individuals choosing to live opulent lifestyles, there has been a significant increase in global energy demand and consumption. Two important strategies viz. energy-saving tactics and utilizing green, renewable energy can be used to address the energy demand and consumption issues.1–5 Researchers are fascinated by a class of rare earth doped nanophosphors in this emerging era of nanotechnology because of its implications in field emission displays (FED),6 white light emitting diodes (wLEDs),7–9 plasma displays (PD)10,11 and opto-electronic devices.12–14 Due to their intriguing properties, such as their strong luminescence reliability, spectacular chemical stability, and adaptable emission color accompanied by a variety of activators, these sorts of nanophosphors are extensively researched.15,16 The wLEDs have been steadily replacing conventional incandescent and fluorescent lamps to become the predominant light sources in our everyday lives because of their high luminous efficiency, long lifespan and energy economy.17–19 However, the current state of commercial wLEDs is still poor due to the absence of a suitable red component, which results in a low color rendering index and subpar light output.20,21 Thus, the creation of novel red phosphors useful for WLEDs is still a significant and pressing subject, one that is gaining more and more attention in the solid state lighting (SSL) field.22
Simply a minor extent of rare earth (RE) ions might significantly alter the phase, stability and photo-physical characteristics of host semiconductor nanoparticles. These doped systems are currently regarded as a crucial class of optoelectronic materials because they can produce dopant light that is effective, stable, and controllable.23,24 Therefore, adopting a host material with exceptional luminescence efficiency qualities is essential for the design and development of innovative optical materials. Gadolinium based GdSr2AlO5 (GSA) is assumed to be a best host material because of its good magnetic and luminescent characteristics which would be substantial and expressive for the potential application in lighting field. GdSr2AlO5 (GSA) is crystallized into tetragonal crystal structure with space group I4/mcm.25 In the unit cell (Z = 4) of GdSr2AlO5, Gd and one Sr atom surrounded with 8 oxygen-atoms, second Sr atom surrounded with ten oxygen-atoms and Al atoms form tetrahedral with four oxygen atoms.26 Eu3+-doped red phosphors are typically thought of as the optimum red components for wLEDs, however using pricey Eu sources makes it difficult to realize low-cost lighting applications. So, in RE family, Sm3+ ion having the usual f–f electronic-transitions are commonly used as effective dopant for orange/red visible light in host matrix.27 To investigate novel red nanophosphor modules for wLEDs, further more research on Sm3+ doped inorganic material has been dynamically launched. Largely, the classic solid-state reaction (SSR) technique is typically used to develop nanophosphor. This method often involves a lengthy, high-temperature heating procedure followed by grinding. The phosphor surfaces are degraded during the grinding process, which reduces the intensity of the emission. Additionally, the product is easily able to include many impurity phases due to inadequate mixing and poor reactivity of the basic ingredients. The phosphor precursor is recently prepared using a variety of wet chemical processes, including co-precipitation,28 sol–gel,29 combustion,30 hydrothermal31 and solvothermal.32
Due to its benefits in producing novel chemical compositions with distinctive properties, excellent purity, smaller particle sizes and relatively low reaction temperature leading to more homogeneous products, the gel-combustion process has recently attracted a lot of devotion among synthetic approaches.33,34 We are aware of no significant reports in the literature regarding the crystallography and opto-electronic characteristics of GdSr2AlO5:Sm3+ nanophosphors produced via the gel-combustion process. Therefore, in the present work, Gd1–xSr2AlO5:xSm3+ (x = 1–7 mol%) phosphors have been fabricated by gel-combustion process. The diffraction, transmission electron microscope (TEM), EDX and photoluminescence spectroscopy have been engaged to illustrate the samples. The photoluminescence properties of GdSr2AlO5:Sm3+ were studied in detail, including excitation and emission spectra and CIE color coordinates. Additionally, the possible energy transfer mechanism from Gd3+ to Sm3+ on tuning of the down-conversion emission properties and lifetime decay investigation of the considered samples were discussed and concluded.
2 Experimental
2.1 Synthesis and instrumentations
A series of Gd1-xSr2AlO5:xSm3+ (x = 1–7 mol%) powder samples were produced using a gel-combustion approach (Fig. 1). According to the chemical formulae, stoichiometric amounts of high grade chemicals of Sigma Aldrich, such as Gadolinium Nitrate Hexahydrate [Gd(NO3)3·6H2O; 99.9% pure] and Samarium Nitrate Hexahydrate [Sm(NO3)3·6H2O; 99.9% pure], Aluminium Nitrate Nonahydrate [Al(NO3)3·9H2O; 99.9% pure], Strontium nitrate [Sr(NO3)2; 99.9% pure] and Urea [NH2CONH2] have been used as raw materials. Double distilled (DD) water has been utilised as a solvent. Primarily, precursor were taken in measured stoichiometry and liquefied completely in solvent (deionized water). Upon warming at ∼80 °C, this prepared mixture was converted to viscous form due to vaporization of solvent molecules. Then, a measured amount of fuel (urea) with deionized (DI) water has been added to resulting viscous solution and further heated. The formed gel type mixture was allowed to combust for fifteen minutes in a furnace which is already heated at 600 °C. In this one step preparation technique, gaseous products viz., oxides of carbon and nitrogen excluded out during combustion.35 The low temperature of reaction is answerable for development of nano-crystalline materials. This combustion method is exothermic in nature which results in excessive crystallinity of produced materials. The product formed is porous in nature was allowed to cool and successive grinding of product to obtain fine crushed material. The formed powdered sample was further calcined at 1350 °C in the muffle-furnace to obtain desired product.
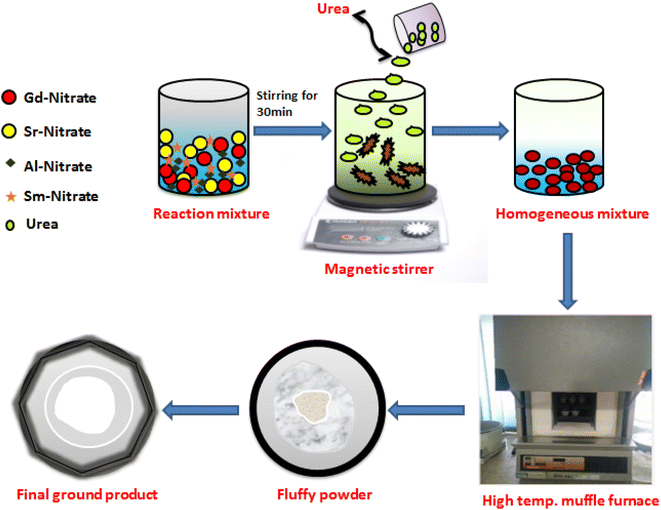 |
| Fig. 1 Schematic diagram of GC method for the synthesis of Gd1−xSr2AlO5:xSm3+ (x = 1–7 mol% Sm3+) nanopowders. | |
The engineered nanosamples were studied via diverse techniques at normal conditions. Phase and other crystal parameters were explored by operating on Rigaku Ultima-IV X-ray diffractometer (with Cu-Kα-radiation; wavelength is 0.15405 nm, current is 40 mA, voltage is 40 kV) with a step-width of 0.02° and scanning-frequency 2° per minute. The shape and size of the particle were inspected by TECNAI TEM (field-emission gun of 200 kV). Ametek-energy-dispersive X-ray spectrometer was employed for recording EDX profiles for elemental investigation. The reflectance spectra of phosphors have been noted on a UV-3600 Plus, Shimadzu Spectrophotometer for locating optical band data gap. Horiba Fluorolog (Jobin YVON) spectrophotometer was operated for achieving the photoluminescence individualities of nano-particles by recording spectral profiles on it.
3 Results and discussion
3.1 Analysis of crystal phase and structure
Diffraction exploration was used to complete the crystalline structure examination and the final Gd1–xSr2AlO5:xSm3+ (x = 1–7 mol%) samples were determined to be compatible with the normal host GdSr2AlO5 matrix framework and well indexed with standard patterns (JCPDS number 70-2197),36 as displayed in Fig. 2(a). This finding indicates that no rare earth ion peak or secondary phase residues can be identified in the XRD graph. Hence, materials created by synthesis are made of single phase. However, in the present case, there is peak shifting mechanism was observed. The position of the diffraction peak shifted to the left, as indicated in the Fig. 2(b), in accordance with the Bragg diffraction hypothesis 2d
sin
θ = nλ.37 The θ-value decreased because the radius of the doped ions (Sm3+) was bigger, which increased the value d-spacing, listed in Table 1. The GdSr2AlO5 crystal lattice is composed of GdSrO3+ and SrAlO43+ layers, as displayed in Fig. 3. Around the rare earth ion Gd3+ at the half 8 h position are eight oxygen atoms forming GdO8 polyhedron. Two distinct crystallographic locations exist for the alkaline earth ion Sr2+: 8 h (Sr1) and 4a (Sr2). Sr1 atoms are positioned to place the other half of the 8 h sites, and Sr2 atoms are nearby to create 10-fold coordinated polyhedra. There are three lattice-sites with two different valences that are evidently available for the assimilation of samarium ion.38 The incoming activator Sm3+ ions are thought to enter in Gd3+ sites. To further support the idea that the Sm3+ ion may be readily integrated into the GSA host, which is described as the following (eqn (1)), the percentage difference of radius (Dr) should be examined.39 |
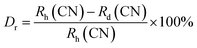 | (1) |
here, the radius of Sm3+ ion (Rd) is 1.079 Å when the coordinate number (CN) is 8. The radius of the possible substituted Gd3+ ion (Rh) was equal to be 1.053 Å (CN = 8). Moreover, because of the balance of chemical valence, the incoming Sm3+ ions would substitute the Gd3+ ion locations. When CN = 8, it was simple to compute the percentage difference in radius (Dr) between the Sm3+ and Gd3+ ions to be around −2.46%, which is significantly less than 30%,40 further indicating that the activator Sm3+ ion would occupy the host Gd3+ ions positions. Additionally, it is exposed through refinement process that every sample in the synthesized Sm3+ series has a pure tetragonal crystal structure having I4/mcm (140) space group symmetry. As a result, it can be concluded that the combustion-derived products are extremely pure and do not alter with variations in Sm3+ concentration. With the use of crystallographic data of GSA and Sm3+ (4 mol%) doped GSA samples, the refinement findings with Rp = 4.95% and 6.78%, Rwp = 5.20% and 7.32%, χ2 = 2.22 and 2.61, respectively. The refined profile of GSA and Sm3+ (4 mol%) doped GSA samples are displayed in Fig. 4 (a and b). Table 2 shows the comparative refinement results of synthesized host and optimal doped phosphors. Table 3 listed the well-refined atomistic locations and displacement characteristics of host material. Scherrer's-relation, shown in eqn (2) was used to calculate the crystallite size of the fabricated samples.41 |
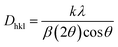 | (2) |
here, X-ray wavelength is symbolized as λ, diffraction angle is denoted with θ, and most intense peak's full width at half maximum (radians) is denoted with β. For the crystalline nanosamples of GdSr2AlO5 and Gd1−xSr2AlO5:xSm3+ (x = 1–7 mol%), the crystallite size was possibly estimated to be in between 29–35 nm. Diffractograms show peak shifting and line broadening due to the presence of micro-strain in crystallized nanomaterial. Thus, the strain existing in the investigated nanosamples was evaluated by utilizing eqn (3) employing W–H linear fitting approach.42 |
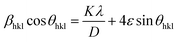 | (3) |
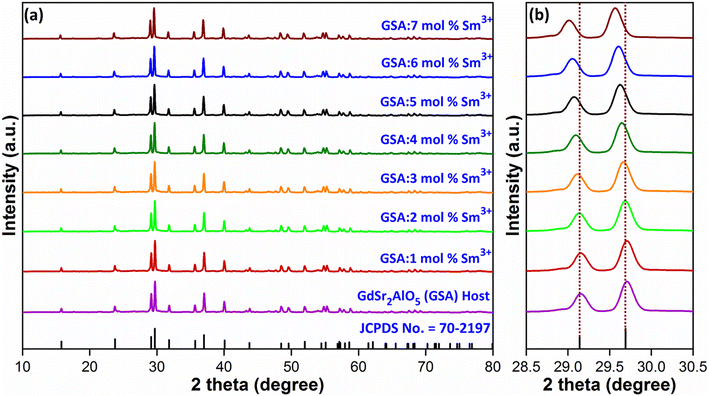 |
| Fig. 2 (a) Diffraction profiles of GdSr2AlO5 and Gd1−xSr2AlO5:xSm3+ (x = 1–7 mol% Sm3+) phosphors (b) enlarged view of respective diffraction patterns. | |
Table 1 Computed interplaner d-spacing values of GSA:xSm3+ (x = 1–7 mol%) phosphors
Sample |
2θ-value |
d-spacing value (Å) |
GSA:0 mol% Sm3+ |
29.70 |
3.0056 |
GSA:1 mol% Sm3+ |
29.69 |
3.0066 |
GSA:2 mol% Sm3+ |
29.67 |
3.0087 |
GSA:3 mol% Sm3+ |
29.66 |
3.0095 |
GSA:4 mol% Sm3+ |
29.64 |
3.0115 |
GSA:5 mol% Sm3+ |
29.61 |
3.0144 |
GSA:6 mol% Sm3+ |
29.59 |
3.0165 |
GSA:7 mol% Sm3+ |
29.56 |
3.0194 |
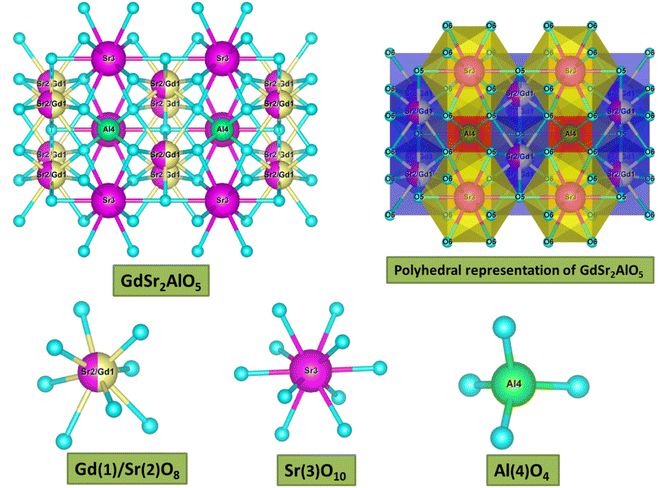 |
| Fig. 3 3-D structural view of host GdSr2AlO5 sample along with coordinative environment of different cations. | |
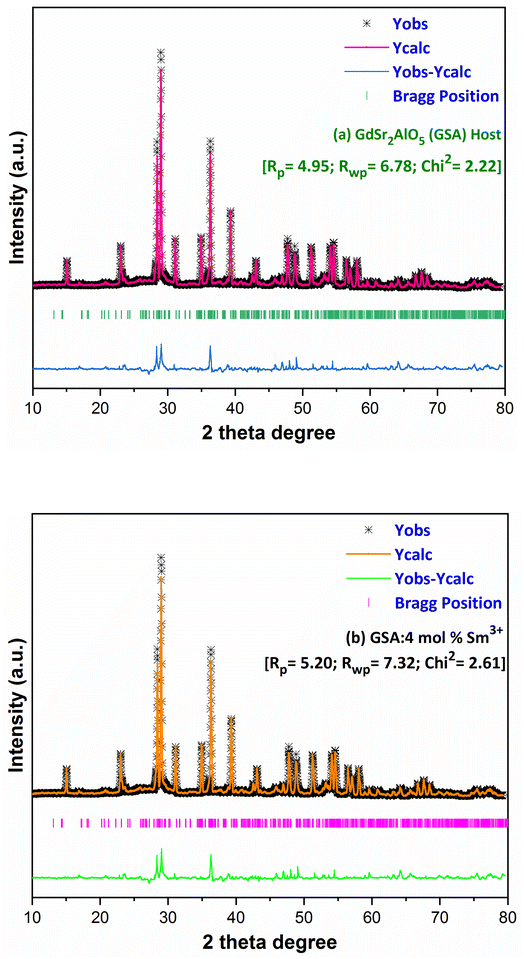 |
| Fig. 4 Rietveld profiles of (a) GdSr2AlO5 and (b) Sm3+ (4 mol%) doped GdSr2AlO5 phosphors. | |
Table 2 Crystallographic parameters for GdSr2AlO5 and optimal Gd0.96Sr2AlO5:4 mol% Sm3+ nanophosphors
Sample |
GdSr2AlO5 |
Gd0.96Sr2AlO5:4 mol% Sm3+ |
Crystal system |
Tetragonal |
Tetragonal |
Lattice symbol |
I |
I |
Space group |
I4/mcm |
I4/mcm |
Pearson symbol |
tI |
tI |
Centro-symmetry |
Centric |
Centric |
Formula unit (Z) |
4 |
4 |
Space group number |
140 |
140 |
α = β = γ |
90.0000 |
90.0000 |
a (Å) |
6.88630 |
6.89217 |
b (Å) |
6.88630 |
6.89217 |
c (Å) |
11.04070 |
11.07214 |
Volume (Å3) |
523.562 |
525.948 |
R-factors |
4.95, 6.78 |
5.20, 7.32 |
χ2 |
2.22 |
2.61 |
Table 3 The atomistic and equivalent isotropic displacement parameters of host GdSr2AlO5
Atom |
x |
y |
z |
Wyck |
U |
Occ |
Sym |
Gd1 |
0.17951 |
0.67951 |
0.00000 |
8h |
0.006 |
0.500 |
m.2 m |
Sr2 |
0.17951 |
0.67951 |
0.00000 |
8h |
0.006 |
0.500 |
m.2 m |
Sr3 |
0.00000 |
0.00000 |
0.25000 |
4a |
0.006 |
1.000 |
422 |
Al4 |
0.00000 |
0.50000 |
0.25000 |
4b |
0.009 |
1.000 |
−42 m |
O5 |
0.00000 |
0.00000 |
0.00000 |
4c |
0.012 |
1.000 |
4/m.. |
O6 |
0.14343 |
0.64343 |
0.64207 |
16l |
0.012 |
1.000 |
..m |
The W–H plot (Fig. 5) reveals that the graph between 4
sin
θhkl v/s cos
θhkl indicates a straight line. The linear fitted profile's slope and intercept values perfectly capture ε and D values of the sample. All manufactured samples have different crystallite sizes that vary within 34–40 nm. It has been revealed that the crystallite sizes derived by using W–H plots are larger than those obtained by the Debye Scherrer's formula. It has been due to the strain component that influences the produced nanocrystalline materials is the primary reason of this minimal variation. The determined outcomes are concise in Table 4.
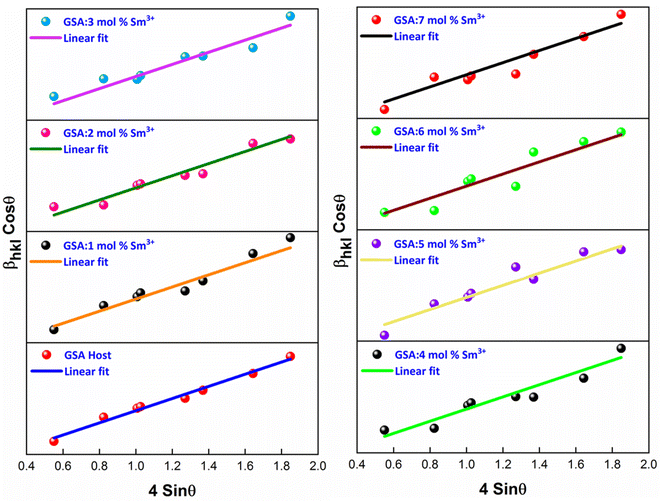 |
| Fig. 5 W–H plots of Gd1−xSr2AlO5:xSm3+ (x = 1–7 mol% Sm3+) phosphors. | |
Table 4 XRD characteristics of host GSA and GSA:xSm3+ (x = 1–7 mol% Sm3+) phosphors
Sample |
FWHM |
Crystallite size (nm) |
Microstrain (ε × 10−4) |
Scherrer's |
W–H |
GSA:0 mol% Sm3+ |
0.2368 |
34.71 |
43.29 |
5.2314 |
GSA:1 mol% Sm3+ |
0.2403 |
34.20 |
41.11 |
5.7890 |
GSA:2 mol% Sm3+ |
0.2479 |
33.15 |
40.24 |
5.9504 |
GSA:3 mol% Sm3+ |
0.2561 |
32.09 |
39.03 |
6.2896 |
GSA:4 mol% Sm3+ |
0.2633 |
31.21 |
37.89 |
7.2560 |
GSA:5 mol% Sm3+ |
0.2682 |
30.64 |
36.28 |
7.7221 |
GSA:6 mol% Sm3+ |
0.2733 |
30.07 |
35.48 |
7.9730 |
GSA:7 mol% Sm3+ |
0.2819 |
29.16 |
34.87 |
8.5634 |
3.2 TEM examination
TEM examination has been employed to comprehend dimensional features i.e. shape & size of crystalline materials. As we can see in Fig. 6, a non-uniform morphology has been observed comprising agglomerated nanoparticles in the range of 30–65 nanometers. The optical performance of the synthesized nanomaterials in the luminous field is supported by the findings of the diffraction probe and TEM examination, which closely resemble to one another.
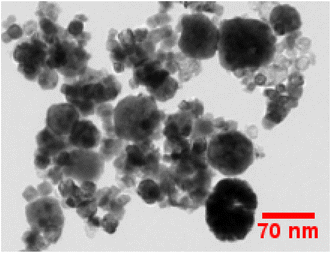 |
| Fig. 6 TEM micrograph of as-prepared Gd0.96Sr2AlO5:4 mol% Sm3+ phosphors. | |
3.3 EDX exploration
EDX spectrum analysis has shown that Gd0.96Sr2AlO5:4 mol% Sm3+ nanophosphor were successfully fabricated. The dominating components in the manufactured nanosample might be identified owing to the spectral-lines in EDX shown in Fig. 7. Gd, Sr, Al, O, and Sm are the only spectral peaks in the EDX spectrum of the optimal sample, demonstrating that no other atoms are existing in the nanophosphor and that the desired material has produced with exact composition. The distinctive Sm3+ signal demonstrated that the former ion had been dispersed uniformly throughout the produced crystalline nanomaterial. EDX data (atomic & weight %) for Gd0.96Sr2AlO5:4 mol% Sm3+ is shown in Table 5. The precise elemental distribution and stoichiometry of the homogenous nanophosphor composition are revealed by the EDX results.
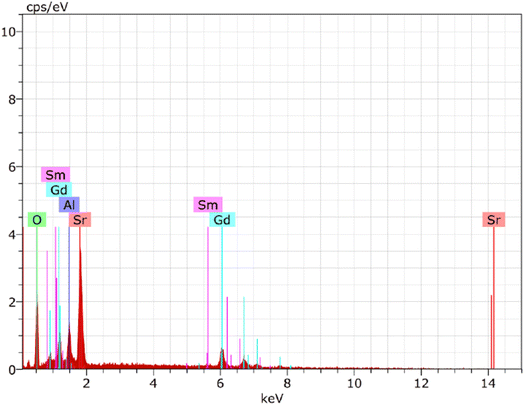 |
| Fig. 7 EDX spectrum of as-prepared Gd0.96Sr2AlO5:4 mol% Sm3+ phosphors. | |
Table 5 Atomic & Weight percent of GSA:4 mol% Sm3+ nanophosphors
Element |
Series |
Atomic (%) |
Weight (%) |
Strontium (Sr) |
L-series |
18.51 |
4.51 |
Oxygen (O) |
K-series |
62.34 |
10.83 |
Aluminium (Al) |
K-series |
7.73 |
0.81 |
Gadolinium (Gd) |
L-series |
10.37 |
3.75 |
Samarium (Sm) |
L-series |
1.05 |
0.67 |
3.4 Reflectance spectra and energy band gap analysis
To evaluate the optical characteristics and band gap of GdSr2AlO5 host and optimized Gd0.96Sr2AlO5:4 mol% Sm3+ nanophosphors, reflectance spectra in the 200–800 nm wavelength range were measured, as displayed in Fig. 8. This graph unequivocally shows that the trivalent samarium ions distinctive peaks are associated to the DRS spectrum. Band gap of derived phosphor may be determined using Kubelka–Munk function (eqn (4)), which is defined as.43 |
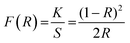 | (4) |
here, R, S and K stand for reflectance, scattering coefficient and absorption coefficient correspondingly. However, Tauc's formulation (eqn (5)) provides the optical band gap (Eg) values.44 |
[F(R)hν]2 = C2(hν − Eg)
| (5) |
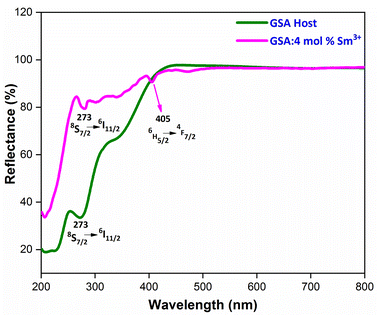 |
| Fig. 8 Diffuse reflectance spectra of host GdSr2AlO5 and Sm3+ (4 mol%) doped GdSr2AlO5 phosphors. | |
Upon extrapolation, [F(R)hv]2 to 0 offset, the acquired Eg values are 4.94 & 5.29 eV for host and optimal doped nanophosphor, separately (Fig. 9). Optical band-gap values found to be increased with addition of Sm3+ ion content in host material, which was defined via Burstein–Moss effect.
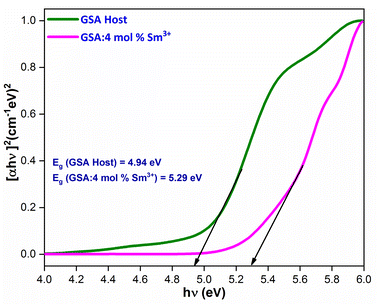 |
| Fig. 9 for the GdSr2AlO5 and Sm3+ (4 mol%) doped GdSr2AlO5 phosphors. | |
3.5 Photoluminescence analysis
3.5.1 PL investigation of undoped GdSr2AlO5. The excitation and emission spectra of undoped GdSr2AlO5 crystalline nanomaterial synthesized via gel-combustion method is shown in Fig. 10(a and b) respectively. Fig. 10(a) demonstrates the PLE spectrum of GdSr2AlO5 in the spectral region of 200–300 nm which obtained by monitoring the emission wavelength at 324 nm. The major excitation peak at 276 nm is ascribed to the 8S7/2 → 6I11/2 transition of Gd3+ and minor peaks at 246 nm and 311 nm correspond to the 8S7/2 → 6D7/2 and 8S7/2 → 6P7/2 transition of Gd3+ ion.45 Fig. 10(b) displays the emission spectrum of GdSr2AlO5 under 276 nm excitation. The sharp peak at 324 nm was observed due to the 6PJ → 8S7/2 transition of Gd3+ ion.46
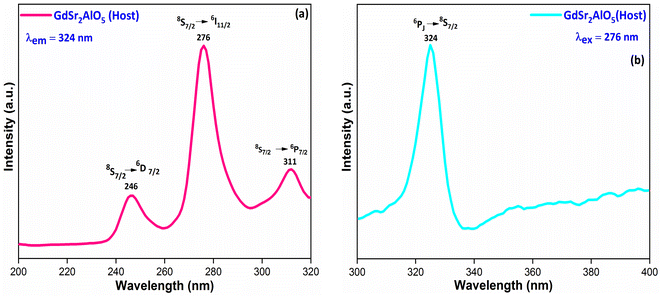 |
| Fig. 10 Photoluminescence behaviour (a) excitation spectrum and (b) emission spectrum of host GdSr2AlO5 nanomaterial. | |
3.5.2 PLE investigation Sm3+ doped GdSr2AlO5. The PLE spectrum is examined at ∼600 nm emission-wavelength of Sm3+ ion, represented in Fig. 11. There are numerous peaks perceived in the range 200–600 nm due to host ion and dopant ion. In PLE spectrum, charge transfer band (CTB) at 251 nm (O2−–Sm3+) and peaks due to the host ion are observed at 273 nm and 313 nm with 8S7/2 → 6I11/2 and 6P7/2 separately.47 While peaks perceived at 346 nm, 362 nm, 375 nm, 405 nm, 421 nm, 462 nm, 476 nm and 562 nm with transitions: 6H5/2 → 4K7/2, 4H7/2, 6P7/2, 4F7/2, 6P5/2, 4G9/2, 4F5/2, 4G7/2 and 4G5/2 correspondingly are due to intra f–f transitions of trivalent samarium ions.48 The strongest band at ∼273 nm is substantially intense than the other peaks detected owing to samarium ion, demonstrating that there is proficient energy-transfer takes place from Gd3+ to Sm3+ ion, displayed in Fig. 12.
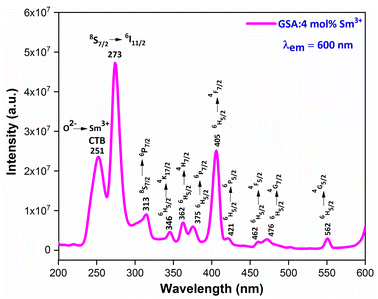 |
| Fig. 11 PL excitation spectra of Sm3+ (4 mol%) doped GdSr2AlO5 phosphors. | |
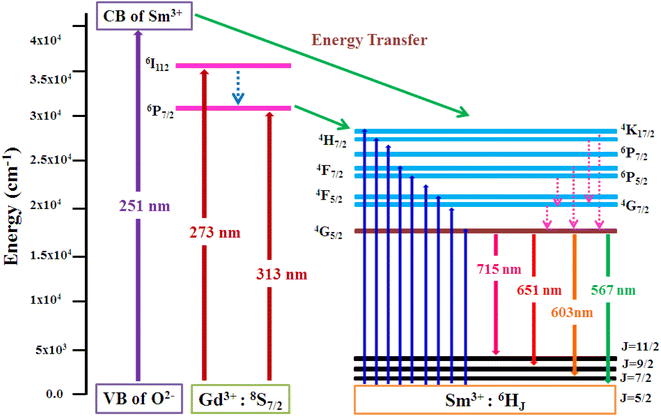 |
| Fig. 12 Pictorial representation of energy transfer in the synthesized nanophosphors. | |
3.5.3 PL investigation Sm3+ doped GdSr2AlO5. At an excitation wavelength of 273 nm, emission spectra in the range of 500–750 nm are captured for Gd1–xSr2AlO5:xSm3+ (x = 1–7 mol%) phosphors, as pictured in Fig. 13. The transition of the excited state 4G5/2 of the Sm3+ ion to its ground states 6H5/2, 6H7/2, 6H9/2, and 6H11/2 is responsible for the narrow peaks at 567 nm (yellowish), 603 nm (orangish-red), 651 nm (red) and 715 nm (deep-red), separately.49 The most dominant peak at 603 nm in all transitions of Sm3+ (1–7 mol%) doped GdSr2AlO5 phosphors corresponds to transition 4G5/2 → 6H7/2 of Sm3+ ion, which gives fabricated nanophosphors their orangish-red color. The quality of the Sm3+ ions transition 4G5/2 → 6H7/2 (ΔJ = ± 1) positioned at 603 nm, is partly electric-dipole (ED) and partly magnetic dipole (MD). The transition 4G5/2 → 6H5/2 (ΔJ = 0), which is entirely MD in quality, is credited with producing the peak at 567 nm. Electronic transition 4G5/2 → 6H9/2 (ΔJ = ±2) is considered to be completely ED and is responsible for the peak at 651 nm. Nature forbids of the peak at 715 nm, which corresponds to 4G5/2 →6H11/2 (ΔJ = ±3).50 The local environment of the host matrix is extremely sensitive towards the ED transition. The Sm3+ ion occupies both symmetric and antisymmetric sites in the host lattice when the spectrum exhibits both the MD and ED transitions. The symmetry of Sm3+ ions in the coordination environment was assessed using the emission asymmetry ratio R = I(4G5/2 → 6H9/2)/I(4G5/2 →6H5/2) to explore the symmetry of the local environment.51 It was widely known that the Sm3+ ions would present at low-symmetric locations if the ED transition was higher than the MD transition. The integrated-intensity of ED is lesser than the MD-transition, as seen in Fig. 12. Therefore, the dopant Sm3+ ions occupied the higher symmetry positions with inversion centre in the GdSr2AlO5 crystal structure, as demonstrated by the R value which computed to be lower than one.
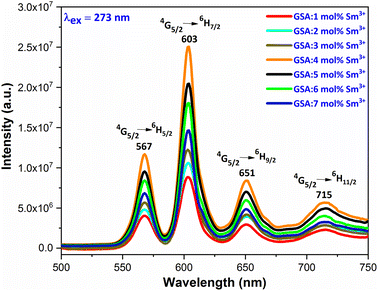 |
| Fig. 13 Emission spectra of Gd1−xSr2AlO5:xSm3+ (x = 1–7 mol% Sm3+) phosphors. | |
3.5.4 Concentration quenching mechanism. When a dopant concentration is high, the distance between them shortens, causing increased non-radiative energy loss and a steady fall in luminescence intensity. This is known as the concentration quenching phenomena. Under 273 nm excitation, the emission spectra of Sm3+-doped GdSr2AlO5 phosphors were perceived for a variation of Sm3+ concentrations (1–7 mol%), a comparable representation is shown in Fig. 14. Emission strength increases by up to 4 mol% as the Sm3+ ion concentration increases. Additionally, it drops for a small proportion due to the concentration quenching function. The historical profile (inset of Fig. 14) presents the intensity of the all synthesized samples. Thus, 4 mol percent is thought to be the optimal Sm3+ ion concentration. The non-radiative energy transfer process takes place when the distance between neighbouring Sm3+ ions is so short that only making contact with one another will cause this effect. According to Blasse's calculations, the increase in dopant ions density permits the distance between them to close, which may be advantageous for non radiative straits by promoting interchange of electrons (e−) between incoming ions rather than radiative-emanations. Concentration quenching refers to the slowing down of emission caused by non-radiative stimulation at high activator-ion concentrations. Remarkably, the critical distance i.e. RC between the nearby ions value is roughly measured via applying the following relation (eqn (6)).52 |
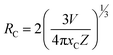 | (6) |
here V, xc & Z signifies the volume of the unit cell, optimized dopant concentration and the number of cationic sites offered for the dopant ions per unit cell, individually. For optimum sample, V = 525.948, Z = 4, and xc = 0.04, Rc was 18.45 Å which was greater than 5 Å. Elimination luminous effect can only be explained by multipolar interface since there is no convergence between the PLE and PL spectra in present context and the critical-gap is more than 5. To further authenticate the mechanism of energy transfer in Gd0.96Sr2AlO5:4 mol% Sm3+ nanophosphors, Dexter's formula is used (eqn (7))53 |
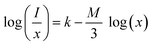 | (7) |
here, I signify the PL intensity at dopant concentration x, K and β are constants & M is the interactions type. M denotes the concentration quenching, which includes three different mechanisms: dipole–dipole, dipole-quadrupole, and quadrupole–quadrupole. These three mechanisms correspond to the values of M = 6, 8, and 10.54 According to Fig. 15, which shows a straight-line connection between log(I/x) and log(x) with a slope value of −1.886, and M value determined to be 5.658 (nearby to 6). The calculated value suggested that the primary mechanism of energy transfer in GdSr2AlO5:Sm3+ nanophosphors are dipolar–dipolar (d–d) interaction.
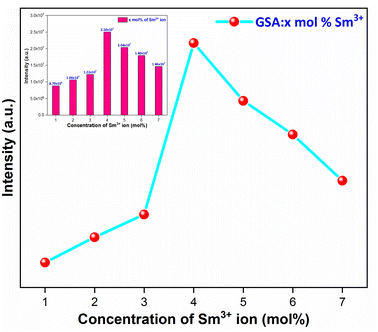 |
| Fig. 14 Relative PL intensity of Gd1−xSr2AlO5:xSm3+ (x = 1–7 mol% Sm3+) phosphors and inset represent the intensity of respective samples. | |
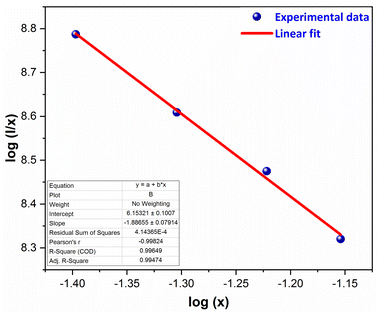 |
| Fig. 15 Straight-line fitting of log(I/x) vs. log(x). | |
3.6 Luminescence decay time
Fig. 16 displays the PL decay curve of Gd0.96Sr2AlO5:4 mol% Sm3+ powdered nanocrystalline material under excitation (Ex) and emission (Em) wavelengths of 273 and 603 nm. The Sm3+ ions are initially stimulated to higher excited states while monitored with excitation wavelength of 273 nm, and then subsequently relaxed to the ground state via relaxation or non-radiative energy transfer. The lifetime-curve is perfectly fitted through Bi-exponential approach followed by the eqn (8) (ref. 55) |
It = I0 + A1exp(−t/τ1) + A2 exp(−t/τ2)
| (8) |
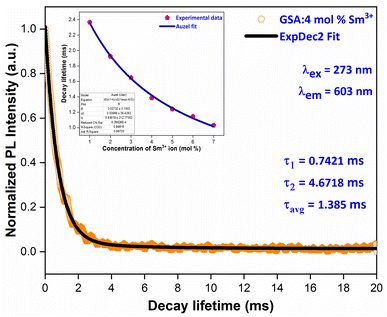 |
| Fig. 16 PL decay curve Gd0.96Sr2AlO5:4 mol% Sm3+ phosphors and inset represent the Auzel's fitting profile of all samples. | |
Among them, It correspond to the emission intensity at time = t, I0 is initial emission intensity, τ1 and τ2 refers to the decay lifetimes and A1 & A2 are their fitting constants. Average decay time (τavg) of Sm3+ ions can be determined by using following expression (eqn (9)).56
|
τavg = (A1t21 + A2t22)/(A1t1 + A2t2)
| (9) |
In
Table 6, the average lifespan values (avg) for all doped nanosamples are displayed. We can see that decay times diminish as Sm
3+ ion doping level raises. The ions originate near to one another and rapidly transfer energy as the content of activator ions rises, resulting in a new decay route with shorter decay duration. Inset of
Fig. 16 proves that the Auzel's fitting (
eqn (10)) way results in a
τc value of 3.027 ms.
57 |
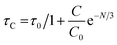 | (10) |
here,
τC = decay-time,
C0 = constant and
N tells about the phonon number. Also, quantum-efficiency (
η) of activated nanomaterials is assessed by the help of formulation given below (
eqn (11))
58
Table 6 Lifetime and quantum efficiency of GSA:xSm3+ (x = 1–7 mol% Sm3+) phosphors
Sample |
Decay-lifetime (ms) |
Quantum-efficiency (η%) |
GSA:1 mol% Sm3+ |
2.367 |
78.19 |
GSA:2 mol% Sm3+ |
1.924 |
63.56 |
GSA:3 mol% Sm3+ |
1.624 |
54.47 |
GSA:4 mol% Sm3+ |
1.385 |
45.75 |
GSA:5 mol% Sm3+ |
1.241 |
40.99 |
GSA:6 mol% Sm3+ |
1.147 |
37.89 |
GSA:7 mol% Sm3+ |
1.031 |
34.07 |
The obtained quantum efficiency (η) values of synthesized nanophosphors are listed in Table 6, which shows that η values are gradually declined with rise in the doping level of Sm3+ ions owed to the proportionate rise in the rate of radiation less energy transfer. The measured quantum efficiency is obtained within suitable range in the present case, suggesting that the processed phosphors are beneficial for LED applications.
3.7 Colorimetric investigation
To verify the color of the emitting visible radiation, CIE diagrams and coordinates were employed. Using Commission Internationale de I'Eclairage (CIE) 1931 standards, the samples CIE chromaticity values were key technical characteristics in the CIE 1931 chromaticity diagram. The Gd1−xSr2AlO5:xSm3+ (x = 1–7 mol%) phosphors chromaticity coordinates, as seen in Fig. 17, are placed near the reddish-orange color area, which marks the edge of the CIE 1931 chromaticity chart. Furthermore, while dealing with thin band emanation spectrums, the measurement of color purity (CP) is important. In this instance, we were able to observe the narrow band emission in the orange-reddish province at 603 nm. Therefore, using the following eqn (12), we assess the CP % of generated nanomaterials that produce orange–red color.59 |
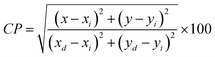 | (12) |
Here, (x, y) are CIE points, (xi, yi) are illuminate points and (xd, yd) are the dominating color-coordinates. The observed average CP value is 82.18%. Furthermore, because of CIE-points being at border of the CIE-chart, the CP value of 4 mol% activated powder is maximal (87.55%). When combined with other phosphor powders, the color characteristics of the generated powders show that these phosphors may be employed successfully in orange-reddish emanating devices and as prospective applicant in fabrication of wLEDs. One crucial luminous characteristic used to assess the performance of the phosphors is CCT. Typically, lights are regarded as producing warm light when their CCT was lower than 3000 K. The lights were classified as cool light even though their CCT was more than 4000 K. The McCamy empirical formulation (eqn (13)) served as a means of expressing the values of CCT.60 |
CCT = −437n3 + 3601n2 − 6861n + 5514.31
| (13) |
where, n = (x − xe)/(y − ye), (x, y) are product's chromaticity coordinates and (xe, ye) is the epicenter position with xe = 0.332 and ye = 0.186. The variation of CIE-point (x, y to u′, v′) to evaluate CCT values encompasses following calculations (eqn (14)).61 |
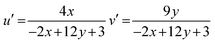 | (14) |
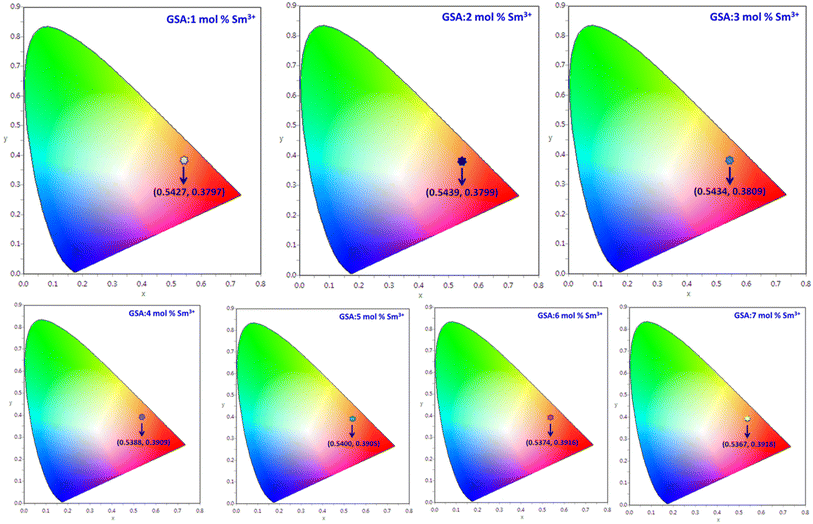 |
| Fig. 17 CIE coordinates of Gd1−xSr2AlO5:x mol% Sm3+ (1–7 mol%) phosphors. | |
The Gd1−xSr2AlO5:xSm3+ (x = 1–7 mol%) phosphor's u′, v′ along with their CCT values, are seen in Fig. 18. Table 7 lists all the CCT values along with their corresponding u′, v′, color purity and CIE color coordinates. It was also observed that the CCTs varied within a color space, with a minimum value of 1683 K and a high value of 1792 K. The low CCTs demonstrated that warm light LEDs have a promising future for using the phosphors in their as-prepared state.
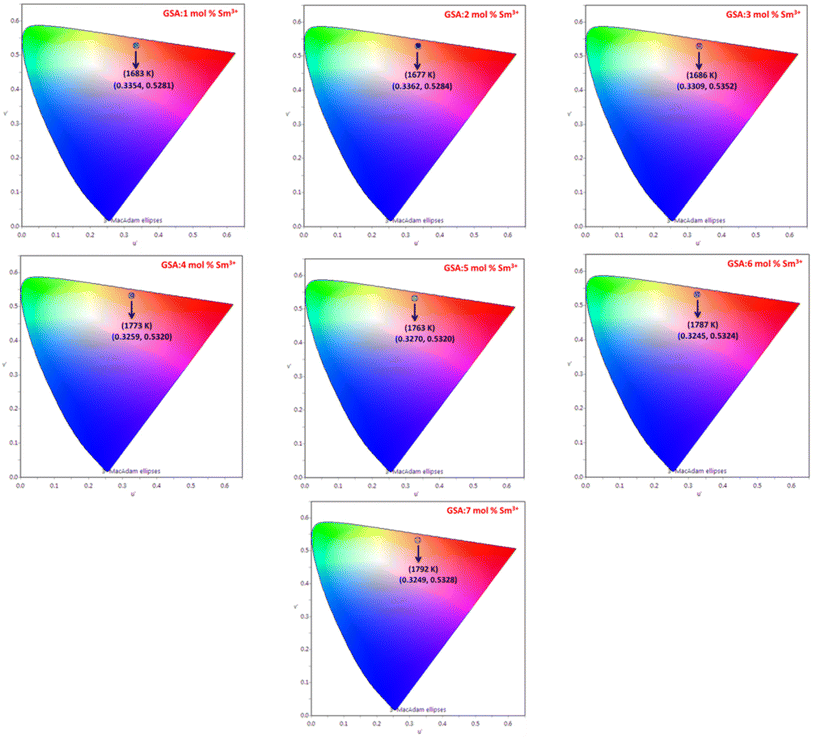 |
| Fig. 18 Representation of u′, v′ with CCT values of Gd1−xSr2AlO5:x mol% Sm3+ (1–7 mol%) phosphors. | |
Table 7 Chromaticity parameters of Gd1−xSr2AlO5:xSm3+ (x = 1–7 mol% Sm3+) nanophosphors
Sample |
(x, y) |
CP (%) |
(u′, v′) |
CCT (K) |
GSA:1 mol% Sm3+ |
0.5427, 0.3797 |
79.81 |
0.3354, 0.5281 |
1683 |
GSA:2 mol% Sm3+ |
0.5439, 0.3799 |
81.22 |
0.3362, 0.5284 |
1677 |
GSA:3 mol% Sm3+ |
0.5434, 0.3809 |
82.04 |
0.3309, 0.5352 |
1686 |
GSA:4 mol% Sm3+ |
0.5388, 0.3909 |
87.55 |
0.3259, 0.5320 |
1773 |
GSA:5 mol% Sm3+ |
0.5400, 0.3905 |
86.27 |
0.3270, 0.5320 |
1763 |
GSA:6 mol% Sm3+ |
0.5374, 0.3916 |
82.03 |
0.3245, 0.5324 |
1787 |
GSA:7 mol% Sm3+ |
0.5367, 0.3918 |
81.75 |
0.3249, 0.5328 |
1792 |
3.8 Thermal stability behavior
Thermal stability is closely related to the application of the nanophosphors. In order to explore the thermal stability of materials, the temperature-dependent emission spectra of Gd0.96Sr2AlO5:4 mol% Sm3+ were measured in the temperature range of 298–473 K with the interval of 25 K, as shown in Fig. 19. It is beneficial to have a comprehensive understanding of the thermal deterioration of the phosphors so as to improve their performance via material design. These emission spectra present almost same spectral distribution i.e. the shape and position of the observed peaks are almost similar, suggesting that this phosphor has excellent color stability. With the temperature increase, the emission intensities decrease gradually due to the well-known thermal quenching effect.62,63 Generally, with the increase of temperature, the probabilities of multiphonon relaxation and energy transfer for quenching the emitting levels may be enhanced, which are responsible for the decrease of luminescence intensity
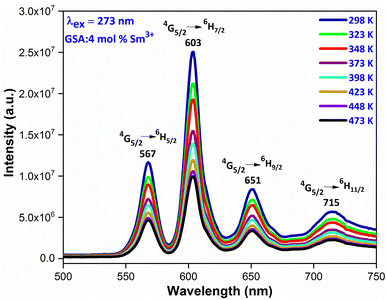 |
| Fig. 19 Temperature dependent emission spectra of GSA: 4 mol% Sm3+ nanophosphor in the temperature range of 298–473 K. | |
4 Conclusions
The extensive effort made to produce white light-emitting diodes (wLEDs), which have the ability to provide with enhanced photoluminescence (PL) capabilities, is an indication of modernity. The orange phosphor, one of the crucial elements needed for the mass manufacture of warm wLEDs. This research makes an effort to synthesize a series of Gd1−xSr2AlO5:xSm3+ (x = 1–7 mol%) phosphor via combustion route to address this problem.
The produced phosphors are in a single phase, according to XRD measurements. It found to be a pure tetragonal crystal structure with I4/mcm (140) space group symmetry. The 4G5/2 → 6H7/2 transition of Sm3+ ions which was exhibited by the synthesized phosphors excited at 273 nm is responsible for the vivid orange–red emission at 603 nm. The dipole–dipole (d–d) interaction is the major reason for the concentration quenching process, as determined by calculations (Rc = 18.45 and Q = 6) and investigation. According to calculations, the prepared crystalline nanophosphors having decay lifetimes ranged from 2.367 ms to 1.031 ms. The colorimetric examination i.e. chromaticity coordinates (CIE), low CCTs values and high color purity (CP) endorses their potential usages in the fabrication of warm wLEDs.
5 Credit author statement
Pawan Kumar: Data curation, writing – original draft, investigation, methodology, software; Devender Singh: Writing – review & editing, resources, supervision; Isha Gupta: visualization, validation.
Conflicts of interest
The authors declare that they have no known competing financial interests or personal relationships that could have appeared to influence the work reported in this paper.
Data availability
Data will be made available on request
Acknowledgements
The author (Pawan Kumar) is thankful to UGC-New Delhi for providing JRF [117/(CSIRNETJUNE2019)].
References
- Q. M. Le, T. H. Tran, T. H. Nguyen, T. K. Hoang, T. B. Nguyen, K. T. Do and N. A. T. Nguyen, Development of a fluorescent label tool based on lanthanide nanophosphors for viral biomedical application, Adv. Nat. Sci.: Nanosci. Nanotechnol., 2012, 3, 035003 Search PubMed.
- A. Bergh, G. Craford, A. Duggal and R. Haitz, The promise and challenge of solid-state lighting, Phys. Today, 2001, 54, 42–47 CrossRef CAS.
- I. Gupta, S. Singh, S. Bhagwan and D. Singh, Rare earth (RE) doped phosphors and their emerging applications: A review, Ceram. Int., 2021, 47, 19282–19303 CrossRef CAS.
- X. Li, W. Cai, H. Guan, S. Zhao, S. Cao, C. Chen and Z. Zang, Highly stable CsPbBr3 quantum dots by silica-coating and ligand modification for white light-emitting diodes and visible light communication, J. Chem. Eng., 2021, 419, 129551 CrossRef CAS.
- H. Guan, S. Zhao, H. Wang, D. Yan, M. Wang and Z. Zang, Room temperature synthesis of stable single silica-coated CsPbBr3 quantum dots combining tunable red emission of Ag–In–Zn–S for High-CRI white light-emitting diodes, Nano Energy, 2020, 67, 104279 CrossRef CAS.
- S. Itoh, M. Tanaka and T. Tonegawa, Development of field emission displays, J. Vac. Sci. Technol., B: Nanotechnol. Microelectron.: Mater., Process., Meas., Phenom., 2004, 22, 1362–1366 CrossRef CAS.
- P. Kumar, D. Singh, I. Gupta, S. Singh and V. Kumar, Structural and luminescent characteristics of orthorhombic GdAlO3:Sm3+ nanocrystalline materials for solid state lighting, Chem. Phys. Lett., 2022, 812, 140277 CrossRef.
- X. Li, W. Ma, D. Liang, W. Cai, S. Zhao and Z. Zang, High-performance CsPbBr3@ Cs4PbBr6/SiO2 nanocrystals via double coating layers for white light emission and visible light communication, eScience, 2022, 6, 646–654 CrossRef.
- R. Wang, H. Xiang, J. Chen, Y. Li, Y. Zhou, W. C. Choy, Z. Fan and H. Zeng, Energy regulation in White-Light-Emitting Diodes, ACS Energy Lett., 2022, 7, 2173–2188 CrossRef CAS.
- P. Kumar, S. Singh, I. Gupta, V. Kumar and D. Singh, Preparation and luminescence behaviour of perovskite LaAlO3:Tb3+ nanophosphors for innovative displays, Optik, 2022, 267, 169709 CrossRef CAS.
- D. Yan, S. Zhao, Y. Zhang, H. Wang and Z. Zang, Highly efficient emission and high-CRI warm white light-emitting diodes from ligand-modified CsPbBr3 quantum dots, Opto-Electron. Adv., 2022, 5, 200075 CAS.
- R. Sharma, R. Laishram, B. K. Gupta, R. Srivastva and O. P. Sinha, A review on MX2 (M= Mo, W and X= S, Se) layered material for opto-electronic devices, Adv. Nat. Sci.: Nanosci. Nanotechnol., 2022, 13, 023001 Search PubMed.
- I. Gupta, S. Singh, P. Kumar, S. Bhagwan, V. Kumar and D. Singh, Structural, morphological and optoelectronic aspects of YAlO3:Dy3+ doped nanocrystalline materials for NUV energized WLEDs, Curr. Appl. Phys., 2022, 42, 78–89 CrossRef.
- B. Karthikeyan and R. Vettumperumal, Optical and electrochemical properties of pure and Ti-doped CdO nanoparticles for device applications, Mater. Sci. Eng., B, 2022, 281, 115754 CrossRef CAS.
- L. Zhao, P. Xu, F. Fan, J. Yu, Y. Shang, Y. Li, L. Huang and R. Yu, Synthesis and photoluminescence properties of Sm3+ and Dy3+ ions activated double perovskite Sr2MgTeO6 phosphors, J. Lumin., 2019, 207, 520–525 CrossRef CAS.
- I. Gupta, D. Singh, S. Singh, P. Kumar, S. Bhagwan and V. Kumar, Structural and photophysical
measurements of Er3+ doped Gd4Al2O9 nanophosphors for NUV excitable solid-state lighting applications, Chem. Phys. Lett., 2023, 814, 140350 CrossRef CAS.
- D. N. Chung, D. N. Hieu, T. T. Thao, V. V. Truong and N. N. Dinh, Synthesis and characterization of Ce-doped Y3Al5O12 (YAG:Ce) nanopowders used for solid-state lighting, J. Nanomater., 2014, 2014, 1–7 CrossRef.
- C. Liu, S. Pokhrel, C. Tessarek, H. Li, M. Schowalter, A. Rosenauer and L. Mädler, Rare-earth-doped Y4Al2O9 nanoparticles for stable light-converting phosphors, ACS Appl. Nano Mater., 2019, 3, 699–710 CrossRef.
- S. R. Anishia, M. T. Jose, O. Annalakshmi and V. Ramasamy, Thermoluminescence properties of rare earth doped lithium magnesium borate phosphors, J. Lumin., 2011, 131, 2492–2498 CrossRef CAS.
- I. Gupta, D. Singh, S. Singh, P. Kumar, S. Bhagwan and V. Kumar, Phase recognition and spectroscopic characteristics of single-phase Tb3+ doped Gd4Al2O9 nanophosphors for NUV energized advanced photonic appliances, J. Lumin., 2022, 252, 119327 CrossRef CAS.
- Q. Mo, C. Chen, W. Cai, S. Zhao, D. Yan and Z. Zang, Room temperature synthesis of stable zirconia-coated CsPbBr3 nanocrystals for white light-emitting diodes and visible light communication, Laser Photonics Rev., 2021, 15, 2100278 CrossRef CAS.
- V. Tanwar, S. Singh, I. Gupta, P. Kumar, H. Kumar, B. Mari and D. Singh, Preparation and luminescence characterization of Eu (III)-activated Forsterite for optoelectronic applications, J. Mol. Struct., 2022, 1250, 131802 CrossRef CAS.
- A. Varma, A. S. Mukasyan, A. S. Rogachev and K. V. Manukyan, Solution combustion synthesis of nanoscale materials, Chem. Rev., 2016, 116, 14493–14586 CrossRef CAS PubMed.
- P. Kumar, D. Singh, I. Gupta, S. Singh, V. Kumar, H. Kumar and S. K. Chhikara, Perovskite GdAlO3:Dy3+ nanophosphors: A gel-combustion synthesis, phase evaluation and down conversion luminescent characteristics for lighting applications, J. Lumin., 2022, 252, 119409 CrossRef CAS.
- G. Zhang, Q. Qiang, S. Du and Y. Wang, An upconversion luminescence and temperature sensor based on Yb3+/Er3+ co-doped GdSr2AlO5, RSC Adv., 2018, 8, 9512–9518 RSC.
- W. T. Hong and H. K. Yang, Luminescence Properties of a Yellow GdSr2AlO5:Ce3+ Phosphor for a White LED, New Phys., 2015, 65, 1181–1186 CAS.
- I. Gupta, P. Kumar, S. Singh, S. Bhagwan, V. Kumar and D. Singh, Phase recognition, structural measurements and photoluminescence studies of reddish-orange-emissive YAlO3:Sm3+ perovskite nanophosphors for NUV energized WLEDs, J. Mol. Struct., 2022, 1267, 133567 CrossRef CAS.
- R. Amari, E. Benrezgua, B. Deghfel, Z. Abdelhalim, M. K. Yaakob, W. J. Basirun, A. Boukhari, S. Kheawhom and A. A. Mohamad, Ni doping effect on the electronic, structural and optical properties of ZnO nanoparticles prepared by Co-precipitation route, Opt. Mater., 2022, 128, 112398 CrossRef CAS.
- S. Singh and D. Singh, Down-conversion and structural characterizations of trivalent terbium- doped garnet nanocrystalline phosphors for lighting applications, J. Mater. Sci.: Mater. Electron., 2021, 32, 17674–17685 CrossRef CAS.
- I. Gupta, P. Kumar, S. Singh, S. Bhagwan, S. K. Chhikara and D. Singh, Crystal configuration, spectroscopic and optical characteristics of Er3+ doped YAlO3 perovskites for advanced photonic appliances, Inorg. Chim. Acta, 2022, 543, 121183 CrossRef CAS.
- S. Tajik and H. Beitollahi, Hydrothermal synthesis of CuFe2O4 nanoparticles for highly sensitive electrochemical detection of sunset yellow, Food Chem. Toxicol., 2022, 165, 113048 CrossRef CAS PubMed.
- A. Li, Z. Li, L. Pan, Z. Wang, W. Chen, Q. Shao and Y. Tang, Upconversion luminescent nanoheater based on NaGd(MoO4)2:Yb3+/Tm3+ nanocrystals: Surfactant-free solvothermal synthesis, upconversion photoluminescence and photothermal conversion, J. Alloys Compd., 2022, 904, 164087 CrossRef CAS.
- P. Kumar, D. Singh, I. Gupta, S. Singh and V. Kumar, Emerging green light emission of Er3+-activated single phased GdAlO3 phosphors for lighting applications, Luminescence, 2022, 37, 2028–2040 CrossRef CAS PubMed.
- P. Kumar, S. Singh, I. Gupta, K. Nehra, V. Kumar and D. Singh, Structural refinement and optical characteristics of single-phase Gd3Al5O12:Er3+ nanophosphors for luminescent applications, J. Lumin., 2022, 252, 119338 CrossRef CAS.
- D. Singh and S. Kadyan, Synthesis and optical characterization of trivalent europium doped M4Al2O9 (M= Y, Gd and La) nanomaterials for display applications, J. Mater. Sci.: Mater. Electron., 2017, 28, 11142–11150 CrossRef CAS.
- G. Li, Y. Wang, W. Zeng, W. Chen, S. Han and H. Guo, Luminescent and magnetic properties of the afterglow phosphors GdSr2AlO5: RE3+ (RE3+= Eu3+, Sm3+, Pr3+ and Dy3+), RSC Adv., 2015, 5, 20884–20889 RSC.
- P. Kumar, S. Singh, I. Gupta, V. Kumar and D. Singh, Luminous LaAlO3:Dy3+ perovskite nanomaterials: Synthesis, structural and luminescent characteristics for WLEDs, Luminescence, 2022, 37, 1932–1941 CrossRef CAS PubMed.
- G. U. Xiguang, F. U. Renli, W. Jiang, P. Zhang, T. A. N. G. Ye and A. CoŞGun, Photoluminescence properties of an orange-red LaSr2AlO5: Sm3+ phosphor prepared by the Pechini-type sol-gel process, J. Rare Earths, 2015, 33, 954–960 CrossRef.
- S. Singh, I. Gupta and D. Singh, Sm3+-activated YAG nanocrystals: Synthesis, structural and spectroscopic analysis for orange-red emitting LEDs, Optik, 2021, 238, 166482 CrossRef CAS.
- K. Li, H. Lian and R. V. Deuna, Site occupancy and photoluminescence properties of a novel deep-red-emitting phosphor NaMgGdTeO6:Mn4+ with perovskite structure for w-LEDs, J. Lumin., 2018, 198, 155–162 CrossRef CAS.
- U. Holzwarth and N. Gibson, The Scherrer equation versus the 'Debye-Scherrer equation', Nat. Nanotechnol., 2011, 6, 534 CrossRef CAS PubMed.
- S. Bathula, B. Gahtori, M. Jayasimhadri, S. K. Tripathy, K. Tyagi, A. K. Srivastava and A. Dhar, Microstructure and mechanical properties of thermoelectric nanostructured n-type silicon-germanium alloys synthesized employing spark plasma sintering, Appl. Phys. Lett., 2014, 105, 061902–061904 CrossRef.
- P. K. Jisha, S. C. Prashantha and H. Nagabhushana, Luminescent properties of Tb doped gadolinium aluminate nanophosphors for display and forensic applications, J. Sci.: Adv. Mater. Devices, 2017, 2, 437–444 Search PubMed.
- P. Kumar, D. Singh, I. Gupta, S. Singh, S. Nehra and R. Kumar, Realization of warm reddish-orange light emitter single phase Y4Al2O9: Sm3+ nanophosphors for indoor lighting applications, J. Lumin., 2023, 257, 119703 CrossRef CAS.
- P. K. Jisha, S. C. Prashantha and H. Nagabhushana, Luminescent properties of Tb doped gadolinium aluminate nanophosphors for display and forensic applications, J. Sci.: Adv. Mater. Devices, 2017, 2, 437–444 Search PubMed.
- P. Gupta, A. K. Bedyal, V. Kumar, Y. Khajuria, V. Sharma, O. M. Ntwaeaborwa and H. C. Swart, Energy transfer mechanism from Gd3+ to Sm3+ in K3Gd(PO4)2:Sm3+ phosphor, Mater. Res. Express, 2015, 2, 076202 CrossRef.
- P. Kumar, D. Singh, I. Gupta, S. Singh, V. Kumar, H. Kumar and S. K. Chhikara, Cool green light emitting GdAlO3:Tb3+ perovskite nanomaterials: Crystal structure and spectroscopic characteristics for advance display appliances, Inorg. Chem. Commun., 2022, 154, 110064 CrossRef.
- P. Kumar, S. Singh, I. Gupta, V. Kumar and D. Singh, Structural and optical characterization of trivalent samarium-activated LaAlO3 nanocrystalline materials for solid-state lighting, J. Mol. Struct., 2022, 1265, 133362 CrossRef CAS.
- P. Kumar, S. Singh, I. Gupta, A. Hooda, V. Kumar and D. Singh, Reddish-orange color tunable Sm3+ activated Gd3Al5O12 phosphors: Crystallographic and photophysical investigation for lighting applications, J. Mol. Struct., 2022, 1271, 134074 CrossRef.
- R. B. Basavaraj, S. Kumar, D. P. Aarti, G. Nagaraju, H. S. Kumar, R. Soundar and M. Shahsank, Color tunable orange-red light emitting Sm3+ doped BaZrO3 nanopowders: Photoluminescence properties for w-LED applications, Inorg. Chem. Commun., 2021, 128, 108577 CrossRef CAS.
- W. U. Khan, L. Zhou, X. Li, W. Zhou, D. Khan, S. I. Niaz and M. Wu, Single phase white LED phosphor Ca3YAl3B4O15: Ce3+, Tb3+, Sm3+ with superior performance: color-tunable and energy transfer study, Chem. Eng. J., 2021, 410, 128455 CrossRef.
- G. Blasse, Energy transfer in oxidic phosphors, Philips Res. Rep., 1969, 24, 131–144 CAS.
- I. Gupta, D. Singh, S. Singh, P. Kumar, S. Bhagwan and V. Kumar, Study of structural and spectroscopic characteristics of novel color tunable yellowish-white Dy3+ doped Gd4Al2O9 nanophosphors for NUV-based WLEDs, J. Mol. Struct., 2022, 1272, 134199 CrossRef.
- P. Kumar, S. Singh, I. Gupta, V. Kumar and D. Singh, Er3+-activated LaAlO3 perovskite phosphor: Crystal structure and down conversion photoluminescent behaviour for optoelectronic devices, Inorg. Chem. Commun., 2022, 1265, 109578 CrossRef.
- P. Kumar, S. Singh, I. Gupta, A. Dalal, V. Kumar and D. Singh, Preparation, structural and photometric properties of single-phased Gd3Al5O12:Tb3+ green-emitting phosphors for solid state lighting purpose, Mater. Sci. Eng., 2023, 288, 116189 CrossRef CAS.
- I. Gupta, S. Singh, P. Kumar, S. Bhagwan, V. Tanwar, S. Nehra and D. Singh, Synthetic, structural and optical characteristic of novel color tunable reddish-orange Gd4Al2O9: Sm3+ nanocrystalline materials for solid-state photonic appliances, Inorg. Chem. Commun. B., 2022, 148, 110332 CrossRef.
- P. Dang, G. Li, S. Liang, H. Lian and J. Lin, Multichannel photoluminescence tuning in Eu-doped apatite phosphors via coexisting cation substitution, energy transfer and valence mixing, J. Mater. Chem. C, 2019, 7, 5975–5987 RSC.
- P. Kumar, S. Singh, I. Gupta, K. Nehra, V. Kumar and D. Singh, Structural and luminescent behaviour of Dy(III) activated Gd3Al5O12 nanophosphors for white-LEDs applications, Mater. Chem. Phys., 2022, 295, 127035 CrossRef.
- K. Nehra, A. Dalal, A. Hooda, P. Kumar, D. Singh, S. Kumar, R. S. Malik and P. Kumar, Luminous terbium and samarium complexes with diacetylmethane and substituted 1,10-phenanthroline derivatives for display applications: Preparation and optoelectronic investigations, J. Lumin., 2022, 249, 119032 CrossRef CAS.
- A. Hooda, A. Dalal, K. Nehra, P. Kumar, D. Singh, S. Kumar, R. S. Malik, R. Kumar and P. Kumar, Mononuclear luminous β-diketonate Ln(III) complexes with heteroaromatic auxiliary ligands: synthesis and luminescence characteristics, Luminescence, 2022, 37, 1921–1931 CrossRef CAS PubMed.
- A. Hooda, A. Dalal, K. Nehra, P. Kumar, D. Singh, R. S. Malik and S. Kumar, Heteroleptic Eu(III) emissive complexes: Luminescent, optoelectronic and theoretical investigation, J. Lumin., 2022, 252, 119272 CrossRef CAS.
- L. Zhang, J. Che, Y. Ma, J. Wang, R. Kang, B. Deng and H. Geng, Luminescent and thermal properties of novel orange-red emitting Ca2MgTeO6:Sm3+ phosphors for white LEDs, J. Lumin., 2020, 225, 117374 CrossRef CAS.
- Y. Ma, S. Tang, C. Ji, D. Wu, S. Li, J. Xu and Y. Peng, Sm3+ doped novel Sr2Ga2GeO7 based high thermal stability red-emitting phosphors for efficient WLED, J. Lumin., 2022, 242, 118530 CrossRef CAS.
|
This journal is © The Royal Society of Chemistry 2023 |
Click here to see how this site uses Cookies. View our privacy policy here.