DOI:
10.1039/D3RA00997A
(Paper)
RSC Adv., 2023,
13, 12270-12275
Pillar[5]arene-based supramolecular pseudorotaxane polymer material for ultra-sensitive detection of Fe3+ and F−†
Received
13th February 2023
, Accepted 10th April 2023
First published on 19th April 2023
Abstract
Recent advancements in ultra-sensitive detection, particularly the Aggregation Induced Emission (AIE) materials, have demonstrated a promising detection method due to their low cost, real-time detection, and simplicity of operation. Here, coumarin functionalized pillar[5]arene (P5C) and bis-bromohexyl pillar[5]arene (DP5) were successfully combined to create a linear AIE supramolecular pseudorotaxane polymer (PCDP-G). The use of PCDP-G as a supramolecular AIE polymer material for recyclable ultra-sensitive Fe3+ and F− detection is an interesting application of the materials. According to measurements, the low detection limits of PCDP-G for Fe3+ and F− are 4.16 × 10−10 M and 6.8 × 10−10 M, respectively. The PCDP-G is also a very effective logic gate and a material for luminous displays.
Introduction
The significance of ultrasensitive response in biological, chemical, and environmental applications has recently attracted a lot of attention.1–5 Many techniques have been employed up to this point for the crucial guest detection. Liu et al.6 show an ultra-sensitive photodetector based on a graphene/monolayer MoS2 vertical heterostructure operating at room temperature, while Liao and colleagues7 present an orthogonal framework for cfDNA cancer monitoring via genome-wide mutational integration, enabling ultra-sensitive detection, overcoming the limitation of cfDNA abundance, and empowering treatment optimization in low-disease-burden oncology care. The development of an effective material for the ultrasensitive response to special guests is still a fascinating task, despite the abundance of approaches that have been described.
Pseudorotaxanes, a type of typical molecular machine built by interlocked molecules,8 have been greatly implied in drug delivery,9–11 conducting materials,12,13 artificial molecular machines,14 gene delivery,15,16 functional bioimaging,17 supra-amphiphiles,18,19 as well as functional supramolecular systems.20–23 The supramolecular polymers created from pseudorotaxanes can sense, process, and actuate responses to external change on their own because of the dynamic and reversible nature of noncovalent interactions.24,25 Due to this characteristic, supramolecular polymers have a considerable advantage over other materials when used as ultrasensitive response material.
Pillar[5]arenes, first reported by Ogoshi,26 have been the main body of the new-generation macrocyclic ring due to its sophisticated pillar structure with electron-rich cavities, ease of functionalization,27 and aggregation-inducing properties.28 Moreover, the pillar[5]arene group provides supramolecular systems with a variety of assembly-driving forces including C–H⋯π, π⋯π and cation⋯π interactions, which can be employed as the primary building block for creating stimuli-responsive supramolecular assembly systems.29–33 Nowadays, nonporous adaptive crystals,34–36 chemical sensors,37–40 catalysis,41 pseudorotaxanes,42,43 and supramolecular materials44,45 have all been made using new supramolecular systems based on functionalized pillar[5]arenes. The development of a novel supramolecular system based on pillar[5]arene for the ultra-sensitive detection of ions is thus of tremendous significance.
In this work, we rationally designed and synthesized a novel pillar[5]arene host P5C by joining a pillar[5]arene group and coumarin group via a hydrazide group in light of the aforementioned factors and our long-standing interest in supramolecular systems. A novel AIE bi-component supramolecular polypseudorotaxane polymer PCDP-G that was created from P5C and DP5 has the potential to be used as an ultra-sensitive luminous material. Our strategies are as follows. Firstly, pseudorotaxanes are formed by one side of the alkyl chain of DP5 being threaded into the pillar[5]arene cavities of P5C and the other being threaded into the pillar[5]arene cavity of DP5. Secondly, both the coumarin and pillar[5]arene moieties of P5C and DP5 could not only act as π–π interaction sites but also serve as fluorophores. As expected, P5C and DP5 could self-assemble into the stable supramolecular polymer PCDP-G. Interestingly, the polymer PCDP-G shows strong AIE properties and could ultra-sensitively detect Fe3+ and F−. In addition, the PCDP-G could serve as a fluorescent display material as well as an effective logical gateway.
Results and discussion
The synthetic details of pillar[5]arene P5C are shown in Scheme S1.† P5C and their intermediates have been characterized by 1H NMR, 13C NMR and ESI mass spectrometry (Fig. S1–S4, ESI†).
At first, the P5C and DP5 could self-assemble into a supramolecular pseudorotaxanes polymer in cyclohexanol solution (Table S1, ESI†). The lowest critical gelation concentration (CGC) is 10% (w/v, 10 mg mL−1 = 1%), and the higher gel–sol transition temperature (Tgel) is 58–60 °C. Interestingly, the mixture solution of P5C and DP5 in cyclohexanol showed negligible fluorescence (T > Tgel); with the temperature of the hot cyclohexanol solution cooling below the Tgel, the supramolecular pseudorotaxanes polymer PCDP-G showed strong yellow fluorescence at 365 nm and reached a steady state within three minutes (Fig. S5, ESI†). Meanwhile, the sol–gel transition process exhibits excellent circularity (Fig. S6, ESI†). These results indicated that the strong yellow fluorescence of PCDP-G was induced by aggregation-induced emission (AIE).46
The self-assembly mechanism of PCDP-G was carefully investigated by 1H NMR, 2D NOESY NMR and scanning electron microscopy (SEM). As shown in 1H NMR (Fig. 1 and S7, ESI†), the addition of 1 equiv. DP5 caused the proton signal peaks Ha, Hb, Hc on P5C and H1, H2, H3 on DP5 to show distinct down-field shifts, respectively, while the proton signal peak H4 on DP5 showed an up-field shifts, which implied that one side of the alkyl chain of DP5 partially threaded into the pillar[5]arene cavity of P5C and the other side threaded into the pillar[5]arene cavity of DP5. As shown in the 2D NOESY spectra (Fig. S8, ESI†), the correlation peaks A and B between the signals of proton H4 on DP5 with Ha,c on P5C and H1,2 on DP5, respectively, also evidenced the pseudorotaxanes formed. At the same time, the correlation peaks C of proton H3 with H1,2 on DP5 and D of proton Hb with Ha,c on P5C indicated that the pillar[5]arene groups of P5C and DP5 are self-assembled through C–H⋯π interactions, respectively. Moreover, the proton signal peaks Hi, Hj, Hk, Hl, Hm on P5C shifted to downfield also supported that the π⋯π interactions of coumarin moieties were present. The π⋯π interactions mechanism is also supported by the peak E correlations of Hf, Hm with Hi, Hl on P5C. SEM morphological features of PCDP showed a regular spherical morphology, which also supported the self-assemble process (Fig. S9, ESI†). Therefore, there are firstly formed the supramolecular pseudorotaxanes, and then self-assembly via C–H⋯π interactions of the pillar[5]arene groups on P5C and DP5, respectively, and π⋯π interactions of the coumarin group in the formation of supramolecular pseudorotaxanes in the system (Scheme 1).
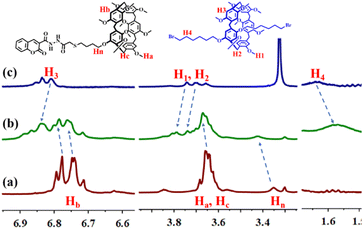 |
| Fig. 1 Partial concentration-dependent 1H NMR spectra (600 MHz, 298 K) in DMSO-d6: (a) free P5C 10 mg mL−1; (b) P5C 10 mg mL−1 and DP5 10.0 mg mL−1; (c) DP5 10.0 mg mL−1. | |
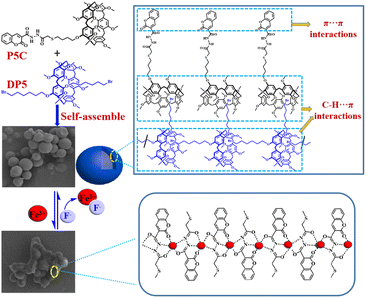 |
| Scheme 1 The chemical structures, and the self-assemble process of P5C and DP5 for the formation of the supramolecular polypseudorotaxane polymer PCDP-G as well as the mechanism of PCDP-G for fluorescence responses of Fe3+ and F−. | |
The fluorescence response abilities of PCDP-G toward cations were studied by diffusion with various cations: Cu2+, Co2+, Cd2+, Ni2+, Pb2+, Zn2+, Cr3+, Ca2+, Fe3+, Mg2+, Ba2+, Tb3+, Ag+, Hg2+ and La3+ (using their solid perchlorate salts as sources). After addition of above cations (water solution, 0.1 M), only Fe3+ caused the fluorescence of PCDP-G to quench, and other cations did not show a similar response (Fig. 2), which implied that PCDP-G could selectively detect Fe3+. The fluorescence titration of PCDP-G for Fe3+ was also carefully carried out. Only 0.009 equiv. Fe3+ (water solution, 0.1 M) can induce the fluorescence of PCDP-G to be quenched (Fig. S10, ESI†). Moreover, according to the fluorescence titration of PCDP-G for Fe3+ and calculations based on the 3σ/m method,47 the lowest detection of the fluorescence spectra change (LOD) of the PCDP-G for Fe3+ is 4.16 × 10−10 M, which indicated the PCDP-G could ultra-sensitively detect Fe3+ (Fig. S10 and S11, ESI†).
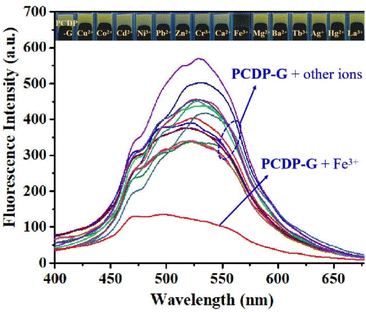 |
| Fig. 2 Fluorescence response of the PCDP-G upon addition of various mental aqueous solution (λex = 375 nm) in cyclohexanol system. | |
Furthermore, the successive response properties of the supramolecular metal polymer PCDP-GFe (PCDP-G containing 0.01 equiv. Fe3+) towards various anions were further discussed by adding aqueous solutions (C = 0.1 M) of various anions, including F−, Cl−, Br−, I−, ClO4−, AcO−, HSO4−, SCN−, CN− and N3− (for F−, Cl−, Br−, I−, HSO4−, AcO− and ClO4−, using their tetrabutylammonium (TBA) salts; for CN−, OH−, N3−, SCN− and S2−, using their sodium salts). As shown in Fig. 3, only F− induced the quenching of the fluorescence of PCDP-GFe changed to yellow, and other anions could not induce any change. These results indicated that PCDP-GFe could selectivity detect F− in water.
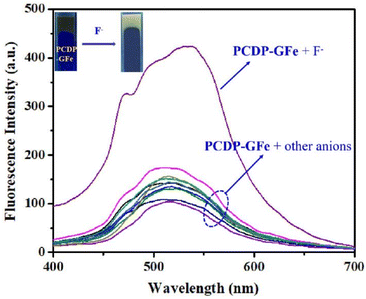 |
| Fig. 3 Fluorescence response of the PCDP-GFe upon addition of various anions aqueous solution (λex = 375 nm) in cyclohexanol system. | |
Then, in order to investigate the fluorescent response efficiency of the PCDP-GFe towards F−, we carried out fluorescence emission titration experiments (Fig. S12, ESI†). After the increasing concentration of F− was gradually added into the PCDP-GFe, the emission intensity of PCDP-GFe recovered. The limits of the lowest detection of the fluorescence spectra change calculated on the basis of 3δ/s method are 6.8 × 10−10 M (Fig. S13, ESI†), which indicated that the PCDP-GFe could be used for ultra-sensitive detection of F−. Based on the successive response properties of PCDP-G for Fe3+ and F−, we can see that the cyclic fluorescence detection was recyclable at least 3 times (Fig. S14, ESI†). These results illustrate that PCDP-G has higher sensitivity when compared with other fluorescence sensors for Fe3+ and F− (Tables S3 and S4, ESI†). Therefore, the supramolecular pseudorotaxanes polymer PCDP-G can be used for recyclable ultrasensitive detections of Fe3+ and F−.
The fluorescence response mechanisms of PCDP-G were carefully investigated via FT-IR and SEM. As shown in the FT-IR spectrum (Fig. 4), the stretching vibration peaks of –NH on PCDP-G appeared at 3419 cm−1. After addition of Fe3+, the stretching vibration peaks of –NH shifted to 3455 and 3293 cm−1, and the lactone carbonyl stretching vibration on coumarin moieties at 1722 cm−1 got shifted to a lower wave number at 1717 cm−1 upon complexation. The results are attributed to the oxygen atom and nitrogen atom in the acylhydrazone-based derivatives having strong coordination abilities with Fe3+ and the Fe3+ trigger amide tautomerization probably.48–50 The further addition of F− induced the stretching vibration peaks of –NH to shift to 3450 cm−1 and 3298 cm−1, and the stretching vibration peaks of C
O on coumarin returned to 1720 cm−1. These were attributed to the strong combination of F− with Fe3+.51 According to the SEM spectra, the regular spherical morphology of PCDP changed to the cross-linked spherical morphology by adding Fe3+ and subsequently changed to the dumbbell pattern. These results also support the above supposition. Therefore, according to these results, the proposed reversible Fe3+ and F− response mechanism is shown in Scheme 1, which is based on coordination interactions and competitive binding processes.
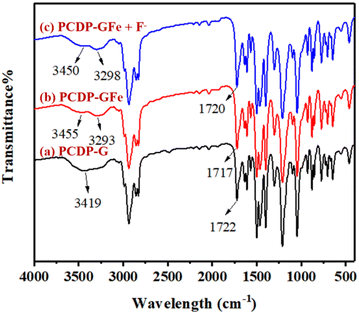 |
| Fig. 4 FT-IR spectra of CPDP-G, CPDP-GFe and CPDP-GFe + F− complex powdered in KBr disks. | |
As the PCDP-G could act as an Fe3+ and F− controlled “OFF-On-OFF” fluorescence response switch, this inspired us to apply it as a logic gate strategy for the detection of ions. Herein, the sensitization of PCDP-G luminescence enabled the design of an “IMPLICATION” logic gate. In this logic gate (Fig. 5a and b), the two inputs are Fe3+ and F−; their absence and presence are defined as “0” and “1”, respectively. The change in fluorescence intensity of PCDP-G acted as an output. The defined “1” and “0” represent the strong fluorescence intensity and weak fluorescence intensity, respectively. When there is no input (0, 0), the output is “1”. Similarly, only the Fe3+ input (1, 0) caused the fluorescence intensity to be extremely weak, and the output was “0”. With only F− input, there was a strong fluorescence intensity, and the output was “1”. When the system was input with Fe3+ and F− together (1, 0), the fluorescence intensity increased and gave an output signal of “1”. Therefore, this simple supramolecular polymer material was a potential candidate for the development of new generations of digital devices.
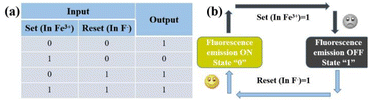 |
| Fig. 5 Implementation and truth table for IMP logic function using the supramolecular polymer PCDP-G. | |
Conclusions
In summary, a novel coumarin-functionalized pillar[5]arene derivative (P5C) was successfully synthesized. P5C and bis-bromohexyl pillar[5]arene (DP5) could form a linear AIE supramolecular poly-pseudorotaxane material (PCDP-G) by C–H⋯π and π⋯π stacking interactions, and the PCDP-G exhibits strong yellow aggregation-induced emission. Interestingly, PCDP-G can be used as an AIE supramolecular polymer material for recyclable ultra-sensitive detection of Fe3+ and F−. The low detection limits of PCDP-G for Fe3+ and F− were measured to be 4.16 × 10−10 M and 6.8 × 10−10 M, respectively. Moreover, the fluorescence changes of PCDP-G upon the addition of Fe3+ and F− were utilized as an “IMPLICATION” logic gate. The new strategy of preparing supramolecular polymer material with the yellow AIE effect for sensitive detection of Fe3+ and F− may stimulate the development of new approaches to fabricating functional supramolecular materials.
Conflicts of interest
There are no conflicts to declare.
Acknowledgements
This work was supported by the Young Doctor Fund Projects of Gansu, China (No 2021QB-119), the Doctoral Start-up Founds from Long Dong University (No XYBY202013), the Youth Science Foundation of Gansu province (No 21JR7RM194) and the Natural Science Foundation of Qingyang City (No QY2021A-F010).
Notes and references
- B. Tan, H. Zhao, W. Wu, X. Liu, Y. Zhang and X. Quan, Fe3O4-AuNPs anchored 2D metal-organic framework nanosheets with DNA regulated switchable peroxidase-like activity, Nanoscale, 2017, 9, 18699–18710 RSC.
- H. Li, D.-X. Chen, Y.-L. Sun, Y. B. Zheng, L.-L. Tan, P. S. Weiss and Y.-W. Yang, Viologen-Mediated Assembly of and Sensing with Carboxylatopillar[5]arene-Modified Gold Nanoparticles, J. Am. Chem. Soc., 2013, 135, 1570–1576 CrossRef CAS PubMed.
- M. Sun, R. Bai, X. Yang, J. Song, M. Qin, Z. Suo and X. He, Hydrogel Interferometry for Ultrasensitive and Highly Selective Chemical Detection, Adv. Mater., 2018, 30, 1804916 CrossRef PubMed.
- A. T. Aron, K. M. Ramos-Torres, J. A. Cotruvo Jr and C. J. Chang, Recognition- and Reactivity-Based Fluorescent Probes for Studying Transition Metal Signaling in Living Systems, Acc. Chem. Res., 2015, 48, 2434–2442 CrossRef CAS PubMed.
- L. Wang, N. Wang, J. Wen, Y. Jia, S. Pan, H. Xiong, Y. Tang, J. Wang, X. Yang, Y. Sun, Y. Chen and P. Wan, Ultrasensitive sensing of environmental nitroaromatic contaminants on nanocomposite of Prussian blue analogues cubes grown on glucose-derived porous carbon, J. Chem. Eng., 2020, 397, 125450 CrossRef CAS.
- Y. Liu, T. Gong, Y. Zheng, X. Wang, J. Xu, Q. Ai, J. Guo, W. Huang, S. Zhou, Z. Liu, Y. Lin, T.-L. Ren and B. Yu, Ultra-sensitive and plasmon-tunable graphene photodetectors for micro-spectrometry, Nanoscale, 2018, 10, 20013–20019 RSC.
- A. Zviran, R. C. Schulman and M. Shah, et al., Genome-wide cell-free DNA mutational integration enables ultra-sensitive cancer monitoring, Nat. Med., 2020, 26, 1114–1124 CrossRef CAS PubMed.
- J. E. M. Lewis, P. D. Beer, S. J. Loeb and S. M. Goldup, Metal ions in the synthesis of interlocked molecules and materials, Chem. Soc. Rev., 2017, 46, 2577–2591 RSC.
- S. Loethen, J.-M. Kim and D. H. Thompson, Biomedical Applications of Cyclodextrin Based Polyrotaxanes, Polym. Rev., 2007, 47, 383–418 CrossRef CAS.
- S. F. Taveira, A. Varela-Garcia, B. S. Souza, R. N. Marreto, M. Martin-Pastor, A. Concheiro and C. Alvarez-Lorenzo, Cyclodextrin-based poly(pseudo)rotaxanes for transdermal delivery of carvedilol, Carbohydr. Polym., 2018, 200, 278–288 CrossRef CAS PubMed.
- Z. Li, N. Song and Y.-W. Yang, Stimuli-Responsive Drug-Delivery Systems Based on Supramolecular Nanovalves, Matter, 2019, 1, 345–368 CrossRef.
- F. Cacialli, J. S. Wilson, J. J. Michels, C. Daniel, C. Silva, R. H. Friend, N. Severin, P. Samori, J. P. Rabe, M. J. O'Connell, P. N. Taylor and H. L. Anderson, Cyclodextrin-threaded conjugated polyrotaxanes as insulated molecular wires with reduced interstrand interactions, Nat. Mater., 2002, 1, 160–164 CrossRef CAS PubMed.
- L. Li, L. He, B. Wang, P. Ge, L. Jing, H. Liu, C. Gong, B. Zhang, J. Zhang and W. Bu, Secondary dialkylammonium salt/crown ether [2]pseudorotaxanes as nanostructured platforms for proton transport, Chem. Commun., 2018, 54, 8092–8095 RSC.
- G. Du, E. Moulin, N. Jouault, E. Buhler and N. Giuseppone, Muscle-like Supramolecular Polymers: Integrated Motion from Thousands of Molecular Machines, Angew. Chem., Int. Ed., 2012, 51, 12504–12508 CrossRef CAS PubMed.
- T. Ooya, H. S. Choi, A. Yamashita, N. Yui, Y. Sugaya, A. Kano, A. Maruyama, H. Akita, R. Ito, K. Kogure and H. Harashima, Biocleavable Polyrotaxane−Plasmid DNA Polyplex for Enhanced Gene Delivery, J. Am. Chem. Soc., 2006, 128, 3852–3853 CrossRef CAS PubMed.
- J. J. Li, F. Zhao and J. Li, Polyrotaxanes for applications in life science and biotechnology, J. Appl. Microbiol. Biotechnol., 2011, 90, 427–443 CrossRef CAS PubMed.
- S. S. Liow, H. Zhou, S. Sugiarto, S. Guo, M. L. S. Chalasani, N. K. Verma, J. Xu and X. J. Loh, Highly Efficient Supramolecular Aggregation-Induced Emission-Active Pseudorotaxane Luminogen for Functional Bioimaging, Biomacromolecules, 2017, 18(3), 886–897 CrossRef CAS PubMed.
- X. Chi, X. Ji, D. Xia and F. Huang, A Dual-Responsive Supra-Amphiphilic Polypseudorotaxane Constructed from a Water-Soluble Pillar[7]arene and an Azobenzene-Containing Random Copolymer, J. Am. Chem. Soc., 2015, 137, 1440–1443 CrossRef CAS PubMed.
- X. Chi, G. Yu, X. Ji, Y. Li, G. Tang and F. Huang, Redox-Responsive Amphiphilic Macromolecular [2]Pseudorotaxane Constructed from a Water-Soluble Pillar[5]arene and a Paraquat-Containing Homopolymer, ACS Macro Lett., 2015, 4, 996–999 CrossRef CAS PubMed.
- W. Zhu, W. Li, C. Wang, J. Cui, H. Yang, Y. Jiang and G. Li, CB[8]-based rotaxane as a useful platform for sensitive detection and discrimination of explosives, Chem. Sci., 2013, 4, 3583–3590 RSC.
- L. Ma, R. Tang, Y. Zhou, J. Bei, Y. Wang, T. Chen, C. Ou, Y. Han, C.-G. Yan and Y. Yao, Pillar[5]arene-based [1]rotaxanes with salicylaldimine as the stopper: synthesis, characterization and application in the fluorescence turn-on sensing of Zn2+ in water, Chem. Commun., 2022, 58, 8978–8981 RSC.
- Y. Shi, Z. Yang, H. Liu, Z. Li, Y. Tian and F. Wang, Mechanically Linked Poly[2]rotaxanes Constructed via the Hierarchical Self-Assembly Strategy, ACS Macro Lett., 2015, 4, 6–10 CrossRef CAS PubMed.
- Q. Shi, X. Wang, B. Liu, P. Qiao, J. Li and L. Wang, Macrocyclic host molecules with aromatic building blocks: the state of the art and progress, Chem. Commun., 2021, 57, 12379–12405 RSC.
- Z.-Y. Li, Y. Zhang, C.-W. Zhang, L.-J. Chen, C. Wang, H. Tan, Y. Yu, X. Li and H.-B. Yang, Cross-Linked Supramolecular Polymer Gels Constructed from Discrete Multi-pillar[5]arene Metallacycles and Their Multiple Stimuli-Responsive Behavior, J. Am. Chem. Soc., 2014, 136, 8577–8589 CrossRef CAS PubMed.
- K. Yang, Y. Pei, J. Wen and Z. Pei, Recent advances in pillar[n]arenes: synthesis and applications based on host-guest interactions, Chem. Commun., 2016, 52, 9316–9326 RSC.
- T. Ogoshi, S. Kanai, S. Fujinami, T. Yamagishi and Y. Nakamoto, para-Bridged Symmetrical Pillar[5]arenes: Their Lewis Acid Catalyzed Synthesis and Host-Guest Property, J. Am. Chem. Soc., 2008, 130, 5022–5023 CrossRef CAS PubMed.
- T. Ogoshi, T. Yamagishi and Y. Nakamoto, Pillar-Shaped Macrocyclic Hosts Pillar[n]arenes: New Key Players for Supramolecular Chemistry, Chem. Rev., 2016, 116, 7937–8002 CrossRef CAS PubMed.
- J.-F. Chen, G. Meng, Q. Zhu, S. Zhang and P. Chen, Pillar[5]arenes: a new class of AIEgen macrocycles used for luminescence sensing of Fe3+ ions, J. Mater. Chem. C, 2019, 7, 11747–11751 RSC.
- L. Chen, Y. Cai, W. Feng and L. Yuan, Pillararenes as macrocyclic hosts: a rising star inmetal ion separation, Chem. Commun., 2019, 55, 7883–7898 RSC.
- H. Zhang, Z. Liu and Y. Zhao, Pillararene-based self-assembled amphiphiles, Chem. Soc. Rev., 2018, 47, 5491–5528 RSC.
- Y. Wang, D. Wang, J. Wang, Ch. Wang, J. Wang, Y. Ding and Y. Yao, Pillar[5]arene-derived covalent organic materials with pre-encoded molecular recognition for targeted and synergistic cancer photo- and chemotherapy, Chem. Commun., 2022, 58, 1689–1692 RSC.
- J.-F. Chen, Q. Lin, Y.-M. Zhang, H. Yao and T.-B. Wei, Pillararene-based fluorescent chemosensors: recent advances and perspectives, Chem. Commun., 2017, 53, 13296–13311 RSC.
- H. Li, Y. Yang, F. Xu, T. Liang, H. Wen and W. Tian, Pillararene-based supramolecular polymers, Chem. Commun., 2019, 55, 271–285 RSC.
- Y. Zhou, K. Jie, R. Zhao, E. Li and F. Huang, Highly Selective Removal of Trace Isomers by Nonporous Adaptive Pillararene Crystals for Chlorobutane Purification, J. Am. Chem. Soc., 2020, 142(15), 6957–6961 CrossRef CAS PubMed.
- Y. Wu, J. Zhou, E. Li, M. Wang, K. Jie, H. Zhu and F. Huang, Selective Separation of Methylfuran and Dimethylfuran by Nonporous Adaptive Crystals of Pillararenes, J. Am. Chem. Soc., 2020, 142, 19722–19730 CrossRef CAS PubMed.
- X. Sheng, E. Li, Y. Zhou, R. Zhao, W. Zhu and F. Huang, Separation of 2-Chloropyridine/3-Chloropyridine by Nonporous Adaptive Crystals of Pillararenes with Different Substituents and Cavity Sizes, J. Am. Chem. Soc., 2020, 142, 6360–6364 CrossRef CAS PubMed.
- J. Wang, M. Cen, J. Wang, D. Wang, Y. Ding, G. Zhu, B. Lu, X. Yuan, Y. Wang and Y. Yao, Water-soluble pillar[4]arene[1]quinone: synthesis, host-guest property and application in the fluorescence turn-on sensing of ethylenediamine in aqueous solution, organic solvent and air, Chin. Chem. Lett., 2022, 33, 1475–1478 CrossRef CAS.
- C. Li, S. Chen, J. Li, K. Han, M. Xu, B. Hu, Y. Yu and X. Jia, Novel Neutral Guest Recognition and Interpenetrated Complex Formation from Pillar[5]arenes, Chem. Commun., 2011, 47, 11294–11296 RSC.
- X. Shu, J. Fan, J. Li, X. Wang, W. Chen, X. Jia and C. Li, Complexation of Neutral 1,4-Dihalobutanes with Simple Pillar[5]-arenes that is Dominated by Dispersion Forces, Org. Biomol. Chem., 2012, 10, 3393–3397 RSC.
- X. Shu, S. Chen, J. Li, Z. Chen, L. Weng, X. Jia and C. Li, Highly Effective Binding of Neutral Dinitriles by Simple Pillar[5]-arenes, Chem. Commun., 2012, 48, 2967–2969 RSC.
- C. Li, J. Ma, L. Zhao, Y. Zhang, Y. Yu, X. Shu, J. Li and X. Jia, Molecular Selective Binding of Basic Amino Acids by a Water-Soluble Pillar[5]arene, Chem. Commun., 2013, 49, 1924–1926 RSC.
- C. Li, L. Zhao, J. Li, X. Ding, S. Chen, Q. Zhang, Y. Yu and X. Jia, Self-assembly of [2]Pseudorotaxanes Based on Pillar[5]arene and Bis(imidazolium) Cations, Chem. Commun., 2010, 46, 9016–9018 RSC.
- S. Dong, B. Zheng, Y. Yao, C. Han, J. Yuan, M. Antonietti and F. Huang, LCST-Type Phase Behavior Induced by Pillar[5]arene/Ionic Liquid Host-Guest Complexation, Adv. Mater., 2013, 25, 6864–6867 CrossRef CAS PubMed.
- Q. Duan, Y. Cao, Y. Li, X. Hu, T. Xiao, C. Lin, Y. Pan and L. Wang, pH-Responsive Supramolecular Vesicles Based on WaterSoluble Pillar[6]arene and Ferrocene Derivative for Drug Delivery, J. Am. Chem. Soc., 2013, 135, 10542–10549 CrossRef CAS PubMed.
- Z.-D. Tang, X.-M. Sun, T.-T. Huang, J. Liu, B. Shi, H. Yao, Y.-M. Zhang, T.-B. Wei and Q. Lin, Pillar[n]arenes-based materials for detection and separation of pesticides, Chin. Chem. Lett., 2023, 34, 107698 CrossRef CAS.
- H. Su, W. Luo, J. W. Y. Lam, J. Qian, K. S. Wong and B. Z. Tang, Functionalized AIE nanoparticles with efficient deep-red emission, mitochondrial specificity, cancer cell selectivity and multiphoton susceptibility, Chem. Sci., 2017, 8, 4634–4643 RSC.
- H. N. Kim, W. X. Ren, J. S. Kim and J. Yoon, Fluorescent and colorimetric sensors for detection of lead, cadmium, and mercuryions, Chem. Soc. Rev., 2012, 8, 3210–3244 RSC.
- Z. Liao, Y. Liu, S.-F. Han, D. Wang, J.-Q. Zheng, X.-J. Zheng and L.-P. Jin, A novel acylhydrazone-based derivative as dual-mode chemosensor for Al3+, Zn2+ and Fe3+ and its applications in cell imaging, Sensor. Actuator. B, 2017, 244, 914–921 CrossRef CAS.
- V. Kumar, A. Kumar, U. Diwan and K. K. Upadhyay, A Zn2+-responsive highly sensitive fluorescent probe and 1D coordination polymer based on a coumarin platform, Dalton Trans., 2013, 42, 13078–13083 RSC.
- Š. Budzák and D. Jacquemin, Mechanism of fluorescence switching in one ESIPT-based Al3+ probe, J. Phys. Chem. B, 2016, 120, 6730–6738 CrossRef PubMed.
- W. Zhu, H. Fang, J.-X. He, W.-H. Jia, H. Yao, T.-B. Wei, Q. Lin and Y.-M. Zhang, Novel 2-(hydroxy)-naphthyl imino functionalized pillar[5]arene: a highly efficient supramolecular sensor for tandem fluorescence detection of Fe3+ and F- and the facile separation of Fe3+, New J. Chem., 2018, 42, 11548–11554 RSC.
Footnote |
† Electronic supplementary information (ESI) available: Experimental details, synthesis of P5C and DP5, 1H NMR, 13C NMR and MS spectra, and other materials. See DOI: https://doi.org/10.1039/d3ra00997a |
|
This journal is © The Royal Society of Chemistry 2023 |
Click here to see how this site uses Cookies. View our privacy policy here.