DOI:
10.1039/D3RA01052J
(Review Article)
RSC Adv., 2023,
13, 12438-12454
Implication of nanotechnology to reduce the environmental risks of waste associated with the COVID-19 pandemic
Received
15th February 2023
, Accepted 14th April 2023
First published on 20th April 2023
Abstract
The COVID-19 pandemic is the largest global public health outbreak in the 21st century so far. It has contributed to a significant increase in the generation of waste, particularly personal protective equipment and hazardous medical, as it can contribute to environmental pollution and expose individuals to various hazards. To minimize the risk of infection, the entire surrounding environment should be disinfected or neutralized regularly. Effective medical waste management can add value by reducing the spread of COVID-19 and increasing the recyclability of materials instead of sending them to landfill. Developing an antiviral coating for the surface of objects frequently used by the public could be a practical solution to prevent the spread of virus particles and the inactivation of virus transmission. Relying on an abundance of engineered materials identifiable by their useful physicochemical properties through versatile chemical functionalization, nanotechnology offers a number of approaches to address this emergency. Here, through a multidisciplinary perspective encompassing various fields such as virology, biology, medicine, engineering, chemistry, materials science, and computer science, we describe how nanotechnology-based strategies can support the fight against COVID-19 well as infectious diseases in general, including future pandemics. In this review, the design of the antiviral coating to combat the spread of COVID-19 was discussed, and technological attempts to minimize the coronavirus outbreak were highlighted.
Environmental significance
The COVID-19 pandemic has significantly impacted waste generation, particularly in the form of personal protective equipment. Proper management of such waste is essential to prevent the spread of infectious agents and protect public health. This review article examines the potential applications of nanotechnology to reduce the environmental risks of waste associated with the COVID-19 pandemic.
|
1 Introduction
Since the emergence of Coronavirus disease (COVID-19), the threat of waste pollution has grown exponentially, with strong attention on the environmental and human health consequences of millions of personal protective equipment (PPE) (e.g., face masks, shields, gloves, and wipes),1–3 and hazardous medical wastes (HMW) being used and discarded.4,5
Billions of PPE6 (e.g., face masks, shields, gloves, and wipes) and HMW (Table 1) are generated globally every day, and these wastes may eventually enter the environment via disposal in landfills or littering. Disposable PPE wastes are mainly made of plastic polymers such as polypropylene, polystyrene, polycarbonate, polyethylene, or polyester, although other types of fabrics, such as cotton, are also used. The mass consumption of PPE has brought about wide concern over the generation of a huge amount of plastic waste, which is likely to become a source of secondary microplastics (MPs, < 5 mm) or even nano plastics (NPs, < 1 μm). Hospitals and laboratories, in particular, have generated increased amounts of hazardous medical waste due to the increased use of PPE and other single-use items. Proper waste management is essential to prevent the spread of infectious agents and protect public health.7
Table 1 Hazardous COVID-19 pandemic health care waste
Hazard |
Example |
Chemical waste |
Laboratory reagents, film-developing reagents, solvents, expired/unused disinfectants, and waste containing heavy metals (batteries, broken thermometers, blood-pressure gauges, etc.) |
Infectious waste |
Materials contaminated with blood and body fluids, human excreta, laboratory cultures, and microbiological products PPE, i.e. boots, long-sleeved gowns, heavy-duty gloves, masks, goggles, and faces hields |
Radioactive waste |
Unused liquids from radiotherapy or laboratory research. Radioactive contaminated glassware, packages/absorbent paper, urine, and excreta from patients treated or tested with unsealed radionuclides also constitute radioactive waste |
Sharps waste |
Needles, auto-disable syringes, syringes with attached needles, infusion sets, scalpels, pipettes, knives, blades, and broken glasses |
Pharmaceutical waste |
Pharmacies, distribution centers, and hospital wastes |
Expired and contaminated pharmaceutical products |
Several risks are associated with the waste generated during the COVID-19 pandemic.4 One of the primary concerns is the potential for waste to contribute to land and water pollution. This can occur when waste is improperly disposed of, for example, discarded in streets or waterways, or when it is not adequately contained or treated during the disposal process. This can lead to the contamination of soil and water sources and can have serious consequences for the environment and human health. Another risk associated with COVID-19 waste is the potential for exposure to contaminated materials. This can be a concern for waste workers and individuals who may come into contact with discarded PPE or other potentially contaminated materials.8 For example, discarded gloves or face masks could be touched or handled by individuals who are not wearing gloves, potentially leading to the transmission of the virus. According to the World Health Organization (WHO), the improper disposal of PPE can lead to the contamination of soil and water, as well as the potential transmission of the virus to others through the handling of discarded materials.9
Overall, the risk of waste associated with the COVID-19 pandemic highlights the importance of robust systems for managing waste and the need for ongoing efforts to minimize this waste's environmental and health impacts.10,11 Proper disposal and handling of COVID-19 waste are essential to minimize these risks and protect both the environment and public health.4,5
Nanotechnology is a promising approach for addressing the waste management challenges associated with the COVID-19 pandemic.12 One example of a nanotechnology-based approach for waste management is using nanocomposites,13 which combine a matrix material with nanoscale fillers. These materials can potentially improve traditional materials' properties, such as increasing their strength or decreasing their weight. In the context of waste reduction, nanocomposites could be used to develop more durable products, such as masks and gloves, which would reduce the amount of waste generated. Nanocomposite can also be used to develop biodegradable plastics, which would help reduce the environmental impacts of plastic waste.14 Other potential applications of nanotechnology for waste management include using nanocatalysts to enhance the efficiency of waste treatment processes and using nanosensors to monitor the quality of recycled materials and improve the efficiency of recycling systems. Overall, using nanotechnology in waste management holds significant potential for reducing the environmental risks associated with the COVID-19 pandemic.15–17
A massive research effort has been launched to understand, characterize, and estimate the exposure risks of PPE and HMW-associated contaminants. While the number of studies examining the impacts of PPE is increasing, the emerging impacts of the COVID-19 pandemic on the environment remain unelucidated, which is a significant knowledge gap requiring further investigation.
According to SCOPUS data, there is increased growth in the number of publications in 2020–2022, demonstrating the broad interest given by the scientific community to waste associated with the COVID-19 pandemic. Moreover, few papers reported and discussed the nanotechnological approaches for waste management associated with the COVID-19 pandemic (Fig. 1).
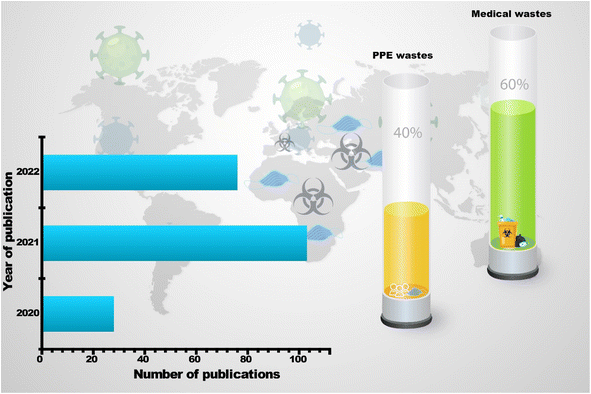 |
| Fig. 1 Number of publications related to waste associated with the COVID-19 pandemic between 2020 and 2022 (Scopus database 01-12-2022), and the percentage of each waste group. | |
In light of the current situation regarding Covid-19 disease, a discussion is proposed on the need for research focused on the presence and evolution of SARS-CoV-2 in water, soil, and other environmental compartments affected by the application of wastewater and sewage sludge. The evaluation of current wastewater and sewage sludge treatments, as well as the possible development of new specific techniques based on sorption, nanotechnology, etc., would also be of great interest for controlling the environmental spread of these viruses in current and future epidemics.
2 Implications of COVID-19 on waste generation
Nzediegwu used the population data and reported the estimated total daily face masks for Egypt (≈70.4 M), Morocco (≈37.8 M), Algeria (≈51.2 M), and Tunisia (≈13.2 M).18 While Benson et al. reported the estimated face mask disposal for Algeria (≈22.4 M), Egypt (≈30.8 M), Libya (≈3.8 M), Morocco (≈16.5 M), Sudan (≈10.7 M), and Tunisia (≈5.9 M).2 The General Authority for Statistics of Saudi Arabia forecasted daily mask use to be 33.5 M.7 A large cross-section study (n = 6770) performed in Hello the MENA region during April and May 2021 showed a significant increase in thrown PPE on streets, rivers, and lakes in urban areas.19 Surveying health care workers in Qatar (n = 1757) showed that 49.7% complied with PPE use.20 Similarly, (45.16%, n = 98) were satisfied with their PPE in a Kuwaiti study.21 A clean-up campaign in June–August 2020 in Jeddah, Saudi Arabia, resulted in the collection of 848 plastic wastes and PPE, representing 49% of the total plastic items. The results indicated the presence of 0.86 PPE item m−2 in Jeddah, and PPE was increased significantly (P < 0.001) by 48% on weekends. Also, Hassan et al. suggested the presence of 0.29 and 2.79 PPE item m−2 in Hurghada and Alexandria. Interestingly, PPE was increased significantly (P < 0.001) by 76.3% in Alexandria during the weekends, while the increase in Hurghada was insignificant (7%).22 In Morocco, a survey involving 185 individuals from Salé-Kénitra Casablanca-Settat and Rabat-regions revealed that 70% used their PPE once and dropped them in household waste or trash bins23 – results from Tighassaline and Khenifra cities were comparable as 87% of participants mixed PPE with household waste.3 The Mejjad et al. survey suggested using and disposing of five million face masks daily in Casablanca-Settat and Rabat-Salé-Kénitra, which is almost 35% of the total Moroccan-engendered face mask waste.23 Also, Haddad et al. collected 689 PPE (face masks, 96.81%) from the Agadir coastline in Morocco and showed an average density of 1.13 × 10−5 PPE m−2.6 The average daily PPE weight for five health care facilities in Bahrain was 1849.5 Most PPE in the Caspian Sea (Iran's coast) in Mazandaran province were face masks (95.3%). The average PPE density was 1.02 × 10−4 PPE m−2 (range, 0.00 to 7.16 × 10−4 PPE m−2).24
Additionally, Web of Science (a tool from Clarivate Analytics, 2022) was searched (September 03, 2022) using the keywords of “Medical wastes, Hazardous medical wastes, and Laboratories hazardous wastes” during the last twelve years from 2010 to 2021 (Fig. 2). In this regard, and as it is a serious environmental concern, several works have been carried out for MW safe treatment.10,25–30
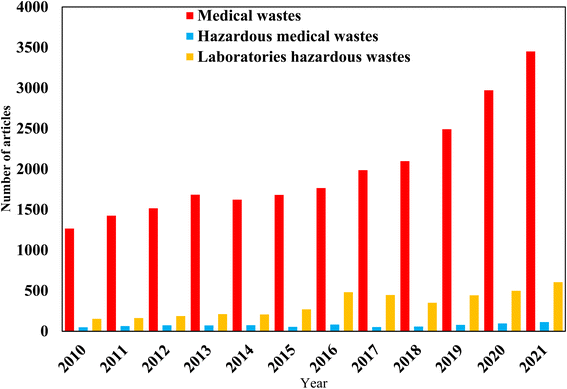 |
| Fig. 2 Literature on Hospital hazardous wastes, Hazardous medical wastes, and Laboratories hazardous wastes topics by web of science (a tool from Clarivate Analytics, 2020) since 2010, analyzed by years. | |
3 Risks of waste associated with COVID-19
Excessive PPE use has generated many contaminants (Fig. 3). Subsequently, all the wastes released are also likely to cause serious harm to the environment, animals, and human health (Fig. 4). Polypropylene is commonly used in N-95 masks, whereas Tyvek is used in medical face shields, suits, and protective gloves. Microplastics have a long life span and can pollute the environment with dioxin and other toxic substances.31,32
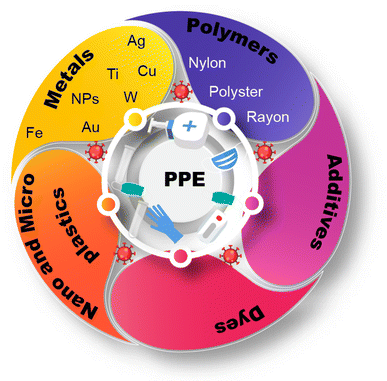 |
| Fig. 3 Contaminants associated with PPE. | |
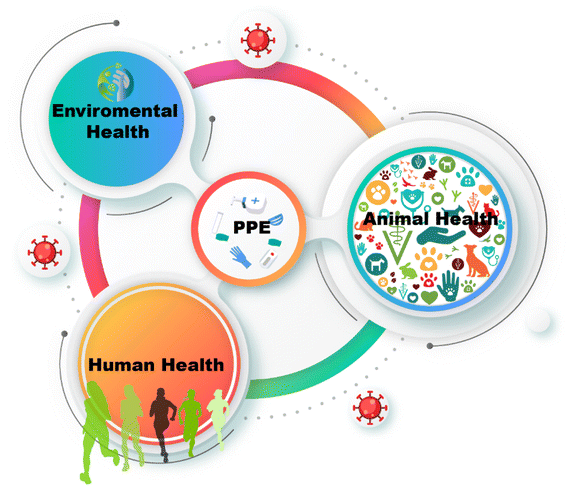 |
| Fig. 4 Potential effect of PPE on the environment, animal and human health. | |
Three layers make up surgical masks. The front and back are made of polypropylene and have fibres that are around 20 m in diameter.1,33 The core layer of surgical masks is made of melt-blown fabric, which is made from melt-blown fabrics made of polypropylene, one of the major components.34 In general, melt-blown fabrics exhibit high filtration performance, allowing them to eliminate bacteria, suspended particles, droplets and aerosols because their fiber diameters are approximately 1–5 m. The melt-blown filter, which is produced by conventional micro- and nanofiber manufacture, is the main filtering layer of the mask. Molten polymer is extruded via small nozzles with high-speed blowing gas in this process.35 As a result of using and reusing masks made from the aforementioned materials, microplastics and nano plastics can be produced.1,33 Breathing under these conditions can result in microplastic inhalation. It is also possible to breathe in microplastics while breathing the air36,37 (Fig. 5). Abbasi et al.38 detected 900 microplastics and 250 microrubbers of various sizes and shapes in 15 g of street dust collected in Iran.38
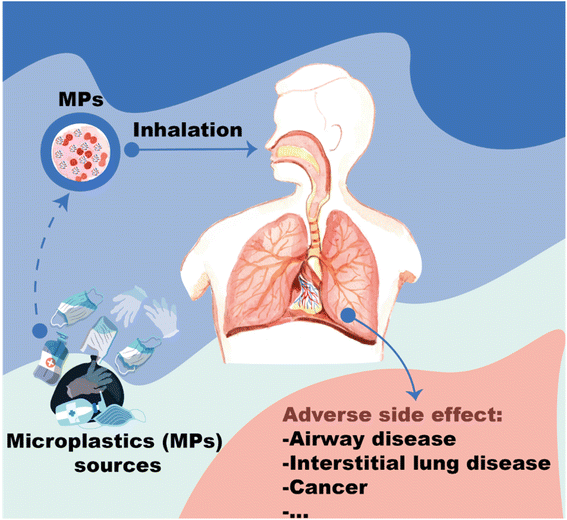 |
| Fig. 5 Risks of micro-plastics and nano-plastics on human health. | |
Lai et al.39 show that wearing a mask increases the danger of breathing microplastics, particularly microfibers.39 One should also consider inhaling microplastic from the air to protect human health. In this situation, masks serving as a barrier may help to minimize the amount of microplastic inhaled while breathing.40 Lai et al.39 proposed that even when masks are worn continuously for 720 hours, they greatly reduce the risk of breathing particles. Tables 2 and 3 show the total quantity of fiber-like microplastics and particles observed collected throughout the 720 hours breathing simulation test while wearing various types of masks.39
Table 2 The total quantity of fiber-like micro-plastics that were collected throughout the 720 hours breathing simulation test while wearing various types of masks
Time (h) |
No mask |
Surgical-A |
Surgical-B |
Fashion |
Non-woven |
Cotton |
Activated carbon |
N95 |
2 |
172 |
38 |
112 |
69 |
47 |
92 |
135 |
25 |
4 |
197 |
57 |
138 |
83 |
69 |
123 |
249 |
45 |
8 |
237 |
91 |
214 |
133 |
115 |
180 |
447 |
80 |
24 |
275 |
137 |
264 |
185 |
150 |
222 |
540 |
110 |
48 |
348 |
202 |
374 |
245 |
211 |
303 |
725 |
179 |
96 |
428 |
301 |
516 |
397 |
341 |
484 |
958 |
268 |
120 |
482 |
392 |
620 |
478 |
418 |
581 |
1120 |
308 |
168 |
643 |
515 |
780 |
654 |
540 |
741 |
1352 |
366 |
360 |
911 |
961 |
1556 |
1318 |
1026 |
1337 |
2086 |
719 |
720 |
1835 |
1913 |
3180 |
2576 |
2134 |
2824 |
3984 |
1521 |
Table 3 The total quantity of particles observed that were collected throughout the 720 hours breathing simulation test while wearing various types of masks
Time (h) |
No mask |
Surgical-A |
Surgical-B |
Fashion |
Non-woven |
Cotton |
Activated carbon |
N95 |
2 |
3918 |
1808 |
3090 |
3110 |
2152 |
2241 |
2212 |
1695 |
4 |
7946 |
2648 |
6568 |
6622 |
3612 |
3567 |
3417 |
2268 |
8 |
15.732 |
3797 |
12.625 |
12.158 |
6292 |
6963 |
6033 |
3290 |
24 |
39.700 |
6631 |
24.279 |
24.643 |
7814 |
12.848 |
9988 |
4678 |
48 |
92.236 |
10.495 |
42.119 |
47.722 |
14.598 |
26.690 |
16.174 |
6790 |
96 |
184.618 |
22.081 |
76.833 |
91.800 |
25.705 |
46.892 |
29.912 |
9294 |
120 |
212.994 |
26.585 |
99.487 |
109.986 |
29.269 |
55.932 |
34.814 |
10.810 |
168 |
249.114 |
36.953 |
128.054 |
145.374 |
36.423 |
72.006 |
47.028 |
12.660 |
360 |
562.842 |
71.545 |
268.897 |
295.832 |
85.664 |
158.660 |
92.453 |
23.265 |
720 |
1.121.316 |
140.069 |
523.791 |
597.980 |
169.316 |
302.242 |
181.017 |
44.853 |
Additionally, Fish and marine species exposed to treated wastewater have been found to have plasma levels of ketoprofen, ibuprofen, naproxen, and diclofenac.41 Spilled alcohol in water harms aquatic life, and spilled alcohol on soil can contaminate groundwater.31 Our water systems, soil, and biota will suffer long-term effects from this unwelcome set of events.32,42 Excessive use of soap as a preventive strategy for COVID-19 results in a massive concentration of soap in wastewater, which can harm aquatic plants. A 120 mg L−1 of soap can stop algal formation and proliferation. Plants like Potamogeton and Ranunculus aquatilis cannot flourish at detergent concentrations of 2.5 ppm.43 The soil quality may be affected by the accumulation of toxic chemicals in the soil brought on by heavy soap use. Domestic waste contaminates rivers, which contaminates lakes and oceans.42
It is worth mentioning that adequate waste management may solve occupational hazards to a large extent. Another way, such as incineration, is considered one of the available solutions and widely practiced medical waste disposal. Nevertheless, incineration of medical wastes releases pollutants into the air and ash residue. Also, the incineration of materials that contain heavy metals like lead, mercury, and cadmium caused an increment in their concentrations, while some materials that contain dioxins, furans, and metals accumulate in the environment. Thus, materials containing chlorine, dioxins, and furan or metals should not be incinerated because it considered human carcinogens44 (Fig. 6).
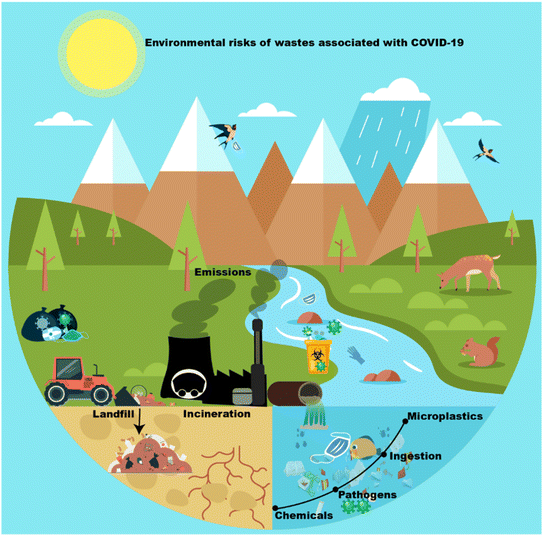 |
| Fig. 6 Environmental risks of wastes associated with COVID-19. | |
4 Waste management strategies during the COVID-19 pandemic
During COVID-19, environmental health awareness and waste management have become more prominent since generating gigantic and infectious health-care waste (HCW) quantities without proper treatment, control, and management could increase the widespread outbreaks probability of virus infection.45 The first and most important guideline provided by numerous member states of the EU, is increasing the capacity for each country to manage HCW due to the vast quantities of HCW during the pandemic. The HCW should be stored in sealed packages and containers in specially protected areas that only allow authorized personnel to enter. Disinfectants such as chlorine must be used on the outer and inner packages and containers to avoid the possible transmission of the virus. Furthermore, all the staff members, collectors, and authorized workers must be follows safety measures.46 Generally, lacking or incomplete, vague, and inconsistent information adds difficulties and uncertainty in waste management strategies decision-making.45 However, the challenges in underdeveloped African countries are even more significant due to less budget for WM compared to the other developed countries.47 Some improper WM strategies in some countries exacerbate the COVID-19 spread. India generates ≈517 tons of Biomedical Waste (BMW) per day, but only 501 tons per day can be treated in the 200 BMW treatment facilities spread over 28 states. While some states do not have these treatment BMW facilities at all.48 The garbage trucks in Tehran are principally equipped with compactors which facilitate the increase of waste collection. However, the high moisture content of the collected wastes generally results in overflowing the leachate from garbage trucks all over the road and in the landfill.46 In Tehran, followed the same WM strategies that were instructed by some cities in the USA during the COVID-19 outbreak, legal separation and recycling of municipal solid wastes have been completely stopped by the government due to concerns about the risk of COVID-19 spreading at transfer stations and recycling centers, while the illegal separation and recycling of wastes is still ongoing.46 Other governments, such as Italy, believe that waste separation is crucial during COVID-19 and that only households with infected individuals should be exempted. For example, Italy has only banned separating or recycling waste from infected residents.49
There are many reasons for the failure of adequate WM strategy in several countries:
• Inability to raise awareness about the health hazards related to HCW.
• Inappropriate training for adequate waste management.
• Absence of regular WM and disposal systems.
• Insufficient financial and human resources.
• Absence of proper regulations and rules in many countries or do not enforce them.
• Absence of surveillance system.
More attention and diligence should be focused on HCW management to overcome these challenges to avoid adverse health outcomes, including exposure to infectious agents and toxic substances associated with poor practice.
WHO has introduced the first universal and inclusive guidance document for safe WM from health care activities, including the regulatory framework, planning issues, handling, waste minimization, recycling, storage, transport, treatment, and disposal options.50 Strategies instructed by the WHO to improve HCW management:
• Supporting the practices that increase the amount of generated HCW and ensure proper waste separation.
• Improving systems and strategies as well as strong monitoring and regulation to progressively improve the practices of waste segregation and disposal practices to meet national and international standards and be easy to follow.
• Preferring the safe and environmentally sound treatment of hazardous health care wastes (i.e., by autoclaving, microwaving, steam treatment, and chemical treatment) over incineration. Also, selecting safe and environmentally friendly management options through all HCW management processes, to protect humans from hazards or danger when, handling, collecting transporting, storing, treating or disposing of waste.
• Building a comprehensive HCW management system, addressing responsibilities, resource allocation, handling, recycling, storage, transportation, treatment, and disposal. This is a long-term process, sustained by gradual improvements from the government's commitment; raise of consciousness about the risks associated with health care waste and safe handling practices.
5 Proposed approaches for waste management associated with the COVID-19 pandemic: nanotechnological solutions
The world is facing the current COVID-19 pandemic, which points to the urgent need to develop new medical innovations, technologies, or innovative strategies to fight COVID-19 infection and adapted solutions to manage the waste generation due to the coronavirus pandemic.2 The coronavirus infection route is still poorly understood and appears to be spread by air, surface, and water. Controlling the ways of surface transmission of coronavirus may be promising in reducing the spread of the infections in public, indoor, and outdoor environments.15,51 Developing antiviral coatings based on nanotechnological approaches may be one of the most prominent ways to counter viral transmission.52–59 It could be a potential strategy for practical applications, not only in hospitals for clinical purposes but also to control the spread of coronaviruses and inactivate any possible contamination.14 Accordingly, the entire surrounding environment must be disinfected at all times.9 To achieve this, nanomaterials with excellent antiviral properties should be sought that are cost-effective, environmentally friendly, and practically applicable.51
This section discusses several approaches to waste management associated with COV-19, based on nanotechnology solutions.
5.1 Antiviral coatings
Nanomaterial-based antiviral surfaces or coatings that can work effectively in both indoor and outdoor environments might be more prospective for such purposes, as the infectious viruses are located in close proximity to patients in hospitals at home or in common public areas. In this regard, photocatalytic surfaces could be more promotive as they permanently inactivate, oxidize and destroy micro-organisms such us viruses and bacteria under normal ambient lighting conditions, i.e., they are also effective in an indoor environment.13,52,60,61 Recent studies point to the tremendous potential of photocatalytic material surfaces-based nanomaterials for the inactivation of coronaviruses' (Table 4).60,62
Table 4 Antiviral coatings for COVID-19 degradation
Materials |
Outcomes |
Reference |
TiO2/Ti photocatalyst coating |
Significant antiviral activity, with a decrease rate of virus reached 99.96% for influenza virus and 99.99% for SARS-CoV-2 |
71 |
water-borne polymer coating |
SARS-CoV-2 variants inactivation within 30 minutes of exposure |
88 |
Ag–TiO2 nanocomposite coatings on ceramic tiles |
Inactivate SARS-CoV-2 under ambient indoor lighting, with an 87% reduction in titers at 1 h and total loss at 5 h of exposure |
67 |
Titanium-based structures are among the most often used photocatalysts due to their high photo-oxidation of organic compounds, superior chemical stability, high oxidizing power under UV light, and outstanding chemical resistance and photostability. TiO2 has also been shown to have the ability to eradicate both Gram-positive and Gram-negative bacteria, as well as different viral types and parasites.58
TiO2 has been the focus of much interest these last years in the domain of microbial remediation by photocatalysis due to its potent photocatalytic antimicrobial action against various types of microbes. Under exposure to oxygen and UV light, water decomposes on the surface of TiO2 into ROS which act as oxidizing or reducing agents causing the decomposition of organic and microbial materials. In addition, TiO2 has proven to be very useful in visible light activity in both indoor and outdoor applications for environmental disinfection. This photocatalytic disinfection effect has also shown great promise in controlling various viruses as an antiviral photocatalyst.63 The ROS formed at the surface of TiO2 (˙OH and O2−˙) due to UV activation, have good performance to degrade the capsid and or the envelope proteins of non-enveloped viruses as well as the phospholipids of the enveloped ones.64 In addition, due to the resulting leaching and degradation of nucleic acids, the virus particles eventually become inactive, as shown in Fig. 7.
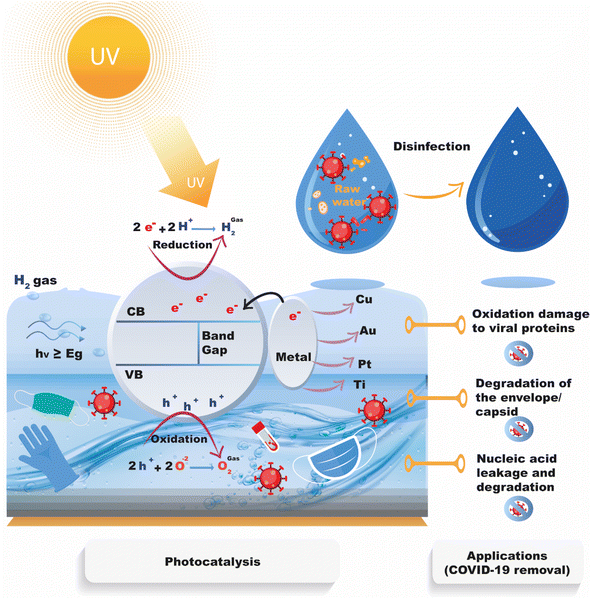 |
| Fig. 7 Mechanisms of photocatalytic inactivation of coronavirus for water treatment application. | |
Former studies demonstrated that the illumination of TiO2-based photocatalysts allow to obtain high added value oxidizing/reducing free radicals with outstanding antiviral action against various viruses such as the COVID-19 virus which is aerosol transmitted and leads respiratory tract infection.65,66 Evidence shows that TiO2-based nanomaterials have potential properties to combat COVID-19.66–68 Recently, Khaiboullina et al.66 proved that a photosensitive coating based on TiO2 NPs under exposure to UV light may be employed as an effective way against the spread infection by COVID-19 substitutes and could play a major role in the destruction of viral particles. Using the same photocatalyst material Micochova et al.67 investigated the stability of SARS-CoV-2 virus using thin Ag–TiO2 nanocomposite coatings on ceramic tiles under ambient light conditions. The viral titer was reduced by 4 orders of magnitude, reaching a complete inactivation after 20 minutes of light exposure and no detectable active virus was found after 5 hours of treatment. Importantly, SARS-CoV-2 on uncoated tiles was fully infectious after 5 hours of virus's addition. Overall, tiles coated 120 days earlier were able to inactivate SARS-CoV-2 under ambient indoor lighting, with an 87% reduction in titers at 1 h and total loss at 5 h of exposure.
Mathur et al.69 provided evidence regarding the UV destruction mechanism of TiO2 that can provide effective results in air purification by destroying or inactivation of various microorganisms such as bacteria and pathogenic viruses. It was suggested that this model could be promising for various air conditioning systems to make free cabin from bacterial and viral infections. Recently Uppal et al.70 proposed TiO2 photocatalytic coating for virucidal activity against HCoV-OC43 virus which is a member of beta coronavirus family just like SARS CoV-2 under the influence of UV irradiation. It was found that the TiO2-coated glass surface exhibited good antiviral response against the virus compared to uncoated glass using T-qPCR and virus infectivity tests. The results show a strong reduction in viral RNA copies and viral infectivity with increasing exposure time, reaching complete disinfection within 60 minutes of UV exposure.
Various TiO2 nanostructures such as nanotubes, nanoparticles, and nanowires, have also been researched and developed to enhance antibacterial and antiviral photocatalysis to inactivate viruses, including the COVID-19 virus, with high efficiency. Negrete et al.62 demonstrated an excellent photocatalytic effect of Ag–TiO2 nanomaterials to eliminate SARS-COV-2 after exposure to UV light for 9 h. Similarly, Hamza et al.68 investigated the effect of TiO2 NTs for disinfection of SARS-CoV-2 which showed strong anti-SARS-CoV-2 activity at very low cytotoxic concentrations in vitro as well as excellent antiviral activity at a very low concentration (IC50 = 568 ng mL−1) showing the relevance of TiO2 nanostructures as a coating nanomaterial with potent disinfectant properties to combat SARS-CoV-2. Interestingly, Matsuura et al.65 evidenced that TiO2 coating could be effective in inactivating SARS-CoV-2 via time-dependent TiO2-mediated photocatalytic reactions. From the findings, the photocatalytic reaction induced by TiO2 nanostructures showed good results in removing SARS-CoV-2 from contaminated water. Recently, Lu et al.71 developed a TiO2 photocatalyst coating on aluminium oxide (Al2O3) balls that may successfully inactivate influenza and SARS-CoV-2 viruses. The coating was discovered to be non-toxic and chemically stable, and it may be applied in working environments without requiring people to evacuate. The coating, according to the researchers, might be employed in air filtration systems to help reduce the spread of airborne viruses. According to the findings, photoelectrochemical oxidation-aided air purifiers might be useful instruments for reducing indoor SARS-CoV-2 exposure. Because of their strong decomposition function for C2H4O and CH2O, the TiO2/Ti photocatalyst coatings were also shown to be useful for environmental purification. Importantly, TiO2/Ti photocatalyst coatings are capable of considerable viral inactivation, with 99.96% inactivation for influenza virus and 99.99% inactivation for novel coronavirus (Fig. 8).
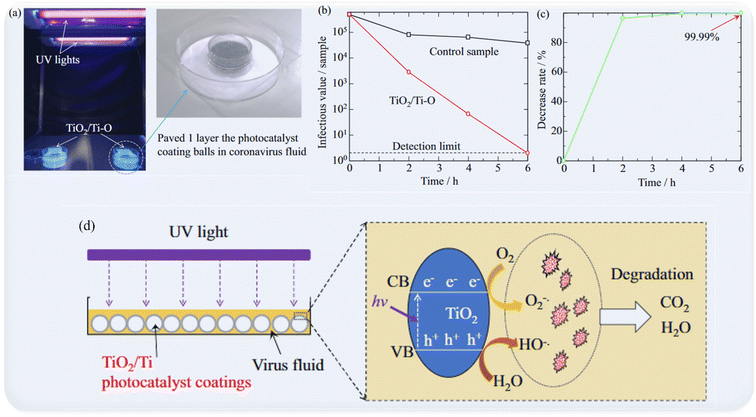 |
| Fig. 8 The inactivation test for SARS-CoV-2 of the TiO2/Ti–O sample. (a) The set-up, (b) the infectious value change of SARS-CoV-2, (c) the decrease rate of SARS-CoV-2, (d) proposed mechanisms of viral inactivation induced by TiO2/Ti photocatalyst coatings. Adapted from https://www.nature.com/articles/s41598-022-20459-2. | |
Various metal, nanocomposite, and semiconductor materials have been utilized to engineer antibacterial as well as antiviral coating surfaces for the deactivation of viruses or bacteria.57,72–83 Likewise, diverse surfaces-based nanomaterials consisting of metals, semiconductors, and alloys have been applied to fight COVID-19.52,53,58,84–87 Versatile nanomaterial-based antiviral surfaces or coatings that can work effectively outdoors and indoors would be more promising in this regard, as the most infectious viruses are found close to patients in hospitals or in their homes. In this sense, nano-adsorbents for COVID-19 removal, such as aerogel, graphene oxide, hydrogel, carbon-based nanomaterials, and metallic adsorbents (Fig. 9), might be more promising because they remove viruses permanently and induce their inactivation from the environment. Metal nanoparticles and ionic species were discovered as potential materials to fight COVID virus.58 The interaction mechanisms between nanoparticles and COVID-19 can be divided into two main groups. In indirect interactions, nanoparticles do not directly affect viruses. However, they enhance the antiviral activity of the drug. An indirect mechanism uses nanoparticles to deliver antiviral agents to increase stability and improve bioavailability.58 Recently, graphene was used as a nano-adsorbent and developed a self-cleaning mask using a laser-induced dual-mode forward transfer method to deposit several graphene layers on a non-woven mask. Super hydrophobicity is confirmed on the graphene-coated mask surface, and incoming water droplets bounce off the mask surface. Since the surface temperature of the mask rapidly reaches 80 °C or higher in direct sunlight, the mask can be reused by sterilizing it in the sun. While ordinary masks absorb only a small amount of sunlight, graphene-coated masks absorb over 95% of the entire sunlight spectrum from 300 to 2500 nm. As SARS-CoV-2 is heat sensitive, the development of graphene-coated photo-thermal masks with promising self-sterilization capabilities holds great promise for large-scale production of effective PPE to combat coronavirus.87
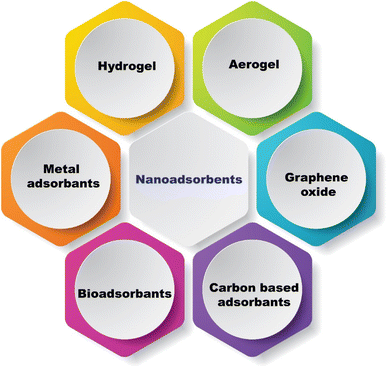 |
| Fig. 9 Nano-adsorbants for COVID 19 removal. | |
Recently, polymeric coatings have been investigated to combat COVID 19. In this regard, Bobrin et al.88 designed an environmentally friendly polymeric water-borne nanoworms coating that can completely inactivate the highly mutated variants of SARS-CoV of concern: Alpha, Delta and Omicron, independent of the infectious variant (Fig. 10). The coating can be applied to masks and many other surfaces to capture and inactivate the virus, helping to reduce the transmission of SARS-CoV-2 and the evolution of new variants of concern. The polymer was developed to target the highly glycosylated spike protein on the surface of the virion and to inactivate the virion through nanomechanical disruption of the viral membrane. The results reveal that even with minimal levels of surface coating (1 g m−2), alpha, delta, and omicron viruses were completely inactivated, and their viral genome was degraded. In addition, the results reveal that the polymer causes little or no skin sensitization in mice and is non-toxic when consumed orally in rats.
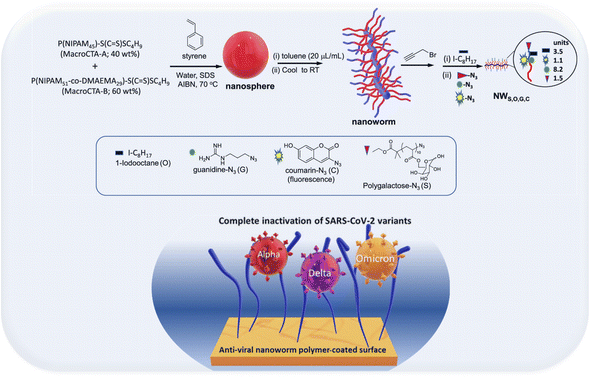 |
| Fig. 10 Synthesis of the functional nanoworms through the Environmentally Friendly Emulsion Polymerization Method and Post-Modification with Chemically Functional Groups for Capturing (Polygalactose) and Rupturing (Octane Groups) of SARS-CoV-2, Degradation of its mRNA (Guanidine Groups), and Detection of Polymer Coating (Coumarin Groups) adapted from Surface Inactivation of Highly Mutated SARS-CoV-2 Variants of Concern: Alpha, Delta, and Omicron|Biomacromolecules (https://acs.org). | |
5.2 Nanofiltration solutions
The most common pathway of transmission of respiratory diseases is by direct contact or indirect exposure to particles and droplets containing viruses.89 Effective elimination of these droplets is achieved through antiviral nanofiltration (Table 5, and Fig. 11). Recently, Issman et al.90 proposed an antiviral air filter using CNTs, which are mechanically reinforced by permeable and porous polyester with approximately 100 mm holes to improve filtration efficiency and structural stability. For this purpose, through an easy and continuous procedure, CNT aerogels were spun onto the support, and a bilayer hybrid is formed, a thin CNT membrane on a 0.4 mm thick, porous polyester. The prepared air filter showed 99.9% filtration efficiency. The electrically conductive feature of this air filter can be instantly heated to 130 °C in seconds for complete inactivation or removal of viruses on the filter surfaces, such as beta-coronavirus.90 Given the rapid spread of the Delta and Omicron variants of COVID-19, which pose a significant global public health risk, Cano-Vicent and collaborators investigated the cytotoxicity and antiviral action of calcium alginate films to inactivate COVID-19 enveloped virus.12 Calcium alginate biomaterial films demonstrated no cytotoxicity effects on human keratinocytes showing good antiviral performance against enveloped viruses, including Delta variant of SARS-CoV-2 and the bacteriophage phi 6, with 96, and 94% inactivation, respectively. The high antiviral effectiveness was related to the negative charge density of the alginate polymer network that binds to viral envelopes by inactivating their membrane receptors.
Table 5 Nano-filters for virus removal
Materials |
Outcomes |
References |
Alginate/copper sulfate |
Effective against COVID-19 by 99.99% |
91 |
Low cytotoxicity |
Polypropylene/tannic acid |
High-performance filtration (up to 2730 pfu mm−2) |
92 |
Speedy filtration process (10 minutes) |
Cellulose fibers modified multi-walled carbon nanotubes and phenol-formaldehyde |
0.64% of filtration efficiency |
93 |
High air permeability |
CNT on porous polyester |
99.999% of filtration efficiency |
90 |
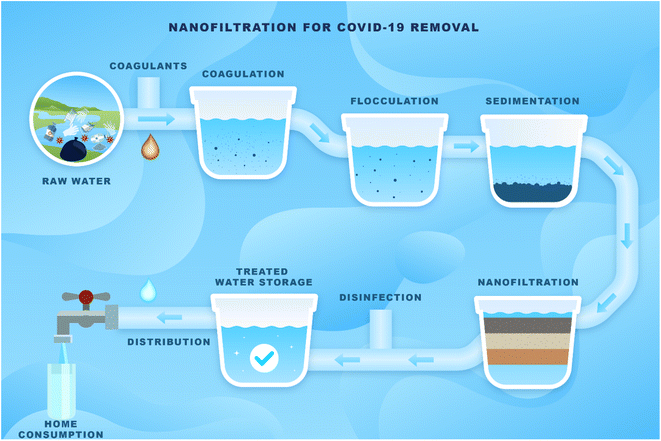 |
| Fig. 11 Nanofiltration solution for COVID-19 removal. | |
An essential strategy for preventing coronavirus high spread is the use of masks. However, masks quickly become contaminated after use. Bataglioli et al.91 designed a coating textil surface based on copper sulfate/alginate on disposable masks for COVID-19 inactivation. The advantages of the developed new material are the use of inexpensive materials, processed using simple and affordable methods to allow easy scaling of an effective antiviral coating. The designed disposable face masks demonstrated an efficacy of 99% against coronaviruses, with low or neglect cytotoxicity to L 929 cells.91
6 Conclusions and recommendations for further research
The daily production rate of different types of COVID-19-related PPE and hazardous medical wastes are increasing significantly due to the high pandemic rate. A crowd of many thousands of people has been previously affected by the Covid-19 respiratory infection in several areas in the MENA region and the world. Indeed, it should be kept in mind that symptomatic and asymptomatic patients can spread coronavirus through their excreta, As they spread through the environment, some potential steps to consider for these viruses are moved from one compartment to another, entry into living beings, proliferation, possible mutation, transmission, etc. Currently, these coronaviruses are thought to survive in the environment outside of living cells for only a few days, which is enough to reach other organisms and mutate, change their properties, etc. It's possible that we should consider several possible scenarios in the near future. These possible short-term considerations should be considered as promptly as possible, in addition to previously aggressive measures to control direct airborne and indirect spread through other environmental vectors. Proper disposal of medical waste can therefore add value to the economy for sustainable-development in the MENA region and beyond. Additionally, it helps reduce the large-scale spread of the COVID-19 virus and other viruses in a given location. In addition, the efficacy and consequences of wastewater and sludge treatment and the potential for further diffusion into environmental compartments should be considered. Additional research will also examine the potential engineered treatments to retain and/or inactivate this virus and other pathogens circulating in such ecosystem compartments before and after their release, including sorbents and other materials, must be guessed. Nanomaterials such as metal oxide nanostructures, graphene, carbon nanotubes, carbon quantum dots, and titanium dioxide, as well as bio-nanoparticles such as chitosan, capped silver, graphene, gold, and silicon nanoparticles, are thought to play a key role in the development of antiviral coatings. While creating prospective coating materials, some of the most important things to examine are ease of use, low toxicity, health concerns, long-term efficiency, and sustainable manufacturing. The globe is in a difficult period, and offering a single solution for all sorts of surfaces is difficult, but it is feasible to build unique surface coating solutions based on already existing research studies. The multidisciplinary collaboration would undoubtedly aid the rapid development of antiviral surface coating materials. Furthermore, additional research to develop eco-friendly disinfection techniques based on nanotechnology that can potentially remove viruses from the environment without the effects of chronic exposure on other species.
Finally, an accurate, inexpensive, affordable, and straightforward to use on-site tool for monitoring COVID-19 in soil, water, and air, as well as to soften the socio-economic impact of COVID-19. We need to develop a biosensor. COVID-19 pandemics and other undesirable impact on the environment, human and animal health. The SARS-CoV-19 pandemic may be over by the end of 2022, but we must keep a watchful eye on future large-scale pandemics.
Author contributions
GSE suggested the research topic, conceptualization, investigation, writing—original draft, visualization reviewing and editing. DE suggested the research topic, conceptualization, investigation, writing—original draft, visualization, editing and figure drawings MSG suggested the research topic, conceptualization, investigation, writing—original draft, visualization reviewing and editing. DME suggested the research topic, conceptualization, investigation, writing—original draft, visualization reviewing and editing. MA suggested the research topic, conceptualization, investigation, writing—original draft, visualization reviewing and editing. HGN suggested the research topic, conceptualization, investigation, writing—original draft, visualization reviewing and editing. MSK suggested the research topic, conceptualization, investigation, writing—original draft, visualization reviewing and editing. MAG suggested the research topic, conceptualization, investigation, writing—original draft, visualization reviewing and editing.
Conflicts of interest
The authors state that the study was conducted without commercial or financial relationships that could be construed as a potential conflict of interest.
Acknowledgements
Mohamed Abouzid is a participant of the STER Internationalization of Doctoral Schools Program from the NAWA Polish National Agency for Academic Exchange No. PPI/STE/2020/1/00014/DEC/02.
References
- T. A. Aragaw, Surgical face masks as a potential source for microplastic pollution in the COVID-19 scenario, Mar. Pollut. Bull., 2020, 159, 111517 CrossRef CAS PubMed.
- N. U. Benson, O. H. Fred-Ahmadu, D. E. Bassey and A. A. Atayero, COVID-19 pandemic and emerging plastic-based personal protective equipment waste pollution and management in Africa, J. Environ. Chem. Eng., 2021, 9(3), 105222 CrossRef CAS PubMed.
- R. E. Caraka, Y. Lee, R. Kurniawan, R. Herliansyah, P. A. Kaban, B. I. Nasution, P. U. Gio, R. C. Chen, T. Toharudin and B. Pardamean, Impact of COVID-19 large scale restriction on environment and economy in Indonesia, Global J. Environ. Sci. Manage., 2020, 6(Special Issue), 65–84 CAS.
- O. Alagha, A. Alomari and N. Jarrah, Medical waste management and production rate in the Eastern Province of the Kingdom of Saudi Arabia, Euro-Mediterr. J. Environ. Integr., 2018, 3(1), 1–8 CrossRef.
- K. Al-Omran, E. Khan, N. Ali and M. Bilal, Estimation of COVID-19 generated medical waste in the Kingdom of Bahrain, Sci. Total Environ., 2021, 801, 149642 CrossRef CAS PubMed.
- M. B. Haddad, G. E. De-la-Torre, M. R. Abelouah, S. Hajji and A. A. Alla, Personal protective equipment (PPE) pollution associated with the COVID-19 pandemic along the coastline of Agadir, Morocco, Sci. Total Environ., 2021, 798, 149282 CrossRef CAS PubMed.
- A.-H. Alomari, O. Aga, L. El Sahmarany, M. Hegazi and L. Almulla, Public perception towards medical waste generated in the environment during the COVID-19 pandemic in Eastern Province, Saudi Arabia, Heliyon, 2021, 7(11), e08363 CrossRef CAS PubMed.
- H. Lemma, L. Asefa, T. Gemeda and D. Dhengesu, Infectious medical waste management during the COVID-19 pandemic in public hospitals of West Guji zone, southern Ethiopia, Clin. Epidemiol. Global Health, 2022, 15, 101037 CrossRef CAS PubMed.
- P. C. Ojha, S. S. Satpathy, A. K. Ojha, L. B. Sukla and D. Pradhan, Overcoming challenges due to enhanced biomedical waste generation during COVID-19 pandemic, Sci. Total Environ., 2022, 155072 CrossRef CAS PubMed.
- P. Saxena, I. P. Pradhan and D. Kumar, Redefining bio medical waste management during COVID-19 in India: A way forward, Mater. Today: Proc., 2022, 60, 849–858 CAS.
- C.-W. Yoon, M.-J. Kim, Y.-S. Park, T.-W. Jeon and M.-Y. Lee, A Review of Medical Waste Management Systems in the Republic of Korea for Hospital and Medical Waste Generated from the COVID-19 Pandemic, Sustainability, 2022, 14(6), 3678 CrossRef CAS.
- A. Cano-Vicent, R. Hashimoto, K. Takayama and Á. Serrano-Aroca, Biocompatible films of calcium alginate inactivate enveloped viruses such as SARS-CoV-2, Polymers, 2022, 14(7), 1483 CrossRef CAS PubMed.
- M. Hamdi, H. M. Abdel-Bar, E. Elmowafy, A. El-Khouly, M. Mansour and G. A. Awad, Investigating the internalization and COVID-19 antiviral computational analysis of optimized nanoscale zinc oxide, ACS Omega, 2021, 6(10), 6848–6860 CrossRef CAS PubMed.
- M. Eloffy, D. M. El-Sherif, M. Abouzid, M. Abd Elkodous, H. S. El-nakhas, R. F. Sadek, M. A. Ghorab, A. Al-Anazi and G. S. El-Sayyad, Proposed approaches for coronaviruses elimination from wastewater: Membrane techniques and nanotechnology solutions, Nanotechnol. Rev., 2022, 11(1), 1–25 CAS.
- B. Paital, K. Das, F. Malekdar, M. A. Sandoval, E. K. Niaragh, Z. Frontistis, T. R. Behera, G. Balacco, S. Sangkham and A. K. Hati, A State-of-the-Art Review on SARS-CoV-2 Virus Removal Using Different Wastewater Treatment Strategies, Environments, 2022, 9(9), 110 CrossRef.
- J. Howard, A. Huang, Z. Li, Z. Tufekci, V. Zdimal, H.-M. van der Westhuizen, A. von Delft, A. Price, L. Fridman, L.-H. Tang, V. Tang, G. L. Watson, C. E. Bax, R. Shaikh, F. Questier, D. Hernandez, L. F. Chu, C. M. Ramirez and A. W. Rimoin, An evidence review of face masks against COVID-19, Proc. Natl. Acad. Sci., 2021, 118(4), e2014564118 CrossRef CAS PubMed.
- S. Ilyas, R. R. Srivastava and H. Kim, Disinfection technology and strategies for COVID-19 hospital and bio-medical waste management, Sci. Total Environ., 2020, 749, 141652 CrossRef CAS PubMed.
- C. Nzediegwu and S. X. Chang, Improper solid waste management increases potential for COVID-19 spread in developing countries, Resour., Conserv. Recycl., 2020, 161, 104947 CrossRef PubMed.
- M. Abouzid, D. M. El-Sherif, Y. Al Naggar, M. M. Alshehri, S. Alothman, H. R. El-Seedi, R. Trabelsi, O. M. Ibrahim, E. H. Temraz, A. Buimsaedah, I. A. Aziz, M. Alwan, N. H. J. Al Hasan, H. N. Ragab, A. M. Koraiem, M. H. Ahmed, H. H. Temraz, A. K. Madeeh, M. O. Alshareif, F. S. Elkhafeefi, I.-E. Badis, A. E. Abdelslam, A. A. M. Ali, N. E. I. Kotni and T. Amer, Investigating the current environmental situation in the Middle East and North Africa (MENA) region during the third wave of COVID-19 pandemic: urban vs. rural context, BMC Public Health, 2022, 22(1), 177 CrossRef CAS PubMed.
- M. Abed Alah, S. Abdeen, N. Selim, D. Hamdani, E. Radwan, N. Sharaf, H. Al-Katheeri and I. Bougmiza, Compliance and barriers to the use of infection prevention and control measures among health care workers during COVID-19 pandemic in Qatar: A national survey, J. Nurs. Manag., 2021, 29(8), 2401–2411 CrossRef PubMed.
- A. Abbas, T. Al-Otaibi, O. A. Gheith, A. M. Nagib, M. M. Farid and M. Walaa, Sleep Quality Among Healthcare Workers During the COVID-19 Pandemic and Its Impact on Medical Errors: Kuwait Experience, Turk. Thorac. J., 2021, 22(2), 142–148 CrossRef PubMed.
- I. A. Hassan, A. Younis, M. A. Al Ghamdi, M. Almazroui, J. M. Basahi, M. M. El-Sheekh, E. K. Abouelkhair, N. S. Haiba, M. S. Alhussaini, D. Hajjar, M. M. Abdel Wahab and D. M. El Maghraby, Contamination of the marine environment in Egypt and Saudi Arabia with personal protective equipment during COVID-19 pandemic: A short focus, Sci. Total Environ., 2022, 810, 152046 CrossRef CAS PubMed.
- N. Mejjad, E. K. Cherif, A. Rodero, D. A. Krawczyk, J. El Kharraz, A. Moumen, M. Laqbaqbi and A. Fekri, Disposal Behavior of Used Masks during the COVID-19 Pandemic in the Moroccan Community: Potential Environmental Impact, Int. J. Environ. Res. Public Health, 2021, 18(8), 4382 CrossRef CAS PubMed.
- T. Hatami, M. R. J. Rakib, R. Madadi, G. E. De-la-Torre and A. M. Idris, Personal protective equipment (PPE) pollution in the Caspian Sea, the largest enclosed inland water body in the world, Sci. Total Environ., 2022, 824, 153771 CrossRef CAS PubMed.
- J. Bujak, Thermal treatment of medical waste in a rotary kiln, J. Environ. Manage., 2015, 162, 139–147 CrossRef CAS PubMed.
- J. W. Bujak, Heat recovery from thermal treatment of medical waste, Energy, 2015, 90, 1721–1732 CrossRef.
- S. Lee, Y. Kim, J. Park, H. K. Shon and S. Hong, Treatment of medical radioactive liquid waste using Forward Osmosis (FO) membrane process, J. Membr. Sci., 2018, 556, 238–247 CrossRef CAS.
- G. Mantzaras and E. A. Voudrias, An optimization model for collection, haul, transfer, treatment and disposal of infectious medical waste: Application to a Greek region, Waste Manage., 2017, 69, 518–534 CrossRef PubMed.
- Y. Qi, J. He, Y. Li, X. Yu, F.-R. Xiu, Y. Deng and X. Gao, A novel treatment method of PVC-medical waste by near-critical methanol: Dechlorination and additives recovery, Waste Manage., 2018, 80, 1–9 CrossRef CAS PubMed.
- M. Liu, J. Wen, Y. Feng, L. Zhang, J. Wu, J. Wang and X. Yang, A benefit evaluation for recycling medical plastic waste in China based on material flow analysis and life cycle assessment, J. Cleaner Prod., 2022, 368, 133033 CrossRef.
- K. Selvaranjan, S. Navaratnam, P. Rajeev and N. Ravintherakumaran, Environmental challenges induced by extensive use of face masks during COVID-19: A review and potential solutions, Environ. Challenges, 2021, 3, 100039 CrossRef CAS.
- S. Sridharan, M. Kumar, L. Singh, N. S. Bolan and M. Saha, Microplastics as an emerging source of particulate air pollution: A critical review, J. Hazard. Mater., 2021, 418, 126245 CrossRef CAS PubMed.
- O. O. Fadare and E. D. Okoffo, Covid-19 face masks: A potential source of microplastic fibers in the environment, Sci. Total Environ., 2020, 737, 140279 CrossRef CAS PubMed.
- Y. Pu, J. Zheng, F. Chen, Y. Long, H. Wu, Q. Li, S. Yu, X. Wang and X. Ning, Preparation of polypropylene micro and nanofibers by electrostatic-assisted melt blown and their application, Polymers, 2018, 10(9), 959 CrossRef PubMed.
- K. C. Dutton, Overview and analysis of the meltblown process and parameters, J. Text. Appar. Technol. Manag., 2009, 6(1), 1–25 Search PubMed.
- J. Gasperi, S. L. Wright, R. Dris, F. Collard, C. Mandin, M. Guerrouache, V. Langlois, F. J. Kelly and B. Tassin, Microplastics in air: are we breathing it in?, Curr. Opin. Environ. Sci. Health, 2018, 1, 1–5 CrossRef.
- Q. Zhang, E. G. Xu, J. Li, Q. Chen, L. Ma, E. Y. Zeng and H. Shi, A review of microplastics in table salt, drinking water, and air: direct human exposure, Environ. Sci. Technol., 2020, 54(7), 3740–3751 CrossRef CAS PubMed.
- S. Abbasi, B. Keshavarzi, F. Moore, A. Turner, F. J. Kelly, A. O. Dominguez and N. Jaafarzadeh, Distribution and potential health impacts of microplastics and microrubbers in air and street dusts from Asaluyeh County, Iran, Environ. Pollut., 2019, 244, 153–164 CrossRef CAS PubMed.
- Y. Lai, L. Hao, L. Dong, S. Yu and J. Liu, Coating zirconium oxide-nanocomposite with humic acid for recovery of mercury and chromium in hazardous waste of chemical oxygen demand test, J. Environ. Sci., 2023, 126, 40–47 CrossRef PubMed.
- S. Faridi, R. N. Nodehi, S. Sadeghian, M. Tajdini, M. Hoseini, M. Yunesian, S. Nazmara, M. S. Hassanvand and K. Naddafi, Can respirator face masks in a developing country reduce exposure to ambient particulate matter?, J. Exposure Sci. Environ. Epidemiol., 2020, 30(4), 606–617 CrossRef CAS PubMed.
- M. I. Vasquez, A. Lambrianides, M. Schneider, K. Kümmerer and D. Fatta-Kassinos, Environmental side effects of pharmaceutical cocktails: what we know and what we should know, J. Hazard. Mater., 2014, 279, 169–189 CrossRef CAS PubMed.
- W. Leal Filho, E. Price, T. Wall, C. Shiel, U. M. Azeiteiro, M. Mifsud, L. Brandli, C. S. Farinha, S. Caeiro, A. L. Salvia, C. R. Vasconcelos, L. O. De Sousa, P. Pace, F. Doni, L. Veiga Avila, B. Fritzen and T. J. Levasseur, COVID-19: the impact of a global crisis on sustainable development teaching, Environ. Dev. Sustain., 2021, 23(8), 11257–11278 CrossRef PubMed.
- A. Kumar, V. Jain, A. Deovanshi, A. Lepcha, C. Das, K. Bauddh and S. Srivastava, Environmental impact of COVID-19 pandemic: more negatives than positives, Environ. Sustainability, 2021, 4(3), 447–454 CrossRef.
- Y. Babanyara, D. Ibrahim, T. Garba, A. Bogoro and M. Abubakar, Poor Medical Waste Management (MWM) practices and its risks to human health and the environment: a literature review, J. Environ. Health Sci. Eng., 2013, 11(7), 1–8 Search PubMed.
- A. Belhadi, S. S. Kamble, S. A. R. Khan, F. E. Touriki and M. D. Kumar, Infectious waste management strategy during COVID-19 pandemic in Africa: An integrated decision-making framework for selecting sustainable technologies, Environ. Manage., 2020, 66(6), 1085–1104 CrossRef PubMed.
- A. D. Zand and A. V. Heir, Emanating challenges in urban and healthcare waste management in Isfahan, Iran after the outbreak of COVID-19, Environ. Technol., 2021, 42(2), 329–336 CrossRef CAS PubMed.
- I. A. Idowu, W. Atherton, K. Hashim, P. Kot, R. Alkhaddar, B. I. Alo and A. Shaw, An analyses of the status of landfill classification systems in developing countries: Sub Saharan Africa landfill experiences, Waste Manage., 2019, 87, 761–771 CrossRef PubMed.
- S. Ramteke and B. L. Sahu, Novel coronavirus disease 2019 (COVID-19) pandemic: considerations for the biomedical waste sector in India, Case Stud. Chem. Environ. Eng., 2020, 2, 100029 CrossRef.
- M. A. Zambrano-Monserrate, M. A. Ruano and L. Sanchez-Alcalde, Indirect effects of COVID-19 on the environment, Sci. Total Environ., 2020, 728, 138813 CrossRef CAS PubMed.
- W. H. Organization, Water, sanitation and hygiene in health care facilities: status in low and middle income countries and way forward. 2015 Search PubMed.
- A. Núñez-Delgado, What do we know about the SARS-CoV-2 coronavirus in the environment?, Sci. Total Environ., 2020, 727, 138647 CrossRef PubMed.
- M. Miyauchi, K. Sunada and K. Hashimoto, Antiviral effect of visible light-sensitive CuxO/TiO2 photocatalyst, Catalysts, 2020, 10(9), 1093 CrossRef CAS.
- G. Kampf, D. Todt, S. Pfaender and E. Steinmann, Persistence of coronaviruses on inanimate surfaces and their inactivation with biocidal agents, J. Hosp. Infect., 2020, 104(3), 246–251 CrossRef CAS PubMed.
- G. W. Park, M. Cho, E. L. Cates, D. Lee, B.-T. Oh, J. Vinjé and J.-H. Kim, Fluorinated TiO2 as an ambient light-activated virucidal surface coating material for the control of human norovirus, J. Photochem. Photobiol., B, 2014, 140, 315–320 CrossRef CAS PubMed.
- R. Nakano, M. Hara, H. Ishiguro, Y. Yao, T. Ochiai, K. Nakata, T. Murakami, J. Kajioka, K. Sunada and K. Hashimoto, Broad spectrum microbicidal activity of photocatalysis by TiO2, Catalysts, 2013, 3(1), 310–323 CrossRef CAS.
- S. Akhtar, K. Shahzad, S. Mushtaq, I. Ali, M. H. Rafe and S. M. Fazal-ul-Karim, Antibacterial and antiviral potential of colloidal Titanium dioxide (TiO2) nanoparticles suitable for biological applications, Mater. Res. Express, 2019, 6(10), 105409 CrossRef CAS.
- J. Hasan, Y. Xu, T. Yarlagadda, M. Schuetz, K. Spann and P. K. Yarlagadda, Antiviral and antibacterial nanostructured surfaces with excellent mechanical properties for hospital applications, ACS Biomater. Sci. Eng., 2020, 6(6), 3608–3618 CrossRef CAS PubMed.
- K. Shirvanimoghaddam, M. K. Akbari, R. Yadav, A. K. Al-Tamimi and M. Naebe, Fight against COVID-19: The case of antiviral surfaces, APL Mater., 2021, 9(3), 031112 CrossRef CAS PubMed.
- Z. Sun and K. K. Ostrikov, Future antiviral surfaces: Lessons from COVID-19 pandemic, Sustainable Mater. Technol., 2020, 25, e00203 CrossRef CAS.
- J. Prakash, J. Cho and Y. K. Mishra, Photocatalytic TiO2 nanomaterials as potential antimicrobial and antiviral agents: Scope against blocking the SARS-COV-2 spread, Micro Nano Eng., 2021, 100100 Search PubMed.
- M. Reid, V. Whatley, E. Spooner, A. M. Nevill, M. Cooper, J. J. Ramsden and S. J. Dancer, How does a photocatalytic antimicrobial coating affect environmental bioburden in hospitals?, Infect. Control Hosp. Epidemiol., 2018, 39(4), 398–404 CrossRef PubMed.
- O. Negrete; S. Bradfute; S. R. Larson; A. Sinha; K. R. Coombes; R. S. Goeke; L. A. Keenan; J. Duay; M. S. Van Heukelom and M. Rodriguez Photocatalytic Material Surfaces for SARS-CoV-2 Virus Inactivation, Sandia National Lab.(SNL-CA), Livermore, CA (United States), 2020 Search PubMed.
- N. Bono, F. Ponti, C. Punta and G. Candiani, Effect of UV Irradiation and TiO2-Photocatalysis on Airborne Bacteria and Viruses: An Overview, Materials, 2021, 14(5), 1075 CrossRef CAS PubMed.
- S. Kumar, Bhawna, R. Sharma, A. Gupta, K. K. Dubey, A. M. Khan, R. Singhal, R. Kumar, A. Bharti, P. Singh, R. Kant and V. Kumar, TiO2 based Photocatalysis membranes: An efficient strategy for pharmaceutical mineralization, Sci. Total Environ., 2022, 845, 157221 CrossRef CAS PubMed.
- R. Matsuura, C.-W. Lo, S. Wada, J. Somei, H. Ochiai, T. Murakami, N. Saito, T. Ogawa, A. Shinjo and Y. Benno, SARS-CoV-2 disinfection of air and surface contamination by TiO2 photocatalyst-mediated damage to viral morphology, RNA, and protein, Viruses, 2021, 13(5), 942 CrossRef CAS PubMed.
- S. Khaiboullina, T. Uppal, N. Dhabarde, V. R. Subramanian and S. C. Verma, Inactivation of human coronavirus by titania nanoparticle coatings and UVC radiation: throwing light on SARS-CoV-2, Viruses, 2020, 13(1), 19 CrossRef PubMed.
- P. Micochova, A. Chadha, T. Hesseloj, F. Fraternali, J. J. Ramsden and R. K. Gupta, Rapid inactivation of SARS-CoV-2 by titanium dioxide surface coating, Wellcome Open Res., 2021, 6, 56 Search PubMed.
- R. Z. Hamza, A. A. Gobouri, H. M. Al-Yasi, T. A. Al-Talhi and S. M. El-Megharbel, A New Sterilization Strategy Using TiO2 Nanotubes for Production of Free Radicals that Eliminate Viruses and Application of a Treatment Strategy to Combat Infections Caused by Emerging SARS-CoV-2 during the COVID-19 Pandemic, Coatings, 2021, 11(6), 680 CrossRef CAS.
- G. Mathur, COVID Killing Air Purifier Based on UV & Titanium Dioxide Based Photocatalysis System, SAE Int. J. Adv. Curr. Pract. Mobil., 2021, 4, 143–150 CrossRef.
- T. Uppal, S. Reganti, E. Martin and S. C. Verma, Surface Inactivation of Human Coronavirus by MACOMA™ UVA-TiO2 Coupled Photocatalytic Disinfection System, Catalysts, 2022, 12(7), 690 CrossRef CAS.
- Y. Lu, S. Guan, L. Hao, H. Yoshida, S. Nakada, T. Takisawa and T. Itoi, Inactivation of SARS-CoV-2 and photocatalytic degradation by TiO2 photocatalyst coatings, Sci. Rep., 2022, 12(1), 16038 CrossRef CAS PubMed.
- E. J. Haas, F. J. Angulo, J. M. McLaughlin, E. Anis, S. R. Singer, F. Khan, N. Brooks, M. Smaja, G. Mircus, K. Pan, J. Southern, D. L. Swerdlow, L. Jodar, Y. Levy and S. Alroy-Preis, Impact and effectiveness of mRNA BNT162b2 vaccine against SARS-CoV-2 infections and COVID-19 cases, hospitalisations, and deaths following a nationwide vaccination campaign in Israel: an observational study using national surveillance data, The Lancet, 2021, 397(10287), 1819–1829 CrossRef CAS PubMed.
- R. Nakano, H. Ishiguro, Y. Yao, J. Kajioka, A. Fujishima, K. Sunada, M. Minoshima, K. Hashimoto and Y. Kubota, Photocatalytic inactivation of influenza virus by titanium dioxide thin film, Photochem. Photobiol. Sci., 2012, 11(8), 1293–1298 CrossRef CAS PubMed.
- J. Hasan, Y. Xu, T. Yarlagadda, M. Schuetz, K. Spann and P. K. D. V. Yarlagadda, Antiviral and Antibacterial Nanostructured Surfaces with Excellent Mechanical Properties for Hospital Applications, ACS Biomater. Sci. Eng., 2020, 6(6), 3608–3618 CrossRef CAS PubMed.
- H. Ghaffari, A. Tavakoli, A. Moradi, A. Tabarraei, F. Bokharaei-Salim, M. Zahmatkeshan, M. Farahmand, D. Javanmard, S. J. Kiani and M. Esghaei, Inhibition of H1N1 influenza virus infection by zinc oxide nanoparticles: another emerging application of nanomedicine, J. Biomed. Sci., 2019, 26(1), 1–10 CrossRef CAS PubMed.
- Y. K. Mishra, R. Adelung, C. Röhl, D. Shukla, F. Spors and V. Tiwari, Virostatic potential of micro–nano filopodia-like ZnO structures against herpes simplex virus-1, Antiviral Res., 2011, 92(2), 305–312 CrossRef CAS PubMed.
- T. E. Antoine, Y. K. Mishra, J. Trigilio, V. Tiwari, R. Adelung and D. Shukla, Prophylactic, therapeutic and neutralizing effects of zinc oxide tetrapod structures against herpes simplex virus type-2 infection, Antiviral Res., 2012, 96(3), 363–375 CrossRef CAS PubMed.
- T. E. Antoine, S. R. Hadigal, A. M. Yakoub, Y. K. Mishra, P. Bhattacharya, C. Haddad, T. Valyi-Nagy, R. Adelung, B. S. Prabhakar and D. Shukla, Intravaginal zinc oxide tetrapod nanoparticles as novel immunoprotective agents against genital herpes, J. Immunol., 2016, 196(11), 4566–4575 CrossRef CAS PubMed.
- A. Agelidis, L. Koujah, R. Suryawanshi, T. Yadavalli, Y. K. Mishra, R. Adelung and D. Shukla, An intra-vaginal zinc oxide tetrapod nanoparticles (ZOTEN) and genital herpesvirus cocktail can provide a novel platform for live virus vaccine, Front. Immunol., 2019, 10, 500 CrossRef CAS PubMed.
- J. Prakash, J. Pivin and H. Swart, Noble metal nanoparticles embedding into polymeric materials: From fundamentals to applications, Adv. Colloid Interface Sci., 2015, 226, 187–202 CrossRef CAS PubMed.
- J. Prakash, S. Sun, H. C. Swart and R. K. Gupta, Noble metals-TiO2 nanocomposites: from fundamental mechanisms to photocatalysis, surface enhanced Raman scattering and antibacterial applications, Appl. Mater. Today, 2018, 11, 82–135 CrossRef.
- M. Abd Elkodous, H. M. El-Husseiny, G. S. El-Sayyad, A. H. Hashem, A. S. Doghish, D. Elfadil, Y. Radwan, H. M. El-Zeiny, H. Bedair and O. A. Ikhdair, Recent advances in waste-recycled nanomaterials for biomedical applications: Waste-to-wealth, Nanotechnol. Rev., 2021, 10(1), 1662–1739 CrossRef.
- D. Elfadil, W. F. Elkhatib and G. S. El-Sayyad, Promising advances in nanobiotic-based formulations for drug specific targeting against multidrug resistant microbes and biofilm-associated infections, Microb. Pathog., 2022, 105721 CrossRef CAS PubMed.
- S. Gurunathan, M. Qasim, Y. Choi, J. T. Do, C. Park, K. Hong, J.-H. Kim and H. Song, Antiviral potential of nanoparticles—Can nanoparticles fight against coronaviruses?, Nanomaterials, 2020, 10(9), 1645 CrossRef CAS PubMed.
- J. Hasan, A. Pyke, N. Nair, T. Yarlagadda, G. Will, K. Spann and P. K. Yarlagadda, Antiviral nanostructured surfaces reduce the viability of SARS-CoV-2, ACS Biomater. Sci. Eng., 2020, 6(9), 4858–4861 CrossRef CAS PubMed.
- G. H. Attia, Y. S. Moemen, M. Youns, A. M. Ibrahim, R. Abdou and M. A. El Raey, Antiviral zinc oxide nanoparticles mediated by hesperidin and in silico comparison study between antiviral phenolics as anti-SARS-CoV-2, Colloids Surf., B, 2021, 203, 111724 CrossRef CAS PubMed.
- H. Zhong, Z. Zhu, J. Lin, C. F. Cheung, V. L. Lu, F. Yan, C.-Y. Chan and G. Li, Reusable and recyclable graphene masks with outstanding superhydrophobic and photothermal performances, ACS Nano, 2020, 14(5), 6213–6221 CrossRef CAS PubMed.
- V. A. Bobrin, S.-P. R. Chen, C. F. Grandes Reyes, T. Smith, D. F. J. Purcell, J. Armstrong, J. L. McAuley and M. J. Monteiro, Surface Inactivation of Highly Mutated SARS-CoV-2 Variants of Concern: Alpha, Delta, and Omicron, Biomacromolecules, 2022, 23(9), 3960–3967 CrossRef CAS PubMed.
- S. Mallakpour, E. Azadi and C. M. Hussain, Fabrication of air filters with advanced filtration performance for removal of viral aerosols and control the spread of COVID-19, Adv. Colloid Interface Sci., 2022, 102653 CrossRef CAS PubMed.
- L. Issman, B. Graves, J. Terrones, M. Hosmillo, R. Qiao, M. Glerum, S. Yeshurun, M. Pick, I. Goodfellow and J. Elliott, Filtration of viral aerosols via a hybrid carbon nanotube active filter, Carbon, 2021, 183, 232–242 CrossRef CAS.
- R. A. Bataglioli, J. B. Rocha Neto, G. B. Calais, L. M. Lopes, J. Tsukamoto, A. P. de Moraes, C. W. Arns and M. M. Beppu, Hybrid alginate–copper sulfate textile coating for coronavirus inactivation, J. Am. Ceram. Soc., 2022, 105(3), 1748–1752 CrossRef CAS.
- S. Kim, J. Chung, S. H. Lee, J. H. Yoon, D.-H. Kweon and W.-J. Chung, Tannic acid-functionalized HEPA filter materials for influenza virus capture, Sci. Rep., 2021, 11(1), 1–7 CrossRef PubMed.
- W.-H. Sun, L.-F. Hui, Q. Yang and G.-D. Zhao, Nanofiltration filter paper based on multi-walled carbon nanotubes and cellulose filter papers, RSC Adv., 2021, 11(2), 1194–1199 RSC.
Footnote |
† Mohamed A. Ghorab is currently affiliated with The Office of Chemical Safety and Pollution Prevention, U.S. Environmental Protection Agency (EPA), Washington, DC 20460, USA. |
|
This journal is © The Royal Society of Chemistry 2023 |
Click here to see how this site uses Cookies. View our privacy policy here.