DOI:
10.1039/D3RA01606D
(Paper)
RSC Adv., 2023,
13, 16034-16038
The one/two atom size-reduction of [Au23SCy16]− induced by the [Au6(dppp)4]2+ cluster†
Received
11th March 2023
, Accepted 22nd May 2023
First published on 30th May 2023
Abstract
The recent progress in atomically precise metal (Au, Ag etc.) nanoclusters has greatly enriched the molecular-level mechanistic understanding of metal nanomaterials. Herein, using two meta-stable (easy formation, easy transformation) clusters, i.e. [Au23SCy16]− and [Au6(dppp)4]2+ (HSCy and dppp denote cyclohexanethiol and 1,3-bis(diphenylphosphino)propane), as the reaction precursors, the etching of Au23 occurs smoothly, giving the one/two-atom size-reduced [Au21SCy12(dppp)2]+ and [Au22SCy14(dppp)]2+ as the major products. Structural analysis and DFT calculations indicate that the active reaction site of Au23 lies in the core–shell interference of the bi-capped icosahedral Au15 core and the AuS2 motifs. The fluorescence, band gap, and thermostability of the Au21 cluster products are improved compared to that of the Au23 precursors.
Introduction
The atomic precision of noble metal nanoclusters (with single crystal X-ray diffraction, mass spectra etc.) makes it possible to elucidate the fantastic chemistry and the inherent structure–property correlations of nanomaterials at a molecular level.1–3 The stimuli response (to pH changes, additives etc.4) represents one of the most appealing characteristics of metal nanoclusters, providing sound proof for the traditional theories (such as the Lamer/aggregative size growth),5 and opening the door for the practical applications in catalysis,6,7 sensing8,9 and bioclinics.10 In this scenario, the inter-cluster reactions have become a novel synthetic strategy to prepare atomically precise metal nanoclusters,11 and to shed light on the dynamics of the cluster precursors.
So far, most of the reported inter-cluster reactions feature ligand exchange or/and metal exchange characteristics. Typically, the size-maintained ligand exchange occurs between two cluster analogs bearing different ligands, such as the reaction of [Au25(PET)18]− with [Au25(SBut)18]− (HPET and HSBut are short for 2-phenyl ethanethiol and 1-butanethiol),12 and the reaction of [Au25(SC10H21)18]− with [Au25(SC12H25)18]− (linear alkyl thiolates in both cases).13 Meanwhile, the metal exchange has been widely reported in the interparticle reactions between two clusters of different metal components (or isotopic ones). For example, the size- and framework-maintained metal exchange occurs in the reaction of [Ag25(DMBT)18]− with [Au25(PET)18]−,14 [Ag7(H){S2P(OiPr)2}6] with [Cu7(H){S2P(OiPr)2}6],15 and the isotopic exchange reactions of [107/109Ag25(DMBT)18]− (ref. 16) and [107/109Ag29(BDT)12(TPP)43−].17 Of note, the inter-cluster reactions between two structurally distinct clusters have also been reported. In the pioneering studies, the reaction of [Au25(FTP)18]− with [Ag44(FTP)30]3−,18 [Au25(PET)18]− with [Ir9(PET)6]+ (ref. 19) and [Au25(SBut)18]− with [Ag51(BDT)19(TPP)3]3− (ref. 20) each generates an alloy cluster product with the same framework as one of the precursors. The distinct metal components and the predominant doping processes in these reactions arise an interesting question as to the reaction mode between two same-metal clusters. To our knowledge, only one such reaction has been reported, i.e. the formation of [Ag16(TBT)8(TFA)7(CH3CN)3Cl]+ and [Ag17(TBT)8(TFA)7(CH3CN)3Cl]+ cocrystals via the reaction of [Ag12(TBT)8(TFA)5(CH3CN)]+ and [Ag18(TPP)10H16]2+ (TBT = tert-butylthiolate, TFA = trifluoroacetate, CH3CN = acetonitrile, TPP = triphenyl-phosphine).21 The structure of the co-crystalized Ag16 and Ag17 products are distinct from the precursors.
Inspired by the inter-particle reaction of the two Ag clusters bearing totally different ligands, herein we chose [Au23SCy16]− and [Au6(dppp)4]2+ (abbreviated as Au23 and Au6) as the reactants. Both of them are meta-stable (easy formation, easy transformation). The single crystal structure of Au6
22 and Au23
23 has been reported, demonstrating their stability during synthesis and under crystallization conditions. But on the other hand, Au6 easily react with the Au(I) complex or Ag+ salt to generate [Au8(dppp)4Cl2]2+,24 or [Au7(dppp)4]3+.25 While upon heating or oxidation with H2O2, Au6 easily converts to [Au11(dppp)5]3+ or [Au8(dppp)4Cl2]2+.26 Similarly, rich chemistry has been reported for the Au23 clusters. The addition of different thiolate ligands (TBBzT/TBBT/2-NPT) results in the size-growth of Au23 to Au24/Au25/Au28,27,28 while the addition of phosphine ligand results in a distinct size-reduction of Au23 → Au22 (ref. 29 and 30)/Au21.30 Meanwhile, the addition of MSCy (M = Ag/Au) complexes results in the formation of heavily Ag-doped alloy (AuAg)25
31 and Au28,32 respectively. Of note, the Au23 → Au28 conversion has also been regulated by oxidation33 and photooxidation34 conditions. In this context, the reaction of Au6 with Au23 clusters will aid the elucidation on the relative stability of the two cluster precursors, and shed light on the inherent structure–activity relationships therein.
In this study, the inter-cluster reaction of [Au23SCy16]− with [Au6(dppp)4]2+ were conducted. In an equimolar reaction of Au23 and Au6, two main products, i.e. [Au21SCy12(dppp)2]+ (Au21), and [Au22SCy14(dppp)]2+ (Au22 for short) were identified and characterized by ESI-MS and UV-vis etc. The framework of Au23 is largely maintained in Au22 and Au21, while the one or two groups of Au(SCy)2 motifs were each replaced by a dppp ligand. With the combination of DFT and structural analysis, the active etching site on the Au23 precursor was found to be the Au(core)-S(on AuS2 motif) bonds. Meanwhile, replacing the AuS2 motifs with the dppp ligands results in significantly higher luminescence, a relatively larger O1–R1 gap, and higher thermal stability.
Experimental
Materials
All reagents were commercially available and used without further purification: dichloromethane (CH2Cl2, HPLC grade, ≥99.9%), methanol (MeOH, HPLC grade, ≥99.9%), n-hexane (n-Hex, HPLC grade, ≥98.0%) HAuCl4·4H2O (≥99.99%, metal basis), and cyclohexane-thiol (HSCy, ≥98%), sodium borohydride (NaBH4, ≥98%), tetrabutylammonium bromide (TOABr, ≥99%), 1,3-bis(diphenylphosphine)propane (≥98%) were purchased from Shanghai Macklin Biochemical Co., Ltd. All glassware was thoroughly cleaned with aqua regia (HCl/HNO3 3/1 v/v), rinsed with copious amounts of pure water, and then dried in an oven before use.
Synthesis of [Au23SCy16]− and [Au6(dppp)4]2+
[Au23SCy16]− and [Au6(dppp)4]2+ were prepared via the previously reported methods,35,36 and verified by UV-vis, and ESI-MS analysis (please see supporting information Fig. S1 and S2†). Briefly, Au23 was synthesized by adding HSCy, TOAB, and NaBH4 into the aqueous solution of HAuCl4 in methanol, and [Au6(dppp)4]2+ was formed via the reaction of HAuCl4, dppp and NaBH4 under room temperature in ethanol.
Characterization
UV-vis absorption spectra were recorded on a UV-6000PC instrument. All fluorescence spectra were obtained using a HORIBA FluoroMax-4P fluorescence spectrophotometer.
Electrospray ionization mass spectrometry measurement was recorded using a Waters Xevo G2-XS QT mass spectrometer.
Results and discussion
The reaction of [Au23SCy16]− and [Au6(dppp)4]2+
The reaction of [Au23SCy16]− and [Au6(dppp)4]2+ was conducted in a 1
:
1 molar ratio. In detail, 20 mg [Au23SCy16]− was dissolved in 15 mL of DCM, and then 8.3 mg of [Au6(dppp)4]2+ was added. Stirring for about 3 hours, the solution colour changed from brownish black to crimson black (Fig. 1a). The crude product was then concentrated and purified by preparative thin-layer chromatography (abbreviated as PTLC) with DCM/Hex (1
:
2) for the first time, and by PTLC with DCM/MeOH (10
:
1) for the second time (Fig. 1b inset).
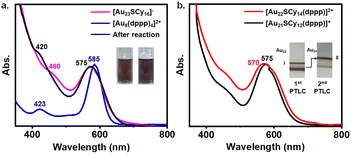 |
| Fig. 1 The UV-vis spectra of the Au23, Au6 precursors and the solution after reacting for 3 hours (a), inset: digital photo of the reaction solution before (left) and after reaction (right); and the UV-vis of the different band components after PTLC separation (b) insets: digital photo of the band distribution. | |
According to Fig. 1b, the UV-vis spectra of the DCM solution of the two bands are very similar, each of which shows a prominent peak at ∼570 nm, and a shoulder peak at ∼460 nm. Meanwhile, ESI-MS characterization of the band I component shows a prominent cluster peak at m/z = 3179.37, corresponding to [Au22SCy14(dppp)]2+ (Fig. 2a). The ESI-MS of the band II component shows a cluster peak at m/z = 6343.40 (Fig. 2b), corresponding to a cluster formula of [Au21SCy12(dppp)2]+ (Au21 for short). For both Au22 and Au21, the isotopic pattern is in excellent agreement with the theoretical one (Fig. 2a and b inset).
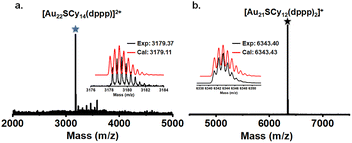 |
| Fig. 2 The ESI-MS and the correlation of the experimental isotopic pattern with the theoretical one (inset) of Au22 (a) and Au21 clusters (b). | |
Of note, the UV-vis spectra of the formed Au21 and Au22 clusters are very similar to the reported spectra of [Au22SCy14(dppp)], and [Au21SCy12L2]+ (L = dppm/dppe/CDPE).30 But the conversion details and the components of the Au21 and Au22 clusters are distinct from the reported ones. First, the reported Au21 and Au22 clusters were formed via the AuIL (L = diphosphate) etching of Au23 precursor, while the inter-cluster reaction of Au23 and Au6 was used in this study. Second, the charge state of the Au22 clusters in this study is distinct from the reported one (+2 vs. 0). Besides, the Au21 cluster co-protected by SCy and dppp ligands was not reported,30 and the etching of Au23 with AuIdppp generates Au22 cluster exclusively in the early study. Herein, using Au6 as a dppp-donating reagent, the Au21 cluster co-protected by SCy and dppp ligand was gained as a main product. Nevertheless, given the similarity in the UV-vis of the formed Au22/Au21 cluster with the reported ones, and the plausibility of using UV-vis absorption curve to determine the cluster frameworks,35,36 we anticipated that the framework of the formed Au21 and Au22 clusters is similar to the reported ones. Accordingly, the structure of the [Au23SCy16]− has been largely maintained after the reaction.
As shown in Fig. 3, the structure of Au23 could be viewed as protecting the bicapped icosahedral Au15 core with two Au3S4, two AuS2 staple motifs, and four bridging thiolate ligands. Replacing one or two AuS2 motifs with one/two dppp ligands generates the structure of Au22/Au21. On the basis of the structural analysis, we performed density functional theory (DFT) calculations on the bond dissociation energy (BDE) of the Au–S bonds (see ESI† for the details of the computational method). The detailed results are given in Fig. S3,† and the BDE of the Au–S bonds between Au15 core and S on AuS2 is remarkably lower than all other ones, while the Aucap–S bond (Fig. 3) is slightly lower than that of the Aucore–S (8.1 vs. 10.8 kcal mol−1). According to the calculation results, both bonds could be easily broken under experimental conditions due to the low energy demands.
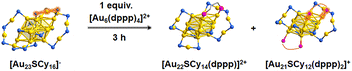 |
| Fig. 3 The size-conversion of the [Au23SCy16]− and [Au6(dppp)4]2+ clusters with the related structures. | |
Given the reaction mechanism, PTLC monitoring on the target reaction system (Fig. S4†) indicates the rapid formation of Au22 and Au21 within the first 10 minutes. After that, the amount of the Au22 slightly diminished in the following reaction time. By contrast, the amount of Au21 gradually increased, associated with the continuous reduction of both Au22 and the Au6 components. The results imply the easy replacement of the first AuS2 motif, but the relatively difficult replacement of the second AuS2 motif on the Au23 precursor. In other words, the reactivity for the ligand exchange of AuS2 to dppp has been greatly reduced after the first time exchange.
Fluorescence
Albeit the similar framework, [Au23(SCy)16]− showed a weak emission peak at 703 nm, while [Au22SCy14(dppp)]2+ and [Au21SCy12(dppp)2]+ showed approximately 2-fold emission enhancement, with a tiny redshift of the emission maximum wavelength (708 nm for Au22 and 707 nm for Au21, Fig. 4).
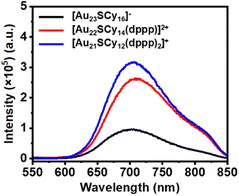 |
| Fig. 4 Emission spectra of Au23, Au22 and Au21 under excitation upon 365 nm light irradiation. | |
Differential pulse voltammetry (DPV)
The differential pulse voltammetry (DPV) curves of both Au23 precursor and the Au22/Au21 product clusters (Fig. 5) feature molecular-like electrochemical characteristics, with distinctive oxidation and reduction potentials. In detail, the first oxidation peaks (O1) of the Au23, Au22 and Au21 clusters are at 0.13, 0.23 and 0.66 V, respectively. The first reduction peaks (R1) are observed at −0.78, −1.00 and −0.80 V, respectively. Accordingly, the R1–O1 gap of the Au23, Au22 and Au21 clusters are 0.91, 1.23 and 1.46 V, respectively. The enlarged gap of Au22 and Au21 than that of Au23 demonstrates the enhanced electrochemical stability induced by the AuS2 → dppp exchange.
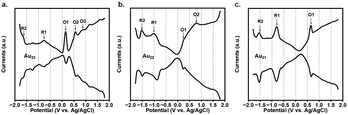 |
| Fig. 5 DPV spectra of Au23 (a), Au22 (b) and Au21 (c) in 0.1 M Bu4NPF6–CH2Cl2 solutions that are degassed for 15 min and blanketed with N2 at room temperature. Open-circuit voltage is 0.204 V. | |
Thermal stability
Associating with the size-reduction, the thermal stability of the clusters has been significantly improved. Following the recent studies,37,38 the stability of the Au21, Au22 and Au23 clusters under heating conditions were tracked with the UV-vis spectrum. As shown in Fig. 6, upon heating at 60 °C, the characteristic peak on UV-vis spectra of Au22 and Au21 maintains after even 18 hours, and the Au21 cluster is even stable after 60 hours. By contrast, the characteristic peak of Au23 attenuated within 1 hour, and becomes very weak after 18 hours. Herein, both the higher thermal stability and the co-presence of thiolate and diphosphine ligands will be helpful for the future application of the Au22/Au21 clusters.
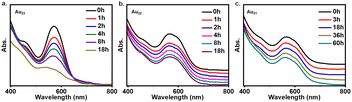 |
| Fig. 6 Thermal stability test of Au23 (a), Au22 (b) and Au21 (c) clusters at 60 °C in toluene. | |
Conclusions
Herein, the inter-cluster reaction of [Au23SCy16]− and [Au6(dppp)4]2+ clusters were conducted. [Au21SCy12(dppp)2]+, and [Au22SCy14(dppp)]2+ were identified as the main products. The preliminary mechanistic insights with the combination of experimental and DFT calculations indicates the Au–S bond in the core–shell interference, and especially the Au–S bond of AuS2 motif and the core structure, is the active reaction site. After incorporating the diphosphine ligands, the Au22/Au21 clusters show stronger luminescence than the Au23 precursor. Meanwhile, both the electrochemical and thermo-stability tests indicate the higher stability of the Au21 than that of the Au23 precursors. The enhanced stability of the produced clusters might show high potential in future applications.
Author contributions
All authors have given approval to the final version of the manuscript. L. Z.: experiments, data analysis, manuscript draft; D. F.: investigation, formal analysis; M.C.: initial experiments; S. H. and Y. S.: theoretical calculation; H. Y.: conceptualization, supervision, review & editing, funding acquisition; M. Z.: conceptualization, supervision.
Conflicts of interest
There are no conflicts to declare.
Acknowledgements
We acknowledge financial support from National Science Foundation of Anhui Province (2108085J08), The University Synergy Innovation Program of Anhui Province (GXXT-2021-023).
References
- J. Yang, Y. Peng, S. Li, J. Mu, Z. Huang, J. Ma, Z. Shi and Q. Jia, Coord. Chem. Rev., 2022, 456, 214391 CrossRef CAS.
- X. Kang, H. Chong and M. Zhu, Nanoscale, 2018, 10, 10758–10834 RSC.
- K. A. Benavides, I. W. H. Oswald and J. Y. Chan, Acc. Chem. Res., 2018, 51, 12–20 CrossRef CAS PubMed.
- M. Waszkielewicz, J. Olesiak-Banska, C. Comby-Zerbino, F. Bertorelle, X. Dagany, A. K. Bansal, M. T. Sajjad, I. D. W. Samuel, Z. Sanader, M. Rozycka, M. Wojtas, K. Matczyszyn, V. Bonacic-Koutecky, R. Antoine, A. Ozyhar and M. Samoc, Nanoscale, 2018, 10, 11335–11341 RSC.
- Q. Yao, X. Yuan, V. Fung, Y. Yu, D. T. Leong, D. Jiang and J. Xie, Nat. Commun., 2017, 8, 927 CrossRef PubMed.
- Y. Li, S. Li, A. V. Nagarajan, Z. Liu, S. Nevins, Y. Song, G. Mpourmpakis and R. Jin, J. Am. Chem. Soc., 2021, 143, 11102–11108 CrossRef CAS PubMed.
- B. Kim, H. Seong, J. T. Song, K. Kwak, H. Song, Y. C. Tan, G. Park, D. Lee and J. Oh, ACS Energy Lett., 2020, 5, 749–757 CrossRef CAS.
- L. Shang, J. Xu and G. U. Nienhaus, Nano Today, 2019, 28, 100767 CrossRef.
- X. Song, N. Goswami, H. Yang and J. Xie, Analyst, 2016, 141, 3126–3140 RSC.
- Z. Qiao, J. Zhang, X. Hai, Y. Yan, W. Song and S. Bi, Biosens. Bioelectron., 2021, 176, 112898 CrossRef CAS PubMed.
- E. Khatun, P. Chakraborty, B. R. Jacob, G. Paramasivam, M. Bodiuzzaman, W. A. Dar and T. Pradeep, Chem. Mater., 2019, 32, 611–619 CrossRef.
- G. Salassa, A. Sels, F. Mancin and T. Burgi, ACS Nano, 2017, 11, 12609–12614 CrossRef CAS PubMed.
- Y. Niihori, W. Kurashige, M. Matsuzaki and Y. Negishi, Nanoscale, 2013, 5, 508–512 RSC.
- M. Neumaier, A. Baksi, P. Weis, E. K. Schneider, P. Chakraborty, H. Hahn, T. Pradeep and M. M. Kappes, J. Am. Chem. Soc., 2021, 143, 6969–6980 CrossRef CAS PubMed.
- Y.-J. Zhong, J.-H. Liao, T.-H. Chiu, Y.-Y. Wu, S. Kahlal, M. J. McGlinchey, J.-Y. Saillard and C. W. Liu, Dalton Trans., 2021, 50, 4727–4734 RSC.
- P. Chakraborty, P. Bose, J. Roy, A. Nag, B. Mondal, A. Chakraborty and T. Pradeep, J. Phys. Chem. C, 2021, 125, 16110–16117 CrossRef CAS.
- P. Chakraborty, A. Nag, G. Natarajan, N. Bandyopadhyay, G. Paramasivam, M. K. Panwar, J. Chakrabarti and T. Pradeep, Sci. Adv., 2019, 5, eaau7555 CrossRef.
- K. R. Krishnadas, A. Baksi, A. Ghosh, G. Natarajan and T. Pradeep, ACS Nano, 2017, 11, 6015–6023 CrossRef CAS.
- G. Hou, C. Liu, R.-Z. Li, H. Xu, Y. Q. Gao and W. Zheng, J. Phys. Chem. Lett., 2017, 8, 13–20 CrossRef CAS.
- A. Ghosh, D. Ghosh, E. Khatun, P. Chakraborty and T. Pradeep, Nanoscale, 2017, 9, 1068–1077 RSC.
- W. A. Dar, M. Bodiuzzaman, D. Ghosh, G. Paramasivam, E. Khatun, K. S. Sugi and T. Pradeep, ACS Nano, 2019, 13, 13365–13373 CrossRef CAS PubMed.
- J. W. A. Van der Velden, J. J. Bour, J. J. Steggerda, P. T. Beurskens, M. Roseboom and J. H. Noordik, Inorg. Chem., 1982, 21, 4321–4324 CrossRef CAS.
- A. Das, T. Li, K. Nobusada, C. Zeng, N. L. Rosi and R. Jin, J. Am. Chem. Soc., 2013, 135, 18264–18267 CrossRef CAS PubMed.
- Y. Kamei, Y. Shichibu and K. Konishi, Angew. Chem., Int. Ed., 2011, 50, 7442–7445 CrossRef CAS PubMed.
- Y. Shichibu, M. Zhang, Y. Kamei and K. Konishi, J. Am. Chem. Soc., 2014, 136, 12892–12895 CrossRef CAS PubMed.
- X. Ren, J. Fu, X. Lin, X. Fu, J. Yan, R. Wu, C. Liu and J. Huang, Dalton Trans., 2018, 47, 7487–7491 RSC.
- M. P. Maman, A. S. Nair, A. M. A. H. Nazeeja, B. Pathak and S. Mandal, J. Phys. Chem. Lett., 2020, 11, 10052–10059 CrossRef CAS PubMed.
- M. P. Maman, A. S. Nair, H. Cheraparambil, B. Pathak and S. Mandal, J. Phys. Chem. Lett., 2020, 11, 1781–1788 CrossRef CAS PubMed.
- Z. He, Y. Yang, J. Zou, Q. You, L. Feng, M.-B. Li and Z. Wu, Chem. - Eur. J., 2022, 28, e202200212 CAS.
- Q. Li, S. Yang, J. Chai, H. Zhang and M. Zhu, Nanoscale, 2022, 14, 15804–15811 RSC.
- Q. Li, S. Wang, K. Kirschbaum, K. J. Lambright, A. Das and R. Jin, Chem. Commun., 2016, 52, 5194–5197 RSC.
- N. Xia, J. Yuan, L. Liao, W. Zhang, J. Li, H. Deng, J. Yang and Z. Wu, J. Am. Chem. Soc., 2020, 142, 12140–12145 CrossRef CAS PubMed.
- T. Higaki, C. Liu, Y. Chen, S. Zhao, C. Zeng, R. Jin, S. Wang, N. L. Rosi and R. Jin, J. Phys. Chem. Lett., 2017, 8, 866–870 CrossRef CAS PubMed.
- L. Tang, X. Kang, S. Wang and M. Zhu, Langmuir, 2019, 35, 12350–12355 CrossRef CAS PubMed.
- X. Lin, C. Liu, K. Sun, R. Wu, X. Fu and J. Huang, Nano Res., 2019, 12, 309–314 CrossRef CAS.
- X. Liu, W. Xu, X. Huang, E. Wang, X. Cai, Y. Zhao, J. Li, M. Xiao, C. Zhang, Y. Gao, W. Ding and Y. Zhu, Nat. Commun., 2020, 11, 3349 CrossRef CAS PubMed.
- Z.-J. Guan, J.-L. Zeng, S.-F. Yuan, F. Hu, Y.-M. Lin and Q.-M. Wang, Angew. Chem., Int. Ed., 2018, 57, 5703–5707 CrossRef CAS PubMed.
- X. Fu, X. Lin, X. Ren, H. Cong, C. Liu and J. Huang, Chin. Chem. Lett., 2021, 32, 565–568 CrossRef CAS.
Footnote |
† Electronic supplementary information (ESI) available: Synthesis and characterization of the [Au23SCy16]−[TOA]+ and [Au6(dppp)4]2+Cl2 nanocluster (PDF) and density functional theory calculation on the Au–S bond dissociation energy. See DOI: https://doi.org/10.1039/d3ra01606d |
|
This journal is © The Royal Society of Chemistry 2023 |
Click here to see how this site uses Cookies. View our privacy policy here.