DOI:
10.1039/D3RA02698A
(Paper)
RSC Adv., 2023,
13, 25350-25359
A polystyrene-based ESIPT fluorescent polymeric probe for highly sensitive detection of chromium(VI) ions and protein staining†
Received
24th April 2023
, Accepted 11th July 2023
First published on 23rd August 2023
Abstract
A “two-step” preparation method of an excited-state intermolecular proton transfer (ESIPT) fluorescent polymer (f-PP) is reported here. The synthesis of f-PP involves the acetylation of polystyrene and a “multicomponent one pot” reaction. The as-prepared polymer bears a group of ESIPT fluorescent units, enabling it to exhibit high brightness, moderate solubility and ESIPT fluorescence. F-PP gives off tautomeric bright green fluorescence under UV-tamp and the dual-emission could be specifically suppressed by Cr(VI). This phenomenon cannot be elicited by other competing species. On this basis, an ESIPT polymeric probe-based method for the determination of Cr(VI) was developed, offering high sensitivity (19.5 nM) and selectivity. The f-PP was successfully used to detect Cr(VI) in real water samples by standard adding methods, indicating its application feasibility.
1. Introduction
The rapid development of industry has caused serious heavy metal ion contamination of the water environment and elicited strong concern throughout the world.1–3 Among the heavy metal ion pollutants, the dispersion of cadmium ions is one of the most concerning environmental issues. Chromium tends to exist in two stable oxidation sates in the environment, namely Cr(III) and Cr(VI). Cr(III) is an indispensable trace ion for biological processes, yet, Cr(VI) is one of the most toxic and ubiquitous pollutants to the ecosystem due to its mutagenic and carcinogenic features.4,5 Some biochemical experiments demonstrated that the Cr(VI) ion was easily ingested into cells and reduced to Cr(III), which tends to generate radicals of various active species.6 Once Cr(VI) is taken into the cells, it can disturb several biochemical processes in cells, which can elicit toxicity to them and result in DNA damage via activating DNA reaction.7 As a result, the World Health Organization (WHO) has suggested the maximum allowable concentration for Cr(VI) in the ground and drinking water should not exceed 50 μg L−1, which were set by many nations.8 Consequently, to develop a sensitive, simple and rapid analytical method is highly desirable for monitoring Cr(VI) in water, environmental, food and biological samples.
Many analytical methods are available for the detection of Cr(VI) in various samples. Among them, atomic absorption spectroscopy (AAS),9 surface plasmon resonance (SPR),10 UV-visible spectroscopy (UV-vis),11 surface-enhanced Raman scattering (SERS),12 high pressure liquid chromatography-inductively coupled plasma mass spectrometry (HPLC-MS),13 X-ray absorption spectrometry (X-AS)14 and electrochemistry (EC)15 are frequently used for the detection of Cr(IV) in aqueous samples. Although the above techniques can afford high sensitivity and multi-element analysis, most of them involve complicated operating procedures and expensive equipment, which are associated with complex sample preparation and are time-consuming.16 Currently, fluorescent molecular probes have drawn great attention due to their high sensitivity, instantaneous response, simplicity and low-cost.17 So far, a large number of fluorescent probes including small organic small dyes,18 nanostructures (for instance, organic or inorganic nanoparticles, metal nanoclusters, quantum dots, carbon dots) and fluorescent polymers have been used in the design of probes for the detection of Cr(IV).19–25 However, the mentioned fluorophores are often suffered from insufficient sensitivity, cytotoxicity, complicated synthetic process, photo-bleaching or high fabrication cost. Developing new fluorescent dyes for probe application that are more sensitive, cheaper, low toxic, robust and facile for the highly selective and sensitive detection of Cr(IV) ions is still very necessary.
Compared to small molecular fluorophores, fluorescent polymers have the advantages of good stability, high fluorescence brightness, simple synthesis, and high safety. The polymeric fluorophores generally originate from the conjugated polymers (CPs) which bear conjugated main chain or π-aromatic scaffolds.26,27 Because of their excellent photo-physical properties, CPs has been employed for designing molecular probes for the detection of heavy metal ions or organic active molecules in the past decades.28–30 CP-based probes often include two major functional units, namely conjugated backbones and functional side chains. In the polymeric probes, the backbone structures endow the probes with different intrinsic fluorescent features, offering ‘‘turn on” or ‘‘turn off” fluorescent switch during analyte recognition.31,32 However, except for few polymers, such as the reported polydiacetylene and polythiophene,33,34 the absorption and fluorescence spectra can alter a lot after their interaction with targets, almost polymeric fluorophores require the modification with different functional groups, including ionization of side chains, quenching groups, chromogenic dyes, and responsive units to obtain active sites for guest recognition.35,36 The modified side chains many further enhance the sensing performance of probes, enabling CP-based probes to show higher sensitivity than that of small molecular probes.37,38 Actually, some non-conjugated polymers such as poly(amidoamine), polyurethane, polyethylenimine, poly(ether amide) can also exhibit strong fluorescence when they come into existence in nanostructures under favorable conditions, which can employed for the design of probes.39,40 However, these nano-fluorophores usually bear a single functional group and they must be grafted with additional groups to meet the requirements of probe recognition. Such modifications usually lead to the destruction of nanostructures and discount fluorescence advantages of nano-fluorophores.
During previous efforts to construct small excited-state intermolecular proton transfer (ESIPT) fluorescent dyes,41,42 we demonstrated that the ESIPT molecules can give off tautomeric emission benefited from the formation of intermolecular hydrogen bonds. In this study, a Cr(VI)-specific probe was designed and synthesized via simple modification of polystyrene polymers. For the preparation of ESIPT fluorescent polymers, excited-state intermolecular proton transfer (inter-ESPT) moieties were grafted to the polymers as fluorophores and recognition units, and the amino and hydroxyl groups in the ESIPT scaffolds were used to improve the solubility. The ESIPT fluorescent polymer-based probe exhibited excellent ESIPT fluorescent features and a quenching effect on Cr(VI).
2. Experimental section
2.1. Materials and instruments
All reagents and chemicals were used directly without any further purification. Polystyrene and acetyl chloride were purchased from China Pharmatech Ltd. (Shanghai, China). 4-(Diethylamino)-2-hydroxybenzaldehyde, malonitrile and ammonium acetate were obtained from Macklin (Shanghai, China). The ionic solutions were prepared by dissolving their relative sodium and chloride salts, respectively. Deionized water was employed in all experiments in this study.
The 1H- and 13C-NMR spectra were measured using an AV-600 spectrometer (Bruker, Switzerland) with tetramethylsilane (TMS; δ = 0 ppm) as an internal reference. The UV-visible absorption experiments were performed on a UV-1800 spectrophotometer (Shimadzu, Japan). Fluorescence spectra were obtained from an F-7000 spectrophotometer (Hitachi, Japan). The time-resolved fluorescence spectra were recorded on the transient fluorescence spectrometer (Edinburgh-FLS1000, England). The fluorescence quantum yields were measured by HORIBA FluoroMax-4P (HORIBA Jobin Yvon).
2.2. Synthesis of probe f-PP
As shown in Scheme 1, ESIPT fluorescent polymer (f-PP) was prepared by a two-step procedure of acetylation and “multicomponent one pot” reaction. Briefly, acetyl polystyrene was synthesized using Friedel–Crafts acetylation method. The acetylated polystyrene polymers were then prepared via “multicomponent one pot” reaction method. Details of synthesis and characterization of f-PP can be found in ESI.†
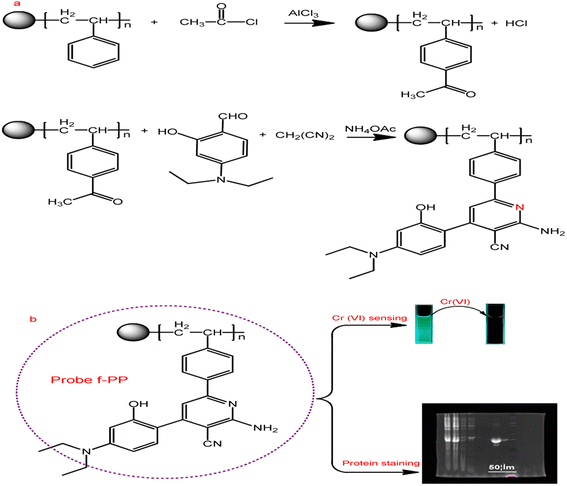 |
| Scheme 1 Synthesis strategy of the ESIPT fluorescent polymeric probe f-PP and its application. (a) Synthetic route of f-PP; (b) the applications of f-PP to Cr(VI)-sensing and protein staining. | |
2.3. General spectra measurements of f-PP
The stock solution of f-PP (0.01 g L−1) was prepared by dissolving f-PP products in DMF-HEPES mixed system (20 mM, pH 7.2, 3
:
7, v/v). The Cr(VI) stock solution (0.1 M L−1) was obtained from the dissolution of 2.942 g potassium dichromate in 100 mL deionized water. The other solutions containing competitive species (0.1 M) were prepared by dissolving responsive compounds with deionized water. For the measurements of Cr(VI), different concentrations of competing metal ions or anion were introduced in the f-PP-containing solutions in portions. After 6 min-incubation at room temperature, the resulting mixtures were measured by UV-visible absorption and fluorescence spectrometry.
2.4. Detection of Cr(VI) in real water samples
The lake water and tap water were collected from our campus (Changsha, China). The wastewater was also obtained from drainage ditch in our campus. For the sample preparation, 10 mL wastewater was collected in sample tubes. These samples were then filtered three times using a 0.17 μm membrane to reject any solid suspensions. Finally, the filtrated samples were spiked with different Cr(VI) concentrations and their fluorescence spectra were measured as above. The lake water and tap water samples were directly treated with the standard additions of Cr(VI) and the fluorescence spectra were monitored.
2.5. Protein staining using f-PP
The protein samples were the expressed products of Mambalglin gene and the inclusions of Escherichia coli, which were obtained from the State Key Laboratory of Bioorganic and Natural Products Chemistry, Shanghai Institute of Organic Chemistry, Chinese Academy of Sciences (Shanghai, China) in this study. Firstly, the proteins derived from Mambalglin gene or the protein inclusions of Escherichia coli were separated and fixed via SDS-PAGE. Next, the protein bands (https://www.baidu.com/link?url=79xGmSk81dLifzk1gXLc9Py_BEF3NT0GHcZv3EHgHIB75iloi0lg68-zvmYUjsl84NFLa2Zsi3_0Wkfts_mcTa%26wd=%26eqid=ec8b59280000aabf000000066428feeb) was stained with f-PP or Coomassie brilliant blue R 250, respectively. The stained electrophoresis gel bands were then decolorized by immersing in an ethanol–water system (1
:
4, v/v) for 1 h and washed with HEPES (10 mM, pH 6.5) for three times. Finally, fluorescence scanning of the resulting electrophoresis gels was performed on a Typhoon 9410 imaging system. Coomassie brilliant blue R250 was employed as a reference to stain the same proteins. The f-PP staining solution was prepared by dissolving 0.2 mg f-PP in 5 mL DMF. When used, the f-PP-containing solution was diluted to 100 mL with a DMF–water mixed system (3/7, v/v) to obtain a 2.0 μg mL−1 f-PP staining solution. 0.25% Coomassie brilliant blue R250 solution was obtained from dissolving 0.25 g Coomassie in 45 mL methanol, and followed by adding 45 mL water and 10 mL glacial acetic acid.
3. Results and discussion
3.1. Synthesis and characterization
As shown in Scheme 1, the inter-ESPT fluorescent polystyrene polymers (f-PP) were synthetised (http://www.baidu.com/link?url=lh5i62PCwBezWEgITjCZxME-1Okvhcy8G8Ygz7kgfCYFG-h91-qVDLYsZxWBCiISwUVSXwtxycW5dKNGIbEV3xOpI0uKv_3VY3YqpAxaRhG) through acetylation and “multicomponent one pot” reaction with moderate yield. The structures of the prepared f-PP have been confirmed by 1H-NMR and 13C-NMR (Fig. S1-1, S1-2, ESI†).
The structure of f-PP was also characterized using XPS analysis. As shown in Fig. 1 in the survey spectrum, the probe f-PP exhibits three distinct signals at 287.5, 402.5 and 534 eV, which corresponds to C 1s, N 1s and O 1s peaks, respectively. The XPS analysis results indicated the existence of 72.1% C, 22.3% O and 5.6% N in the f-PP molecule system. Since the carbon atoms present in the probes f-PP are faced to four chemical microenvironments, four different bands at 288.53, 286.57, 284.71, and 284.41, eV can be observed in the high-resolution C 1s spectrum (Fig. 1b), attributing to the C
O/C
N, C–O, C–N, and C
C/C–C species, respectively. The high-resolution spectrum of O 1s shows three bands at 533.74, 532.77 and 531.68 eV, ascribing to C–OH/C–O–C, C–O, and C
O binding atoms, respectively. The N 1s spectrum (Fig. 1d) showed two obvious peaks at 401.057, and 399.24 eV, related to N–H, and C–N bonds, respectively.
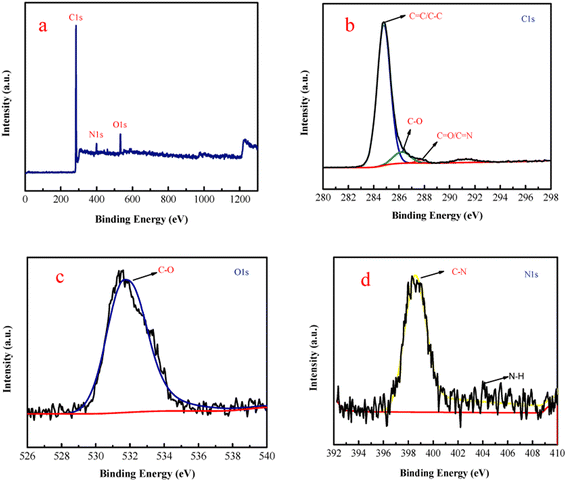 |
| Fig. 1 (a) XPS survey spectrum of f-PP and high-resolution spectra of C 1s (b), O 1s (c), and N 1s (d). | |
In addition, the as-prepared ESIPT polymer was further confirmed using infrared spectrometry. It is clear that two new peak at 3265 and 1507 cm−1 appeared in the spectrum of f-PP, ascribed to the –NH2 (connecting to benzene ring) stretching vibration compared to native acetyl polystyrene (Fig. S2a and b†). As for the spectrum of –CN groups, a distinct new peak at 2227 cm−1 can be observed. These results imply the presence of large numbers of amino and cyano groups on the side chains of f-PP.
3.2. Photophysical properties and response behavior of f-PP
In order to investigate the optical performance of f-PP, we investigated the UV-visible absorption and fluorescence spectra of f-PP. As shown in Fig. 2a three absorption bands located at 255 nm, 355 nm and 440 nm can be observed in the UV-vis spectrum. The former two absorption bands can be assigned to the n–π* transitions of the C
O bonds, and the absorption band at 440 nm can be ascribed to the n–π* transition of p–π conjugated systems between benzenes and pyridine rings. When added with Cr(VI), the absorption peaks of f-PP containing solution increased significantly. This change in absorption spectra may generated from the aggregation of f-PP chain induced by Cr(VI), which is agree with the reported event in literature.43 This result reveals that the interaction between f-PP and Cr(VI) occurred. Fluorescence spectra of f-PP indicates that there are two obvious emission peaks at 310 nm and 512 nm, in which the short wavelength fluorescence is attributed to the normal form and another one corresponding to the ESIPT emission of f-PP. Upon the addition of Cr(VI), the dual-emission was obviously quenched (Fig. 2b and c). Considering that the inter-ESPT reaction is seriously affected by solvents, we evaluated the fluorescence behavior of f-PP in different solvents. As shown in Fig. 2d, f-PP exhibit normal fluorescence in conventional aprotic solvents and can give off tautomeric fluorescence in protic solvents. Surprisingly, the glacial acetic acid can also cause triple emission, implying that there is another luminescence mechanism involved. These results confirm that ESIPT process of f-PP requires the assistance of solvent molecules with hydrogen receptor/donor.
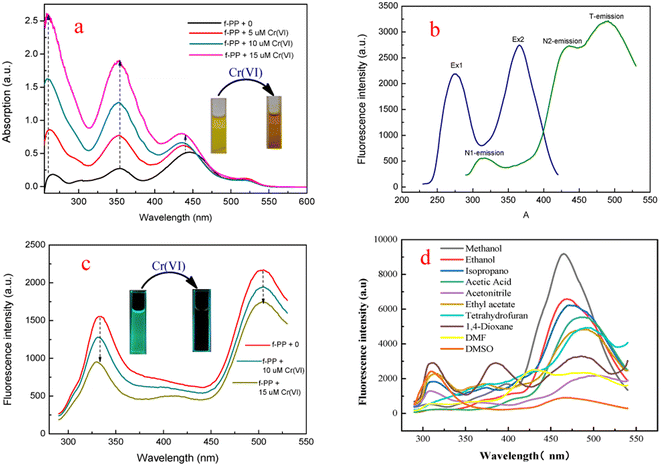 |
| Fig. 2 Absorption and fluorescence spectra of f-PP and the f-PP with the addition of Cr(VI). (a) Absorption spectra of f-PP and the f-PP with the addition of Cr(VI) in DMF-HEPES mixed system (3/7, v/v, pH 7.2). (b) Excitation and fluorescence spectra of f-PP in the same DMF-HEPES system. (c) The fluorescence spectra of f-PP in the presence of different concentrations of Cr(VI). (d) Fluorescence spectra of f-PP in different solvents. Ex = 275 nm. | |
To further understand the fluorescence mechanism, we monitored the fluorescent lifetime of f-PP in DMF–HEPES buffer (20 mM, pH 7.2, 3
:
7, v/v) at different emission bands. As shown in Fig. S2c and d,† the fluorescence lifetimes of the f-PP at 310 and 512 nm are 1.176 ns and 2.714 ns, respectively. It is clear that the fluorescence decay of f-PP at 512 nm is significantly slower than that at 310 nm, which imply that the extended time may be used to undertake energy conversion between the two tautomers of f-PP.
3.3. Studies on the selectivity and anti-interference of f-PP
The high selectivity of probes for detecting analytes over the potentially competing species is one of the important indicators of its performance. For this purpose, the competitive measurements on Cr(VI) were used to evaluate the selectivity of the probe f-PP for Cr(VI). As shown in Fig. 3a, only Cr(VI) elicited a remarkable fluorescence quenching, whereas all conventional metal ions, including alkali, alkali-earth, transition metal ions (K+, Na+, Ca2+, Mg2+, Ba2+, Ag+, Zn2+, Mn2+, Co2+, Pd2+, Hg2+, Ni2+, Cd2+, Fe3+, Cr3+, Al3+, Pr3+, Ce4+) and anions (F−, Cl−, I−, CO32−, SO42−, NO3−, HPO42−) merely result in negligible change in the fluorescence, even when the alkali and alkali-earth K+, Na+, Mg2+ and Ca2+ were added up to micromolar levels. The results reveal the specific oxidation of f-PP by Cr(VI) and exhibit the ability to specially detect Cr(VI).
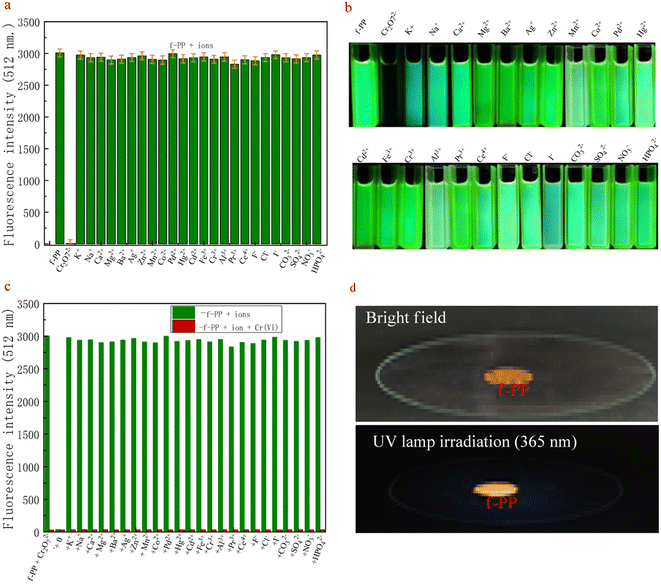 |
| Fig. 3 Fluorescence change of f-PP upon the addition of 10 μM Cr(VI) and different ions (100 μM). (a) Fluorescence decrease at 512 nm of f-PP in the presence of Cr(VI) and various species in HEPES-DMF system (10 mM, pH = 7.2, 7 : 3, v/v) at room temperature (λex = 275 nm. Slits: 2.5 nm). (b) The fluorescence images of the probe f-PP with the addition of Cr(VI) and other ions, respectively; (c) red bars represent the f-PP solutions in the presence of Cr(VI) (15 μM) and various ions (150 μM); (d) the images of the product f-PP under door light and UV-lamp (365 nm), respectively. | |
Apart from selectivity, the anti-interference capability is another essential parameter to be thought over when estimating probe performance. Consequently, we examined the changes in fluorescence intensity by adding different metal ions (50 μM), including K+, Na+, Ca2+, Mg2+, Ba2+, Ag+, Zn2+, Mn2+, Co2+, Pd2+, Hg2+, Cd2+, Fe3+, Cr3+, Al3+, Pr3+, Ce4+, F−, Cl−, CO32−, SO42−, NO3−, urea, citric acid, glucose, sucrose, methanol and ethanol to the f-PP solutions containing 20 μM Cr(VI) ions, according to the procedure described in the Experimental section. The fluorescence spectra displayed in Fig. 3b suggest that Cr(VI) effectively lowered the fluorescence intensity of f-PP even with the addition of other metal ion species. These results confirmed that the proposed ESIPT probes f-PP could act as a unique sensing.
3.4. Condition optimization
In order to fully explore the sensing performance of f-PP, we optimized the reaction conditions. Medium pH is an important factor for the performance of probes because of that the pH of real wastewater samples may vary from one to the other. Therefore, the fluorescent intensity of f-PP and the f-PP with the addition of Cr(VI) was inspected under moderate broad pH. As shown in Fig. 4a, the ESIPT fluorescence of f-PP was weak at acidic and basic pH because of the protonation of –NH2 groups at acidic pH and the deprotonation of –OH groups on f-PP, resulting to develop “protective shell” with positive (acidic pH) and negative (basic pH) charges on the side chain of f-PP, which can affect ESIPT process, accompanied by fluorescence quenching of f-PP. It is clear that the maximum fluorescence intensity appeared at HEPES pH 7.2, suggesting that f-PP were stable and exhibited maximum intensity at HEPES pH 7.2. From the perspective of the oxidation of Cr(VI), Cr2O72− had strong oxidability in acid medium. Meanwhile in basic solutions Cr2O72− will convert to CrO42−, which still occur in high valence state Cr(VI) and hold moderate oxidation capacity. Therefore, HEPES pH 7.2 was selected as the optimum pH for sensing Cr(VI).
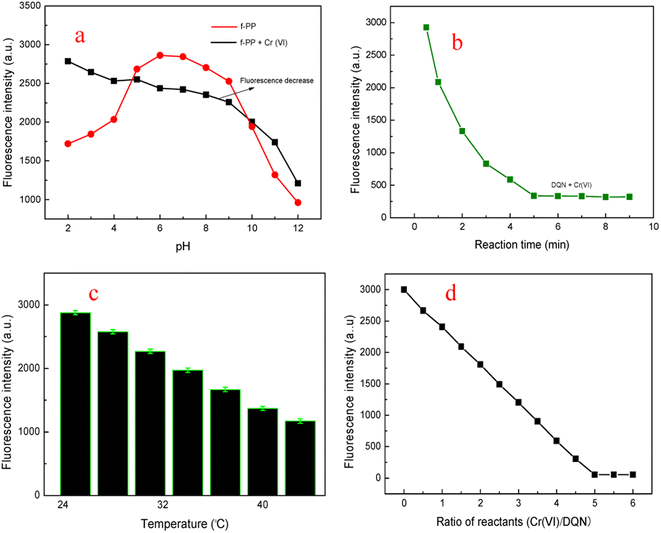 |
| Fig. 4 The effect of reaction conditions on the fluorescent performance of f-PP and f-PP + Cr(VI). (a) pH; (b) reaction time; (c) temperature; (d) ratio of f-PP to Cr(VI). The concentrations of f-PP were 20 μg L−1. Ex = 275 nm; Em = 512 nm. | |
The influence of reaction time on the fluorescent behavior of f-PP in the presence of Cr(VI) were investigated. The fluorescence spectra of f-PP-containing solutions with the addition of Cr(VI) were recorded at different reaction times from 1.0 to 9.0 min at room temperature. The fluorescence intensity of the f-PP-Cr(VI) mixture was gradually decreased with increasing reaction time extension owing to the oxidization of f-PP, extending the minimum value of fluorescence at 5 min. After 5 min-reaction, the fluorescence intensity of the f-PP-Cr(VI) solutions has hardly decreased again, which stimulates that the oxidization of f-PP reaches to saturation (Fig. 4b). Therefore, 5 min was selected as the best reaction time for the fluorescence quenching of f-PP.
Next, we evaluated the effect of temperature on the oxidation reaction of Cr(VI) with f-PP. As shown Fig. 4c, the fluorescent intensity of the f-PP-Cr(VI) mixed system decreased slowly to the elevated temperature from room temperature to 45 °C. Decidedly, the increased temperature can provide more energy to promote the forward ox-reductive reaction between f-PP and Cr(VI). For the consideration of experimental operation, room temperature was used as the reaction temperature for the fluorescence quenching of f-PP. Finally, the effect of the ratio f-PP/Cr(VI) on the fluorescence quenching was also investigated. Fig. 4d showed that when the ratio of f-PP to Cr(VI) reaches to 1
:
5, a plateau of fluorescence quenching appears. This may suggest that the structure stability of f-PP destroyed, accompanied with the fluorescence quenching.
3.5. Determination of Cr(VI) in real water samples using f-PP
The feasibility of the fluorescent probe f-PP for the detection of Cr(VI) in real water samples was investigated. Firstly, the calibration graph was established by adding different concentrations of Cr(VI) into the f-PP solutions. As shown in Fig. 5a, with the increase of Cr(VI) concentration, the ESIPT emission intensity of f-PP is progressively decreased, and a good linear relationship (R2 = 0.99748) was showed between the fluorescence quenching and the Cr(VI) concentration in the range of 0–40 μM (Fig. 5b). The limit of detection was calculated as 19.5 nM (3σ/slope), which is lower than the permissible levels of Cr(VI) in water with 0.95 μM (0.05 mg L−1) recommended by the World Health Organization and US Environmental Protection Agency.44,45 The detection limit of our proposed f-PP based method is lower than that of the reported ones in the literature.22,46.
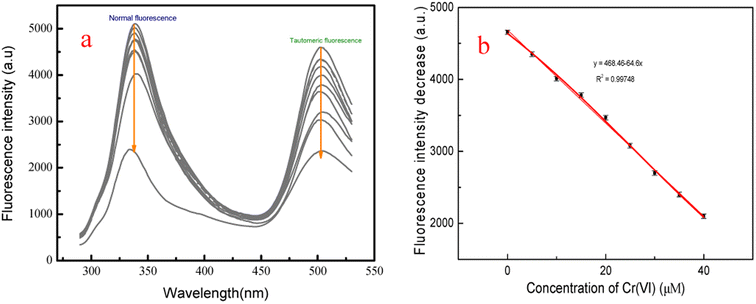 |
| Fig. 5 (a) Fluorescent spectra of 5 μg L−1 f-PP in HEPES-DMF buffer (20 mM, HEPES : DMF = 7/3, pH = 7.2, v/v) in the presence of increasing concentrations of Cr(VI) (from top to bottom: 0, 10.0, 15.0, 20.0, 25.0, 30.0, 35.0 and 40 μM), excited at 275 nm; (b) plot of the fluorescent intensity at 512 nm of f-PP as a function of the concentrations of Cr(VI). | |
Subsequently, the measurements of Cr(VI) in real water samples was carried out with standard addition method. As shown in Table 1, the concentrations of Cr(VI) in tap water and lake water is undetected. After the addition of fixed Cr(VI) amount, the recoveries of Cr(VI) range from 98.0 to 103.2% with the relative standard deviations (RSD) of 1.75–2.55%. The tracked concentrations of Cr(VI) in each groups are in good agreement with the initial added ones. Besides the above natural water samples, the practicality of f-PP for the detection of Cr(VI) in waste water was also evaluated using f-PP-based probe. The results showed that there are no significant differences for the determination of Cr(VI), indicating the accuracy of our proposed method. These results demonstrated that the ESIPT f-PP as a novel fluorescent probe has application feasibility for the selective determination of Cr(VI) in real water samples.
Table 1 Determination of Cr(VI) in real water samples
Samples |
Added (μM) |
Found (μM) |
Recovery (%) |
RSDa (%, n = 3) |
Relative standard deviations (RSD) of measurements are calculated from three independent experiments. |
Tap water |
2.50 |
2.45 |
98.0 |
1.76 |
10.00 |
10.32 |
103.2 |
1.75 |
30.00 |
29.75 |
99.2 |
2.29 |
Lake water |
2.50 |
2.55 |
102.0 |
2.55 |
10.00 |
10.21 |
101.0 |
1.87 |
30.00 |
30.47 |
101.6 |
2.05 |
Waste water |
2.50 |
2.79 |
101.5 |
1.96 |
10.00 |
10.56 |
103.0 |
2.25 |
30.00 |
30.74 |
101.6 |
1.82 |
3.6. Application of f-PP to fluorescence imaging of in vitro protein
We evaluated the performance of f-PP for protein imaging in vitro using the expressed proteins of Mambalglin gene as analytic models. Firstly, the inclusions of the gene transduced Escherichia coli were separated and fixed by sodium dodecyl sulfate-polyacrylamide gel electrophoresis (SDS-PAGE). Next, the electrophoretic gel bands with different proteins were immersed in the f-PP-containing solution for 2 h, followed by washing three times with PBS. Finally, fluorescence imaging is carried out on the electrophoretic gel bands. As displayed in Fig. 6, f-PP could stain all the proteins which were colored by Coomassie brilliant blue R250 in the electrophoretic gels. These results reveal that the f-PP can anchor the expressed proteins of Mambalglin gene and the other proteins in Escherichia coli. Compared to Coomassie brilliant blue R250, the main advantages of f-PP are: (1) it possesses high sensitivity owing to the inherent natures of fluorometry; (2) the reagent dosage is low in the gel chromatography; CBBR250 is usually used at the concentration of 0.1%, while the usage of f-PP is at a relatively lower concentration (<10−3 μM). (2) The decolorization treatment of the DQN is simpler than that of CBBR250 (immersed in ethanol for 30 min).
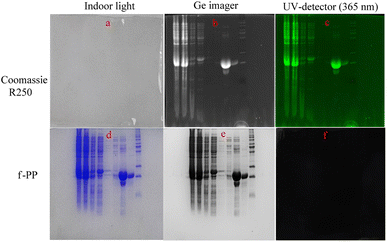 |
| Fig. 6 Fluorescence images of the proteins in SDS-PAGE electrophoresis gel bands stained with f-PP or Coomassie brilliant blue R250. (a–c) The expressed proteins of Mambalglin gene and the inclusions of Escherichia coli in electrophoresis gels were stained with f-PP. The samples are as follows: (1) bacterial extract of Escherichia coli expressed with Mambalglin gene; (2) the centrifugal supernate of bacterial extract containing Mambalglin; (3) the penetrating fluid of the centrifuged supernate through nickel column; (4) the eluent separated by Ni-column and eluted without imidazole (the first elution); (5) the eluent separated by Ni-column and eluted without imidazole (the second elution); (6) the eluent separated by Ni-column and eluted with 20 mmol L−1 imidazole; (7) the eluent separated by Ni-column and eluted with 300 mmol L−1 imidazole; (8) the eluent separated by Ni-column and eluted 500 mmol L−1 imidazole (the first elution); (9) the eluent separated by Ni-column and eluted 500 mmol L−1 imidazole (the second elution); (10) protein ruler. (d–f) The same expressed proteins and their protein inclusions in electrophoresis gels were stained with Coomassie brilliant blue R250. | |
4. Conclusions
In summary, we have developed an inter-ESPT-based polymeric fluorescent probe for Cr(VI) via a “multicomponent one pot reaction” method. The as-prepared polymeric probe (f-PP) was endowed with multiple inter-ESPT units, enabling it to exhibit the advantage of large Stokes'-shift (http://www.baidu.com/link?url=68s935ol4-Dyo6BP21y1kfng_UREtF55_vB2efqU06r_pLwp6AhJ6_vi-xvEwds-4g-oWwj2JensG60DSCJW0a), high brightness and good stability. The probe f-PP can show two light ways of normal fluorescence in aprotic solvents and tautomeric fluorescence in protic solvents, respectively. Up on the addition of Cr(VI), the ESIPT fluorescence of f-PP was obviously suppressed, while the presence of other competitive ions could merely cause negligible changes in fluorescence. Taking advantages of Cr(VI) to quench the ESIPT emission of f-PP, we established a novel method for the detection of Cr(VI). The our proposed probe exhibits satisfactory sensitivity and selectivity for determination of Cr(VI) in real water samples, offering the detection limits of 19.5 nM. Given the mentioned advantages, we believe that the inter-ESPT-based polymeric fluorescent probes would have potential applications in environmental and bio-analysis.
Author contributions
Fuchun Gong: supervision, investigation designed and planned; Jiaoyun Xia: fluorescence spectrum test and analysis. Xiaoling Qin: the design and synthesis of the organic compounds, wrote the manuscript. Pan Ma: characterization of the target molecular and interpretation of the NMR spectra. Lusen Chen: protein staining experiments. Guoqiang Zhou and Lujie Xu: the practical sample detection, cell incubation and fluorescence imaging experiments. All authors read and approved the final manuscript.
Conflicts of interest
The authors declare that they have no known competing financial interests.
Acknowledgements
This work was financially supported by the Research Foundation of Education Bureau of Hunan Province (No. 18A141) and the State Key Laboratory of Bio-organic and Natural Product Chemistry (No. SKLBNPC19250).
References
- M. Elavarasi, S. A. Alex, N. Chandrasekaran and A. Mukherjee, Simple fluorescence-based detection of Cr(III) and Cr(VI) using unmodified gold nanoparticles, Anal. Methods, 2014, 6(24), 9554–9560 RSC
. - X. Zhang, M. Zhu, Y. Jiang, X. Wang, Z. Guo, J. Shi, X. Zou and E. Han, Simple electrochemical sensing for mercury ions in dairy product using optimal Cu(II)-based metal-organic frameworks as signal reporting, J. Hazard. Mater., 2020, 400, 123222 CrossRef CAS PubMed
. - S. Dey, A. Kumar, P. K. Mondal, K. M. Modi, D. Chopra and V. K. Jain, An oxacalix[4]arene derived dual sensing fluorescent probe for the detection of As(V) and Cr(VI) oxyanions in aqueous media, Dalton Trans., 2020, 49(22), 7459–7466 RSC
. - T. Madrakian, A. H. Mohammadzadeh, S. Maleki and A. Afkhami, Preparation of polyacrylonitrile nanofibers decorated by N-doped carbon quantum dots:application as a fluorescence probe for determination of Cr(VI), New J. Chem., 2018, 42(23), 18765–18772 RSC
. - B. K. John, N. John, S. Mathew, B. K. Korah, M. S. Punnoose and B. Mathew, Fluorescent carbon quantum dots as a novel solution and paper strip-based dual sensor for the selective detection of Cr(VI) ions, Diamond Relat. Mater., 2022, 126, 109138 CrossRef CAS
. - J. B. Vincent, Elucidating a biological role for chromium at a molecular level, Acc. Chem. Res., 2000, 33, 503–510 CrossRef CAS PubMed
. - A. Levina, R. Codd, C. T. Dillon and P. A. Lay, Chromium in biology: toxicology and nutritional aspects, Prog. Inorg. Chem., 2002, 51, 145–250 Search PubMed
. - Z. Wang, X. Wang, W. Niu and L. Feng, A specific fluorescence probe for chromium(VI) anions and its application, Sens. Actuators, B, 2017, 244, 727–731 CrossRef CAS
. - E. Rossi, M. I. Errea, M. M. F. de Cortalezzi and J. Stripeikis, Selective determination of Cr(VI) by on-line solid phase extraction FI-SPE-FAAS using an ion exchanger resinas sorbent: An improvement treatment of the analytical signal, Microchem. J., 2017, 130, 88–92 CrossRef CAS
. - S. A. Abayzeed, R. J. Smith, K. F. Webb, M. G. Somekh and C. W. See, Responsivity of the differential-intensity surface plasmon resonance instrument, Sens. Actuators, B, 2016, 235, 627–635 CrossRef CAS
. - H. Sereshti, M. V. Farahani and M. Baghdadi, Trace determination of chromium(VI) in environmental water samples using innovative thermally reduced graphene (TRG) modified SiO(2) adsorbent for solid phase extraction and UV-vis spectrophotometry, Talanta, 2016, 146, 662–669 CrossRef CAS PubMed
. - N. D. Jayram, D. Aishwarya, S. Sonia, D. Mangalaraj, P. S. Kumar and G. M. Rao, Analysis on superhydrophobic silver decorated copper Oxide nanostructured thin films for SERS studies, J. Colloid Interface Sci., 2016, 477, 209–2019 CrossRef CAS PubMed
. - H. J. Wang, X. M. Du, M. Wang, T. C. Wang, H. Ou-Yang, B. Wang, M. T. Zhu, Y. Wang, G. Jia and W. Y. Feng, Using ion-pair reversed-phase HPLC ICP-MS to simultaneously determine Cr(III) and Cr(VI) in urine of chromate workers, Talanta, 2010, 81(4), 1856–1860 CrossRef CAS PubMed
. - E. Marguí, C. Fontàs, M. Toribio, M. Guillem, M. Hidalgo and I. Queralt, Determination of Water-Soluble Hexavalent Chromium in Clinker Samples by Wavelength-Dispersive X-ray Fluorescence Spectrometry after Concentration in Activated Layers, Appl. Spectrosc., 2010, 64(5), 547–551 CrossRef PubMed
. - W. Jin, G. Wu and A. Chen, Sensitive and selective electrochemical detection of chromium(VI) based on gold nanoparticle-decorated titania nanotube arrays, Analyst, 2014, 139(1), 235–241 RSC
. - M. Zheng, Z. Xie, D. Qu, D. Li, P. Du, X. Jing and Z. Sun, On-off-on fluorescent carbon dot nanosensor for recognition of chromium(VI) and ascorbic acid based on the inner filter effect, ACS Appl. Mater. Interfaces, 2013, 5(24), 13242–13247 CrossRef CAS PubMed
. - X. Luo, P. Bai, X. Wang, G. Zhao, J. Feng and H. Ren, Preparation of nitrogen-doped carbon quantum dots and its application as a fluorescent probe for Cr(VI) ion detection, New J. Chem., 2019, 43(14), 5488–5494 RSC
. - R. Iftikhar, I. Parveen, Ayesha, A. Mazhar, M. S. Iqbal, G. M. Kamal, F. Hafeez, A. L. Pang and M. Ahmadipour, Small organic molecules as fluorescent sensors for the detection of highly toxic heavy metal cations in portable water, J. Environ. Chem. Eng., 2023, 11(1), 109030 CrossRef CAS
. - Y. Zhao, Y. Sun, Y. Jiang, S. Song, T. Zhao, Y. Zhao, X. Wang, B. Li, B. Yang and Q. Lin, Fluorescent probe gold nanodots to quick detect Cr(VI) via oxidoreduction quenching process, Sci. China: Chem., 2018, 62(1), 133–141 CrossRef
. - W. Qing, K. Chen, Y. Yang, Y. Wang and X. Liu, Cu2+-doped carbon dots as fluorescence probe for specific recognition of Cr(VI) and its antimicrobial activity, Microchem. J., 2020,(152), 104262 CrossRef CAS
. - L. Sheng, B. Huangfu, Q. Xu, W. Tian, Z. Li, A. Meng and S. Tan, A highly selective and sensitive fluorescent probe for detecting Cr(VI) and cell imaging based on nitrogen-doped graphene quantum dots, J. Alloys Compd., 2020, 820, 15319 CrossRef
. - L. M. T. Phan, S. H. Baek, T. P. Nguyen, K. Y. Park, S. Ha, R. Rafique, S. K. Kailasa and T. J. Park, Synthesis of fluorescent silicon quantum dots for ultra-rapid and selective sensing of Cr(VI) ion and biomonitoring of cancer cells, Mater. Sci. Eng., C, 2018, 93, 429–436 CrossRef CAS PubMed
. - J.-B. Qu, S.-H. Li, Y.-L. Xu, Y. Liu and J.-G. Liu, Inherently fluorescentpolystyrene microspheres as a fluorescent probe for highly sensitive determination of chromium(VI) and mercury(II) ions, Sens. Actuators, B, 2018, 272, 127–134 CrossRef CAS
. - A. Tall, F. Antônio Cunha, B. Kaboré, E. S. d'Angeles do, C. Barbosa, U. Rocha, T. O. Sales, M. O. Fonseca Goulart, I. Tapsoba and S. J. Carinhanha Caldas, Green emitting N, P-doped carbon dots as efficient fluorescentnanoprobes for determination of Cr(VI) in water and soil samples, Microchem. J., 2021, 166, 106219 CrossRef CAS
. - M. Cao, Y. Li, Y. Zhao, C. Shen, H. Zhang and Y. Huang, A novel method for the preparation of solvent-free, microwave-assisted and nitrogen-doped carbon dots as fluorescent probes for chromium(VI) detection and bioimaging, RSC Adv., 2019, 9(15), 8230–8238 RSC
. - Y. Deng, W. Niu, Z. Wang and L. Feng, Synthesis, photoelectric properties and application of a polymer fluorescent probe with quinoline and benzene groups, Sens. Actuators, B, 2017, 238, 613–618 CrossRef CAS
. - A. Sil, S. N. Islam and S. K. Patra, Terpyridyl appended poly(metaphenylene-alt-fluorene) π-conjugated fluorescent polymers: Highly selective and sensitive turn off probes for the detection of Cu2+, Sens. Actuators, B, 2018, 254, 618–628 CrossRef CAS
. - Y. Shan, W. Yao, Z. Liang, L. Zhu, S. Yang and Z. Ruan, Reaction-based AIEE-active conjugated polymer as fluorescent turn on probe for mercury ions with good sensing performance, Dyes Pigm., 2018, 156, 1–7 CrossRef CAS
. - N. Choudhury, B. Ruidas, B. Saha, K. Srikanth, C. Das Mukhopadhyay and P. De, Multifunctional tryptophan-based fluorescent polymeric probes for sensing, bioimaging and removal of Cu2+ and Hg2+ ions, Polym. Chem., 2020, 11(12), 2015–2026 RSC
. - S. He, L. Marin and X. Cheng, Novel water soluble polymeric sensors for the sensitive and selective recognition of Fe3+/Fe2+ in aqueous media, Eur. Polym. J., 2022, 162, 110891 CrossRef CAS
. - N. Choudhury, B. Saha, B. Ruidas and P. De, Dual-Action Polymeric Probe: Turn-On Sensing and Removal of Hg2+; Chemosensor for HSO4−, ACS Appl. Polym. Mater., 2019, 1(3), 461–471 CrossRef CAS
. - D. Giri, A. Bankura and S. K. Patra, Poly(benzodithieno-imidazole-alt-carbazole) basedπ-conjugated copolymers: Highly selective and sensitive turn-off fluorescent probes for Hg2+, Polymer, 2018, 158, 338–353 CrossRef CAS
. - L. Feng, Y. Deng, X. Wang and M. Liu, Polymer fluorescent probe for Hg(II) with thiophene, benzothiazole and quinoline groups, Sens. Actuators, B, 2017, 245, 441–447 CrossRef CAS
. - S. W. Chen, X. Chen, Y. Li, Y. Yang, Y. Dong, J. Guo and J. Wang, Polydiacetylene-based colorimetric and fluorometric sensors for lead ion recognition, RSC Adv., 2022, 12(34), 22210–22218 RSC
. - M. Gupta, S. Sahana, V. Sharma and P. K. Bharadwaj, Benzothiazole integrated into a cryptand for ESIPT-based selective chemosensor for Zn(II) ions, Dalton Trans., 2019, 48(22), 7801–7808 RSC
. - W. Feng, G. Fu, Y. Huang, Y. Zhao, H. Yan and X. Lü, ESIPT-capable Eu3+-metallopolymer with colour-tunable emission for selective visual sensing of Zn2+ ion, J. Mater. Chem. C, 2022, 10(3), 1090–1096 RSC
. - A. I. Costa, P. D. Barata, C. B. Fialho and J. V. Prata, Highly sensitive and selective fluorescent probes for Cu(II) detection based on calix[4]arene-oxacyclophane architectures, Molecules, 2020, 25(10), 2456 CrossRef CAS PubMed
. - H. Diao, L. Guo, W. Liu and L. Feng, A novel polymer probe for Zn(II) detection with ratiometric fluorescence signal, Spectrochim. Acta, Part A, 2018, 196, 274–280 CrossRef CAS PubMed
. - X. Wang, C. Zhang, Y. Zhang, J. Sun, L. Cao, J. Ji and F. Feng, Facile crosslinking of polythiophenes by polyethylenimine via ester aminolysis for selective Cu(II) detection in water, Biosens. Bioelectron., 2018, 109, 255–262 CrossRef CAS PubMed
. - D. Luo, S. G. Liu, N. B. Li and H. Q. Luo, Water-soluble polymer dots formed from polyethylenimine and glutathione as a fluorescent probe for mercury(II), Mikrochim. Acta, 2018, 185(6), 284 CrossRef PubMed
. - Y. Zhu, X. Gong, Z. Li, X. Zhao, Z. Liu, D. Cao and R. Guan, A simple turn-on ESIPT and PET-based fluorescent probe for detection of Al(III) in real-water sample, Spectrochim. Acta, Part A, 2019, 219, 202–205 CrossRef CAS PubMed
. - D. Jiang, M. Zheng, X. Yan, B. Huang, H. Huang, T. Gong, K. Liu and J. Liu, A “turn-on” ESIPT fluorescence probe of 2-(aminocarbonyl)phenylboronic acid for the selective detection of Cu(II), RSC Adv., 2022, 12(48), 31186–31191 RSC
. - Y. Zhang, Z. Li, Q. Sun, Z. Li, Y. Zhi, R. Nie, H. Xia, Y. Yu and X. Liu, Light-Emitting Conjugated Organic Polymer as an Efficient Fluorescent Probe for Cu2+ Ions Detection and Cell Imaging, Macromol. Rapid Commun., 2021, 42(19), 2100469 CrossRef CAS PubMed
. - A. Mahapatra, B. G. Mishra and G. Hota, Studies on electro-spun alumina nanofibers for the removal of chromium(VI) and fluoride toxic ions from an aqueous system, Ind. Eng. Chem. Res., 2013, 52(4), 1554–1561 CrossRef CAS
. - F.-M. Li, J.-M. Liu, X.-X. Wang, L.-P. Lin, W.-L. Cai, X. Lin, Y.-N. Zeng, Z.-M. Li and S.-Q. Lin, Non-aggregation based label free colorimetric sensor for the detection of Cr(VI) based on selective etching of gold nanorods, Sens. Actuators, B, 2011, 155(2), 817–822 CrossRef CAS
. - Y. Wang, X. Hu, W. Li, X. Huang, Z. Li, W. Zhang, X. Zhang, X. Zou and J. Shi, Preparation of boron nitrogen co-doped carbon quantum dots for rapid detection of Cr(VI), Spectrochim. Acta, Part A, 2020, 243, 118807 CrossRef CAS PubMed
.
|
This journal is © The Royal Society of Chemistry 2023 |
Click here to see how this site uses Cookies. View our privacy policy here.