DOI:
10.1039/D3RA03852A
(Paper)
RSC Adv., 2023,
13, 21685-21689
Metal-free C-3 selective C(sp2)–C(sp3) heteroarylation of anilines with imidazo[1,2-a]pyridine derivatives via cross-dehydrogenative coupling†
Received
8th June 2023
, Accepted 9th July 2023
First published on 19th July 2023
Abstract
A general and straightforward method for the regioselective construction of C-3 heteroaryl-containing imidazo[1,2-a]pyridines via cross-dehydrogenative coupling under transition-metal-free conditions has been reported, utilizing N,N-dimethylaniline as the methylenation source and furnishing the C(sp2)–C(sp3) functionalized products in good to excellent yields. Mechanism studies indicate that a radical pathway is responsible for this transformation.
Introduction
As classical N-heterocyclic compounds, imidazopyridine and its derivatives have always been present in organic synthesis, materials and pharmaceuticals.1 Among which, imidazo[1,2-a]pyridines are considered to be privileged scaffolds due to their pharmaceutical activities,2 like antiherpes,2a antifungal,2b,c antiinflammatory,2d and antiviral.2i All these unparalleled characteristics make the development of efficient synthetic methods for the characteristic compounds receive more attention than ever before. Thus, sustained efforts toward functionalized imidazo[1,2-a]pyridine derivatives have been developed, particularly on the C-3 position.3
Besides, the introduction of anilines has also obtained significant attention due to their specific bioactives in pharmaceuticals, natural products and advanced materials.4 Therefore, substantial investigations have been committed to the exploring of novel methods for aniline-containing compounds via direct C–H functionalization.5 Despite all the efforts, prefunctionalized substrates, transition metal catalysts and organic ligands are always inevitable, resulting in poor synthesis efficiency and low atom economy.
Taking all the above into account, cross-dehydrogenative coupling,6 which with hydrogen as the main by-product, have successfully attracted the attention of chemists and been applied into the construction of C–C,7 C–X (X = N, O, S),8–10 and N–X (X = N, O, S) bonds.11–13 With the help of the efficient strategy, in 2021, the direct C–C heteroarylation on C-3 position of quinoxalinones with anilines was reported by Li's group (Scheme 1b).7c Same year, Lei and Zhang's group declared a novel direct C–H amination reaction for the preparation of triarylamine derivatives (Scheme 1c).7d The next year, Li, Wang and Cheng's group achieved a switchable progress for the C3-aminomethylation and C3-arylmethylation of imidazo[1,2-a]pyridines using N-methylanilines under electro-chemical conditions.7e
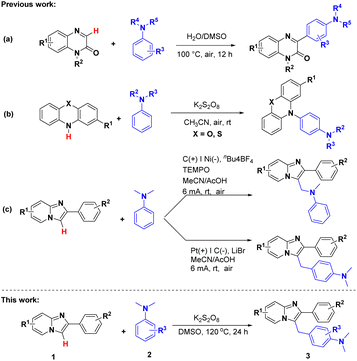 |
| Scheme 1 Direct C–C cross-dehydrogenative coupling of anilines. | |
Herein, we achieve a metal-free method for the synthesis of C-3 heteroarylation-containing imidazo[1,2-a]pyridine via direct cross-dehydrogenative coupling, affording the desired products in good to excellent yields under mild conditions.
Results and discussion
Initially, 2-phenylimidazo[1,2-a]pyridine 1a and N,N-dimethyl-aniline 2a were selected as the model substrates. Delightfully, 27% of the cross-coupling product 3a was generated in presence of 2.0 equivalents K2S2O8 in DCE (Table 1, entry 1). Encouraged by the results, solvents including DMSO, THF, CH3CN, H2O and 1,4-dioxane were then investigated, and DMSO exhibited the optimal activity, giving the best yields at 65% (entries 2–6). Follow researches on the screening of oxidants manifested that K2S2O8 was superior to other oxidants including (NH4)2S2O8, Na2S2O8, Oxone, PhI(OAc)2, DTBP, TBHP and m-CPBA (entries 7–13). At the same time, shortening the reaction time to 18 hours, the yield was descended to 58% (entry 14). Similarly, when the reaction was implemented under an oxygen atmosphere and prolonged the reaction time to 36 h, the yield was reduced to 45% (entry 15). Finally, with the amount of K2S2O8 declined to 1.5 equivalent or been removed, the yields dropped to 54% and 37%, respectively (entry 2, 15).
Table 1 Optimization of reaction conditionsa,b

|
Entry |
Solvent |
Oxidant |
Yieldb [%] |
Reaction conditions: 1a (0.3 mmol), 2a (2.0 eq.), oxidant (2.0 eq.), solvent (3.0 mL), stirred at 120 °C, under air, 24 h. Isolated yields. K2S2O8 (1.5 eq.). K2S2O8 (2.0 eq.), 18 h. Under O2, 36 h. |
1 |
DCE |
K2S2O8 |
27 |
2 |
DMSO |
K2S2O8 |
65 (54)c |
3 |
THF |
K2S2O8 |
Trace |
4 |
CH3CN |
K2S2O8 |
13 |
5 |
H2O |
K2S2O8 |
nr |
6 |
1,4-Dioxane |
K2S2O8 |
20 |
7 |
DMSO |
(NH4)2S2O8 |
54 |
8 |
DMSO |
Na2S2O8 |
48 |
9 |
DMSO |
Oxone |
52 |
10 |
DMSO |
PhI(OAc)2 |
Trace |
11 |
DMSO |
DTBP |
50 |
12 |
DMSO |
TBHP |
43 |
13 |
DMSO |
m-CPBA |
40 |
14 |
DMSO |
K2S2O8 |
58d |
15 |
DMSO |
— |
37 (45)e |
With the aforementioned optimized reaction protocol in hand, the generality and limitations of this transformation were then inspected (Table 2). Substrates containing electron-donating or electron-withdrawing groups (–Me, –OMe and –OBn) on both aryl ring and imidazo[1,2-a]pyridine ring (–F, –Cl and –Br) could take place this transformation smoothly and furnish the desired products from 38% to 68% yields (3a–3n). Ulteriorly, the electron-donating ones showed superior to the electron-withdrawing ones. Furthermore, with the electron-absorbent getting strong, the reaction would be less favorable. And no product could be obtained when 2-phenyl-6-(trifluoromethyl)imidazo[1,2-a]pyridine was employed as beginning material (3l). To our satisfaction, this transformation could be smoothly implemented with series of N,N-dimethyl-4-((2-phenylbenzo[d]imi-dazo[2,1-b]thiazol-3-yl)methyl)aniline derivatives, and resulted the coupling products in 40–59% yields (3p–3s). At the same time, substrates with large hindrance substituents showed poor reactivity and gave the desired products up to 45% yields, which indicating that steric effect had an obvious influence on the reaction activity (3o, 3s). Follow investigation of N-methylaniline and N,N-diethylaniline manifested that the changes in the source of methylene have a decisive effect on the reaction (3t–3ub).
Table 2 Scope of substratesa,b
Reaction conditions: 1 (0.3 mmol), 2 (2.0 eq.), K2S2O8 (2.0 eq.), DMSO (3.0 mL), stirred at 120 °C, under air, 24 h. Isolated yields. |
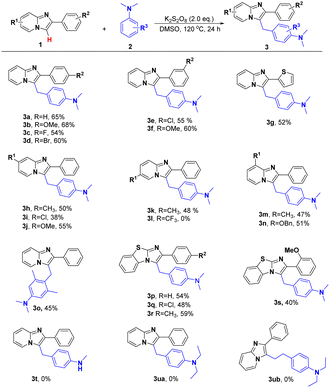 |
Next, a gram-scale reaction was conducted and the desired product could be obtained in 54% yields (Scheme 2a). Then, to gain more insights into the reaction mechanism, several control experiments were then conducted. Firstly, 4-methyl-N,N-dimethylaniline (2e) was utilized to replace 2a as the starting material (Scheme 2b) and no desired product was detected. Next, in all of these cases, the yield of 3a had a significantly decrease with the addition of 2,2,6,6-tetramethyl-piperidine-1-oxyl (TEMPO), suggesting a radical pathway may be included for this transformation (Scheme 2c).
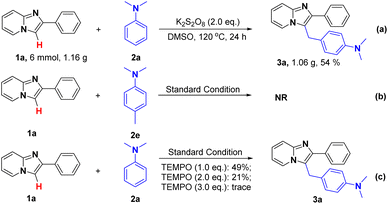 |
| Scheme 2 Gram-scale reaction and mechanistic studies. | |
Based on the aforementioned experiments and previous reports,7e,8d,e,14,15 a plausible radical pathway mechanism was proposed (Scheme 3). Initially, N,N-dimethylaniline-radical-cation (A) was generated in the presence of K2S2O8. Then, hydrogen transfer from (A) to furnish the crucial iminium species (B). Next, (B) attacked 1a at C3 position to produce intermediate (C), which underwent further oxidation to generate (D). Next, the sulfate radical anion was generated from K2S2O8 under thermolysis and absorbed a hydrogen radical from (D) to give intermediate (E). Subsequently, species (F) was obtained from (E) via the nucleophilically attacked of 2a and deprotonated to provide (G). Finally, the target product 3a was furnished by eliminating N-methylaniline under the addition of hydrogen proton.
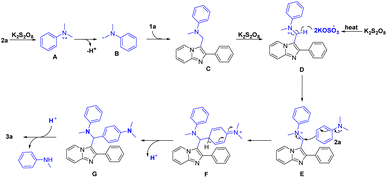 |
| Scheme 3 Plausible mechanistic pathway. | |
Conclusions
In summary, we have developed a practical and metal-free method for the preparation of C-3 heteroarylation of anilines with imidazo[1,2-a]pyridine derivatives via cross-dehydrogenative coupling under mild conditions, exhibiting excellent functional group tolerance and giving the desired products in good to excellent yields with highly atom economic. Herein, N,N-dimethylaniline was utilized as the methylenation source, which further enriched the content of methylation reaction. Control experiments revealed that a radical pathway was included in this transformation, and the gram-scale reaction showed that the ideal method possessed further application value.
Experimental section
General information
All the chemicals were obtained commercially and used without any prior purification. 1H NMR, 13C NMR and 19F NMR spectra were recorded on a BrukerAvanceII 400 spectrometer. All products were isolated by short chromatography on a silica gel (200–300 mesh) column using petroleum ether (60–90 °C) and ethyl acetate. Unless otherwise noted. All compounds were characterized by 1H NMR, 13C NMR, 19F NMR and HRGC-HRMS, which are consistent with those reported in the literature.
General procedure for the synthesis of 1a
A dried round-bottom flask was charged with 2-aminopyridine (3.0 mmol) and 2-bromoacetophenone (3.0 mmol) placed in a glass tube under neat conditions were added methanol (3.0 mL), the mixture was sonicated at 30 °C for 1 hour and then dried under vacuum. The solution of PE/AcOEt was added, the mixture was filtered under reduced pressure, and the residue was washed with PE and gave 1a as white solid.
General procedure for synthesis of 3a
A mixture of the 1a (0.3 mmol), 2a (2.0 eq.), K2S2O8 (2.0 eq.) in DMSO (3.0 mL) was placed in a Schlenk tube and stirred at 120 °C under air atmosphere for 24 h. Then the mixture was then allowed to reach room temperature and poured into water (5.0 mL). The mixture was extracted with ethyl acetate (5.0 mL × 3) and the combined organic layer was washed with brine (10.0 mL), dried with Na2SO4, and the solvent was removed under reduced pressure. The product 3a was purified by flash column chromatography using PE/AcOEt as an eluent.
Conflicts of interest
There are no conflicts to declare.
Acknowledgements
This work was supported by Natural Science Foundation of Shandong Province (ZR2022QB125).
Notes and references
-
(a) C. M. Marson, Chem. Soc. Rev., 2011, 40, 5514–5533 RSC;
(b) C. Queffélec, M. Petit, P. Janvier, D. A. Knight and B. Bujoli, Chem. Rev., 2012, 112, 3777–3807 CrossRef PubMed;
(c) R. Lefin, M. M. van der Walt, P. J. Milne and G. Terre'Blanche, Bioorg. Med. Chem. Lett., 2017, 27, 3963–3967 CrossRef CAS PubMed;
(d) S. C. Goodacre, L. J. Street, D. J. Hallett, J. M. Crawforth, S. Kelly, A. P. Owens, W. P. Blackaby, R. T. Lewis, J. Stanley, A. J. Smith, P. Ferris, B. Sohal, S. M. Cook, A. Pike, N. Brown, K. A. Wafford, G. Marshall, J. L. Castro and J. R. Atack, J. Med. Chem., 2006, 49, 35–38 CrossRef CAS PubMed;
(e) S. Kang, R. Y. Kim, M. J. Seo, S. Lee, Y. M. Kim, M. Seo, J. J. Seo, Y. Ko, I. Choi, J. Jang, J. Nam, S. Park, H. Kang, H. J. Kim, J. Kim, S. Ahn, K. Pethe, K. Nam, Z. No and J. Kim, J. Med. Chem., 2014, 57, 5293–5305 CrossRef CAS PubMed.
-
(a) K. S. Gudmundsson and B. A. Johns, Bioorg. Med. Chem. Lett., 2007, 17, 2735–2739 CrossRef CAS PubMed;
(b) Y. Rivall, G. Grassyl, A. Taudou and R. Ecalle, Eur. J. Med. Chem., 1991, 26, 13–18 CrossRef;
(c) M. H. Fisher and A. Lusi, J. Med. Chem., 1972, 15, 982–985 CrossRef CAS PubMed;
(d) K. C. Rupert, J. R. Henry, J. H. Dodd, S. A. Wadsworth, D. E. Cavender, G. C. Olini, B. Fahmy and J. J. Siekierka, Bioorg. Med. Chem. Lett., 2003, 13, 347–350 CrossRef CAS PubMed;
(e) J. J. Kaminski and A. M. Doweyko, J. Med. Chem., 1997, 40, 427–436 CrossRef CAS PubMed;
(f) E. Badawey and T. Kappe, Eur. J. Med. Chem., 1995, 30, 327–332 CrossRef CAS;
(g) Y. Rival, G. Grassy and G. Michel, Chem. Pharm. Bull., 1992, 40, 1170–1176 CrossRef CAS PubMed;
(h) M. Hranjec, M. Kralj, I. Piantanida, M. Sedić, L. Suman, K. Pavelić and G. Karminski-Zamola, J. Med. Chem., 2007, 50, 5696–5711 CrossRef CAS PubMed;
(i) S. K. Kotovskaya, Z. M. Baskakova, V. N. Charushin, O. N. Chupakhin, E. F. Belanov, N. I. Bormotov, S. M. Balakhnin and O. A. Serova, Pharm. Chem. J., 2005, 39, 574–578 CrossRef CAS.
-
(a) Z. Y. Gan, X. L. Zhu, Q. L. Yan, X. Y. Song and D. S. Yang, Chin. Chem. Lett., 2021, 32, 1705–1708 CrossRef CAS;
(b) Y. S. Zhu, Y. T. Xue, W. N. Liu, X. J. Zhu, X. Q. Hao and M. P. Song, J. Org. Chem., 2020, 85, 9106–9116 CrossRef CAS PubMed;
(c) H. M. Su, L. Y. Wang, H. H. Rao and H. Xu, Org. Lett., 2017, 19, 2226–2229 CrossRef CAS PubMed;
(d) J. R. Zhang, L. Z. Zhan, L. Wei, Y. Y. Ning, X. L. Zhong, J. X. Lai, L. Xu and R. Y. Tang, Adv. Synth. Catal., 2018, 360, 533–543 CrossRef CAS;
(e) H. Chen, H. Yi, Z. L. Tang, C. L. Bian, H. Zhang and A. W. Lei, Adv. Synth. Catal., 2018, 360, 3220–3227 CrossRef CAS;
(f) N. Li, J. K. Bai, X. L. Zheng and H. H. Rao, J. Org. Chem., 2019, 84, 6928–6939 CrossRef CAS;
(g) M. S. Franco, S. Saba, J. Rafique and A. L. Braga, Angew. Chem., Int. Ed., 2021, 60, 18454–18460 CrossRef CAS;
(h) S. Mondal, S. Samanta, S. Santra, A. K. Bagdi and A. N. Hajraa, Adv. Synth. Catal., 2016, 358, 3633–3641 CrossRef CAS;
(i) R. Kumar, D. Rawat and S. Adimurthy, Eur. J. Org. Chem., 2020, 23, 3499–3507 CrossRef;
(j) Y. Y. Gao, Y. Wang, J. Zhou, H. B. Meia and J. L. Han, Green Chem., 2018, 20, 583–587 RSC;
(k) S. C. Wang, S. Y. Zhang, M. C. Liu, J. W. Zang, G. B. Jiang and F. H. Ji, Org. Chem. Front., 2020, 7, 697–701 RSC.
-
(a) N. K. Boaen and M. A. Hillmyer, Chem. Soc. Rev., 2005, 4, 267–275 RSC;
(b) R. Hili and A. K. Yudin, Nat. Chem. Biol., 2006, 2, 284–287 CrossRef CAS;
(c) X. Chen, F. Xiao and W.-M. He, Org. Chem. Front., 2021, 8, 5206–5228 RSC.
-
(a) K. Sun, Y. Li, T. Xiong, J. Zhang and Q. Zhang, J. Am. Chem. Soc., 2011, 133, 1694–1697 CrossRef CAS PubMed;
(b) J. Xu, C. F. Liang, J. B. Shen, Q. Chen, W. M. Li and P. F. Zhang, Green Chem., 2023, 25, 1975–1981 RSC;
(c) A. M. Martínez, N. Rodríguez, R. G. Arrayás and J. C. Carretero, Chem. Commun., 2014, 50, 2801–2803 RSC;
(d) H.-L. Li, M. Kanai and Y. Kuninobu, Org. Lett., 2017, 19, 5944–5947 CrossRef CAS PubMed;
(e) C. Shen, M. Yang, J. Xu, C. Chen, K. Zheng, J. B. Shen and P. F. Zhang, RSC Adv., 2017, 7, 49436–49439 RSC;
(f) D. C. Simkó, P. Elekes, V. Pázmándi and Z. Novák, Org. Lett., 2018, 20, 676–679 CrossRef PubMed;
(g) J. Xu, K. Cheng, C. Shen, R. R. Bai, Y. Y. Xie and P. F. Zhang, ChemCatChem, 2018, 10, 965–970 CrossRef CAS.
-
(a) W. B. Shang, S. Y. Li, Y. Z. Ding, Z. Q. Pan, X. G. Tong and C. F. Xia, Org. Chem. Front., 2017, 4, 1789–1793 RSC;
(b) H. M. Wang, X. L. Gao, Z. C. Lv, T. Abdelilah and A. W. Lei, Chem. Rev., 2019, 119, 6769–6787 CrossRef CAS PubMed;
(c) W. Zhang, P. Wang, X. Zhang, R. Wang, T. Wang, Z. C. Liu and Z. T. Zhang, Chin. J. Chem., 2021, 39, 2213–2219 CrossRef CAS;
(d) A. K. Bagdi, M. Rahman, D. Bhattacherjee, G. V. Zyryanov, S. Ghosh, O. N. Chupakhin and A. Hajra, Green Chem., 2020, 22, 6632–6681 RSC;
(e) C. J. Li, Acc. Chem. Res., 2009, 42, 335–344 CrossRef CAS PubMed;
(f) C. Liu, J. W. Yuan, M. Gao, S. Tang, W. Li, R. Y. Shi and A. W. Lei, Chem. Rev., 2015, 115, 12138–12204 CrossRef CAS PubMed;
(g) J.-Y. Chen, C.-T. Zhong, Q.-W. Gui, Y.-M. Zhou, Y.-Y. Fang, K.-J. Liu, Y.-W. Lin, Z. Cao and W.-M. He, Chin. Chem. Lett., 2021, 32, 475–479 CrossRef CAS.
- Selected examples for C–C bond formation, see:
(a) L. Huanga, J. Xua, L. He, C. F. Liang, Y. N. Ouyang, Y. P. Yu, W. M. Li and P. F. Zhang, Chin. Chem. Lett., 2021, 32, 3627–3631 CrossRef;
(b) Z. Yong, W. B. Wen, W. W. Xin, D. L. Ling, N. L. Jun, L. H. Qing and F. X. Lin, RSC Adv., 2017, 7, 1229–1232 RSC;
(c) J. Xu, L. Huang, L. He, C. F. Liang, Y. N. Ouyang, J. B. Shen, M. Jiang and W. M. Li, Green Chem., 2021, 23, 6632–6638 RSC;
(d) H. Zhang, S. C. Wang, X. Y. Wang, P. J. Wang, H. Yi, H. Zhang and A. W. Lei, Green Chem., 2022, 24, 147–151 RSC;
(e) S. F. Li, T. M. Wang, X. C. Li, L. J. Fang, H. B. Zhai and B. Cheng, Green Chem., 2022, 24, 9482–9488 RSC.
- Selected examples for C–N bond formation, see:
(a) D. Sar, R. Bag, A. Yashmeen, S. S. Bag and T. Punniyamurthy, Org. Lett., 2015, 17, 5308–5311 CrossRef CAS PubMed;
(b) G. Naresh and T. Narender, RSC Adv., 2014, 4, 11862–11866 RSC;
(c) N. S. Thirukovela, R. Balaboina, R. Vadde and C. S. Vasam, Tetrahedron Lett., 2018, 59, 3749–3752 CrossRef CAS;
(d) K. Wang, T. T. Wei, Y. J. Zhang, J. H. Hou, R. R. Bai and Y. Y. Xie, Org. Biomol. Chem., 2021, 19, 1787–1794 RSC;
(e) J. Xu, K. Du, J. B. Shen, C. Shen, K. J. Chai and P. F. Zhang, ChemCatChem, 2018, 10, 3675–3679 CrossRef CAS;
(f) J. Xu, H. Y. Yang, L. He, L. Huang, J. B. Shen, W. M. Li and P. F. Zhang, Org. Lett., 2021, 23, 195–201 CrossRef CAS PubMed.
- Selected examples for C–O bond formation, see:
(a) Z. C. Guo, X. P. Jiang, C. Jin, J. D. Zhou, B. Sun and W. K. Su, Synlett, 2017, 28, 1321–1326 CrossRef CAS;
(b) X. H. Song, X. Luo, J. F. Sheng, J. H. Li, Z. F. Zhu, Z. B. Du, H. Miao, M. Yan, M. K. Li and Y. Zou, RSC Adv., 2019, 9, 17391–17398 RSC;
(c) X. H. Xu, J. Sun, Y. Y. Lin, J. Y. Cheng, P. P. Li, Y. Y. Yan, Q. Shuai and Y. Y. Xie, Org. Biomol. Chem., 2017, 15, 9875–9879 RSC;
(d) J. Xu, H. Y. Yang, H. Cai, H. Y. Bao, W. M. Li and P. F. Zhang, Org. Lett., 2019, 21, 4698–4702 CrossRef CAS PubMed.
- Selected examples for C–S bond formation, see:
(a) X. Huang, Y. Q. Chen, S. Zhen, L. J. Song, M. Q. Gao, P. K. Zhang, H. Li, B. X. Yuan and G. Y. Yang, J. Org. Chem., 2018, 83, 7331–7340 CrossRef CAS PubMed;
(b) P. Wang, S. Tang and A. W. Lei, Green Chem., 2017, 19, 2092–2095 RSC;
(c) K. Wang, J. H. Hou, C. J. Zhang, K. Cheng, R. R. Bai and Y. Y. Xie, Adv. Synth. Catal., 2020, 362, 2947–2952 CrossRef CAS;
(d) S. RanJit, R. Lee, D. Heryadi, C. Shen, J. E. Wu, P. F. Zhang, K. W. Huang and X. G. Liu, J. Org. Chem., 2011, 76, 8999–9007 CrossRef CAS.
- Selected examples for N–N bond formation, see:
(a) T. Gieshoff, A. Kehl, D. Schollmeyer, K. D. Moeller and S. R. Waldvogel, J. Am. Chem. Soc., 2017, 139, 12317–12324 CrossRef CAS;
(b) C. Y. Chen, G. R. Tang, F. X. He, Z. B. Wang, H. L. Jing and R. Faessler, Org. Lett., 2016, 18, 1690–1693 CrossRef CAS PubMed;
(c) P. Y. Vemuri and F. W. Patureau, Org. Lett., 2021, 23, 3902–3907 CrossRef CAS.
- Selected examples for N–O bond formation, see:
(a) M. R. Kuram, W. G. Kim, K. Myung and S. Y. Hong, Eur. J. Org. Chem., 2016, 438–442 CrossRef CAS;
(b) R. Clarkson, R. I. Dowell and P. J. Taylor, Tetrahedron Lett., 1982, 23, 485–488 CrossRef CAS.
- Selected examples for N–S bond formation, see:
(a) F. Adhami, M. Safavi, M. Ehsani, S. K. Ardestani, F. Emmerling and F. Simyari, Dalton Trans., 2014, 43, 7945–7957 RSC;
(b) H. Xie, J. Cai, Z. Wang, H. Huang and G. J. Deng, Org. Lett., 2016, 18, 2196–2199 CrossRef CAS;
(c) L. M. T. Frija, A. J. L. Pombeiro and M. N. Kopylovich, Eur. J. Org. Chem., 2017, 2670–2682 CrossRef CAS;
(d) X.-T. Cao, Z.-L. Zheng, J. Liu, Y.-H. Hu, H.-Y. Yu, S.-S. Cai and G.-N. Wang, Adv. Synth. Catal., 2022, 364, 689–694 CrossRef CAS.
-
(a) H. B. Zhao and D. Leonori, Angew. Chem., Int. Ed., 2021, 60, 7669–7674 CrossRef CAS PubMed;
(b) K. Liu, S. Tang, T. Wu, S. C. Wang, M. Z. Zou, H. J. Cong and A. W. Lei, Nat. Commun., 2019, 10, 639 CrossRef PubMed;
(c) G. Kibriya, A. K. Bagdi and A. Hajra, J. Org. Chem., 2018, 83, 10619–10626 CrossRef CAS;
(d) Y. Ji, X. Zhang, Y. Wu, Z. L. Dang, W. W. Han, S. C. Wang, S. B. Dong and Q. Z. Zhang, Tetrahedron Lett., 2022, 110, 154175 CrossRef CAS;
(e) J. X. Zhai, B. W. Zhou, H. H. Wu, S. Q. Jia, M. G. Chu, S. T. Han, W. Xia, M. Y. He and B. X. Han, ChemSusChem, 2022, 15, e202201119 CrossRef CAS PubMed;
(f) C. Y. H. Gu, S. S. Wang, Q. R. Zhang and J. Xie, Chem. Commun., 2022, 58, 5873–5876 RSC;
(g) L. Y. Dian, D. Zhang-Negrerie and Y. F. Du, Adv. Synth. Catal., 2017, 359, 3090–3094 CrossRef CAS;
(h) G. L. Wu, Y. Z. Li, X. M. Yu, Y. Gao and H. J. Chen, Adv. Synth. Catal., 2017, 359, 687–692 CrossRef CAS.
-
(a) Y. M. Li, X. H. Wei, X. A. Li and S. D. Yang, Chem. Commun., 2013, 49, 11701–11703 RSC;
(b) M. Yuan, L. Chen, J. Wang, S. Chen, K. Wang, Y. Xue, G. Yao, Z. Luo and Y. Zhang, Org. Lett., 2015, 17, 346–349 CrossRef CAS PubMed;
(c) X. Li and Z.-J. Shi, Org. Chem. Front., 2016, 3, 1326–1330 RSC;
(d) X. Y. Yang, X. Guo, X. L. Yuan and B. H. Chen, Org. Chem. Front., 2022, 9, 4034–4040 RSC;
(e) Y. X. Liu, Y. G. Yan, D. Xue, Z. F. Wang, J. L. Xiao and C. Wang, ChemCatChem, 2020, 12, 2221–2225 CrossRef CAS.
|
This journal is © The Royal Society of Chemistry 2023 |
Click here to see how this site uses Cookies. View our privacy policy here.