DOI:
10.1039/D3RA04127A
(Paper)
RSC Adv., 2023,
13, 29668-29674
Novel decomposition of polycarbonate and effect for marine ecosystem†
Received
19th June 2023
, Accepted 28th September 2023
First published on 12th October 2023
Abstract
Analysis of pollution of the ocean plastics is presently being extensively carried out but special attention should be direct to matters. It is widely believed that plastic dose not decompose in the ocean. Certain contaminants, bisphenol-A (BPA) that serves the material for polycarbonate (PC) and epoxy resin (EPX) both of which may possibly be elute or degrade from commercial products, have often been detected in rivers, lakes and oceans. To clarify in detail the extend of this impact of this situation, purified PC (BPA free) was decomposed at temperatures range 50–230 °C. PC was seen to start decomposing at 50 °C over a 3 day period to generated 11 μg kg−1 BPA. Based on the rate constants of BPA, the activation energy was calculated 42.0 kJ mol−1. Since this value is almost same as the EPX and polystyrene (PS) of each decomposition. Based on the PC decomposition rate and the actual BPA value in the deep sea, the 280 million metric tons (MT) BPA in the world ocean was estimated. Unlike plastics, BPA shows endocrine disrupting in fish. It should thus be considered that degraded PC and EPX pose a serious threat to the marine ecosystem, directly.
1 Introduction
Whether accidentally or intentionally, waste plastic from land sources ultimately makes its way into world oceans.1–3 Since 1972, lumps of plastic breakage into micro/nano-pieces have been clearly shown a serious and large source of ocean pollution.4–6 Drifting plastics were reported accidental entanglements for marine animals, and caused the miss ingestion such as macro to micro/nano-particles for marine biota of whale to plankton has been reported.7–10
Since the late 1980s, BPA contaminations have been detected in rivers, lakes and oceans.11–14 BPA [2,2-bis(4-hydroxyphenyl)propane] is material for PC and EPX, commercial production started in 1957 USA. In 2020, the estimated production of PC in USA was approximately one million metric tons (MT).15 PC has a wide range of application such as heat-resistant, light weight with high durability, transparent, impact resistant and shows flame retardancy. All these qualities are well proportioned. The reasons why PC is developing and is finding new innovative uses after another so that its production is yearly 4% increasing.16 The authors investigated styrene oligomers (SOs)‡ contaminations in sea water and sand more than 25 countries in the Northern Hemisphere costal area and surveyed at open ocean water surface to 5000 m depth in Northwest Pacific Ocean.17–20 SOs and BPA were detected at all survey sites. BPA along the Pacific coast was found to exceed SOs and shown some hot spots.11 The source and elution pathways of BPA in world oceans have yet to clearly elucidated.21 Numerous studies have been conducted to assess the chemical stability of plastics under conditions of ageing and exposure to weather.22–25 However, to date, no kinetic research on PC decomposition has been conducted at the lower temperature range in the natural environment.26–30
Using purified PC, novel decomposition at low temperature 50–230 °C, was thus examined with polyethylene glycol (PEG) as a heating medium. Based on generated BPA from 50 to 200 °C, the rate constants of PC were determined at each temperature range. The kinetic parameters of the PC decomposition were determined and the activation energy of the conversion of PC into BPA was given as 42.0 kJ mol−1. Since this value is almost same as the EPX (42.0 kJ mol−1)29 and PS (47.0 kJ mol−1).17 Based on kinetic data,17,27 one MT of PC was found to decompose at a rate of 0.3 g per year at 30 °C. Simulation27 indicated that the total amount of degraded PC in the ocean has been as much as 450 MT during the period, 1950 to 2050. Drifting PC not only breaks up into micro/nano sized particles, but subsequently degrades into BPA. It should thus be considered that degraded PC and EPX in the oceans pose a direct serious threat to the marine ecosystem.
2 Experimental
2.1 Materials and methods
2.1.1 Reagent. Polyethylene glycol (PEG1540, average molecular weight 1350 to 1650, Wako Pure Chemical Co.) was used. Dichloromethane (DCM) and diethyl ether (DE) for dissolving PC or reprecipitation and tetrahydrofuran (THF) as the GPC eluent, all these were of reagent grades and manufactured by Wako Pure Chemical Co. N,o-Bis(trimethylsilyl)trifluoroacetamide (BSTFA) were used of Supelco Co. Ltd. The BPA standard for calibration is used of reagent grade by Aldrich. The internal standard, phenanthrene (PH) and surrogate, biphenyl (BP) was used after being purified using a reagent grade of Kanto Chemical Co., Inc. following sublimation treatment.
2.1.2 Preparation of reaction material. PC nurdle (virgin pellet) and products contained BPA which caused unreacted BPA or generated from heat treatment of production. In this study, PC nurdle pellets (Panlite/Teijinkasei Co. Ltd Tokyo Japan) from a supplier contained 200 mg kg−1 of BPA and some additives.31,32 10 g nurdle PC solved in 500 mL DCM and add 0.5 mL, 1 mmol sodium hydroxide with 500 mL water. The solution was shaken for 20 min by Yamato shaker SA-31 for ten times. The DCM layer is recovered and add 10 g sodium sulfate (anhydrous) overnight. After filtrations, the DCM solution is poured into 2 L DE and purified PC (BPA-free) is precipitated. Details were shown in Fig. 1. This BPA-free PC (Mn: 28
600, Fig. 2) was used after 10 days drying in vacuum (3 mm torr) at 25 °C.
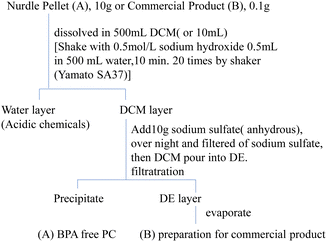 |
| Fig. 1 Preparation for reaction material and commercial samples. | |
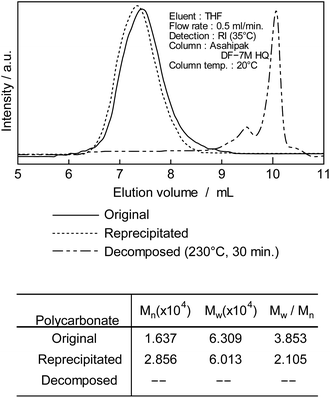 |
| Fig. 2 GPC chromatogram of original, purified and decomposed PC (230 °C, 30 min). | |
2.2 Decomposition method
The silicone oil bath was placed on stirrer bearing with a heat regulator. The thermal decomposition of PC was carried out in 4.9 g of PEG in a 30 ml round-bottomed flask bearing with Y-tube and stirrer chip on the oil bath. The Y-tube was attached with a thermocouple, an introduction tube of N2 gas and reflux condenser, respectively. N2 gas of which was adjusted 50 ml min−1 by the regulator from a N2 cylinder. When the internal temperature reached the fixed temperature, 0.100 g of purified PC was added to the medium with mixing by stirring at 500 rpm. The reaction temperature was adjusted and retained ±1 °C of the fixed temperature by thermocouple and digital thermometer (CUSTOM CT-1310) inserted in the reaction solution directly. The detail of the method was shown previously.11,17,29,30
2.3 Preparation for analysis of reaction mixture
After reaction at the fixed temperature for the fixed time, the reaction mixture dissolved in 10 ml DCM and recovered was transferred to a 300 mL separatory funnel was washed with 50 ml water to remove the PEG. 2 g anhydrate sodium sulfate was added into the DCM layer overnight. After filtrate, the DCM solution was poured into 10 mL DE to precipitate PC/polymer part. The DCM/DE solution was filtrate and drying using a rotary evaporator, evaporated under 30 °C. As a residue an internal standard (PH) was added to 0.5 mg kg−1 used as a sample for GC/MS measurement. The GC/MS sample was prepared by removing the polymer by the method shown in Fig. 1A and B.
2.4 GC-MS analysis
1 μL of samples was separately injected into a 6890 gas chromatograph (Agilent Technologies, Avondale, PA, USA) coupled to a Jeol JMS-AII electron impact ionization mass spectrometer. The analysis was performed in the splitless mode into a DB-5 capillary column (30 m × 0.32 mm I.D., 0.25 μm film thick, Agilent Technologies, Avondale, PA, USA). The oven temperature for the analysis was programmed from 50 °C (hold 2 min) to 290 °C at 15 °C min−1 and then where it was maintained for 5 min. Helium gas was used as a carrier gas at constant flow of 1.2 mL min−1. The MS transfer line temperature was maintained at 300 °C, and the ion source and quadrupole at 210 °C and 255 °C, respectively. Measurements were performed in SCAN (50–400 m/z, 300 ms) and SIM (selection monitoring) modes and the m/z followed are listed in Table 1. Field survey samples were predicted to have low BPA. Therefore, the sample was treated with BSTFA to increase its volatility (TMS derivatives).
Table 1 Mass spectrometry apparatus conditions
Apparatus column |
MS: Jeol LMS-AM II and GC: HP-6890 DB-1 (L: 30 m, ID: 0.32 mm, film thick: 0.25 μm) |
Monitoring ion |
TIM: all m/z, scan 300 ms, 5:10 to 21:00 min |
Column temperature |
50° (2 min hold) upto 290 °C |
SIM: m/z: 76, 135, 150, 154, 213, 228, 325, 340 |
(Program rate: 15 °C min−1 and 5 min hold at 290 °C) |
Injection system |
Split less |
Interface temperature |
255 °C |
Injection |
Manual, 1 μL |
Ion source temperature |
210 °C |
Injection temperature |
250 °C |
Ion acceleration current |
70 eV |
Purge time |
3 min |
Current |
300 μV |
Purge flow |
30.0 mL min−1 |
PM voltage |
600 V |
Total flow |
33.7 mL min−1 |
Carrier gas |
He (flow: 1.4 mL min−1) |
Save flow |
15.0 mL min−1 |
|
|
Save time |
15.0 s |
|
|
2.5 Preparation of a calibration
Preparation of a calibration curve using standard substances for determination 0.1 g 10 mL−1 of the standard stock solution was prepared and diluted to prepare solutions of 0.01, 0.05, 0.1, 1, 3, 5, 10, 20, 50 mg kg−1 10 mL−1, and 1 μL thereof was injected into GC/MS. By analyzing the mass spectrum of each standard substance obtained by GC/MS scan mode measurement (TIM) and grasping the m/z of the main fragment ion, the monitoring channel of the substance to be measured in selective ion monitor (SIM).
2.6 Apparatus
The quantitative determination of decomposition products and field survey was conducted using GC/MS. The following apparatus was used for the separation and identification of the products; nuclear magnetic resonance spectroscopy (NMR): Jeol LNM-LA500 FT NMR system (1H and 13C), gas chromatography-mass spectrometer (GC/MS), GC: HP6890 and MS: Jeol JMS-AMII quadrupole mass spectrometer, gel permeation chromatography kai Co. Ltd. Detector, Shodex RI-71, temp: 35 °C, (column: Asahipak GF-7M HQ, Asahi Chem. Ind. Co., Ltd temp: 20 °C). High-mass spectrometer: Hitachi M-2000 (double-focusing mass), Infrared spectroscopy (IR): JASCO FT-IR300E.
3 Results and discussion
3.1 Accuracy of analysis
Qualitative analysis was performed by total ion monitor (TIM) and quantification was performed by SIM in this study. The conditions of GC/MS were shown in Table 1. In SIM, choosing the monitor ion (qualitative ion Q: m/z 213 and identification ion I: m/z 228 or TMS derivatives, Q: m/z 357, I: m/z 372), the calibration curve of each target chemical was obtained using internal standard PH and the BPA standard solution. The correlation coefficient (r) was obtained based on linearity within 0.01–10 mg kg−1, R = 0.9996–0.9999. The required detection limit was determined as 5 μg kg−1, in S/N = 2. The relative standard deviation of peak area of the PH for each injection was within 2%. The eliminate error for extraction recovery of surrogate DP was found to range from exceed over 90.3–97.8%, with an overall average of 95.2%, and relative standard deviation (RSD), was always within 7.3%.
3.2 Analysis of commercial products
Brotons,33 Howe,34 and Romero35 canned foods obtained from different countries and detected BPA in all samples. But the reasons as to why BPA is present in canned food remain to be determined.21,36 Especially, canned food or nursing bottles are heat at about 100–120 °C for several minutes each time for sterilization. The commercially available PC product, (nursing bottle, food container) was cut with scissors, exactly 0.100 g was weighed by an analytical balance, dissolved in 5 ml of DCM, and BP was added as a surrogate so as to be 0.1 mg kg−1 at the time of measurement as shown. This solution was added to 10 ml of DE to precipitate and remove the PC-polymer portion. The DE solution was concentrated using a rotary evaporator until immediately before drying. Details were shown in Fig. 1B.
To this, PH as an internal standard was added so as to be 0.5 mg kg−1 at the time of measurement to make exactly 10 ml acetone solution. 1 μL of this solution was injected into a GC/MS and separated and quantified by SIM. The commercial products in Table 2 are made by the injection molding. In this method, the nurdle PC pellets are extruded by a screw to 200–250 °C after being dried with air and put into a mold. The mold is heated to 120–150 °C, where a PC is introduced and molded into the desired shape. After cooling, the mold is cooled, and the product is peeled off from the mold and collected. The mold, preheating temperature, time and additives31 for improving durability during this process are not clear as know-how from manufacturers to molding processing companies. BPA was found present in all commercial products28 to 111 mg kg−1 as shown in Table 2.
Table 2 Analytical results of commercial food containers
Sample |
1 |
2 |
3 |
4 |
5 |
6 |
7 |
8 |
9 |
10 |
11 |
12 |
13 |
Component |
Milkbottle |
Cup |
Bottle |
Container |
Coffee dripper |
Milkbottle |
USA |
Germany |
Italy |
France |
Japan |
China |
BOL |
ND |
ND |
9.20 |
ND |
ND |
17.50 |
ND |
ND |
2.30 |
ND |
ND |
12.80 |
ND |
BPA |
111.07 |
38.13 |
52.07 |
88.86 |
45.46 |
50.69 |
46.00 |
42.43 |
47.13 |
47.34 |
28.27 |
102.49 |
94.11 |
|
BOL: t-butylphenol |
(Unit; μg kg−1) |
|
BPA: bisphenol A |
Differences in BPA contain were due to these in heat treatment conditions, temperature and time for the molding and processing of each product, and the synthetic method for nurdle pellet. These BPA would thus appear to be exuded from the PC-polymer matrix temperature/time-dependently into the ocean.
3.3 Temperature effects for PC decomposition
After treating DCM/DE, purified PC was prepared as shown in Fig. 1A. Purified PC for thermal decomposition with a BPA of 5 μg kg−1 or less was prepared due to sodium hydroxide and reprecipitation. Average molecular weight (Mn) of PC was determined as 28
600 from the GPC retention time (TR: 13–17 min) and convention calibration curve as shown in Fig. 2, solid original line. The solid line is the original nurdle PC and the dashed line is the purified (BPA free) PC and double dashed line is decomposed PC at 230 °C, 30 min. The PC molecular is almost same before and after purification of PC sample as shown in Fig. 2.
Thermogravimetric (TG) and differential scanning calorimetry (DSC) analysis showed that the nurdle PC had a glass transition of 130 °C and the purified PC of 160 °C. All significant weight reductions in TG were over 350 °C, respectively.
After decomposition, there were no PC peak but low molecular chemicals were newly appeared the retention time at 10 min.
To confirm the generation of BPA from PC in the oceans, using purified PC as the reactant, a novel thermal decomposition was examined with PEG.17,29,30 BPA was extracted from reaction mixtures for liquid/liquid distribution system with water and DE to remove unreacted PC and PEG as shown in Fig. 1. The quantities of BPA were measured by SIM-GC/MS. The results are presented in Fig. 3 and Table 3. It has been said that PC does not decompose below 250 °C.28 However, this study kinetically revealed that PC is completely decomposed at 230 °C, 30 min as shown in Fig. 2 double dot line. Fig. 3 shows the effect of temperatures at fixed time (time: 60 min). Table 3 shows the rate constant and temperature in absolute temperature at each reaction, calculated based on the actually measured BPA from the extension of reaction time at each temperature on PC decomposition. In Fig. 3, the amount of BPA was small at 50 °C, it could not be expressed on the same axis. Therefore, the velocity constant under 150 °C were shown in Table 3. BPA was noted to increase with rise in temperature rectilinearly at 160 °C in Fig. 3. Main PC decomposition product was BPA and t-butylphenol. Trace amounts of phenol, cresol, t-butylphenol, t-butylhydroxy toluene, diphenylcarbonate and 5-cumyl phenol were qualified at high temperature range (160 °C or more). After 120 °C, 120 min decomposition, new signals were measured in 1H-NMR at 3.50 to 3.79 ppm and 13C-NMR at 61.620, 70.189, 70.502 ppm, respectively. These new signals were attributed to alkanes and alkenes by scission of carbonate or isopropylidene – groups.37,38
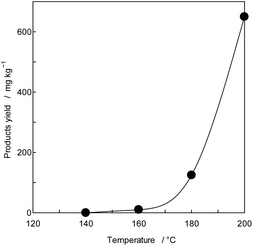 |
| Fig. 3 Effects of reaction temperature (time: 60 min). | |
Table 3 BPA rate constant at each reaction temperature
Reaction temp. |
1/T |
BPA rate constants |
°C |
K |
k |
ln k |
50 |
323.15 |
0.003095 |
0.00279 |
−5.88171 |
80 |
353.15 |
0.002832 |
0.01311 |
−4.33510 |
120 |
393.15 |
0.002544 |
0.0555 |
−2.89137 |
150 |
423.15 |
0.002363 |
0.1587 |
−1.84074 |
180 |
453.15 |
0.002207 |
0.3174 |
−1.14759 |
200 |
473.15 |
0.002113 |
0.4340 |
−0.83471 |
In the IR spectrum, new absorption of 3430 (–OH), 2874 (CH3–C–CH3), 1644 (–CO–) and 1193 (–CO–) cm−1 attributed to BPA was observed.22,24 The result indicates that PC main chain scission may occur in this condition. t-Butylphenol as a polymerization inhibitor was detected at each condition. But the formation of this chemical showed no correlation with reaction temperature.
3.4 Effect of time and calculation of activation energy
BPA increases good linearly with the extension of the reaction time at 50–200 °C as shown in Fig. 4. Assuming that the thermal decomposition of purified PC was the zero-order reaction,29,30 the rate constants of the BPA were determined at a temperature range 50–200 °C. The results were shown in Table 3.
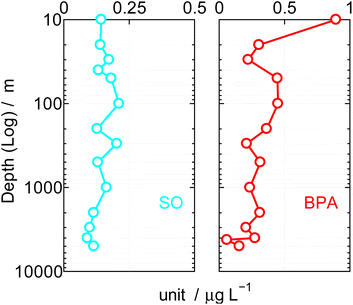 |
| Fig. 4 Vertical BPA and SO value in Northwest Pacific Ocean. | |
Based on Table 3, Arrhenius-plots were made for the reciprocal of absolute temperature (k−1) and ln
k. The logarithmic of each PC rate constant (ln
k) showed good linearity (R2 = 0.997) with the reciprocal of absolute temperature (1/T, k−1). The activation energy of PC from BPA generation was found to be 42.0 kJ mol−1, thus confirming the kinetic data. Thus, PC is clearly shown not to be stable with heat application. Since this value is almost same as the EPX (42.0 kJ mol−1)29 and PS (47.0 kJ mol−1)30 of each decomposition.
3.5 Field survey and simulation of BPA
The coastal region BPA in water and sand were affected by the latitude, longitude meteorology, ocean currents, weather,23,24 and population density. Using the Japanese Government research ship Hakuho along the 10° to 45°N latitude, 133° to 160° longitude, 5000 km north to south and 2000 km east to west, during the cruise (B: KT10-25, 31st October to 17th November 2010. C: KH12-3, 6th July to14th August 2012) in the Northwest Pacific Ocean. Distributions of SOs and BPA were surveyed from surface to bottom (5000 m) with 10 L of water at a fixed depth each (Niskin water sampler/General Oceanics, Inc.).
The results were shown in Table 4 and Fig. 4. Field survey predicted very low BPA. In addition, since BPA is highly adsorbable to the GC column, it was analyzed by TMS derivative. In Fig. 4, the depth of the sea is shown logarithmically on the vertical axis (left/m), and the SOs and BPA value detected at each depth is shown μg L−1 on the horizontal axis. The mean surface current was 0.7 knots during this cruise. SOs and BPA tend to be high in the photic zone at a depth of 200 m in Fig. 4, but at more than 2000 m where there is only minimal horizontally currents and therefore more limited water mixing, means SOs and BPA levels approximated 0.1 to 0.2 μg L−1, respectively. The SOs and BPA both would thus appear to adhere or be adsorbed by organic or inorganic materials and this flocculation to particulate matter may be a mechanism for its vertical movement.
Table 4 Details of BPA at Northwest Pacific Ocean (unit: μg L−1)
Depth (m) |
B6 |
B9 |
B13 |
C1 |
C5 |
C9 |
C12 |
C13 |
C14 |
C15 |
C16 |
C17 |
C18 |
10 |
0.414 |
0.576 |
0.219 |
0.084 |
0.039 |
1.497 |
— |
2.321 |
— |
0.192 |
1.330 |
0.100 |
0.071 |
20 |
0.442 |
0.554 |
0.200 |
0.161 |
0.038 |
0.070 |
— |
1.406 |
0.074 |
0.108 |
0.105 |
0.090 |
0.410 |
30 |
0.397 |
0.504 |
0.202 |
0.150 |
0.062 |
0.072 |
— |
— |
— |
0.321 |
— |
0.066 |
0.220 |
40 |
— |
— |
— |
— |
— |
— |
— |
— |
0.050 |
— |
— |
— |
— |
50 |
0.421 |
0.577 |
0.147 |
0.103 |
0.137 |
0.054 |
— |
1.262 |
|
1.869 |
0.115 |
0.055 |
0.165 |
75 |
— |
— |
0.170 |
— |
— |
— |
— |
— |
— |
— |
— |
— |
— |
100 |
— |
1.269 |
0.476 |
0.145 |
0.063 |
0.212 |
— |
1.590 |
0.116 |
0.249 |
0.073 |
0.526 |
0.234 |
200 |
0.408 |
0.568 |
0.164 |
0.426 |
0.051 |
0.082 |
— |
0.194 |
— |
1.668 |
0.231 |
0.171 |
0.039 |
300 |
— |
— |
— |
0.656 |
0.152 |
0.112 |
— |
— |
— |
0.158 |
— |
0.144 |
0.044 |
500 |
0.314 |
0.302 |
0.090 |
0.132 |
0.107 |
0.056 |
— |
0.396 |
0.070 |
0.956 |
1.071 |
0.204 |
0.043 |
1000 |
— |
0.152 |
0.142 |
0.316 |
0.097 |
0.156 |
— |
0.548 |
0.094 |
0.118 |
0.176 |
0.552 |
— |
2000 |
0.393 |
0.162 |
— |
0.159 |
0.059 |
0.474 |
— |
0.229 |
— |
0.613 |
0.406 |
— |
— |
3000 |
0.462 |
0.100 |
— |
0.520 |
0.044 |
0.226 |
0.074 |
0.071 |
— |
0.184 |
0.169 |
— |
— |
4000 |
0.656 |
0.168 |
— |
0.241 |
0.043 |
0.277 |
0.071 |
0.713 |
— |
0.159 |
0.147 |
— |
— |
5000 |
— |
— |
— |
0.210 |
— |
— |
0.088 |
0.167 |
— |
— |
— |
— |
— |
Average |
0.420 |
0.448 |
0.221 |
0.280 |
0.074 |
0.244 |
0.232 |
0.789 |
0.081 |
0.550 |
0.651 |
0.268 |
0.230 |
Total |
0.359 |
The amounts of waste PC/EPX inflow accumulated in ocean bodies from 1950 to 2020 have been estimated as 1.5 × 107 MT,17 assuming PC/EPX to constitute 7% of plastic global total production16 (4.8 × 108 MT), and 3% of plastics4 to flow into world ocean. Using open ocean deep sea BPA values of 0.2 μg L−1 and total water in oceans' as 1.4 × 1021 L, as with PS,17,30 it is estimated that 280 million MT of BPA are present between 1950 and 2020.
4 Conclusions
It is widely believed that plastic does not decompose in the ocean. The results indicated the typical heat-resistant plastic PC to have little stability toward heat, kinetically. In all thermoplastics of polyethylene (PE), polypropylene (PP), polyvinyl chloride (PVC) and PS production, PC and EPX production is less than 6% of world plastic. World coastal survey indicated most of the drifting plastic to be composed PE, PP, PVC and PS. There are no PC nursing bottles or food container could be found all survey site in the world. But BPA (0.2 μg L−1) deeper than 2000 m in the Northwest Pacific Ocean are twice that of SOs (0.1 μg L−1) and higher than those derived from PS. The PC/EPX decomposition rate of BPA, activation energy was found to be 42.0 kJ mol−1. With the deep-sea BPA, it is estimated that there are 280 million MT of BPA in the world ocean. Surveys in coastal areas around the world have found few washed-up PC products. BPA also has a history of being widely used for thermal paper and a stabilizer for other plastics. On the other hand, EPX had the same activation energy as PC. It was suggested that EPX contributes to the supply of BPA in the ocean because it has been used as a ship hull paint for ocean voyage. Drifting macro plastics are thus shown not only to be crushed into micro-nano fragments, but also to degrade into BPA of which PC/EPX are made. In oceans in which PC or EPX has been discarded, there is the possibility of long-term pollution by BPA, generated via the decomposition of these plastics since tropical and subtropical area beach may remain at 50 °C or more for many years. Contamination by BPA in oceans is progressive since its amount increases with time and temperature. It should thus be considered that degraded PC and EPX pose a new and serious threat to the marine ecosystem, directly.
Ethical statement
This article does not involve any human or animal subjects.
Author contributions
Koshiro Koizumi: field survey, analysis and writing – original draft. Akifumi Okabe: field survey and calculation. Hideki Kimukai: field survey, analysis and editing. Hideto Sato: field survey (voage) and analysis, Hiroyuki Taguchi: PC decomposition, rate calculation and GPC analysis. Masahiko Nishimura: field survey and analysis. Bum-Gun Kwon: field survey and analysis. Katsuhiko Saido: field survey, decomposition, GC/MS analysis, writing & supervision.
Conflicts of interest
There are no conflicts to declare.
References
- E. D. Carpenter and K. L. Smith, Plastics on the Sargasso Sea surface, Science, 1972, 175, 1240–1241 CrossRef CAS PubMed.
- E. van Sebille, C. Wilcox, L. Lebreton, N. Maximenko, B. D. Hardesty, J. A. van Franeker, M. Eriksen, D. Siegel, F. Galgani and K. L. Law, A global inventory of small floating plastic debris, Environ. Res. Lett., 2015, 10, 124006 CrossRef.
- J. Martin, A. Lusher, R. C. Thompson and A. Morley, The deposition and accumulation of microplastics in marine sediments and bottom water from the Irish continental shelf, Sci. Rep., 2017, 7, 10772 CrossRef PubMed.
- J. R. Jambeck, R. Geyer, C. Wilcox, T. R. Siegler, M. Perryman, A. Andrady, R. Narayan and K. L. Law, Plastic waste inputs from land into the ocean, Science, 2015, 347, 768–771 CrossRef CAS PubMed.
- M. Bergman, M. B. Tekman and L. Gutow, Marine litter: Sea change for plastic pollution, Nature, 2017, 544, 297 CrossRef PubMed.
- M. Moore, R. Andrews, T. Austin, J. Bailey, A. Costidis, C. George, K. Jackson, T. Pitchford, S. Landry, A. Ligon, W. McLellan, D. Morin, J. Smith, D. Rotstein, T. Rowles, C. Slay and M. Waldh, Rope trauma, sedation, disentanglement, and monitoring-tag associated lesions in a terminally entangled North Atlantic right whale (Eubalaena glacialis), Mar. Mammal Sci., 2013, 29, E98–E113 Search PubMed.
- R. Allen, D. Jarvis, S. Sayer and C. Mills, Entanglement of grey seals Halichoerus grypus at a haul out site in Cornwall, UK, Mar. Pollut. Bull., 2012, 64, 2815–2819 CrossRef CAS PubMed.
- S. Kühn, E. L. B. Rebolledo and J. A. Franeker, Deleterious effects of litter on marine life, in Marine Anthropogenic Litter, ed. M. Bergmann, L. Gutow and M. Klages, Springer Nature, UK, 2015, pp. 75–116 Search PubMed.
- S. Kühn and J. A. Franeker, Quantitative overview of marine debris ingested by marine megafauna, Mar. Pollut. Bull., 2020, 151, 110858 CrossRef PubMed.
- Y. D. Lin, P.-H. Huang, Y. W. Chen, C. W. Hsieh, Y. L. Tain, B. H. Lee, C. Y. Hou and M. K. Shih, Sources, Degradation, Ingestion and Effects of Microplastics on Humans: A Review, Toxics, 2023, 11, 747 CrossRef PubMed.
- B.-G. Kwon, S.-Y. Chung and K. Saido, Sandy beaches as hotspots of bisphenol A, Environ. Res., 2020, 191, 110175 CrossRef CAS PubMed.
- J. Zhang, X. Li, L. Zhou, L. Wang, Q. Zhou and X. Huang, Analysis of effects of a new environmental pollutant, bisphenol A, on antioxidant systems in soybean roots at different growth stage, Sci. Rep., 2016, 6, 23782 CrossRef CAS PubMed.
- F. M. Ghazali and W. L. W. Johari, The occurrence and analysis of bisphenol A (BPA) in environmental samples, a review, J. Biochem. Microb. Biotechnol., 2015, 3, 279 Search PubMed.
- D. A. Markham, D. A. McNett, J. H. Birk, G. M. Klecka and M. J. Bartels, Quantitative determination of Bisphenol-A in river water by cool on-column injection-gas chromatography-mass spectrometry, Int. J. Environ. Anal. Chem., 1998, 69, 83–98 CrossRef CAS.
- A. L. Fernandez, Bisphenol A production in United States from 1990 to 2019, Statista, US BPA production 1990-2019, 2020 Search PubMed.
- Plastics Europe, Plastics – the facts 2020: an analysis of European plastics production demand and waste data, Plastics Europe, 2020, available online: https://www.plasticseurope.org/en/resources/publications/4321-plastic-facts-2020 Search PubMed.
- H. Kimukai, K. Koizumi, H. Taguchi, A. Okabe, K. Takatama, S.-Y. Chung, B.-G. Kwon, M. Nishimura, S. Mentese and K. Saido, Predicting drifting polystyrene degradation in world oceans based on thermal decomposition, Environ. Sci.: Water Res. Technol., 2022, 2, 1976–1983 CAS.
- B. G. Kwon, K. Amamiya, H. Sato, S.-Y. Chung, Y. Kodera, S.-K. Kim, E. J. Lee and K. Saido, Monitoring of styrene oligomer as indicator of polystyrene plastic pollution in the North-West Pacific Ocean, Chemoshere, 2017, 180, 500–505 CrossRef CAS PubMed.
- K. Amamiya, K. Koizumi, K. Yamada, T. Hiaki, T. Kusui and K. Saido, Analysis of Drifting Polystyrene Degradation Surround Japan, Austin J. Environ. Toxicol., 2020, 6, 1020 Search PubMed.
- K. Amamiya, K. Saido, S.-Y. Chung, T. Hiaki, D.-S. Lee and B.-G. Kwon, Evidence of Transport of Styrene Oligomers Originated from Polystyrene Plastic to Oceans by Runoff, Sci. Total Environ., 2019, 667, 57–63 CrossRef CAS PubMed.
- J. Corrales, L. A. Kirstofco, W. B. Steele, B. S. Yates, C. S. Breed, E. S. Williams and B. W. Brooks, Global assessment of bisphenol A in the environment: Review and analysis of its occurrence and bioaccumulation, Dose-Response, 2015, 13, 1–29 CrossRef CAS PubMed.
- A. Isobe, S. Iwasaki, K. Uchida and T. Tokai, Abundance of non-conservative microplastics in the upper ocean from 1957 to 2066, Nat. Commun., 2019, 10, 417 CrossRef CAS PubMed.
- T. Kukulka, G. Proskurowski, S. Moret-ferguson, D. W. Meyer and K. L. Law, The effect of wind mixing on the vertical distribution of buoyant plastic debris, Geophys. Res. Lett., 2012, 39, L07601 CrossRef.
- J. Brandon, M. Goldstein and M. D. Ohman, Long-term aging and degradation of microplastic particles: Comparing in situ oceanic and experimental weathering patterns, Mar. Pollut. Bull., 2016, 110, 299–308 CrossRef CAS PubMed.
- W. Huang, H. Wang, W. Hu, D. Yang, S. Yu, F. Liu and X. Song, Degradation of polycarbonate to produce bisphenol A catalyzed by imidazolium-based DESs under metal-and solvent-free conditions, RSC Adv., 2021, 11, 1595 RSC.
- I. C. McNeill and A. Rincon, Thermal degradation of poly-carbonates: Reaction condition and reaction mechanisms, Polym. Degrad. Stab., 1993, 39, 13–19 CrossRef CAS.
- G. Madras, J. M. Smith and B. J. McCoy, Thermal degradation kinetics of polystyrene in solution, Polym. Degrad. Stab., 1997, 52, 349–358 CrossRef.
- A. Davis and J. H. Golden, Thermal degradation of polycarbonate, J. Chem. Sci., 1968, 45, 47 Search PubMed.
- K. Saido, H. Taguchi, Y. Kodera, T. Kuroki, J. Park and S.-Y. Chung, Low temperature Decomposition of epoxy resin, Macromol. Res., 2004, 12, 490–492 CrossRef CAS.
- H. Kimukai, Y. Kodera, K. Koizumi, M. Okada, K. Yamada, T. Hiaki and K. Saido, Low temperature decomposition of polystyrene, Appl. Sci., 2020, 10, 5100 CrossRef CAS.
- J. Murphy, Additives for plastics handbook, Elsevier, 2nd edn, 2001 Search PubMed.
- A. Gerhardt, Plastic additive bisphenol A: Toxicity in surface-and groundwater crustaceans, J. Toxicol. Risk Assess., 2019, 5, 017 CAS.
- J. A. Brotons, M. F. Olea-Serrano, M. Villalobos, V. Pedraza and N. Olea, Xenoestrogens released from lacquer coatings in food cans, Environ. Health Perspect., 1995, 103, 608–612 CrossRef CAS PubMed.
- S. R. Howe, L. Borodinsky and R. S. Lyon, Potential exposure to Bisphenol A from food-contact use of epoxy coated cans, J. Coat. Technol., 1998, 70, 69–74 CrossRef CAS.
- J. Romero, F. Ventura and M. Gomez, Characterization of paint samples used in drinking water reservoirs: Identification of endocrine disruptor compounds, J. Chromatogr. Sci., 2002, 40, 191–197 CAS.
- P. R. Tkaczyk, B. Skora and K. A. Szychowski, Toxicity of bisphenol A(BPA) and its derivatives in divers biological models with the assessment of molecular mechanisms of toxicity, Environ. Sci. Pollut. Res., 2023, 30, 75126–75149 CrossRef PubMed.
- Y. Wu, C. Wang and C. Chen, The thermal degradation mechanism and kinetic analysis of hydrogenated bisphenol-A polycarbonate, J. Polym. Res., 2020, 27, 225–240 CrossRef.
- B. N. Jang and C. A. Wilkie, The thermal degradation of bisphenol A polycarbonate in air, Thermochim. Acta, 2005, 426, 73–84 CrossRef CAS.
Footnotes |
† Electronic supplementary information (ESI) available. See DOI: https://doi.org/10.1039/d3ra04127a |
‡ SOs [styrene oligomer: SOs means the mixture with the constant ratio of styrene(monomer) 1: 2,4-diphenyl-1-butene (styrene dimer) 1: and 2,4,6-triphenyl-1-hexene (styrene trimer) 5]. |
|
This journal is © The Royal Society of Chemistry 2023 |
Click here to see how this site uses Cookies. View our privacy policy here.