DOI:
10.1039/D3RA04285E
(Review Article)
RSC Adv., 2023,
13, 21613-21628
Toxicological evaluation of bisphenol analogues: preventive measures and therapeutic interventions
Received
26th June 2023
, Accepted 12th July 2023
First published on 19th July 2023
Abstract
Bisphenol A (BPA) is a prominent endocrine-disrupting compound that shares structural similarities with estrogen. It is widely used, particularly in the production of food packaging, canned goods, and dental sealants. Of the eight bisphenol analogues, BPA is the most frequently utilized chemical in packaging food items, canned foods and dental sealants. However, chronic exposure to BPA can pose severe health risks, particularly in children. To ensure public safety, it is crucial to adopt proper precautionary measures to minimize BPA exposure. This article explores the toxic effects of bisphenols on various body systems and mechanisms, shedding light on their impact on the reproductive and endocrine system, obesity, albuminuria, and the generation of reactive oxygen species. Understanding the detrimental effects of bisphenols on these systems and mechanisms is vital for developing strategies to mitigate their harmful consequences. Furthermore, the article delves into the biotransformation processes of bisphenols, focusing on their occurrence in vertebrates, invertebrates, plants, and microorganisms. Investigating the biotransformation pathways provides valuable insights into the fate of bisphenols in various organisms and ecosystems. Lastly, the article emphasizes preventive measures to avoid bisphenol exposure and highlights the potential use of plant-based bioactive compounds for treatment strategies. By implementing effective preventive measures, such as utilizing BPA-free products and adopting safer alternatives, individuals can reduce their exposure to bisphenols. Additionally, exploring the potential of plant-based bioactive compounds as therapeutic agents offers promising avenues for addressing the adverse effects of bisphenols. The findings presented herein contribute to a better understanding of the novelty, significance, and potential implications of bisphenol research in the field, aiding in the development of safer practices and interventions to safeguard public health.
Introduction
Bisphenol analogues encompass a group of eight chemicals, including bisphenol A (BPA), bisphenol B, bisphenol P, bisphenol F, bisphenol S, bisphenol Z, bisphenol AF, and bisphenol AP.1 Table 1 provides a comprehensive list of these analogues and their respective sources. Among the various analogues, BPA stands out as a highly prevalent chemical worldwide, finding extensive use in the manufacturing of consumer products, such as food containers and epoxy resins.2 These bisphenol analogues exhibit structural and chemical similarities to BPA. Consequently, they are utilized in a wide array of industrial, commercial, and consumer applications. These include resins, coatings, adhesives, electronic components, and plasticizers. The purpose of employing these analogue compounds is to serve as alternatives to BPA due to the potential health risks associated with its usage. Comprising two phenol rings connected by a single carbon–carbon bond, bisphenol analogues possess chemical structures that can vary depending on the substituents (atoms or groups of atoms) attached to the rings. These substituents can significantly influence the properties of bisphenol analogues, including solubility, toxicity, and reactivity. The utilization of bisphenol analogues in consumer products has sparked controversy, particularly due to concerns regarding their potential to disrupt the endocrine system. Several studies have suggested the possibility of these chemicals exhibiting endocrine-disrupting effects. However, a comprehensive understanding of the potential health risks associated with bisphenol analogues necessitates further research and investigation.2 The examination of their effects and the evaluation of their safety profiles will facilitate a better understanding of the implications surrounding the usage of bisphenol analogues in various applications.
Table 1 IUPAC names, molecular formula, molecular weight and sources of contamination of bisphenol analogues
Sr. # |
Bisphenol analogues |
IUPAC name |
Formula |
Molecular weight |
Source of contamination |
1 |
Bisphenol A |
4,4-(Propane-2,2-diyl)diphenol |
C15H16O |
228.29 g mol−1 |
Food, beverages, thermal papers |
2 |
Bisphenol B |
2,2-Bis(4-hydroxyphenyl)butane |
C16H18O2 |
242.31 g mol−1 |
Water bottles, containers |
3 |
Bisphenol F |
Bis(4-hydroxyphenyl)methane |
C13H12O2 |
200.23 g mol−1 |
Environmental contamination, dental sealants |
4 |
Bisphenol S |
4,4-Sulfonyldiphenol |
C12H10O4S |
250.275 g mol−1 |
Contaminated food, water |
5 |
Bisphenol P |
4,4-(1,4-Phenylenediisopropylidene)bisphenol |
C24H26 |
228.28 g mol−1 |
Food packaging, epoxy linings of metal food |
6 |
Bisphenol AF |
4,4-(Hexafluoroisopropylidene)diphenol |
C15H10F6O2 |
228.291 g mol−1 |
Plastic bottles |
7 |
Bisphenol AP |
4,4-(1-Phenylethylidene)bisphenol |
C20H18O2 |
290.36 g mol−1 |
Beverage cans |
BPA is classified as a synthetic xenoestrogen and a derivative of diphenylmethane, characterized by the presence of two hydroxyphenyl groups in its structural formula.3 Its synthesis dates back to 1891 when the Russian chemist Alexandar P. Dianin conducted a condensation reaction between two phenol molecules and a molecule of acetone, catalyzed by hydrogen chloride.4 BPA gained significant attention in the early 1930s during the investigation of estrogen and has since become one of the most widely used chemicals worldwide.5 Exposure to BPA can occur through various routes, including oral ingestion, dermal contact, and inhalation (Fig. 1). BPA has been detected in numerous food products, such as fats, oils, cereals, milk products, and seafood, indicating the potential for dietary exposure.6 Additionally, handling thermal papers poses a risk, as BPA in these papers is present in a free and unbound state, readily released upon contact. Consequently, handling thermal paper and subsequently consuming food can lead to increased BPA exposure. Studies have found that cashiers who regularly handle thermal receipts exhibit higher levels of BPA in their urine. Furthermore, BPA exposure in humans can also arise from sources such as laminated floors, varnishes, and electronic devices.7 Understanding the various routes of BPA exposure and the diverse sources of contamination is crucial for assessing and mitigating its potential health risks. Efforts to reduce exposure should focus on adopting safer alternatives, promoting proper hygiene practices, and implementing regulations to minimize BPA presence in consumer products and the environment.
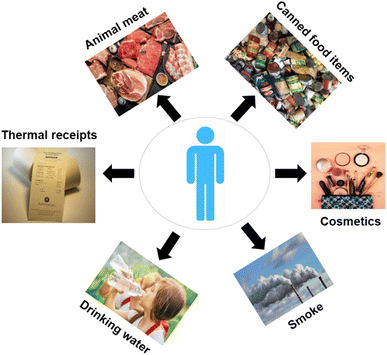 |
| Fig. 1 Schematic representation of routes of administration for bisphenol exposure in human beings. | |
BPA has been found to have the potential to induce mutagenic and carcinogenic effects, as well as disrupt endocrine functions in humans.5 Under normal conditions, BPA can leach from beverage cans, food containers, and dental sealants.8 Dental sealing materials may contain BPA-containing monomers, primarily in the form of BPA–glycidyl methacrylate. After dental sealing procedures, the level of BPA in saliva temporarily increases and then decreases.9 BPA and some of its analogues have been associated with various serious health disorders, including obesity, diabetes mellitus, respiratory disorders, behavioral disorders, breast cancer, and developmental abnormalities in teeth structure.10 Studies have shown that BPA can interfere with hormone signaling pathways by binding to hormone receptors, altering hormone metabolism, or affecting hormone transport. Numerous studies conducted on human populations have consistently revealed a noteworthy association between environmental BPA exposure and adverse health outcomes. These studies have examined diverse populations, including individuals of different age groups, socioeconomic backgrounds, and geographical locations. By analyzing the levels of BPA in urine or blood samples and assessing various health parameters, researchers have identified significant links between higher BPA exposure and a range of negative effects. While establishing causal relationships through epidemiological studies presents challenges, a mounting body of evidence from a wide range of human and laboratory studies, including those involving primates, strongly supports the correlation between environmental BPA exposure and detrimental effects in humans, with a particular focus on children.11 The detrimental effects associated with BPA exposure in humans, particularly in children, are multifaceted. They encompass a wide array of health concerns, including but not limited to developmental disorders, impaired neurobehavioral functions, hormonal disruptions, reproductive abnormalities, and an increased risk of certain cancers. The accumulated evidence not only highlights the potential harm caused by BPA but also underscores the vulnerability of children to its adverse effects due to their developing physiological systems and higher exposure levels through various sources. It is important to note that while the existing body of evidence demonstrates a strong correlation between BPA exposure and negative effects in humans, establishing direct causality can be challenging in epidemiological studies due to ethical considerations and the complex nature of human health. Nonetheless, the consistent findings from a diverse range of studies, including those involving primates, provide compelling support for the hypothesis that environmental BPA exposure contributes to adverse health outcomes, particularly in children. Additionally, laboratory studies, often conducted on primates, have contributed valuable insights into the mechanisms through which BPA exerts its detrimental influence. These studies involve controlled exposure to BPA and meticulous examination of physiological and biochemical changes. The findings from such experiments have consistently aligned with the observations from human studies, reinforcing the understanding of the correlation between BPA exposure and adverse health outcomes.
This review highlights the association between bisphenols, particularly BPA, and their toxicological effects in humans. It also explores the sources of bisphenol exposure. Furthermore, the review provides an in-depth examination of the biotransformation pathways of bisphenols in vertebrates, invertebrates, microorganisms, and plants. It also provides a comprehensive understanding of the toxic effects of bisphenols, with a specific focus on BPA, on various body systems and mechanisms. It highlights the impact of bisphenol exposure on the reproductive and endocrine system, obesity, albuminuria, and the generation of reactive oxygen species. The study also explores the biotransformation processes of bisphenols in different organisms and ecosystems, shedding light on their fate and potential environmental impact. Additionally, the article emphasizes the importance of adopting preventive measures to minimize bisphenol exposure and discusses the potential use of plant-based bioactive compounds as therapeutic agents. The findings contribute to the development of safer practices, interventions, and preventive strategies for protecting public health. This comprehensive approach and valuable insights serve as a valuable resource for researchers, policymakers, and healthcare professionals in the field, paving the way for further advancements and research in this critical area.
Bisphenol analogues
Bisphenol A
BPA (4,4′-(propane-2,2-diyl)diphenol) is a chemical compound resulting from the combination of acetone and phenol (Fig. 2). It consists of two phenol rings joined by a methyl bridge. The molecular weight of BPA is 228.286 g mol−1.8 BPA has an average solubility ranging from 20 to 300 mg L−1 at pH 7, a sediment absorbance capacity with Koc values ranging from 314 to 1524, and exhibits poor volatility.12 It displays estrogenic activity and is equally toxic to aquatic life. It is extensively used in industries for the production of epoxy resins and food containers made from polycarbonate plastics. Based on several studies, the daily intake of BPA from food is less than 1 microgram per kg of body weight. These doses have lethal endocrine-disrupting effects on the human body, especially on the fetus of women.8 Biomonitoring studies, which involve the measurement and analysis of BPA levels in biological samples, have provided valuable insights into the dynamics of BPA exposure in both humans and animals. These studies reveal that exposure to BPA can occur rapidly and persist continuously, raising concerns about the potential long-term effects of this chemical.13 In humans, biomonitoring studies have demonstrated that BPA exposure can happen quickly and ubiquitously. Researchers have detected BPA in various biological specimens, such as urine, blood, breast milk, and saliva, indicating that exposure routes are diverse and encompass multiple sources. This widespread presence of BPA in human samples suggests that individuals can come into contact with this chemical through various means, including ingestion, inhalation, and dermal absorption. Moreover, biomonitoring data have indicated that BPA exposure is not limited to specific demographic groups or geographical locations, emphasizing the universal nature of this exposure. Similarly, biomonitoring studies conducted on animals have yielded comparable results, further emphasizing the rapid and continuous nature of BPA exposure. These studies have utilized laboratory animals, including rodents and primates, to simulate real-life exposure scenarios. Through the analysis of various tissues and fluids, researchers have consistently detected BPA in these animals, indicating that exposure to this chemical can persist over time. The findings suggest that BPA can accumulate in the body and potentially exert its effects for extended periods, even after the initial exposure ceases. The rapid and continuous occurrence of BPA exposure raises concerns about its potential health implications. As BPA is a known endocrine disruptor, the continuous presence of this chemical in the body can interfere with hormone regulation and disrupt normal physiological processes. This interference can have a range of detrimental effects, including developmental abnormalities, reproductive disorders, and increased susceptibility to certain diseases. The overall pathway of BPA exposure, its excretion, and metabolites are illustrated in Fig. 3. BPA leaching from the coating of cans differs depending on the manufacturing materials used in can coating and various manufacturing methods.8 The European Union Commission (EUC) states that the quantity of BPA in food items from varnishes or other coatings via food containers should not exceed 0.05 mg kg−1. However, its use is completely prohibited in containers intended for infants. During sterilization, when products are heated at 121 °C for 90 minutes, approximately 80–100% of unconjugated BPA is released from the can wall into the food. Moreover, the migration of BPA into the food also depends on other factors such as the nature of the food content. Foods with low pH and high fat content will leach out BPA at a higher rate.7 BPA has severe effects on humans, including breast cancer, effects on fertility rate, genotoxicity, and childhood asthma/wheezing, among others.11 Table 2 lists the biological effects of BPA. It is known that BPA disrupts important metabolic processes in the human body, compromising homeostasis processes for energy and glucose and resulting in the incomplete metabolism of proteins, fats, and lipids.7
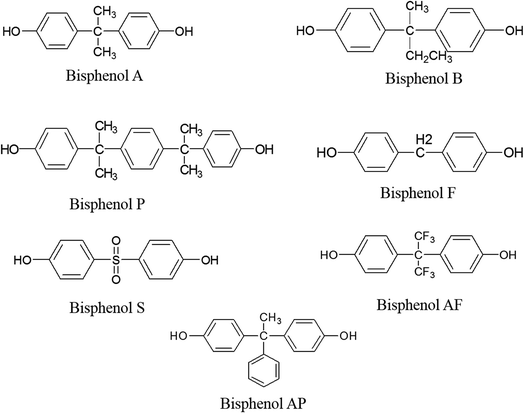 |
| Fig. 2 Chemical structures of bisphenol analogues. | |
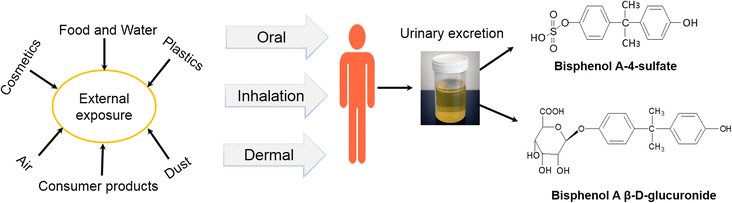 |
| Fig. 3 Schematic representation of pathways of bisphenol A (BPA) exposure, excretion, and metabolites in the human body. | |
Table 2 Bisphenol A-induced metabolic alterations in experimental animals
Sr. # |
Animal models |
BPA doses |
BPA induced effects |
Ref. |
1 |
Adult wistar rats |
250 mg per kg per day for 28 days |
Alter biochemical parameters, including blood urea nitrogen, creatinine and uric acids levels and induce the renal toxicity |
52 |
2 |
F344 female rats |
120 mg per kg per day |
BPA exposure by lactational and transplacental induce insignificant adverse effects on spermatogenesis and male sex organs |
57 |
3 |
Mice |
100 μg per kg per day or 500 μg per kg per day |
Log term BPA exposure induces anxiety-like symptom such as dizziness and cognitive deficits |
58 |
4 |
Rat |
50 μg kg−1 |
A massive rise in oxytocin immunoreactive cell number especially in the pra ventricular nucleus in female rats |
59 |
5 |
Pregnant mice |
10 mg per kg per day for 8 days |
Disturb the placental functions and can induce the abortion |
60 |
6 |
Male adult swiss mice |
25 and 100 μg kg−1 |
Impaired male fertility and spermatogenesis |
61 |
7 |
Pregnant mice |
500 mg per kg per day |
Induce reproductive toxicity in the male offspring |
62 |
8 |
Male C57BL/6 mice |
50 μg per kg per day |
Induce intestinal or gut injury |
63 |
9 |
Mice and rats |
200 mg per kg per day |
Dietary BPA intake is less toxic on sex accessory organs than subcutaneous or intraperitoneal BPA exposure |
64 |
10 |
Mice |
2 ng g−1 and 20 ng g−1 |
Induce aggressive behaviour |
65 |
11 |
Mice |
10 μg per kg per day for female BALB/c mice and 5 μg per kg per day for neonatal mice |
Induce bronchial inflammation, allergic sensation and asthma |
66 |
12 |
Rat |
25 μg per kg per day and 300 μg per kg per day |
Induce prostate gland impairment |
67 |
Bisphenol B
Bisphenol B (BPB) (2,2-bis(4-hydroxyphenyl)butane) is a congener of bisphenol, as shown in Fig. 2. It is involved in the production of epoxy resins on a large scale. The molecular weight of BPB is 242.312 g mol−1. Structurally, it differs slightly from BPA, with an ethyl group present instead of a methyl group on the central carbon atom. When compared to BPA, BPB exhibits comparatively slow aerobic and anaerobic biotransformation. It can be incorporated into food, making it a food contaminant. The presence of BPB in various products such as peeled tomatoes, commercial milk products, beverages, and food cans can induce deleterious effects on human health.14 Human urine analysis has detected BPB following a deconjugation reaction involving the use of β-glucuronidase enzyme, and it gets metabolized through conjugation with glucuronic acid or sulfate. BPB is two times more toxic than BPA and exhibits both cytotoxicity and genotoxicity. The mechanism behind its genotoxicity is the activation of the p53 gene. It has also been categorized as both an estrogenic and anti-androgenic chemical. BPB, being hydrophobic, exhibits more estrogenic activity than BPA and is more metabolically active. Its estrogenic behavior occurs through a non-genomic G-protein coupled estrogen receptor pathway, particularly at nanomolar concentrations.14 BPB is an agonist of the human receptor pregnane-X and shows a decline in corticosterone and cortisol levels.
Bisphenol F
Bisphenol F (BPF), bis(4-hydroxyphenyl)methane, consists of two phenolic rings linked together through a central bridge of methylene (Fig. 2). BPF exhibits low viscosity and high resistance, making it suitable for use in solvents. It has significantly replaced BPA in the industrial sector and is widely utilized in the production of epoxy resins and polycarbonate plastics. BPF has been detected in various consumer products, including beverages, energy drinks, commercial milk, and personal care products.14 When ingested orally, BPF enters the human body and distributes throughout the system, with the potential to cross the placental barrier. A study conducted on BPF revealed that the major excretory pathway is through urine, and the main metabolite observed was the sulfate conjugate. In vitro biotransformation, particularly in humans, leads to the formation of two important metabolites: BPF-sulfate and BPF-glucuronide. Both BPA and BPF undergo a detoxification process in vivo by conjugating with their metabolites glucuronic acid and sulfate, increasing their hydrophilic nature and facilitating excretion. BPF has been found in the human hypothalamus and white matter, suggesting its ability to cross the blood–brain barrier. It has the potential to induce oxidative lesions and single-strand breaks in DNA bases, particularly in peripheral blood mononuclear cells. Furthermore, BPF exhibits thyroid endocrine-disrupting potential by binding to thyroid receptors, resulting in increased TSH levels and altered T3 and T4 levels.
Bisphenol P
Bisphenol P (BPP), 4,4-(1,4-phenylenediisopropylidene)bisphenol, is a congener of BPA, as illustrated in Fig. 2. It plays a significant role in the extensive production of polycarbonate plastics and food containers. The presence of BPP in various products, such as peeled tomatoes, commercial milk products, beverages, and food cans, has been found to have detrimental effects on human health. Similar to other bisphenols, BPP exhibits endocrine-disrupting potential by binding to thyroid receptors and causing alterations in the levels of thyroid-stimulating hormone (TSH), T3, and T4. However, the precise mechanism underlying its effects on oxidative stress and inflammation, as observed in several studies, remains unclear.15 Upon oral ingestion, BPP enters the human body through the digestive system. Studies have reported that the primary metabolite generated during its excretion is BPP sulfate.
Bisphenol S
Bisphenol S (BPS), also known by its IUPAC name 4,4-sulfonyldiphenol (Fig. 2), has gained significant popularity as a replacement for BPA due to consumer concerns and its high toxicity. BPS plays a crucial role in the industrial sector, finding applications in cleaning agents for enhanced stain removal properties, as an important constituent of phenolic resin, and as an electroplating solvent. It has also been widely used in the production of thermal papers and other products marketed as being “BPA-free”. BPS has been detected in various everyday use products, including personal care items such as lotions, shampoos, face washes, cosmetics, as well as paper products like tickets, currency, and newspapers. Limited studies have focused on the hormonal actions of BPS, but in vitro literature suggests that BPS exhibits similar actions and potentials to BPA. Consequently, BPS may have comparable effects on human health as BPA.3 Estimating the exact potency is challenging and depends on factors such as receptor type, tissues, and the magnitude of exposure. Based on these factors, it has been observed that BPS is slightly less potent than BPA. However, the metabolism and excretory pathway of BPS are not yet fully understood.
Bisphenol AF
Bisphenol AF (BPAF), also known by its IUPAC name 4,4-(hexafluoroisopropylidene)diphenol, is a chemical compound that exhibits remarkably potent estrogenic activity (Fig. 2), surpassing other similar compounds in its ability to interact with estrogen receptors. This characteristic has contributed to its widespread usage in the production of various everyday household products. Despite its prevalent application, the precise impact of BPAF on oxidative stress, the female reproductive system, and cellular energy metabolism remains uncertain. Notably, BPAF finds extensive utility as a cross-linker in multiple industries, including electronics, optical fibers, and fluoroelastomers. Moreover, it plays a crucial role in various essential polymer applications such as plastic fibers and waveguides.16 BPAF's effectiveness in these applications has made it a significant substitute for BPA in the manufacture of epoxy resins and polycarbonate plastics.17 BPAF exerts its endocrine-disrupting effects by modulating the estrogen receptor pathway, either by activating or impeding its normal functioning. Several studies have demonstrated that BPAF induces oxidative stress, which emerges as the primary underlying cause of female reproductive dysfunctions and disorders related to ovarian follicular development. These findings highlight the potential adverse effects associated with BPAF exposure, necessitating further research to fully comprehend its implications for human health and the environment.
Bisphenol AP
Bisphenol AP (BPAP), chemically known as 4,4-(1-phenylethylidene)bisphenol, has gained significant prominence in the production of polymer materials, including polyacrylates, polyethers, and polyetherimides (Fig. 2). Its versatile applications extend beyond the realm of polymer materials, finding utility in fine chemical industries and medicinal sectors as well. BPAP has garnered recognition for its role as a flame retardant and essential plasticizer, contributing to the industrial sector's production of plastic, rubber, and other industrial products. Unfortunately, the extensive use of BPAP has resulted in numerous health hazards for human beings, posing a threat of life-threatening diseases. Studies have detected substantial amounts of BPAP in both soil and water, leading to environmental hazards and raising concerns about contamination.18 Research has revealed that BPAP interferes with the normal functioning of adipocytes, potentially contributing to the development of obesity in humans. Furthermore, even in low doses, BPAP exhibits anti-estrogenic properties that can induce serious effects. It also disrupts normal glucose homeostasis and, specifically in immature females, interferes with the process of sexual development.19 The widespread usage of BPAP has contributed to the contamination of both the environment and food sources, exacerbating the potential risks associated with its exposure. The presence of BPAP in soil and water systems underscores the urgent need for comprehensive assessments of its impact on human health and the environment. Efforts should be made to minimize the use of BPAP and explore alternative materials that are safer for both humans and the ecosystem.
Analytical methods for the detection of bisphenols
The detection of bisphenol analogue compounds in the human body typically relies on various chemical analysis techniques. Among the most commonly utilized methods are gas chromatography (GC), high-performance liquid chromatography (HPLC), mass spectrometry (MS), and liquid chromatography-tandem mass spectrometry (LC-MS/MS).20,21 In addition, surface-enhanced Raman scattering and enzyme-linked immunosorbent assay (ELISA) have also been employed for this purpose.20,21 These analytical techniques enable the accurate identification and quantification of bisphenol analogues present in biological samples. The samples used for chemical analysis of bisphenols in the human body can vary and typically include urine, breast milk, and blood serum. Urine tests are commonly employed to measure the excreted amount of bisphenols, providing insights into the level of exposure. Serum tests, on the other hand, measure the concentration of bisphenol analogues in the bloodstream, reflecting their systemic distribution and potential effects on various organs and tissues. In some cases, chemical tests are conducted to detect traces of bisphenol analogues in specific body tissues or organs, further expanding our understanding of their localization and potential health implications.22 These medical analyses, combined with chemical techniques, serve as crucial tools for assessing the presence and impact of bisphenol analogue compounds in the human body. By utilizing various sample types and employing sensitive detection methods, researchers can gather comprehensive data regarding the extent of exposure and potential health risks associated with these compounds.20 These analytical techniques, alone or in combination, provide valuable tools for the detection, identification, and quantification of bisphenols in different sample matrices, enabling researchers to assess exposure levels and potential health risks associated with these compounds.
The life-threatening aspects of bisphenols on human beings
Bisphenols, such as BPA and its analogues, pose life-threatening aspects to human health. These compounds act as endocrine disruptors, interfering with hormonal functions and potentially leading to reproductive health issues, fetal development problems, and hormone-related cancers. Bisphenols have been linked to an increased risk of breast cancer, prostate cancer, and ovarian cancer. Additionally, they can contribute to cardiovascular disorders like hypertension and atherosclerosis, as well as metabolic disorders including obesity, insulin resistance, and diabetes. Bisphenol exposure has been associated with neurodevelopmental disorders, cognitive impairments, and behavioral abnormalities, particularly in children. Furthermore, these compounds can cause organ damage, such as liver dysfunction and kidney damage. While more research is needed to fully understand the long-term impacts, minimizing exposure to bisphenols is crucial to mitigate these life-threatening risks to human beings. In the following subsections, we have briefly discussed these life-threatening effects of bisphenols.
Hormonal dysfunctionalities
Exposure to BPA poses significant risks to reproductive health, including the potential for premature labor. BPA disrupts the normal functioning of the hormone system, which can lead to hormonal imbalances and complications during pregnancy. Furthermore, BPA exposure can induce oxidative stress, an imbalance between the production of harmful free radicals and the body's ability to neutralize them. Oxidative stress can cause inflammation and tissue damage, contributing to the onset of premature labor. Studies have explored the relationship between BPA exposure during pregnancy and its impact on birth outcomes. One study conducted on pregnant Mexican women collected spot urine samples during the third trimester and found a positive association between higher overall BPA levels and premature delivery (less than 37 weeks). The study, although limited by a small sample size (N: 12), suggests that elevated BPA levels may be linked to shortened gestational periods and lower birth weights.11 Bisphenol analogues, including BPA, can also disrupt the oogenesis process, which refers to the development and maturation of oocytes (eggs) in females. These compounds interfere with the initiation of meiosis, the process through which oocytes divide and follicles form. This interference can lead to adverse effects on the quality and quantity of oocytes, classifying bisphenols as ovarian toxicants. The detrimental impact on oogenesis and fertility rates in women further emphasizes the importance of minimizing exposure to bisphenol analogues to safeguard reproductive health.23 Given the potential risks associated with bisphenol exposure, particularly BPA, it is crucial to take measures to limit exposure and protect reproductive health, especially during pregnancy. This includes avoiding products that contain bisphenols, such as certain plastics and food containers, and opting for safer alternatives.
BPA can exert various effects on hormonal homeostasis, which can disrupt normal physiological processes. BPA has been shown to impede cholesterol transport across the mitochondrial membrane, leading to disturbances in cellular cholesterol metabolism. This interference can have implications for hormone synthesis and signaling pathways. Additionally, BPA has been associated with accelerated fatty acid oxidation, which can disrupt energy metabolism and impact hormonal regulation. One notable effect of BPA is its ability to enhance the release of prolactin, a hormone involved in lactation and reproductive function. Increased levels of prolactin can have widespread effects on hormonal balance and potentially lead to reproductive complications. BPA also has the capability to interfere with the transcription process of thyroid hormone receptors, potentially hindering the normal function of these receptors in regulating metabolic activity.24 The structural similarity of BPA to thyroid hormones, such as T3 and T4, enables BPA to bind with serum transport proteins that normally carry thyroid hormones. This interaction can disrupt thyroid hormone homeostasis and interfere with their normal distribution and activity. Consequently, BPA has the potential to impact thyroid function and affect various physiological processes regulated by thyroid hormones.25 In some studies, higher levels of BPA have been associated with alterations in thyroid hormone levels. Specifically, an increase in T3 levels and low levels of TSH have been observed in individuals with elevated BPA exposure.11 These findings suggest that BPA may disrupt the normal balance of thyroid hormones in the body, which can have broad implications for metabolism, growth, and development. Understanding the effects of BPA on hormonal homeostasis, including its impact on cholesterol transport, fatty acid oxidation, prolactin release, and thyroid hormone disruption, helps shed light on the potential mechanisms through which BPA can influence physiological processes and contribute to adverse health outcomes. Further research is necessary to fully elucidate the complex interactions between BPA and hormonal regulation and their implications for human health.
Cardic toxicity
Long-term exposure to bisphenol analogues, such as BPA, can have detrimental effects on the cardiovascular system, specifically causing cardiac toxicity. This refers to the weakening of the heart muscle and its ability to contract effectively. As a result, individuals exposed to bisphenol analogues may face an elevated risk of experiencing heart attacks, strokes, and various other cardiovascular diseases. The adverse impact of bisphenol analogues on the heart can be intensified by the presence of other environmental toxins, like air pollution. When combined, these factors create a synergistic effect that further impairs the cardiovascular system. Additionally, lifestyle choices such as smoking and a poor diet can exacerbate the cardiac toxicity caused by bisphenol analogues. BPA and other bisphenol analogues are classified as endocrine disruptors, meaning they can interfere with the normal functioning of the endocrine system, which regulates hormone production and distribution in the body. Studies have shown a positive association between BPA exposure and the occurrence of cardiovascular diseases.26 Furthermore, these compounds have the capacity to disrupt the cardiovascular system, leading to severe cardiovascular events such as arrhythmias, myocardial infarction (heart attack), atherosclerosis (hardening of the arteries), hypertension (high blood pressure), dilated cardiomyopathy (enlarged heart), and coronary artery disease. Research has revealed that elevated levels of BPA detected in urine samples are linked to specific cardiovascular events, including angina pectoris (chest pain due to reduced blood flow to the heart) and myocardial infarction.27 BPA is classified as an exogenous compound that structurally resembles estrogen, a hormone with significant influence on cardiovascular health. The toxic effects of estrogen on the cardiovascular system depend on various factors, such as an individual's gender, the dosage or strength of exposure, and age.26
Metabolic abnormalities
One potential effect of BPA on diabetes risk is its impact on insulin production and release by the pancreas. BPA has been found to interfere with the proper functioning of pancreatic cells responsible for producing and releasing insulin. This disruption can impair the body's ability to effectively regulate blood sugar levels. Furthermore, BPA has been associated with an increase in the production of glucagon, a hormone that opposes the effects of insulin. Glucagon stimulates the liver to release stored glucose into the bloodstream, thereby raising blood sugar levels. Elevated glucagon levels caused by BPA can contribute to insulin resistance and disrupt glucose homeostasis. Another factor linking BPA to diabetes is its association with hepatic steatosis, or the accumulation of fat in the liver. BPA exposure has been shown to increase fat deposition in the liver, which can lead to insulin resistance and impaired glucose metabolism. In addition to its effects on insulin and glucose regulation, BPA can also disrupt lipid metabolism. It can interfere with the metabolism of fats, leading to an accumulation of fat in the body. This excess fat deposition can further contribute to insulin resistance and increase the risk of diabetes. BPA is believed to disrupt the endocrine system, resulting in imbalances in hormone production and other metabolic changes that contribute to an increased risk of diabetes.28 Further research is still needed to fully elucidate the mechanisms through which BPA influences diabetes development and to better understand the extent of its impact on human health.
Bisphenols, including BPA, are well-known for their endocrine-disrupting properties, meaning they can interfere with the body's normal hormonal regulation and metabolic processes. In the case of BPA, there is evidence linking its exposure to impaired glucose metabolism and insulin sensitivity, which can elevate the risk of developing diabetes. The precise mechanism by which BPA is associated with diabetes is not yet fully understood. However, it is believed that BPA disrupts the endocrine system, leading to a dysregulation of hormone production and signaling. This disruption can ultimately result in insulin resistance, a condition where the body's cells become less responsive to the effects of insulin, leading to elevated blood sugar levels and an increased risk of T2DM. Adipose tissue, commonly known as fat tissue, has been identified as a significant site for the accumulation of BPA due to its unique ability to store and slowly release hormones and other chemicals over time. This characteristic makes adipose tissue a potential reservoir for BPA accumulation, leading to an increased risk of long-term adverse health effects.29 The precise mechanism by which BPA accumulates in adipose tissues is not yet fully understood. However, it is believed that BPA enters the adipose tissue through the circulatory system. Once in the bloodstream, BPA can be transported to adipose tissue, where it can become sequestered within the fatty tissue and accumulate over time. Once inside adipose tissue, BPA may exert its effects by interfering with the production and secretion of adipokines. Adipokines are hormones secreted by adipose tissue that play crucial roles in regulating various metabolic processes, including metabolism and glucose homeostasis. Disruption of adipokine production by BPA can contribute to metabolic dysregulation, insulin resistance, and an increased risk of developing diabetes.30 The specific mechanisms by which BPA affects adipokine production and function are still being investigated. It is hypothesized that BPA may interact with receptors on adipose tissue cells or interfere with intracellular signaling pathways, thereby disrupting the normal synthesis and secretion of adipokines. The alteration of adipokine levels and their subsequent impact on metabolic processes can contribute to the development of diabetes and other metabolic disorders. It is worth noting that BPA accumulation in adipose tissue is a gradual process that occurs over time, as BPA is released slowly from the tissue into the circulation. This slow release of BPA from adipose tissue can contribute to prolonged exposure to the chemical, increasing the potential for adverse health effects associated with BPA exposure. Adipose tissue serves as a major site for BPA accumulation due to its capacity to store and release hormones and chemicals, making it susceptible to long-term BPA exposure. The exact mechanism of BPA accumulation in adipose tissue is not fully understood, but it is believed that BPA enters the tissue through the bloodstream. Once inside, BPA can disrupt the production and release of adipokines, contributing to metabolic dysregulation and an increased risk of developing diabetes. Further research is needed to fully elucidate the mechanisms underlying BPA's effects on adipose tissue and its implications for metabolic health.
BPA has been observed to have detrimental effects on insulin signaling pathways, contributing to metabolic dysfunction. These effects may arise from BPA's ability to bind and activate various nuclear receptors, including the estrogen receptor and the peroxisome proliferator-activated receptors (PPARs). BPA has a structural resemblance to estrogen, allowing it to bind to estrogen receptors. Activation of estrogen receptors by BPA can disrupt insulin signaling pathways and perturb glucose homeostasis. This interference can lead to impaired insulin sensitivity and reduced glucose uptake by cells, contributing to elevated blood glucose levels. In addition to estrogen receptors, BPA can also bind to the insulin receptor, a key component of insulin signaling pathways. By binding to the insulin receptor, BPA can hinder its normal functioning, interfering with the transmission of insulin signals. This disruption can impair the uptake of glucose by cells and result in elevated blood glucose levels. The impairment of insulin signaling pathways by BPA ultimately leads to a condition called insulin resistance. Insulin resistance is characterized by reduced responsiveness of cells to insulin's actions, particularly in stimulating glucose uptake. As a result, higher levels of insulin are needed to achieve normal glucose control. Over time, this chronic state of elevated insulin and impaired glucose regulation can contribute to the development of T2DM. Insulin resistance and subsequent hyperglycemia (high blood sugar) are key components of the pathogenesis of type 2 diabetes. BPA's interference with insulin signaling pathways and its impact on glucose metabolism contribute to insulin resistance and an increased risk of developing T2DM type 2 diabetes.31 It is important to note that the precise mechanisms by which BPA disrupts insulin signaling pathways are still an active area of research. Further studies are needed to fully understand the specific interactions and downstream effects of BPA on insulin receptors, nuclear receptors, and the intricate signaling networks involved in glucose metabolism and insulin sensitivity.
Recent studies have provided evidence suggesting a potential association between bisphenols, particularly BPA, and obesity. BPA has been found to disrupt the body's endocrine system, which can increase the risk of weight gain and the development of obesity. One study identified a higher risk of obesity in individuals who were repeatedly exposed to BPA.10 Long-term exposure to BPA has been linked to a significantly increased risk of higher body mass index (BMI), particularly in non-PCOS (polycystic ovary syndrome) women. However, it is important to note that some studies have indicated that high caloric intake and various lifestyle factors may also contribute to the increase in BMI. Moreover, elevated levels of BPA in the body can be attributed to the use of materials containing BPA in food packaging. Further research has explored the relationship between BPA exposure and BMI in children who received tooth services involving dental composite fillings. It was found that these children did not experience a significant increase in BMI.11 However, a cross-sectional study revealed a link between higher BPA levels and relatively high serum leptin concentrations, as well as increased BMI and fat percentage. Investigational studies have also focused on the inflammatory effects of BPA exposure in women, particularly those with or without PCOS. These studies measured various endpoints related to severe inflammation caused by BPA exposure. The findings revealed a significant increase in serum BPA levels, particularly in individuals with an enlargement of the spleen, which is considered a positive sign of chronic inflammation. Women with PCOS also exhibited elevated levels of inflammation biomarkers such as IL-6, hepatic steatosis, and C-reactive protein, indicating an association between BPA exposure, inflammation, and PCOS-related symptoms. Overall, these studies suggest that bisphenols, especially BPA, may be associated with obesity and related health issues. The disruption of the endocrine system by BPA and its impact on factors such as inflammation, hormone regulation, and metabolic processes contribute to the potential risk of weight gain and obesity. Further research is needed to elucidate the precise mechanisms and establish a clearer understanding of the relationship between bisphenols, obesity, and associated health conditions.
Generation of oxidative stress
Repeated high exposure to BPA at both millimolar and micromolar doses is expected to result in DNA damage and cytotoxic events, particularly hepatocellular carcinoma. Even at low doses, such as nanomolar levels, BPA can cause DNA strand breakage.32 Some studies suggest that the breakage of DNA strands at low doses may be induced by reactive oxygen species (ROS). However, the introduction of antioxidants and specific protein inhibitors may reverse this effect. It has also been reported that high micromolar doses of BPA can lead to transient compaction of chromatin.33 Exposure to BPA during early life, even below the Reference Dose (RfD), which is the safe level of exposure set by regulatory agencies, can contribute to the development of neoplastic changes in the mammary and prostate tissues.34 The generation of phenoxy radicals and ROS following BPA exposure may induce genotoxicity, further increasing the risk of cellular damage.35 BPA exposure has also been associated with albuminuria, an increase in urinary albumin levels. This condition is considered an indicator of endothelial dysfunction, particularly in the kidneys, and is associated with an elevated T2DM and cardiovascular diseases. Several studies have found a strong positive association between BPA exposure and low-grade albuminuria.11 The precise mechanism by which BPA exerts toxic effects on kidney endothelium is not yet fully understood. However, it has been proposed that BPA-induced oxidative stress on renal parenchymal cells plays a role in endothelial dysfunction.36 Moreover, BPA has been found to induce apoptosis, or programmed cell death, in both human and animal cells. The mechanism behind BPA-induced apoptosis involves the generation of reactive oxygen species by mitochondria. These reactive oxygen species can damage mitochondrial proteins, leading to mitochondrial dysfunction. Subsequently, cytochrome c, an apoptotic initiator protein, is released into the cytoplasm. Cytochrome c binds to apoptotic protease activating factor 1 (APAF1), forming the apoptosome, which activates caspase-9. Caspase-9, in turn, initiates a cascade of events involving downstream caspases, such as caspase-3, leading to protein breakdown, DNA fragmentation, and ultimately, cell death.31
Biotransformation of bisphenols
After oral administration, bisphenols, such as BPA, pass through the gastrointestinal tract (GIT) and the liver before reaching their target tissues or organs, including the fetus and uterus. To fully understand the mechanism responsible for the serious and potentially life-threatening effects of bisphenols, such as BPA, it is essential to explore the fate of these compounds as they pass through the hepato-intestinal pathway. The hepato-intestinal pathway serves as a defensive barrier against various harmful chemicals, thanks to the presence of xenobiotic metabolizing enzymes (XMEs). XMEs can be categorized into three main classes: phase I enzymes, phase II enzymes, and drug transporters (as shown in Fig. 4). Phase I enzymes primarily belong to the cytochrome P450 (CYP450) enzyme class. These enzymes are capable of metabolizing BPA through detoxification processes and the activation of inactive prodrugs, if applicable. Phase II enzymes have the ability to conjugate the products generated by phase I enzymes. They can also conjugate intermediate products and other intracellular substrates, such as steroidal products and bilirubin. The third category of XMEs includes drug transporters, which are membrane-bound proteins that play a crucial role in the uptake and excretion of drugs and other compounds. The biotransformation process of BPA occurs in various organisms, including vertebrates, invertebrates, plants, and microorganisms such as bacteria, algae, and fungi.37 Understanding the intricate processes and interactions within the hepato-intestinal pathway and the role of XMEs is essential for comprehending the metabolism and potential toxicity of bisphenols like BPA. Further research is needed to explore the specific mechanisms involved and their implications for human health.
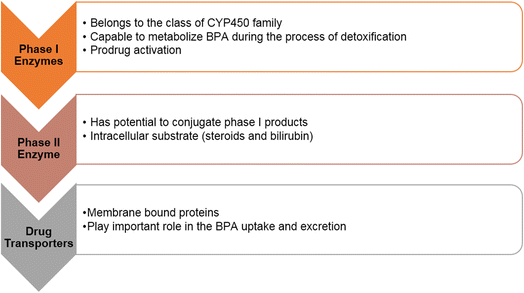 |
| Fig. 4 Involvement of phase I and phase II enzymes and drug transporters in the biotransformation of bisphenols. | |
Biotransformation in animals
In vertebrates, the biotransformation process of BPA involves oxidation, primarily through hydroxylation reactions. These reactions are catalyzed by microsomal enzymes, including the CYP450 enzyme, as observed in studies on the metabolic behavior of orally administered BPA-C14. As a result of direct hydroxylation, important end metabolites such as 2,2-bis(4-hydroxyphenyl)propanol and D-3-hydroxybisphenol-A (3-OH-BPA) are produced, primarily at the BPA ring.5 Furthermore, the cleavage of BPA leads to the formation of a significant metabolite, 4-isopropyl hydroxyphenol. BPA quinone, derived from BPA, is involved in the generation of BPA DNA adducts, which can cause severe damage to genetic material and contribute to increased BPA toxicity.38 BPA quinone participates in redox reactions and produces highly reactive oxygen species, which can induce toxic effects in both humans and animals. These metabolites are subsequently further bio-transformed in the presence of CYP450 enzymes, resulting in compounds that exhibit greater estrogenic activity compared to BPA alone. The enhanced estrogenic activity of these metabolites contributes to their potential impact on hormonal processes and associated biological effects. Understanding the specific metabolic pathways and transformations of BPA and its metabolites is crucial for assessing their toxicity and potential health risks. Further research is needed to comprehensively elucidate the intricate processes involved in BPA biotransformation and their implications for human and environmental health.
It has been well-documented that BPA undergoes conversion to 4-methyl-2,4-bis(4-hydroxyphenyl)pent-1-ene (MBP) in the liver of animals, such as rats, as observed in the S9 fraction.39 These metabolites have been shown to conjugate with glucuronides and sulfates, facilitating their elimination from the body primarily through the fecal route, with only small amounts excreted in the urine.40 BPA can also serve as a substrate for various enzymes, including estrogen sulfotransferase and estrone sulfatase. Less than 1% of BPA undergoes unconjugation and is excreted from the body, while the majority tends to accumulate in organs and tissues. Further investigations have revealed that these metabolites can also form conjugates with glutathione and thiols.41 Regarding invertebrates, there is limited available data on BPA biodegradation. However, a study examining the toxicokinetics of BPA in freshwater organisms, specifically Pisidium amnicum, reported half-lives of BPA at temperatures of 1.8 °C and 11.6 °C as 221 hours and 43 hours, respectively.42 Understanding the fate and elimination pathways of BPA and its metabolites is important for evaluating the potential exposure risks and environmental impact of this compound. Further research is needed to enhance our knowledge of BPA metabolism in various organisms and ecosystems.
Biotransformation in bacterias
Many bacteria have the potential to biotransform BPA, which can be found in soil, river water, and plants involved in wastewater treatment.42 In bacteria, the detoxification process plays an important role in the biotransformation of bisphenols. BPA metabolism primarily occurs through the glucuronidation process, mediated by the UGT2B1 isoform of UDP-glucuronosyltransferase. Glucuronidation is a major elimination process that converts lipophilic substrates into hydrophilic compounds. A study was conducted to evaluate the metabolizing potential of bacterial strains extracted from river water for BPA biotransformation under both aerobic and anaerobic conditions. The study revealed that, in the case of BPA, approximately 60% of the carbon was converted into carbon dioxide through mineralization, 20% was transformed into organic compounds, and the remaining 20% was incorporated into bacterial cells.43 It has been reported that environmental air can be effectively cleared of BPA by microorganisms, specifically bacteria, capable of metabolizing BPA. Studies have shown that BPA can be completely degraded by a mixed culture of Pseudomonas and Sphingomonas species under pressure of 100 ppm within 7 days. The metabolites produced by bacteria may exhibit varying levels of toxicity. It has also been reported that BPA can be O-methylated by Mycobacterium sp. (Mycobacterium vanbaalenii), resulting in the production of derivatives belonging to the mono and dimethyl ether groups.37 Factors such as bacterial counts and temperature can affect the degradation of BPA. The half-lives of BPA biotransformation are typically below 5 days, and bacteria with the potential to degrade BPA are widely distributed in river water.42 Understanding the microbial degradation of BPA is crucial for assessing its environmental fate and the potential for bioremediation strategies. Further research is needed to explore the diversity of bacterial species involved in BPA degradation and to elucidate the mechanisms underlying their metabolic activities.
Biotransformation in fungi
Fungi have the potential to metabolize bisphenols; however, such fungi are relatively rare in nature. They primarily utilize aromatic substrates derived from natural sources, such as lignin and phenolic compounds, to carry out the metabolism of bisphenols.5,42 Lignin-degrading enzymes, particularly manganese peroxidase (MnP) and laccase produced by white rot Basidiomycetes fungi, play a significant role in the biodegradation of BPA, as depicted in Fig. 5. MnP isoforms have different molecular weights ranging from 32 to 62.5 kDa, while laccase has a molecular weight of 150 kDa.44 In the case of laccase, two intermediates, namely 1-hydroxybenzotriazole (HBT) and 2-azino-bis(3-ethylbenzthiazoline-6-sulfonate), enhance the degradation of BPA.42 Some inhibitors, such as Hg2+, Ag+, Pb2+, NaN3, ascorbate, and lactic acid, can inhibit the enzymatic action of MnP,44 whereas laccase activity is strongly influenced by redox mediators.45 BPA degradation mediated by MnP leads to the loss of estrogenic activity, and the resulting metabolites mainly include phenols such as 4-isopropenylphenol, 4-isopropylphenol, and hexestrol. On the other hand, degradation by laccase involves the addition of phenolic moieties or the removal of oligomers, resulting in the production of 4-isopropenylphenol. Studies have shown that ligninolytic fungi, including Trametes versicolor, Stereum hirsutum, and Pleurotus ostreatus, are capable of degrading BPA.42 However, it should be noted that the laccase enzyme of some fungi does not degrade BPA but instead targets other compounds like octylphenol and nonylphenol. Further research is needed to explore the diversity of fungi involved in BPA degradation and to understand the underlying mechanisms of their enzymatic activities.
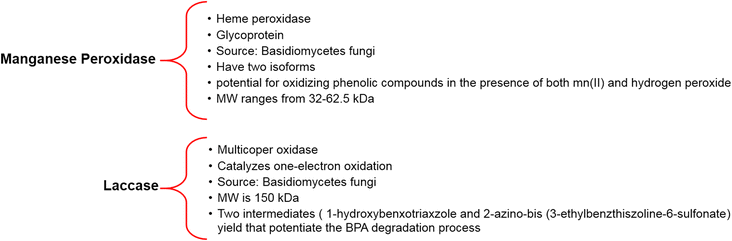 |
| Fig. 5 Lignin-degrading enzymes and their role in the biotransformation of bisphenols. | |
Biotransformation in planktons
Plankton, which are freshwater floating organisms, have the potential to degrade bisphenols like BPA. In addition to BPA, they are also capable of removing various pollutants, including heavy metals like chromium and lead.45 Algae initiate the photo-degradation of BPA through irradiation with near-ultraviolet light. This process leads to the production of certain secretions under heavy irradiation, which generate hydroxyl radicals (OH) capable of removing pollutants from the environment.46 Algae can accelerate the photo-degradation process by undergoing a heating process for 15 minutes. Maintaining an appropriate proportion of humic acid to Fe3+ (e.g., 4 mg L−1 humic acid and 20 mmol L−1 Fe3+) is crucial for enhancing the photo-degradation efficiency of algae. A study revealed that Chlorella fusca var. vacuolata can undergo proper light exposure for 120 hours, resulting in the production of an intermediate called mono-hydroxybisphenol-A.42 Green algae, such as Monoraphidium braunii, have also shown the ability to remove BPA from water.5 Table 3 provides a list of important microorganisms, including bacteria, fungi, and algae, that are capable of BPA biotransformation.
Table 3 Microorganisms that are capable of biotransformation of bisphenol
Microorganisms |
Strains |
Source |
Degradation potential |
Bacteria |
Pseudomonas sp. |
River water |
Effective |
S. bisphenolium |
Soil |
Very effective |
Aeromonas hydrophilia |
Oil spilled soil |
Effective |
S. bisphenolicum |
Soil |
Very effective |
Enterobacter |
Plants rhizosphere |
Average |
Pseudomonas sp. |
Soil |
Very effective |
Bacillus sp. |
Soil |
Effective |
M. vanbaalenii |
Estuarine sediments |
Average |
Algae |
C. fusca |
— |
Average |
M. braunii |
— |
Average |
C. vlgari |
— |
Average |
Fungi |
T. villosa |
Wood |
Effective |
Aspergillus sp. |
Tannery effluents |
Effective |
I. lacteus |
Soil |
Very effective |
C. stipitatum |
Columbian forest |
Very effective |
Biotransformation in plants
The major route of BPA metabolism in plants is glycosylation, a process where BPA is attached to sugar molecules. Two important oxidative enzymes, polyphenol oxidase and peroxidase, play a pivotal role in the degradation of BPA in plants. Various factors such as physiological stress, plant wounds, and infections, including microbial or viral, can initiate self-protection mechanisms in plants.42 Plants primarily acquire BPA from water and then undergo BPA metabolism to produce BPA glycosides. A study conducted on Nicotiana tabacum plant cultures (BY-2) revealed that BPA is mainly absorbed through the roots. The roots produce two important metabolites: mono-O-gentiobioside and trisaccharide-BPA-mono-O-glucopyranosyl-(1→4)-[D-glucopyranosyl-(1→6)]-D-glucopyranoside. In addition to these major metabolites, minor metabolites such as mono and di-O-β-D-glucopyranosides were also detected, which exhibit weak estrogenic behavior.5 Studies have suggested that BPA metabolites are primarily found in the form of BPA bases in the roots (10%), while only trace amounts are present in the stems. Another plant species, Ipomoea aquatica, has been found to have the potential to metabolize BPA through oxidation.47
Preventive measures against bisphenols-induced toxicity
In a recent study, the health communities have investigated the toxic effects of BPA and provided safety guidelines to reduce BPA exposure in humans.48 One of the major sources of BPA exposure is through leaching from food packaging items and canned foods. To minimize BPA exposure, it is recommended to reduce the use of canned food items and replace them with glass jars, which are bisphenol-free and environmentally friendly packaging options. However, it is important to note that these glass jars may be more expensive compared to plastic alternatives.49 Furthermore, another study suggests that if fresh fruits and vegetables are not readily available, it is advisable to purchase frozen varieties instead of canned ones. This helps to avoid BPA exposure from canned foods. In order to protect infants from BPA exposure, it is recommended to use BPA-free bottles and opt for non-toxic wooden toys instead of plastic ones. It is also advised to choose plastic-free baby items and consider replacing plastic products with glass or wooden alternatives. BPA is commonly found in plastic types 3 and 7, while types 1, 2, 4, 5, and 6 are generally free from BPA. Additionally, microwaving food can contribute to the incorporation of bisphenols into the food. It is recommended to heat food using a flame instead of relying on a microwave whenever possible. Thermal receipts, which often contain BPA, can be replaced with virtual receipts that customers can receive through email or other electronic means. When purchasing products, especially for infants or children, it is advisable to look for the “MADE SAFE” seal, indicating that the product meets safety standards and is free from harmful substances. Fig. 6 illustrates some of the preventive measures that can be taken to reduce BPA exposure. Overall, these measures aim to minimize BPA exposure in order to safeguard human health and well-being.
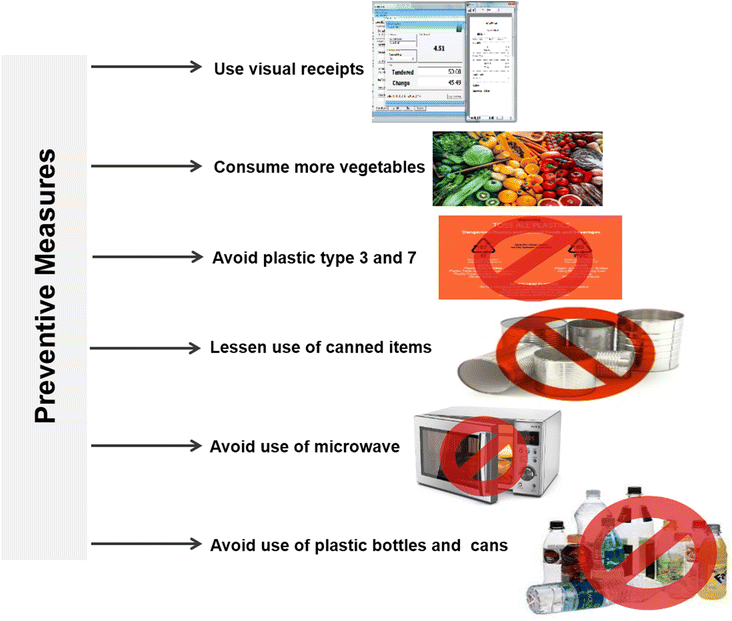 |
| Fig. 6 Preventive measures to minimize bisphenol exposure. | |
Therapeutic interventions of bisphenol-induced metabolic disorders via plant-based bioactive compounds
Plant-based bioactive compounds, known as phytochemicals, are extracted from plants or their by-products and have shown promising roles in mitigating bisphenol-induced dysfunctions.50 In particular, an herbal medicinal product called Asparagus officinalis has been studied for its biological potential, including its anti-inflammatory and antioxidant properties. Additionally, it has been found to possess anti-hepatotoxic properties, providing protection against the harmful effects of bisphenols on the liver.51 One specific flavonoid compound called luteolin, which is found in green pepper, seeds, and perilla leaves, exhibits significant antioxidant activity. When administered orally in moderate doses (100 to 200 mg kg−1), luteolin has been shown to reduce BPA-induced toxic effects on the kidneys and maintain normal levels of blood urea nitrogen, creatinine, and serum uric acid. Furthermore, luteolin enhances the expression of heme oxygenase 1 (HO-1), which provides a protective effect against the detrimental effects of reactive oxygen species generated by BPA.52
Another study has revealed the antioxidant properties of N-acetylcysteine, an organosulfur compound extracted from Allium sativum (garlic). N-Acetylcysteine, when administered at a dose of 10 mg kg−1, has the potential to minimize cardiac toxicity induced by BPA and plays a significant role in reducing inflammation caused by ROS.53 Naringin, a flavonoid glycoside naturally found in the peels of citrus fruits such as grapefruits and oranges, has been shown to protect against BPA-induced oculopathy. Oculopathy is an eye disease that can develop and progress due to long-term exposure to bisphenol A. Studies have demonstrated that naringin, administered at a dose of 80 mg kg−1, effectively prevents BPA-induced oculopathy.54
BPA induces fatal and damaging effects on human kidneys, leading to a reduction in kidney weight. Quercetin, a flavonoid found in green tea, apples, berries, and onions, has the potential to diminish the toxic effects of BPA on kidneys. The protective effect of quercetin against BPA-induced renal damage was determined by assessing parameters such as glutathione content, ROS levels, and mitochondrial membrane damage. It has been observed that quercetin, when administered at a dose of 75 mg kg−1, plays an important role in reducing oxidative damage to mitochondria in cases of kidney damage caused by BPA.55 On the other hand, BPA-induced reproductive toxicities can be treated with the plant extract of Adiantum capillus-veneris, commonly known as southern maidenhair fern. The extract of Adiantum capillus-veneris, when administered at a dose of 25 mg kg−1, exhibits antioxidant potential and helps in diminishing and managing the estrogenic effects of bisphenols.56
Certain flavonoids, such as luteolin and naringin, have also shown therapeutic potential against bisphenol-induced metabolic disorders. Luteolin, found in green pepper, seeds, and perilla leaves, has antioxidant activity and can reduce the toxic effects of bisphenols on the kidneys. Naringin, naturally present in citrus fruit peels like grapefruit and oranges, has been found to safeguard against oculopathy induced by bisphenol exposure, protecting the eyes from long-term damage. Additionally, plant extracts like Adiantum capillus-veneris (southern maidenhair fern) have been studied for their potential in managing bisphenol-induced reproductive toxicities. The extract of Adiantum capillus-veneris exhibits antioxidant properties and can help diminish the estrogenic effects of bisphenols, thereby protecting reproductive health.
Plant-based bioactive compounds offer promising therapeutic interventions for bisphenol-induced metabolic disorders. Their antioxidant, anti-inflammatory, and organ-protective properties make them valuable candidates for mitigating the harmful effects of bisphenols on various organs and metabolic processes in the body.
Conclusion
The key findings of the studies described above provide compelling evidence of the detrimental effects of bisphenol analogues, specifically BPA, on cardiovascular health, metabolic processes, and overall well-being. Bisphenol analogues, classified as endocrine disruptors, have the ability to interfere with the normal functioning of the endocrine system, leading to a cascade of negative health outcomes. One of the significant findings is the association between bisphenol analogue exposure and cardiovascular toxicity. Prolonged exposure to BPA has been linked to an increased risk of cardiovascular diseases such as heart attacks, strokes, and atherosclerosis. This association is further exacerbated by factors such as air pollution, lifestyle choices, and the structural resemblance of BPA to estrogen, a hormone that plays a crucial role in cardiovascular health. The impact on the cardiovascular system emphasizes the need for stricter regulations and measures to reduce bisphenol analogue exposure, especially in vulnerable populations.
Moreover, the data highlights the association between BPA exposure and an elevated risk of developing diabetes. BPA disrupts insulin production and release by the pancreas, interferes with glucose regulation, and disrupts lipid metabolism, all of which contribute to insulin resistance, impaired glucose homeostasis, and an increased risk of type 2 diabetes. The accumulation of BPA in adipose tissue further exacerbates metabolic dysregulation and the risk of diabetes. These findings underscore the urgent need to reduce bisphenol analogue exposure and explore alternative materials and chemicals in various consumer products. Furthermore, bisphenol analogues, particularly BPA, have been linked to obesity. The disruption of the endocrine system by BPA can lead to weight gain and obesity. Inflammation, hormone regulation, and metabolic processes are key factors contributing to this association. This highlights the importance of considering the potential obesogenic effects of bisphenol analogues when evaluating the risk factors for obesity.
Additionally, the data indicates that BPA exposure poses additional health risks, including DNA damage, cytotoxic events, and potential carcinogenic effects. The ability of BPA to induce DNA strand breakage, generate reactive oxygen species, and contribute to neoplastic changes in tissues raises concerns about its long-term impact on human health. Furthermore, BPA exposure has been associated with albuminuria and renal endothelial dysfunction, which are risk factors for T2DM and cardiovascular diseases.
Understanding the fate of bisphenols like BPA as they pass through the hepato-intestinal pathway is crucial for comprehending their metabolism and potential toxicity. The role of xenobiotic-metabolizing enzymes in the detoxification and conjugation processes of BPA within this pathway needs further investigation. These findings underscore the need for stricter regulations, further research, and public awareness regarding the potential health risks associated with bisphenol analogue exposure. Mitigating exposure to these compounds and exploring safer alternatives in various consumer products can contribute to improved public health outcomes and reduce the burden of diseases linked to bisphenol analogues.
Data availability
All data generated and/or analyzed during this study are included in this published article.
Author contributions
KR and MSHA: project administration, supervision, investigation, conceptualization, writing-final draft, and editing. MSHA and SR: literature search, data curation, writing-original draft, validation. MAA and MI: data curation, data analysis, draft preparation and editing.
Conflicts of interest
The authors declare that they do not have any conflict of interest for this article.
Acknowledgements
The authors would like to express their appreciation to the Deanship of Scientific Research at King Khalid University, Saudi Arabia (grant number RGP-2/370/44) and Higher Education Commission (HEC) of Pakistan (5661/Punjab/NRPU/R&D/HEC/2016) for providing funding for this research through the research group program.
References
- H. Sarma and W. Y. Lee, Bacteria enhanced lignocellulosic activated carbon for biofiltration of bisphenols in water, Environ. Sci. Pollut. Res. Int., 2018, 25(18), 17227–17239 CrossRef CAS
. - D. Chen, et al., Bisphenol Analogues Other Than BPA: Environmental Occurrence, Human Exposure, and Toxicity-A Review, Environ. Sci. Technol., 2016, 50(11), 5438–5453 CrossRef CAS PubMed
. - J. R. Rochester and A. L. Bolden, Bisphenol S and F: A Systematic Review and Comparison of the Hormonal Activity of Bisphenol A Substitutes, Environ. Health Perspect., 2015, 123(7), 643–650 CrossRef CAS PubMed
. - D. Gramec Skledar and L. Peterlin Mašič, Bisphenol A and its analogs: Do their metabolites have endocrine activity?, Environ. Toxicol. Pharmacol., 2016, 47, 182–199 CrossRef CAS PubMed
. - J. Michałowicz, Bisphenol A--sources, toxicity and biotransformation, Environ. Toxicol. Pharmacol., 2014, 37(2), 738–758 CrossRef PubMed
. - C. Liao and K. Kannan, Concentrations and profiles of bisphenol A and other bisphenol analogues in foodstuffs from the United States and their implications for human exposure, J. Agric. Food Chem., 2013, 61(19), 4655–4662 CrossRef CAS PubMed
. - M. E. U. Haq, et al., Human exposure to bisphenol A through dietary sources and development of diabetes mellitus: a cross-sectional study in Pakistani population, Environ. Sci. Pollut. Res. Int., 2020, 27(21), 26262–26275 CrossRef CAS PubMed
. - L. N. Vandenberg, et al., Human exposure to bisphenol A (BPA), Reprod. Toxicol., 2007, 24(2), 139–177 CrossRef CAS PubMed
. - M. S. H. Akash, S. Sabir and K. Rehman, Bisphenol A-induced metabolic disorders: From exposure to mechanism of action, Environ. Toxicol. Pharmacol., 2020, 77, 103373 CrossRef CAS PubMed
. - İ. İyİgÜndoĞdu, A. ÜstÜndaĞ and Y. Duydu, Toxicological Evaluation of Bisphenol A and Its Analogues, Turk. J. Pharm. Sci., 2020, 17(4), 457–462 CrossRef
. - J. R. Rochester, Bisphenol A and human health: a review of the literature, Reprod. Toxicol., 2013, 42, 132–155 CrossRef CAS
. - C. A. Staples, et al., A review of the environmental fate, effects, and exposures of bisphenol A, Chemosphere, 1998, 36(10), 2149–2173 CrossRef CAS PubMed
. - A. Abraham and P. Chakraborty, A review on sources and health impacts of bisphenol A, Rev. Environ. Health, 2020, 35(2), 201–210 CAS
. - A. Usman, S. Ikhlas and M. Ahmad, Occurrence, toxicity and endocrine disrupting potential of Bisphenol-B and Bisphenol-F: A mini-review, Toxicol. Lett., 2019, 312, 222–227 CrossRef CAS
. - Y. Zhang and S. Vyazovkin, Curing of Diglycidyl Ether of Bisphenol P with Nitro Derivatives of Amine Compounds, 2, Macromol. Chem. Phys., 2005, 206(11), 1084–1089 CrossRef CAS
. - L. Y. Cao, et al., Bisphenol AF and Bisphenol B Exert Higher Estrogenic Effects than Bisphenol A via G Protein-Coupled Estrogen Receptor Pathway, Environ. Sci. Technol., 2017, 51(19), 11423–11430 CrossRef CAS
. - H. Wang, et al., Human exposure of bisphenol A and its analogues: understandings from human urinary excretion data and wastewater-based epidemiology, Environ. Sci. Pollut. Res. Int., 2020, 27(3), 3247–3256 CrossRef CAS PubMed
. - L. Zhang, et al., Rapid method for the separation and recovery of endocrine-disrupting compound bisphenol AP from wastewater, Langmuir, 2013, 29(12), 3968–3975 CrossRef CAS
. - X. Xiao, et al., Bisphenol AP is anti-estrogenic and may cause adverse effects at low doses relevant to human exposure, Environ. Pollut., 2018, 242(Pt B), 1625–1632 CrossRef CAS PubMed
. - N. Andújar, et al., Bisphenol A Analogues in Food and Their Hormonal and Obesogenic Effects: A Review, Nutrients, 2019, 11(9), 2136 CrossRef PubMed
. - I. A. Wiraagni, et al., Trace Level Detection of Bisphenol A Analogues and Parabens by LC-MS/MS in Human Plasma from Malaysians, BioMed Res. Int., 2020, 2020, 2581287 Search PubMed
. - H. Luo, et al., Rapid and Sensitive Detection of Bisphenol A Based on Self-Assembly, Micromachines, 2019, 11(1), 41 CrossRef PubMed
. - J. S. Siracusa, et al., Effects of bisphenol A and its analogs on reproductive health: A mini review, Reprod. Toxicol., 2018, 79, 96–123 CrossRef CAS
. - U. Eichenlaub-Ritter and F. Pacchierotti, Bisphenol A Effects on Mammalian Oogenesis and Epigenetic Integrity of Oocytes: A Case Study Exploring Risks of Endocrine Disrupting Chemicals, BioMed Res. Int., 2015, 2015, 698795 Search PubMed
. - F. Gorini, et al., Bisphenols as Environmental Triggers of Thyroid Dysfunction: Clues and Evidence, Int. J. Environ. Res. Public Health, 2020, 17(8), 2654 CrossRef CAS
. - Z. Wehbe, et al., Estrogen and Bisphenol A in Hypertension, Curr. Hypertens. Rep., 2020, 22(3), 23 CrossRef PubMed
. - Y. F. Zhang, et al., Cardiovascular toxicity and mechanism of bisphenol A and emerging risk of bisphenol S, Sci. Total Environ., 2020, 723, 137952 CrossRef CAS PubMed
. - M. Pérez-Bermejo, I. Mas-Pérez and M. T. Murillo-Llorente, The Role of the Bisphenol A in Diabetes and Obesity, Biomedicines, 2021, 9(6), 666 CrossRef PubMed
. - X. Hong, et al., Environmental endocrine disruptor Bisphenol A induces metabolic derailment and obesity via upregulating IL-17A in adipocytes, Environ. Int., 2023, 172, 107759 CrossRef CAS
. - M. E. Cinzori, et al., Ovarian volume partially explains associations of phthalate biomarkers with anti-Müllerian hormone and estradiol in midlife women, Environ. Int., 2023, 172, 107771 CrossRef CAS
. - K. Wang, Z. Zhao and W. Ji, Bisphenol A induces apoptosis, oxidative stress and inflammatory response in colon and liver of mice in a mitochondria-dependent manner, Biomed. Pharmacother., 2019, 117, 109182 CrossRef CAS PubMed
. - N. R. Gassman, Induction of oxidative stress by bisphenol A and its pleiotropic effects, Environ. Mol. Mutagen., 2017, 58(2), 60–71 CrossRef CAS PubMed
. - U. Sur, et al., Oxidative stress markers, trace elements, and endocrine disrupting chemicals in children with Hashimoto's thyroiditis, Toxicol. Mech. Methods, 2019, 29(9), 633–643 CrossRef CAS PubMed
. - D. D. Seachrist, et al., A review of the carcinogenic potential of bisphenol A, Reprod. Toxicol., 2016, 59, 167–182 CrossRef CAS
. - I. L. Steffensen, et al., Bisphenols and Oxidative Stress Biomarkers-Associations Found in Human Studies, Evaluation of Methods Used, and Strengths and Weaknesses of the Biomarkers, Int. J. Environ. Res. Public Health, 2020, 17(10), 3609 CrossRef CAS PubMed
. - L. Trasande, T. M. Attina and H. Trachtman, Bisphenol A exposure is associated with low-grade urinary albumin excretion in children of the United States, Kidney Int., 2013, 83(4), 741–748 CrossRef CAS PubMed
. - H. Iwano, et al., Biotransformation of bisphenol A and its adverse effects on the next generation, in Endocrine Disruptors, 2018 Search PubMed
. - L. Kolatorova, et al., Prenatal exposure to bisphenols and parabens and impacts on human physiology, Physiol. Res., 2017, 66(Suppl 3), S305–S315 CAS
. - Z. Li, et al., Role of TET Dioxygenases and DNA Hydroxymethylation in Bisphenols-Stimulated Proliferation of Breast Cancer Cells, Environ. Health Perspect., 2020, 128(2), 27008 CrossRef CAS PubMed
. - M. Grimaldi, et al., Functional profiling of bisphenols for nuclear receptors, Toxicology, 2019, 420, 39–45 CrossRef CAS PubMed
. - J. P. Buckley, et al., Ultra-processed food consumption and exposure to phthalates and bisphenols in the US National Health and Nutrition Examination Survey, 2013-2014, Environ. Int., 2019, 131, 105057 CrossRef CAS PubMed
. - J. H. Kang, Y. Katayama and F. Kondo, Biodegradation or metabolism of bisphenol A: from microorganisms to mammals, Toxicology, 2006, 217(2–3), 81–90 CrossRef CAS PubMed
. - J. H. Lobos, T. K. Leib and T. M. Su, Biodegradation of bisphenol A and other bisphenols by a gram-negative aerobic bacterium, Appl. Environ. Microbiol., 1992, 58(6), 1823–1831 CrossRef CAS PubMed
. - A. Grelska and M. Noszczyńska, White rot fungi can be a promising tool for removal of bisphenol A, bisphenol S, and nonylphenol from wastewater, Environ. Sci. Pollut. Res. Int., 2020, 27(32), 39958–39976 CrossRef CAS PubMed
. - J. Im and F. E. Löffler, Fate of Bisphenol A in Terrestrial and Aquatic Environments, Environ. Sci. Technol., 2016, 50(16), 8403–8416 CrossRef CAS PubMed
. - Z. Peng, F. Wu and N. Deng, Photodegradation of bisphenol A in simulated lake water containing algae, humic acid and ferric ions, Environ. Pollut., 2006, 144(3), 840–846 CrossRef CAS PubMed
. - I. M. Noureddin, et al., Absorption and metabolism of bisphenol A, a possible endocrine disruptor, in the aquatic edible plant, water convolvulus (Ipomoea aquatica), Biosci., Biotechnol., Biochem., 2004, 68(6), 1398–1402 CrossRef
. - J. R. Rochester, A. L. Bolden and C. F. Kwiatkowski, Prenatal exposure to bisphenol A and hyperactivity in children: a systematic review and meta-analysis, Environ. Int., 2018, 114, 343–356 CrossRef CAS PubMed
. - S. Legeay and S. Faure, Is bisphenol A an environmental obesogen?, Fundam. Clin. Pharmacol., 2017, 31(6), 594–609 CAS
. - R. Meli, et al., Oxidative Stress and BPA Toxicity: An Antioxidant Approach for Male and Female Reproductive Dysfunction, Antioxidants, 2020, 9(5), 405 CrossRef CAS PubMed
. - S. M. Poormoosavi, et al., Protective effects of Asparagus officinalis extract against Bisphenol A- induced toxicity in Wistar rats, Toxicol. Rep., 2018, 5, 427–433 CrossRef CAS PubMed
. - G. J. Alekhya Sita, et al., Protective role of luteolin against bisphenol A-induced renal toxicity through suppressing oxidative stress, inflammation, and upregulating Nrf2/ARE/HO-1 pathway, IUBMB Life, 2019, 71(7), 1041–1047 CAS
. - W. Peerapanyasut, et al., Activation of Sirtuin 3 and Maintenance of Mitochondrial Integrity by N-Acetylcysteine Protects Against Bisphenol A-Induced Kidney and Liver Toxicity in Rats, Int. J. Mol. Sci., 2019, 20(2), 267 CrossRef PubMed
. - J. K. Akintunde, et al., Naringin protects against Bisphenol-A induced oculopathy as implication of cataract in hypertensive rat model, Biomed. Pharmacother., 2020, 126, 110043 CrossRef CAS PubMed
. - M. Shirani, et al., The ameliorative effect of quercetin on bisphenol A-induced toxicity in mitochondria isolated from rats, Environ. Sci. Pollut. Res. Int., 2019, 26(8), 7688–7696 CrossRef CAS PubMed
. - B. Yousaf, et al., Bisphenol A exposure and healing effects of Adiantum capillus-veneris L. plant extract (APE) in bisphenol A-induced reproductive toxicity in albino rats, Environ. Sci. Pollut. Res. Int., 2016, 23(12), 11645–11657 CrossRef CAS PubMed
. - H. Yoshino, et al., Lack of significant alteration in the prostate or testis of F344 rat offspring after transplacental and lactational exposure to bisphenol A, J. Toxicol. Sci., 2002, 27(5), 433–439 CrossRef CAS
. - Y. H. Tian, et al., Prenatal and postnatal exposure to bisphenol A induces anxiolytic behaviors and cognitive deficits in mice, Synapse, 2010, 64(6), 432–439 CrossRef CAS PubMed
. - Y. Toyama, et al., Adverse effects of bisphenol A to spermiogenesis in mice and rats, Arch. Histol. Cytol., 2004, 67(4), 373–381 CrossRef CAS PubMed
. - T. Tachibana, et al., Effects of bisphenol A (BPA) on placentation and survival of the neonates in mice, J. Reprod. Dev., 2007, 53(3), 509–514 CrossRef CAS PubMed
. - A. S. Al-Hiyasat, H. Darmani and A. M. Elbetieha, Effects of bisphenol A on adult male mouse fertility, Eur. J. Oral Sci., 2002, 110(2), 163–167 CrossRef CAS
. - S. Ma, et al., Lycopene reduces in utero bisphenol A exposure-induced mortality, benefits hormones, and development of reproductive organs in offspring mice, Environ. Sci. Pollut. Res. Int., 2018, 25(24), 24041–24051 CrossRef CAS PubMed
. - K. Wang, et al., Environmental contaminant BPA causes intestinal damage by disrupting cellular repair and injury homeostasis in vivo and in vitro, Biomed. Pharmacother., 2021, 137, 111270 CrossRef CAS
. - O. Takahashi and S. Oishi, Testicular toxicity of dietarily or parenterally administered bisphenol A in rats and mice, Food Chem. Toxicol., 2003, 41(7), 1035–1044 CrossRef CAS PubMed
. - K. Kawai, et al., Aggressive behavior and serum testosterone concentration during the maturation process of male mice: the effects of fetal exposure to bisphenol A, Environ. Health Perspect., 2003, 111(2), 175–178 CrossRef CAS PubMed
. - T. Midoro-Horiuti, et al., Maternal bisphenol a exposure promotes the development of experimental asthma in mouse pups, Environ. Health Perspect., 2010, 118(2), 273–277 CrossRef CAS PubMed
. - P. Sánchez, et al., Bisphenol A modifies the regulation exerted by testosterone on 5 α -reductase isozymes in ventral prostate of adult rats, BioMed Res. Int., 2013, 2013, 629235 Search PubMed
.
|
This journal is © The Royal Society of Chemistry 2023 |
Click here to see how this site uses Cookies. View our privacy policy here.