DOI:
10.1039/D3RA04384C
(Paper)
RSC Adv., 2023,
13, 29427-29437
Optimized HPLC extraction method of quercetin and berberine based on response surface analysis
Received
30th June 2023
, Accepted 30th September 2023
First published on 9th October 2023
Abstract
In order to establish a method for simultaneous determination and extraction of quercetin and berberine in soil, HPLC-PDA multi-wavelength method was used to detect the content of berberine and quercetin in soil solution. The detection wavelength was 210 nm and 347 nm. The column temperature was 30 °C, the mobile phase A was acetonitrile, the mobile phase B was 0.1% phosphoric acid aqueous solution, and the flow rate was 1.0 mL min−1. Under the condition of isocratic elution, quercetin and berberine were completely separated within 20 min. The detection limit concentration of quercetin was 0.078 mg L−1, and the detection limit of berberine was 0.019 mg L−1. Both of them reached the trace level, and the recovery rate was between 97.2% and 107.4%. The response surface method was used to optimize the ultrasonic extraction method. The three main factors of extraction concentration, extraction temperature and solid–liquid ratio were optimized to obtain the highest extraction efficiency. The optimum extraction efficiency was as follows: 1 g soil sample was extracted with 80% ethanol aqueous solution, ultrasonic time was 10 min, ultrasonic temperature was 44 °C, and solid–liquid ratio was 1
:
17 g mL−1. The extracted quercetin and berberine concentrations were close to the predicted values of response surface optimization. The method of extracting and determining berberine and quercetin from soil established in this experiment is simple, fast, low cost and high safety. The feedback of the results also further verifies the feasibility in practical production and application, and provides reference value for further research and analysis of different allelochemicals in soil.
1 Introduction
Due to the continuous improvement of intensive planting, continuous cropping obstacles mainly caused by soil-borne diseases and autotoxic effects have appeared in the process of crop planting. One of the main causes is that crops secrete allelochemicals and autotoxic substances in agricultural production, resulting in poor growth and development of crops and serious diseases caused by the deterioration of soil physical and chemical properties.1,2 Allelochemicals, as secondary metabolites of plants, are an important cause of allelopathy of plants in the natural environment. Allelochemicals mainly come from root exudates of plants or decomposition of plant residues.3,4 Rice divided these allelochemicals into 14 categories5, such as unsaturated lactones, water-soluble organic acids, phenolic acids, alkaloids, flavonoids, terpenoids and their derivatives. At present, there are many reports on the autotoxicity of alkaloids and flavonoids.
Alkaloids have complex nitrogen-containing ring structures, which are widely found in dicotyledonous plants such as Tilleraceae, Leguminosae, Solanaceae, Menispermaceae, and Papaveraceae.6 Alkaloids can inhibit plant growth by inserting DNA, affecting enzyme activity, protein synthesis and membrane integrity.7 For example, different parts of tea plants can significantly inhibit seed germination and seedling growth by releasing autotoxic substances such as caffeine.8 Berberine, coptisine, palmatine and jatrorrhizine contained in Rhizoma Coptidis have good inhibitory effects on Microcystis aeruginosa.9 Alkaloids released from barley roots can cause sinapis alba cell wall damage, vacuole volume increase and organelle structure damage.10 After 7 days of berberine treatment, the root growth of Eleusine indica, Cyperus rotundus and Mikania micrantha was significantly inhibited.11 Flavonoids are a class of 2-phenylchromone compounds, which can be secreted into the soil through the roots, thereby inhibiting seed germination.12 Some flavonoids are also present in plant leaves or pollen, and they drop into the surrounding soil and likewise inhibit the germination of themselves and surrounding plants.13 For example, flavonoids such as quercetin and luteolin secreted by long-term continuous cropping leguminous forage plants alfalfa and clover are prone to autotoxicity,14 resulting in inhibition of seed germination and significant decrease in plant yield. Eriocitrin, naringenin and quercetin 3,3-dimethyl ether have inhibitory effects on the growth of lettuce roots.15 These flavonoids reduce the cell division frequency in the root meristem region and inhibit the formation of root hairs and resting cells in root cap cells. 5,7,4′-Trihydroxy-3′,5′-dimethoxyflavone is one of the important allelochemicals in rice, which can effectively inhibit the germination of weed seeds such as barnyardgrass.12,16 Daidzein and genistein could induce the expression of nod gene in Bradyrhizobium, but inhibit the expression of nod gene in Sinorhizobium. Naringenin can stimulate the formation of nodulin in pea rhizobia, while quercetin inhibits its formation.13,17
At present, the detection methods of alkaloids and flavonoids include thin layer chromatography, spectrophotometry, capillary electrophoresis, high performance liquid chromatography etc.18–21 The commonly used extraction methods include impregnation method, ultrasonic extraction method, solvent extraction method, supercritical fluid extraction method, microwave-assisted extraction etc.18,22–24 In terms of detection methods, capillary electrophoresis has low sensitivity and is not suitable for the study of two types of allelochemicals in complex soil environments. Compared with other chromatographic techniques, the separation length of thin layer chromatography is limited, and it can only be used for qualitative analysis, but not for quantitative analysis. High performance liquid chromatography (HPLC) has the characteristics of fast analysis speed, high analysis accuracy, and simultaneous qualitative and quantitative analysis of different components. It is commonly used in the detection and analysis of alkaloids and flavonoids. In this study, high performance liquid chromatography combined with ethanol extraction and ultrasonic extraction technology was used to establish a rapid analysis method for simultaneous detection of two allelochemicals by optimizing chromatographic conditions and extraction conditions, which provided reference value for simultaneous detection of alkaloids and flavonoids.
2 Experimental
2.1 Instruments and reagents
LC-20A High Performance Liquid Chromatograph (Shimadzu, Japan), LC-20 AT infusion unit, SIL-20A automatic sampler, COT-20A column temperature box, SPD-M20A PDA diode array detector; One ten thousandth balance (mettler Toledo); tDL-40B centrifuge (Shanghai Anting Scientific Instrument Factory); kH-5200B ultrasonic cleaner (Kunshan Hechuang Ultrasonic Instrument Co., Ltd).
Methanol, acetonitrile and acetic acid were all chromatographically pure (Germany, Sigma). Quercetin and berberine (Fig. 1), purity greater than 95%, were purchased from Shanghai Yuanye Biotechnology Co, Ltd.; the other reagents were analytically pure, the water used was Waha purified water; the soil samples were taken from the Coptis chinensis planting base in Bozhou City, Anhui Province.
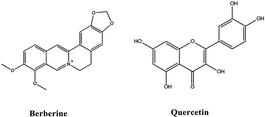 |
| Fig. 1 The structure of berberine and quercetin. | |
2.2 Detection test
2.2.1 Preparation of solutions.
(1) Preparation of single standard stock solution. About 10.0 mg of berberine and quercetin were accurately weighed and transferred to a 10 mL volumetric flask, dissolved with methanol, and the volume was fixed to the scale line. Shake well as a single standard solution.
(2) Preparation of mixing standard solution. The 2.0 mL of berberine and quercetin single standard stock solution was accurately measured and transferred to a 10 mL volumetric flask, and the mobile phase solution with an isocratic elution ratio was used for constant volume as a mixing standard solution.
(3) Preparation of sample solution. 1 g soil sample was placed in a brown wide mouth reagent bottle. After the extraction conditions of ultrasonic temperature 44 °C, ethanol concentration 80%, solid–liquid ratio 1
:
17 g mL−1, the supernatant was separated, filtered by 0.22 μm filter membrane, and stored at 4 °C for analysis.
2.2.2 Chromatographic conditions. Shim-pack VP-ODS column (250 mm × 4.6 mm, 5 μm); the column temperature was 30 °C, and the detection wavelength was 210 nm and 347 nm. The flow rate was 1.0 mL min−1. The injection volume was 10 μL. The mobile phase A was acetonitrile and the mobile phase B was 0.1% phosphoric acid aqueous solution. Isocratic elution: 0 ∼ 20 min, 30% A, 70% B. All solutions were filtered by 0.22 μm membrane before injection.
2.2.3 Instruments and reagents.
(1) Linear relationship investigation. The mixing standard solution was diluted with a pipette, and diluted 2, 6, 8, 10 and 12 times, respectively. Acetonitrile and 0.1% phosphoric acid aqueous solution were used to elute at a ratio of 3
:
7 to constant volume as a linear series solution. The linear series solution was diluted twice step by step, and the limit of quantitation was measured with a 10-fold signal-to-noise ratio (S/N), and the limit of quantitation (LOQ) was used as the lowest concentration in the linear range. The detection was performed according to the 2.2.2 conditions. The linear regression was performed with the concentration as the X axis and the peak area at the maximum absorption wavelength as the Y axis. The regression equations of berberine and quercetin were established and the R2 value was calculated.
(2) Detection limit experiment (LOD). After the lowest concentration solution in the linear series solution was diluted by 2 times, the sample was detected according to 1.4 conditions, and the detection line was measured by 3 times signal-to-noise ratio (S/N).
(3) Specificity experiment. Berberine and quercetin single standard solution, mixed control solution, soil extract and blank solvent solution were injected and detected according to 1.4 conditions. Whether the blank solvent solution interfered with the chromatography of berberine and quercetin was recorded. The relative retention time and maximum absorption wavelength of single standard solution were recorded, and the retention time, tailing factor and resolution of mixed control solution were recorded.
(4) Precision experiment. The mixed control solution was injected continuously for 5 times according to 1.4 conditions, and the relative standard deviation of the peak area of each component was calculated to investigate its precision.
(5) Stability experiment. The mixed control solution was placed at room temperature, and the samples were injected at 0, 2, 4, 6, 8, 16, 24, 34 and 48 h after configuration, respectively. The peak area RSD values of berberine and quercetin were calculated to investigate their stability.
(6) Recovery experiment. Add low medium and high control mixed standard solution to 1 g soil sample (80%, 100%, 120% of the content of each component in the mixed control solution), and three samples were prepared in parallel for each of the three concentrations, and the samples were injected and assayed according to the conditions of item 1.4. The recoveries were calculated by measuring the contents of the two chemically sensitive substances before and after the assay.
2.3 Extract test
2.3.1 Preparation of solutions. The rhizosphere soil of Coptis chinensis was taken, and the obvious stones, grass stems and other impurities were removed. After natural air drying, the 60 mesh sieve was used to screen and reserve.
2.3.2 Extraction test method. 1 g soil sample was placed in a brown wide mouth reagent bottle, and a certain amount of extraction solution was added to ultrasound under different temperature, time and solid–liquid ratio conditions. The supernatant was separated by static separation, filtered by 0.22 μm filter membrane, and stored at 4 °C for analysis.
2.3.3 Single factor test.
(1) Effect of extraction solvent on the concentration of berberine and quercetin. Accurately weigh 1 g of soil samples, select the concentration of the extraction solution is 70%, the ratio of extraction material to liquid is 1
:
10, the extraction ultrasonic time is 20 min, the extraction ultrasonic temperature is 40 °C, the effects of methanol, ethanol and acetonitrile on the concentration of berberine and quercetin were investigated.
(2) Effect of ultrasonic time on the concentration of berberine and quercetin. The concentration of ethanol solution was 70%, the ratio of material to liquid was 1
:
10, and the extraction temperature was 40 °C. The effects of ultrasonic time 10, 20, 30, 40, 50 and 60 min on the concentration of berberine and quercetin were investigated.
(3) The effect of ethanol concentration on the concentration of berberine and quercetin. The 1 g soil sample was accurately weighed, and the extraction ultrasonic time was selected for 20 min. The ratio of material to liquid was 1
:
10, and the extraction ultrasonic temperature was 40 °C. The effects of ethanol concentration of 40, 50, 60, 70, 80, 90, 100% on the concentration of berberine and quercetin were investigated.
(4) Effect of ultrasonic temperature on the concentration of berberine and quercetin. The 1 g soil sample was accurately weighed, the ethanol concentration was 70%, the extraction time was 20 min, and the ratio of material to liquid was 1
:
10. The effects of ultrasonic temperature 40, 50, 60, 70, 80, 90, 100 °C on the concentration of berberine and quercetin were investigated.
(5) Effect of solid–liquid ratio on the concentration of berberine and quercetin. The 1 g soil sample was accurately weighed, and the ethanol concentration was 70%. The extraction ultrasonic time was 20 min, and the extraction ultrasonic temperature was 40 °C. The effects of solid–liquid ratio of 1
:
5, 1
:
10, 1
:
15, 1
:
20, 1
:
25 and 1
:
30 on the concentration of berberine and quercetin were investigate.
2.4 Data processing and statistical analysis
Signals collected from HPLC LabSolutions are output in text document format (*.txt). The original data were plotted with Origin 2018 software. The experimental data of response surface method were imported into Design-Expert 8.0.6 Trial software for drawing.
3 Results and discussion
3.1 Detection test results
3.1.1 Optimization of detection wavelength. In this experiment, SPD-M20A PDA diode array detector was used to scan berberine and quercetin in the full wavelength range of 190 ∼ 800 nm, and the detection wavelength was determined by ultraviolet absorption spectrum. As shown in Fig. 2, according to the maximum absorption wavelength of berberine and quercetin under the chromatographic conditions, considering the difference of sensitivity and anti-interference, 210 nm and 347 nm were selected as the detection wavelengths of berberine and quercetin.
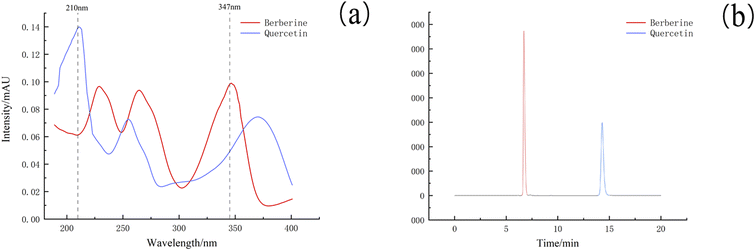 |
| Fig. 2 Spectra (a) and chromatogram (b) of berberine and quercetin standard samples. | |
3.1.2 Optimization of mobile phase composition. The mobile phase has an important influence on the separation process of HPLC. The acetonitrile–water system has a good elution effect in the separation and detection of alkaloids and flavonoids, and the viscosity of acetonitrile is small, which can effectively reduce the system pressure. Studies have shown that in the separation and determination of alkaloids and flavonoids, with an appropriate concentration of formic acid, acetic acid, phosphoric acid aqueous solution as an acid regulator,27–29 can slow down the ionization of phenolic hydroxyl groups and carboxyl groups in aqueous solution, resulting in enhanced polarity, and the surface of the stationary phase forms a double-layer retention, resulting in a serious tailing of the chromatographic peak, After adding a certain acid regulator, the separation and peak shape of the sample were significantly improved.30,31 Through experimental research, it is found that the separation effect of formic acid and acetic acid is not as good as that of phosphoric acid aqueous solution. The final experiment showed that using acetonitrile-0.1% phosphoric acid as the mobile phase, the separation effect of berberine and quercetin was better, and the peak shape symmetry was good, and the tailing factor was between 0.9 and 1.2. When the mobile phase ratio was adjusted several times, when acetonitrile accounted for 30% of the mobile phase, berberine and quercetin could be well separated within 20 minutes.
3.1.3 Optimization of flow rate and column temperature. As shown in Fig. 3, when the flow rate was 0.8 mL min−1, the retention time of berberine and quercetin was significantly delayed, and the peak time of quercetin was at the end of the detection period. When the flow rate was set to 1.2 mL min−1, the retention time of berberine and quercetin could peak earlier than that when the flow rate was 1.0 mL min−1, but the peak time of berberine was similar to that of some miscellaneous peaks, and the column pressure increased significantly, which was close to the critical value of instrument column pressure of 10 MPa, reaching 9.7 MPa.
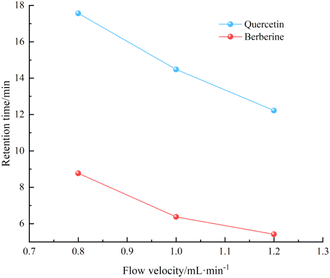 |
| Fig. 3 Effect of flow rate on retention time of berberine and quercetin. | |
In this experiment, the daily average temperature was about 30 °C in summer. When the column temperature was set to 30 °C, the baseline was stable, which was convenient for the calculation of quercetin and berberine.
3.1.4 Analytical method validation. The method follows the guidelines of the International Conference on Harmonization (ICH) 31 and uses standard solutions, blank samples and spiked samples for determination. The linearity, limit of detection and limit of quantitation (LOD and LOQ), precision, accuracy and stability of the HPLC method with diode array.
(1) Linearity and range. The linear results are as shown in Table 2. In a certain concentration range, the mass fraction of berberine and quercetin showed a good linear relationship with the peak area. The R2 of berberine was 0.9991, and the R2 of quercetin was 0.9992. In this paper, the mixed solution also has a good linear relationship after dilution, indicating that berberine and quercetin do not interfere with each other in the mixed solution, thus saving the solution preparation and detection time. The linear detection concentration is broad, which can meet the accurate determination of two allelochemicals in most soil extraction solutions.
Table 2 Relevant data for analytical method validation
Allelochemical |
Retention time (min) |
Separation degrees |
Linear range (mg L−1) |
Regression equation |
Correlation coefficient (R2) |
LOQ (mg L−1) |
LOD (mg L−1) |
Precision |
Stability |
Recovery |
RSD (n = 5, %) |
RSD (n = 8, %) |
Range (%) |
RSD (n = 9, %) |
Quercetin |
14.425 |
23.308 |
0.156 ∼ 51.60 |
y = 54.454x − 696.21 |
0.9992 |
0.156 |
0.078 |
0.28 |
0.59 |
97.2 ∼ 102.3 |
1.95 |
Berberine |
6.31 |
5.837 |
0.03 ∼ 49.90 |
y = 25.146x − 76 |
0.9991 |
0.038 |
0.019 |
0.47 |
0.20 |
99.4 ∼ 107.4 |
2.65 |
(2) Specificity investigation. The results showed that berberine and quercetin could peak in the determination time under the chromatographic conditions, and the peak shape was symmetrical. The solvent peak appeared before 5 min, which had no effect on the peak of berberine and quercetin. The two allelochemicals in the mixed solution could be completely separated, and the separation degree was greater than 1.5, which reached the baseline separation and met the detection conditions. As shown in Fig. 4a, berberine was found near the wavelength of 350 nm. The final test results showed that berberine had the highest absorption intensity at 347 nm, and quercetin had the highest absorption intensity at 210 nm.
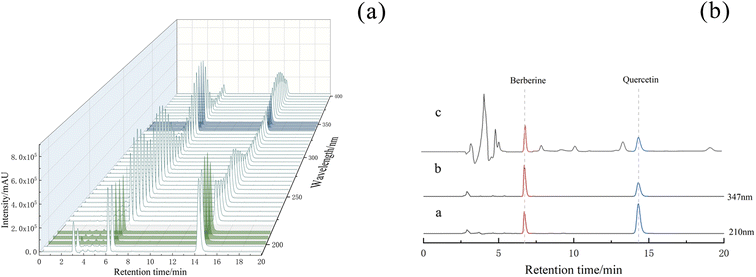 |
| Fig. 4 Full band chromatogram (a), standard sample and soil sample chromatogram (b). *a is the chromatogram of 210 nm standard sample, b is the chromatogram of 347 nm standard sample, c is the chromatogram of soil sample. | |
(3) Detection limit. The experimental results of detection limit are shown in Table 2. Under the chromatographic conditions, the detection limits of berberine and quercetin were between 0.019 mg L−1 and 0.78 mg L−1. The detection limit concentration of alkali reached the trace level, indicating that the method had high sensitivity and was suitable for the quantification of trace berberine and quercetin in soil environment.
(4) Precision. After the same sample was continuously injected for 5 times, the results were as shown in Table 2. The RSD value of the peak area of berberine was 0.47%, and the RSD value of the peak area of quercetin was 0.28%. The precision of the method was good. It shows that the feasibility of simultaneous detection of berberine and quercetin by HPLC-PDA multi-wavelength technology is high.
(5) Solution stability. The stability of the solution is shown in Table 2. The peak area RSD data of berberine and quercetin were less than 2% within 48 h. The mixed solution of phenolic acid was stable at room temperature for 48 h, and no degradation and chemical reaction occurred. The phenolic acid solution prepared by this method can be placed for a long time, and the experimental error caused by long-term placement can be avoided in subsequent applications.
(6) Recovery rate. The recovery results are shown in Table 2. The recovery rates of berberine and quercetin are between 97.2% and 107.4%. The RSD value of berberine is 2.65%, and the RSD value of quercetin is 1.95, indicating that the method has good recovery rate and high accuracy.
(7) Sample determination. The content of berberine and quercetin in soil samples was determined by this method. As shown in Fig. 4b, berberine and quercetin were well separated from the soil extract and were not interfered by other substances.
3.2 Extract the test results
3.2.1 Single factor test results.
(1) Effect of selecting different extraction solvents on extraction. At the beginning of this experiment, acetonitrile, methanol and ethanol were selected as extraction solvents. Among them, acetonitrile had poor extraction effect on two allelochemicals in the preliminary experiment of actual extraction, which may be due to the low solubility of acetonitrile itself to two allelochemicals. Methanol and ethanol have good extraction effect under the condition of suitable extraction temperature. However, considering that methanol is toxic and the use of methanol as extraction solvent will increase more cost investment, which not only cannot guarantee the safety of the experiment, but also affects the selection of other experimental supplies. Therefore, the extraction solvent of the experiment is finally determined as ethanol.
(2) Effect of ultrasound time on ultrasound extraction. It can be seen from Fig. 5a that under the same other conditions, the concentration of berberine increased slowly from 1.968 mg L−1 to 2.395 mg L−1 before 40 min of extraction time within 10 ∼ 60 min, and then the concentration showed a downward trend, and tended to be stable after 50 min, and the concentration was as low as 1.512 mg L−1. The extraction concentration of quercetin has been declining from 5.623 mg L−1 to 2.739 mg L−1, which may be due to the long-term heating effect of excessive exposure to ultrasonic treatment, resulting in changes in the dissolution performance of the extract, resulting in a significant decrease in the extraction concentration.32 Although the extraction concentration of berberine increased steadily, but overall consideration, the consumption of too long time is not in line with the economic benefits, so the extraction time of 10 min is the best.
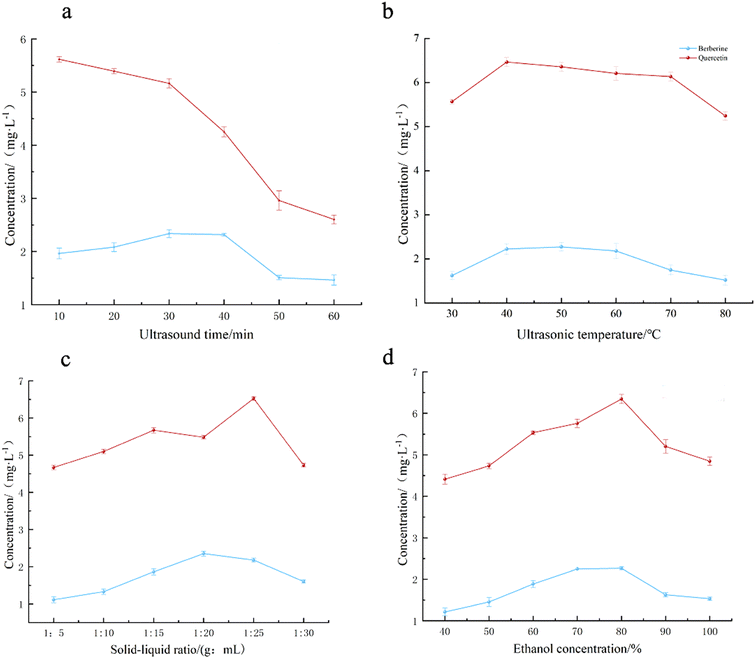 |
| Fig. 5 Effects of ultrasonic time (a), ultrasonic temperature (b), solid-liquid ratio (c) and ethanol concentration (d) on the extraction effect | |
(3) Effect of ultrasonic temperature on ultrasonic extraction. It can be seen from Fig. 5b that when the other conditions were the same, the extraction temperature was in the range of 30–80 °C. Before 50 °C, the concentration of berberine increased from 1.658 mg L−1 to 2.191 mg L−1 with the increase of extraction temperature, while quercetin showed an upward trend before 40 °C, and the concentration increased from 5.534 mg L−1 to 6.307 mg L−1. After that, the extraction concentration of the two allelochemicals showed a downward trend. At 80 °C, the extraction concentration of berberine was reduced to 1.512 mg L−1; the concentration of quercetin decreased to 5.097 mg L−1. The possible reason is that as the temperature increases, the molecular thermal motion gradually increases, making the two types of allelochemicals easier to leach. However, after the temperature gradually increased, the two allelochemicals may be decomposed, resulting in a decrease in concentration detection.33 Considering comprehensively, 40, 50 and 60 °C were selected for optimization test.
(4) Effect of solid–liquid ratio on ultrasonic extraction. According to Fig. 5c, different solid–liquid ratios had a certain effect on the extraction concentration of the two allelochemicals. In the range of 1
:
5–1
:
20 g mL−1 of berberine, quercetin showed an upward trend in the range of 1
:
5–1
:
15 and 1
:
20–1
:
25. The concentration of berberine increased from 1.038 mg L−1 to 2.176 mg L−1, and the concentration of quercetin increased from 4.579 mg L−1 to 6.513 mg L−1, indicating that increasing the amount of extraction solvent to a certain extent was beneficial to the dissolution of the two allelochemicals. In the rest of the interval, with the increase of the dosage of the extraction solvent, the two allelochemicals have a significant downward trend. When the ratio of material to liquid is 1
:
30, berberine decreased to 1.506 mg L−1, quercetin concentration decreased to 4.518 mg L−1. The possible reason is that the substrate is limited, and the ratio of material to liquid is too small, resulting in an increase in water-soluble impurities, which interferes with the detection of the two allelochemicals.34 Among them, the extraction concentration of berberine reached 6.513 mg L−1 when the solid–liquid ratio was 1
:
20, and the maximum concentration of quercetin was 2.176 mg L−1 when the solid–liquid ratio was 1
:
25. Finally, 1
:
15, 1
:
20 and 1
:
25 were selected for optimization test.
(5) Effect of ethanol concentration on ultrasonic extraction. It can be seen from Fig. 5d that when the other conditions are the same, the concentration of extracted ethanol is in the range of 40–100%, and the concentration of berberine and quercetin is in the range of 40–80%. The extraction showed an upward trend, in which the concentration of berberine increased from 1.201 mg L−1 to 2.237, and the concentration of quercetin increased from 4.493 mg L−1 to 6.397 mg L−1. It shows that the extraction efficiency of the two allelochemicals increases with the increase of solution concentration under suitable solvent polarity conditions.33 When the ethanol was 100%, the concentration of berberine decreased to 1.501 mg L−1, and the concentration of quercetin decreased to 6.482 mg L−1. The reason may be that the polarity of the solvent is too high to inhibit the precipitation of allelochemicals in the soil sample. Considering comprehensively, 60, 70 and 80% were selected for optimization test.
3.2.2 Box–Behnken design center combination test results. On the basis of single factor test, the ultrasonic time was fixed for 10 min, and the ultrasonic temperature (A), ethanol concentration (B) and solid–liquid ratio (C) were selected as the influencing factors, and the berberine concentration (Y) and quercetin concentration (Z) were used as the evaluation indexes. The Design-Expert 8.0.6 Trial software was used to design the three-factor and three-level central combination test. The test consisted of 17 groups, with 3 replicates in each group. The factor level is shown in Table 1, and the results are shown in Table 3.
Table 3 Tests and results of response surface method
Serial number |
A/°C |
B/% |
C/(g : mL) |
Y (mg L−1) |
Z (mg L−1) |
1 |
−1 |
−1 |
0 |
2.10 |
5.570 |
2 |
1 |
−1 |
0 |
2.10 |
6.263 |
3 |
−1 |
1 |
0 |
2.19 |
6.550 |
4 |
1 |
1 |
0 |
1.99 |
6.274 |
5 |
−1 |
0 |
−1 |
2.21 |
6.428 |
6 |
1 |
0 |
−1 |
1.94 |
5.667 |
7 |
−1 |
0 |
1 |
1.76 |
5.618 |
8 |
1 |
0 |
1 |
1.81 |
6.719 |
9 |
0 |
−1 |
−1 |
2.13 |
5.886 |
10 |
0 |
1 |
−1 |
2.28 |
6.310 |
11 |
0 |
−1 |
1 |
1.98 |
5.968 |
12 |
0 |
1 |
1 |
1.87 |
6.574 |
13 |
0 |
0 |
0 |
2.31 |
6.517 |
14 |
0 |
0 |
0 |
2.315 |
6.474 |
15 |
0 |
0 |
0 |
2.34 |
6.493 |
16 |
0 |
0 |
0 |
2.33 |
6.432 |
17 |
0 |
0 |
0 |
2.35 |
6.523 |
The regression equation of berberine was Y = 2.23 − 0.056A + 6.250E − 003B − 0.14C − 0.043AB + 0.080AC − 0.065BC − 0.19A2 − 0.053B2 − 0.21C2; the regression equation of quercetin was Y = 6.49 + 0.095A + 0.25B + 0.074C − 0.24AB + 0.47AC + 0.046BC − 0.20A2 − 0.12B2 − 0.18C2.
Variance analysis of quercetin regression equation, the results are shown in Table 4. From Table 4, it can be seen that the first term A, B, C, the interaction term AB, BC and the second term A2, B2, C2 have a very significant effect on the extracted quercetin concentration, and the interaction term AC has a significant effect on the extracted quercetin concentration. It is easy to conclude that the order of factors affecting quercetin is temperature > ethanol concentration > solid–liquid ratio.
Table 4 Variance analysis for the established regression modela
|
Sum of squares |
df |
Mean square |
F-value |
P-value |
Significance |
Note: ** indicates extremely significant difference, * indicates significant difference. |
Modle |
2.145145 |
9 |
0.238349 |
216.5112 |
<0.0001 |
** |
A |
0.071631 |
1 |
0.071631 |
65.06808 |
<0.0001 |
** |
B |
0.510555 |
1 |
0.510555 |
463.7766 |
<0.0001 |
** |
C |
0.043218 |
1 |
0.043218 |
39.25825 |
0.0004 |
** |
AB |
0.23474 |
1 |
0.23474 |
213.2327 |
<0.0001 |
** |
AC |
0.866761 |
1 |
0.866761 |
787.3459 |
<0.0001 |
** |
BC |
0.008281 |
1 |
0.008281 |
7.522271 |
0.0288 |
* |
A2 |
0.168463 |
1 |
0.168463 |
153.0281 |
<0.0001 |
** |
B2 |
0.064246 |
1 |
0.064246 |
58.3596 |
0.0001 |
** |
C2 |
0.13608 |
1 |
0.13608 |
123.6122 |
<0.0001 |
** |
Residual |
0.007706 |
7 |
0.001101 |
|
|
|
Lack of fit |
0.002283 |
3 |
0.000761 |
0.561395 |
0.6685 |
|
Pure error |
0.005423 |
4 |
0.001356 |
|
|
|
Cor total |
2.152851 |
16 |
|
|
|
|
R2 |
|
|
|
|
0.9964 |
|
RAdj2 |
|
|
|
|
0.9918 |
|
RPred2 |
|
|
|
|
0.9791 |
|
C.V.% |
|
|
|
|
0.53 |
|
This model F = 216.5112, P < 0.0001, the response surface regression model reached a very significant level (P < 0.01), the lack of fit F = 0.561395, P = 0.6685 > 0.05, indicating that the lack of fit is not significant, the coefficient of variation was 0.53% (<10%), which indicated that the experimental model could objectively reflect the relationship between various factors and quercetin concentration, and the non-experimental factors had little effect on the extraction results. The model had good experimental stability. The determination coefficient of the regression equation is R2 = 0.9964, indicating that the fitting degree of the equation is good. The regression equation can be used to replace the real point of the test to describe the relationship between the variables and the response value. The corrected coefficient of determination RAdj2 = 0.9918, which is close to R2, shows that the model has sufficient accuracy and versatility. Therefore, this equation can be used to analyze and predict the influence of various factors on the extraction rate in the quercetin extraction experiment.
Analysis of variance of berberine regression equation, the results are shown in Table 5. In the established regression model, F = 370.3793, P < 0.0001, indicating that the difference in the regression model was extremely significant. The lack of fit F = 0.208333, P = 0.8859 > 0.05, the coefficient of variation 0.64% (<10%), indicating that the model prediction is close to the actual situation, the fitting degree is good. The determination coefficient of the regression equation is R2 = 0.9979, which indicates that the test model can fit well with the actual test, and about 99.79% of the results in the actual test can be explained by the fitting model. The corrected determination coefficient RAdj2 = 0.9952, which is close to R2, indicating that the model can explain the change of 99.18% berberine extraction concentration. According to the P value column in Table 5, the extraction temperature (A) and the ratio of material to liquid (C) had a significant effect on the extraction concentration of berberine, and the effect of ethanol concentration (B) was not significant. The effects of AB, AC, BC in the interaction term and A2, B2, C2 in the quadratic term on the extraction concentration of berberine were extremely significant. According to the size of the F value column in Table 5, it can be concluded that the influence degree of the three factors on the concentration of berberine in the extraction is the ratio of material to liquid (C) > extraction temperature (A) > extraction solvent ethanol concentration (C).
Table 5 Variance analysis for the established regression modea
Factors |
Sum of squares |
df |
Mean square |
F-value |
P-value |
Significance |
Note: ** indicates extremely significant difference, * indicates significant difference. |
Modle |
0.616681 |
9 |
0.06852 |
370.3793 |
<0.0001 |
** |
A |
0.025313 |
1 |
0.025313 |
136.8243 |
<0.0001 |
** |
B |
0.000312 |
1 |
0.000312 |
1.689189 |
0.2349 |
|
C |
0.16245 |
1 |
0.16245 |
878.1081 |
<0.0001 |
** |
AB |
0.007225 |
1 |
0.007225 |
39.05405 |
0.0004 |
** |
AC |
0.0256 |
1 |
0.0256 |
138.3784 |
<0.0001 |
** |
BC |
0.0169 |
1 |
0.0169 |
91.35135 |
<0.0001 |
** |
A2 |
0.149213 |
1 |
0.149213 |
806.5562 |
<0.0001 |
** |
B2 |
0.011939 |
1 |
0.011939 |
64.53627 |
<0.0001 |
** |
C2 |
0.187013 |
1 |
0.187013 |
1010.881 |
<0.0001 |
** |
Residual |
0.001295 |
7 |
0.000185 |
|
|
|
Lack of fit |
0.000175 |
3 |
5.83 × 10−5 |
0.208333 |
0.8859 |
|
Pure error |
0.00112 |
4 |
0.00028 |
|
|
|
Cor total |
0.617976 |
16 |
|
|
|
|
R2 |
|
|
|
|
0.9979 |
|
RAdj2 |
|
|
|
|
0.9952 |
|
RPred2 |
|
|
|
|
0.9926 |
|
C.V.% |
|
|
|
|
0.64 |
|
3.2.3 Box–Behnken response surface analysis interaction. Based on the results of regression model analysis of variance, Design-Expert 8.0.6 software was used to draw response surface plots and contour plots based on the regression equation. The effects of ultrasonic temperature, ethanol concentration and solid–liquid ratio on berberine concentration and quercetin concentration were analyzed.When one of the three factors of ultrasonic temperature, ethanol concentration and solid–liquid ratio was fixed, the influence of the interaction of the other two factors on the response value can be expressed by contour lines and response surface diagrams. The results are shown in Fig. 6 and 7. The response surface and contour map can intuitively reflect the effect of interaction on the extraction concentration of the two allelochemicals. The steeper the response surface and the denser the contour, the more significant the effect is. The closer the contour is to the ellipse, the stronger the interaction between the two factors.35,36
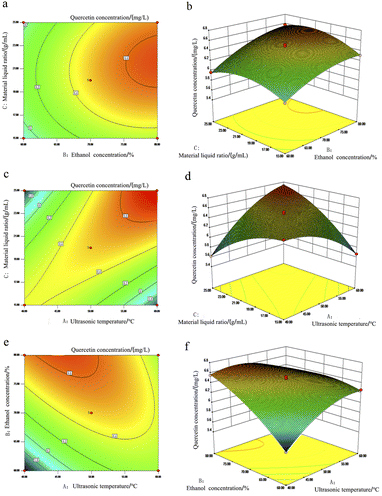 |
| Fig. 6 Three dimensional surface plots (a) and contour plots (b) of ethanol concentration material liquid ratio, three dimensional surface plots (c) and contour plots (d) of ultrasound temperature material liquid ratio, three dimensional surface plots (e) and contour plots (f) of ethanol concentration ultrasound temperature on the interaction of quercetin extraction concentration. | |
It can be seen from the comparison between Fig. 6a and f that the response surface of Fig. 6f is the steepest, and the contour shown in Fig. 6e shows obvious oval shape, P = 0.01 is far less than 0.05, indicating that the interaction between ethanol concentration and ultrasonic temperature has a significant effect on the extraction of quercetin. Secondly, the steepness of the response surface map of Fig. 6d is slightly smaller than that of Fig. 6f but higher than that of Fig. 6b. From the comparison of the contour maps of the two, it can be seen that Fig. 6a is circular as a whole, and the figure is more inclined to ellipse. It can be concluded from the above description that the change of extraction temperature has the most significant effect on the extraction concentration of quercetin, followed by ethanol concentration, and the ratio of material to liquid has the least effect.
From the comparison of Fig. 7a–f, it can be seen that the contour maps of Fig. 7a and e show obvious ellipses, and the contour map of Fig. 7c shows a circle. From the comparison of their respective response surface maps, it can be seen that the slopes of Fig. 7b and f are steeper than those of Fig. 7d, indicating that the solid–liquid ratio and extraction temperature are more significant as influencing factors than ethanol concentration.
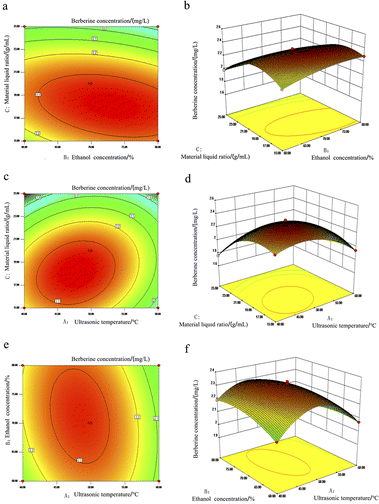 |
| Fig. 7 Three dimensional surface plots (a) and contour plots (b) of ethanol concentration material liquid ratio, three dimensional surface plots (c) and contour plots (d) of ultrasound temperature material liquid ratio, three dimensional surface plots (e) and contour plots (f) of ethanol concentration ultrasound temperature on the interaction of berberine extraction concentration. | |
3.2.4 Optimization process verification experiment. Design-Expert 8.0.0.6 software was used to solve the equation, and the optimal extraction conditions of berberine and quercetin were obtained as follows: ultrasonic temperature 43.84 °C, ethanol concentration 80%, solid–liquid ratio 1
:
17.18 g mL−1. The predicted extraction concentration of quercetin was 6.6693 mg L−1, and the extraction concentration of berberine was 2.34922 mg L−1. Considering the laboratory conditions and the feasibility of actual operation, the extraction conditions were modified to ultrasonic temperature 44 °C, ethanol concentration 80%, solid–liquid ratio 1
:
17 g mL−1. A total of 5 parallel experiments were designed. The average concentration of berberine was 2.27 mg L−1, and the relative deviation was 3.37% (<5%). The average concentration of quercetin was 6.642 mg L−1, and the relative deviation was 0.41% (<5%). It shows that the model is stable, reliable and has practical application value.37
4 Conclusions
In this paper, a rapid and simultaneous determination method of berberine and quercetin was established by HPLC-PDA multi-wavelength method. The response surface method was used to optimize the ultrasonic-assisted extraction technology. The optimized extraction method was similar to the predicted value calculated by model fitting in the detection of berberine and quercetin concentrations in soil samples, which proved that the optimized extraction parameters of the model were stable and reliable. The green organic solvent ethanol aqueous solution used in the study can extract the allelochemicals in the soil well, and the extraction solvent is cheap and easy to obtain, which reduces the experimental cost, and the operation risk is low, and the experiment is relatively safe. In this paper, the extracted berberine and quercetin are less studied and have certain novelty, and the new technical means of fast and complete extraction–detection process are optimized and designed, which has high practicability and high reliability in the production process of medicinal plants, crops and other industries.
Conflicts of interest
There are no conflicts to declare.
Acknowledgements
This work was supported by the Natural Science Foundation of Anhui Province (No. 2008085MC81), Key R&D Program Projects of Anhui Province (No. 202004a06020003), Graduate Science Research Project of Anhui Science and Technology University (No. YK202107) and Enterprise Horizontal Project Fund (No. 2020QX001).
Notes and references
- A. Ratnadass, P. Fernandes, J. Avelino and R. Habib, Agron. Sustainable Dev., 2012, 32, 273–303 CrossRef.
- M. R. Abenavoli, A. Lupini and S. Oliva, Biol. Plant., 2010, 54, 149–153 CrossRef CAS.
- W. Fehrmann, Acta Hortic., 1988, 17–20 CrossRef.
- N. Mahboobi and A. R. Heidarian, J. Fundam. Appl. Sci., 2016, 8, 323–336 CrossRef.
- E. L. Rice, Allelopathy, 2nd edn, 1983 Search PubMed.
- B. B. Buchanan, R. Jones, K. Vickers and W. Gruissem, Biochemistry and molecular biology of plants, 2002 Search PubMed.
- M. T. Gallardo-Williams and D. F. Martin, Chemical Ecology of Plants: Allelopathy in Aquatic and Terrestrial Ecosystems, 2002 Search PubMed.
- Z. Huang, L. Liao, S. Wang and G. Cao, J. Chem. Ecol., 2000, 26, 2211–2219 CrossRef CAS.
- D. Xiang, L. Z. Shu, Z. Bo, D. Wei and Z. X. Ke, Adv. Mater. Res., 2011, 343–344, 1117–1125 CrossRef.
- A. Kotzamani, I. Vasilakoglou, K. Dhima, A. N. Moulas, M. Vaiou and S. Stefanou, J. Plant Growth Regul., 2021, 40, 137–146 CrossRef CAS.
- S. Zhang, B. Zhang, D. Wei and X. Zhang, J. Plant Physiol., 2011, 168, 639–643 CrossRef CAS PubMed.
- K. Saito, K. Yonekura-Sakakibara, R. Nakabayashi, Y. Higashi, M. Yamazaki, T. Tohge and A. R. Fernie, Plant Physiol. Biochem., 2013, 72, 21–34 CrossRef CAS PubMed.
- J. Mierziak, K. Kostyn and A. Kulma, Molecules, 2014, 19, 16240–16265 CrossRef.
- L. A. Weston and U. Mathesius, J. Chem. Ecol., 2013, 39(2), 283–297 CrossRef CAS.
- E. Levizou, P. Karageorgou, Y. Petropoulou, G. Grammatikopoulos and Y. Manetas, Biol. Plant., 2004, 48, 305–307 CrossRef CAS.
- H. Kato-Noguchi, J. Plant Physiol., 2011, 168, 1511–1516 CrossRef CAS PubMed.
- V. V. Lozovaya, A. V. Lygin, O. V. Zernova, S. Li, G. L. Hartman and J. M. Widholm, Plant Physiol. Biochem., 2004, 42, 671–679 CrossRef CAS PubMed.
- I. Ivanov, V. Georgiev, S. Berkov and A. Pavlov, J. Plant Physiol., 2012, 169, 206–211 CrossRef CAS.
- S. J. Kulkarni, K. N. Maske, M. P. Budre and R. P. Mahajan, Int. J. Pharmacol. Pharmaceut. Technol., 2012, 1, 81–84 Search PubMed.
- H. F. Askal, G. A. Saleh and E. Y. T. Backheet, Talanta, 1992, 39, 259–263 CrossRef CAS PubMed.
- S. Joachim, Y. Sheludk, M. Unger, I. Gerasimenko, H. Warzecha and D. Stöckigt, J. Chromatogr. A, 2002, 967, 85–113 CrossRef PubMed.
- J. L. Liu, L. Y. Li and G. H. He, Molecules, 2016, 21(3), 296 CrossRef PubMed.
- M. Bimakr, R. A. Rahman, F. S. Taip, A. Ganjloo, L. M. Salleh, J. Selamat, A. Hamid and I. S. M. Zaidul, Food Bioprod. Process., 2011, 89, 67–72 CrossRef.
- L. Yang, Y. L. Cao, J. G. Jiang, Q. S. Lin, J. Chen and L. Zhu, J. Sep. Sci., 2010, 33.9, 1349–1355 CrossRef.
- D. Manh, C. Jin, J. Abubaker and M. A. Morgan, J. Agric. Food Chem., 2017, 65.33, 7240–7249 Search PubMed.
- J. Pitipanapong, S. Chitprasert, M. Goto, W. Jiratchariyakul, M. Sasaki and A. Shotipruk, Sep. Purif. Technol., 2007, 52, 416–422 CrossRef CAS.
- W. Santos and B. E. A. Magalhaes, An. Acad. Bras. Cienc., 2020, 92, e20190646 CrossRef PubMed.
- E. Valkama, J. P. Salminen, J. Koricheva and K. Pihlaja, Ann. Bot., 2003, 91, 643–655 CrossRef CAS PubMed.
- B. Nian, L. Chen and C. Yi, Electrophoresis, 2019, 40(21), 2837–2844 CrossRef CAS PubMed.
- A. H. Liu, L. Li, M. Xu, Y. H. Lin, H. Z. Guo and D. A. Guo, J. Pharm. Biomed. Anal., 2006, 41, 48–56 CrossRef CAS PubMed.
- L. Zhong, Z. Yuan, L. Rong, Y. Zhang, G. Xiong, Y. Liu and C. Li, Sci. Rep., 2019, 9, 7745 CrossRef PubMed.
- S. Şahin and R. Şamlı, Ultrason. Sonochem., 2013, 20, 595–602 CrossRef PubMed.
- H. Teng and Y. H. Choi, Food Chem., 2014, 142, 299–305 CrossRef CAS PubMed.
- M. Jang, L. Asnin, S. H. Nile, Y. S. Keum, H. Y. Kim and S. W. Park, Int. J. Food Sci. Technol., 2013, 48, 246–252 CrossRef CAS.
- D. Rzabc, L. Tao, Y. Z. D. Zcabc and D. J. C. Yyabc, Appl. Mech. Mater., 2020, 264, 120727 Search PubMed.
- S. R. Omranian, M. O. Hamzah, T. S. Yee and M. R. Mohd Hasan, Int. J. Pavement Eng., 2020, 21.11, 1374–1392 CrossRef.
- D. C. Chen, K. C. Wen and R. S. Yang, Solid State Phenom., 2020, 311, 64–73 Search PubMed.
|
This journal is © The Royal Society of Chemistry 2023 |
Click here to see how this site uses Cookies. View our privacy policy here.