DOI:
10.1039/D3RA04468H
(Paper)
RSC Adv., 2023,
13, 22663-22674
Intense photoluminescence in CaTiO3:Sm3+ phosphors, effect of co-doping singly, doubly and triply ionized elements and their applications in LEDs
Received
4th July 2023
, Accepted 9th July 2023
First published on 26th July 2023
Abstract
In this work, Sm3+-doped and Sm3+/Li+/K+/Mg2+/Ba2+/Gd3+/Bi3+ co-doped CaTiO3 phosphors were synthesized by a solid-state reaction method at 1473 K. The phase of phosphors was identified to be orthorhombic with space group Pnma (62) by XRD measurements. The morphological properties of the prepared samples were studied by SEM measurements. The average crystallite and particle sizes were found to increase in the presence of modifiers and they follow the trend Li+ > Mg2+ > Gd3+ > K+ > Bi3+ > Ba2+. EDX measurements were used to verify the presence of Ca, Ti, O, Sm, K, Mg, Ba, Gd and Bi atoms in the prepared phosphor samples. The Sm3+ ion shows emission peaks at 564, 599 and 646 nm due to 4G5/2 → 6H5/2, 6H7/2 and 6H9/2 transitions upon 407 nm excitation, among which the peak situated at 599 nm has maximum emission intensity. Concentration quenching was observed above 2 mol% of Sm3+ ions in this host. However, the emission intensity of Sm3+ peaks can be enhanced using different modifier (Li+/K+/Mg2+/Ba2+/Gd3+/Bi3+) ions. It was found that the size (ionic radii) and charge compensation of the ion together play a dominant role. The enhancement is more after co-doping with smaller radius ions (Li+, Mg2+ and Gd3+), among which Li+ shows the largest enhancement. This is because ions of smaller size will be able to go closer to the activator ion and the charge imbalance causes a larger field. The CIE color coordinates, correlated color temperature (CCT) and color purity of the phosphors were calculated and show orange-red color emissions with a maximum color purity of ∼93% in the case of CaTiO3:2Sm3+/1.0Li+ phosphor. The lifetime value is increased in the presence of these ions. It is again maximum for the Li+ co-doped CaTiO3:2Sm3+ phosphor sample. Thus, the prepared phosphor samples are suitable sources for orange-red light.
1. Introduction
Rare earth-doped perovskite phosphor materials are chemically and thermally stable and give intense photoluminescence at suitable excitation wavelengths.1–3 These materials are used for various applications such as color tunable devices, display devices, light-emitting devices, plasma display panels, temperature sensors, optical heaters, bio-imaging devices, plant growth and solar cells.4–10 The rare earth ions possess ladder-like energy levels due to which they show multi-modal behaviours such as upconversion (UC), downshifting (DS) and quantum cutting (QC) depending on different excitation detection techniques.11–14
Downshifting is a Stokes emission process, in which a high energy photon is converted into a low energy photon via different relaxation processes. Among the rare earth ions, the Sm3+ ion emits orange-red emissions due to 4G5/2 → 6Hj (j = 5/2, 7/2 and 9/2) transitions under n-UV excitation.15–17 Ha et al. studied the structure and photoluminescence properties of the Sm3+-doped CaTiO3 phosphor and observed intense orange-red emissions due to the 4G5/2 → 6Hj transition under 408 nm excitation.18 Shivaram et al. synthesized Sm3+-doped CaTiO3 by a low-temperature solution combustion method and reported an intense emission peak at 601 nm due to the 4G5/2 → 6H7/2 transition under 407 nm excitation.19 Generally, the orange/red-emitting phosphors show poor luminescence efficiency as compared to green, yellow and blue-emitting phosphors and need to be improved using different sensitizer/modifier ions.20–22 The emission intensity of activator ions may be enhanced in two ways: the first one is by co-doping with surface modifier ions such as Li+, Na+, Sr2+, Ca2+, Ba2+, Mg2+, Bi3+, Gd3+ and the second one is via energy transfer from the sensitizer to activator ions.23–28 Cao et al. observed an enhancement in the emission intensity of Sm3+-doped CaTiO3 phosphors via the addition of Na+ and H3BO3.29 Shanbhag et al. have reported the photoluminescence properties of CaTiO3:Sm3+/Li+.30 Pamuluri et al. have tried to enhance the photoluminescence properties of Sm3+ by energy transfer from Dy3+ to Sm3+ in Dy3+/Sm3+ co-doped Lu3Ga5O12 nano-garnets.31 Zhu et al. also observed an enhancement in the emission intensity of Sm3+ ions via energy transfer from Tb3+ to Sm3+ in Na3Bi(PO)4.32 Dhananjaya et al. have reported the PL properties of Eu3+-doped Gd2O3 phosphors in the presence of alkali ions (M+ = Li+, Na+ and K+).33 They have observed that the PL intensity of phosphors is increased in the presence of alkali ions, which is due to the modification in the local crystal field around the activator ion. In our previous work, we have observed an enhancement in the emission intensity of Eu3+-doped CaTiO3 phosphors in the presence of alkali ions (Li+, Na+ and K+).34 The enhancement in the emission intensity in the presence of alkali ions is due to the modification in crystal field around the activator ion, which increases the average crystallite and particle size. Singh et al. have studied the photoluminescence properties of Eu3+-doped MSiO3 in the presence of alkaline earth ions (M = Mg2+, Ca2+, Sr2+ and Ba2+) and found an intense emission of Eu3+ bands due to the modification in the crystal field around the activator ion.35 Wang et al. reported the enhancement in the luminescence properties of SrIn2O4:Eu3+ phosphors in the presence of Gd3+ ions.36 It is clear from these examples that ions of smaller as well as larger size with single, double and triple ionization state separately have been used to enhance the emission intensity of different rare earth ions in different hosts. All these ions modify the crystal field around the activator ions due to which enhancement in emission intensity is observed. However, from all these studies, it is not clear whether the ionization state or size of the ion or both plays a dominant role in enhancing the photoluminescence emission intensity of the activator ions. Therefore, it will be interesting to study these in detail. The idea is to see whether the ionization state or size of ions and charge compensation behaviors are more effective in enhancing the emission intensity of activators.
In this work, we studied the structural and optical behaviors of Sm3+-doped CaTiO3 phosphors in more detail in the presence of different types of surface modifier ions like singly, doubly and triply ionized elements with smaller and larger ionic radii in a single platform and tried to realize which one is more effective. The Sm3+-doped CaTiO3 and Li+/K+/Mg2+/Ba2+/Gd3+/Bi3+ co-doped CaTiO3:2Sm3+ phosphor materials were prepared by a solid-state reaction method at 1473 K. The XRD measurements showed that phosphor materials have an orthorhombic phase with the Pnma (62) space group. The SEM measurements were carried out to know the effect of different modifier ions on the particle size of the prepared samples. The photoluminescence excitation and emission spectra of the Sm3+-doped CaTiO3 phosphors were studied by taking λem = 599 nm and λex = 407 nm, respectively. The concentration of Sm3+ was optimized for optimum emission. To improve the emission intensity further, Li+/K+/Mg2+/Ba2+/Gd3+/Bi3+ ions (smaller and larger ionic radii of singly, doubly and triply ionized elements) were co-doped in the CaTiO3:2Sm3+ phosphor and their concentrations were varied to obtain the optimum emission. It was found that the enhancement is more in the case of smaller radius ions irrespective of their ionization state and it is optimum for Li+ ions. We also calculated the CIE, CCT and color purity of the Sm3+ ions in the presence of Li+/K+/Mg2+/Ba2+/Gd3+/Bi3+ ions. The value of color purity is larger in the presence of smaller radius (Li+/Mg2+/Gd3+) ions and they follow the trend Li+ > Mg2+ > Gd3+. The lifetime measurements were carried out for the 4G5/2 level of Sm3+ ions in the absence and presence of these modifier ions. It was found that the lifetime of the 4G5/2 level of Sm3+ ions increased in the presence of modifier ions and their order is τLi > τk ∼ τGd > τMg > τBi > τBa.
2. Preparation of phosphor samples and characterization
2.1. Synthesis of materials
The phosphor samples were synthesized by a solid-state reaction technique at 1473 K. The starting materials were calcium carbonate (CaCO3, 99.9%), titanium dioxide (TiO2, 99.9%), samarium oxide (Sm2O3, 99.9%), lithium carbonate (Li2CO3, 99%), potassium carbonate (K2CO3, 99%) magnesium oxide (MgO, 97%), barium carbonate (BaCO3, 99.9%), gadolinium oxide (Gd2O3, 99.9%) and bismuth oxide (Bi2O3, 99.9%). Initially, a series of cSm3+ (c = 1.0, 1.5, 2.0, 2.5, 3.0 and 5.0 mol%) doped CaTiO3 phosphor samples were synthesized to find the Sm3+ concentration for maximum photoluminescence. The Sm3+ ion was found to give optimum emission at 2 mol%. Further, in order to enhance the PL intensity of Sm3+, xLi+ (where x = 0.5, 1.0 and 3.0 mol%), xK+ (where x = 1.0, 3.0, 5.0 and 7.0 mol%), yMg2+ (where y = 1.0, 3.0, 5.0 and 10 mol%), yBa2+ (where y = 3.0, 5.0 and 10 mol%), zGd3+ (where z = 0.1, 0.5 and 0.7 mol%) and zBi3+ (where z = 3.0, 5.0 and 10 mol%) were co-doped in CaTiO3:2 mol% Sm3+ phosphors separately to get the optimum emission of Sm3+ ions in the presence of these ions.
The weighed materials were carefully mixed for one hour in an agate mortar with acetone as a mixing medium. The final mixtures were heated at 1473 K for 4 hours in a programmable electric furnace. The phosphor samples thus obtained were further crushed in an agate mortar to obtain a fine powder for further characterizations.
2.2. Instrumentation
The phase identification of phosphor materials was carried out by XRD measurements using CuKα radiation (λ = 0.15406 nm) with MiniFlex600 (Rigaku, Japan). The morphology of the materials was studied by SEM using a Zeiss, Evo 18 Research system. The Fourier transform infrared measurements were done to know the phonon frequency of phosphor samples using a PerkinElmer I-Frontier system in the 400–3000 cm−1 region. The photoluminescence excitation and emission spectra of the samples were recorded using a Fluorolog-3 spectrophotometer with a 450 W xenon lamp source (Horiba Jobin Yvon). We also measured the lifetime of the 4G5/2 level of Sm3+ ions in the absence and presence of Li+/K+/Mg2+/Ba2+/Gd3+/Bi3+ ions using a 25 W pulsed xenon lamp attached with the same unit (only corresponding to optimized samples).
3. Results and discussion
3.1. Structural characterization
3.1.1. XRD measurements. The powder X-ray diffraction patterns of CaTiO3, CaTiO3:2Sm3+, CaTiO3:2Sm3+/1.0Li+, CaTiO3:2Sm3+/5.0Mg2+, CaTiO3:2Sm3+/0.5Gd3+, CaTiO3:2Sm3+/5.0K+, CaTiO3:2Sm3+/5.0Ba2+ and CaTiO3:2Sm3+/5.0Bi3+ phosphor samples were monitored in the range of 20–80°, 2θ angles, and they are shown in Fig. 1(a)–(h). The phase of the phosphors were identified to be orthorhombic with space group Pnma (62). There were no extra peaks due to substituent ions; however, the XRD peaks show shifts due to substitution. The sharp diffraction peaks also confirm the crystalline nature of the prepared samples. When the lower radius ions (Li+/Mg2+/Gd3+) are co-doped in the CaTiO3:2Sm3+ phosphor at Ca2+ sites, a shifting of peaks in the higher angle side was observed in the diffraction peaks. However, on doping of higher radius ions (K+/Ba2+/Bi3+) at the Ca2+ site, a shifting of peaks in the lower angle side was observed in the diffraction peaks. Upon doping of Sm3+ (0.095) at Ca2+ sites, the diffraction peaks are shifted slightly towards a higher 2θ angle side. These shifts observed in different cases are shown in Fig. 1(i) for intense peak (121) in the range of 32.4 to 33.6°, 2θ angle.
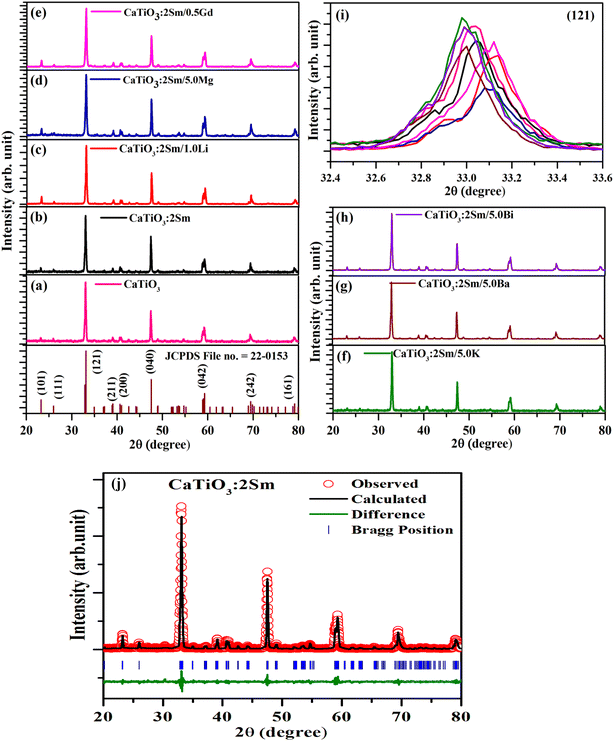 |
| Fig. 1 XRD patterns of (a) CaTiO3, (b) CaTiO3:2Sm3+, (c) CaTiO3:2Sm3+/1.0Li+, (d) CaTiO3:2Sm3+/5.0Mg2+, (e) CaTiO3:2Sm3+/0.5Gd3+, (f) CaTiO3:2Sm3+/5.0K+, (g) CaTiO3:2Sm3+/5.0Ba2+, (h) CaTiO3:2Sm3+/5.0Bi3+ phosphors and (i) shifting in the (121) peak in the range of 32.4 to 33.6°, 2θ angle. (j) Rietveld refinements for the CaTiO3:2Sm3+ phosphor. | |
The ionic radii of Li+, Mg2+ and Gd3+ are 0.076, 0.072 and 0.093 nm, while the ionic radii of Ca2+ is 0.100 nm. Therefore, on substitution of Li+, Mg2+ and Gd3+ ions at the Ca2+ site, the crystal lattice shrinks due to which the XRD peaks are shifted towards a higher 2θ angle side. Wu et al. have also observed the shift in peaks to a higher 2θ angle side on co-doping of Li+ (smaller ionic radii) at the Ca2+ site in CaTiO3:Eu3+ phosphors.37 However, the ionic radii of K+, Ba2+ and Bi3+ are 0.138, 0.135 and 0.103 nm, which are larger than that of Ca2+ ionic radii. Therefore, on substitution of K+, Ba2+ and Bi3+ at the place of Ca2+ the crystal lattice expands due to which the XRD peaks are shifted towards a lower 2θ angle side.
The Rietveld refinements for the CaTiO3:2Sm3+ phosphor sample were carried out, and they are shown in Fig. 1(j). The lattice parameters are a = 5.4420, b = 7.6463, and c = 5.3840, with volume V = 224.0342, and conventional Rietveld parameters were Rp = 14.2, Rwp = 23.7, Rexp = 18.7, and χ2 = 1.59.38
The average crystallite size (D) of the phosphor samples was calculated using the Debye–Scherrer (D–S) equation:39
|
 | (1) |
where
D is the average crystallite size,
k is the shape factor (0.89),
λ is the X-ray wavelength,
β is the full width at half maximum (FWHM) and
θ is the diffraction angle. The average crystallite size values were found to be 30.0, 31.02, 35.51, 33.40, 33.05, 32.80, 31.50 and 32.20, nm for the CaTiO
3, CaTiO
3:2Sm
3+, CaTiO
3:2Sm
3+/1.0Li
+, CaTiO
3:2Sm
3+/5.0Mg
2+, CaTiO
3:2Sm
3+/0.5Gd
3+, CaTiO
3:2Sm
3+/5.0K
+, CaTiO
3:2Sm
3+/5.0Ba
2+ and CaTiO
3:2Sm
3+/5.0Bi
3+ phosphor samples, respectively. This shows that the crystallite size corresponding to Li
+, Mg
2+, and Gd
3+ are slightly larger than that of K
+, Ba
2+, Bi
3+. The dislocation density was used to know the optical efficiency of the phosphor materials. The dislocation density was calculated from the crystallite size using the following relation:
34 |
 | (2) |
The dislocation density values for CaTiO3, CaTiO3:2Sm3+, CaTiO3:2Sm3+/1.0Li+, CaTiO3:2Sm3+/5.0Mg2+, CaTiO3:2Sm3+/0.5Gd3+, CaTiO3:2Sm3+/5.0K+, CaTiO3:2Sm3+/5.0Ba2+ and CaTiO3:2Sm3+/5.0Bi3+ phosphor samples were found to be 11.1 × 10−4, 10.3 × 10−4, 7.9 × 10−4, 8.9 × 10−4, 9.1 × 10−4, 9.3 × 10−4, 10.0 × 10−4 and 9.6 × 10−4 nm−2, respectively. Thus, the dislocation density values were found to decrease and the average crystallite size increase in case of CaTiO3:2Sm3+/1.0Li+, CaTiO3:2Sm3+/5.0Mg2+, CaTiO3:2Sm3+/0.5Gd3+, CaTiO3:2Sm3+/5.0K+, CaTiO3:2Sm3+/5.0Ba2+ and CaTiO3:2Sm3+/5.0Bi3+ phosphors. Therefore, the PL intensity of Sm3+ ions is expected to enhance in the presence of Li+/K+/Mg2+/Ba2+/Gd3+/Bi3+ ions due to the increase in average crystallite size.
3.1.2. SEM and EDX measurements. The surface morphology of the prepared phosphor samples was studied by SEM measurements. Fig. 2(a)–(g) show the SEM images of CaTiO3:2Sm3+, CaTiO3:2Sm3+/1.0Li+, CaTiO3:2Sm3+/5.0K+, CaTiO3:2Sm3+/5.0Mg2+, CaTiO3:2Sm3+/5.0Ba2+, CaTiO3:2Sm3+/0.5Gd3+ and CaTiO3:2Sm3+/5.0Bi3+ phosphor samples, respectively. However, the enlarged picture of a small part of these images in all the cases is shown in the right top corner. In almost all cases, particles are slightly agglomerated to each other and nearly spherical or slightly elongated in shape. It is clear from the enlarged part of the images that the particle sizes are larger in the cases of Li+, Mg2+ and Gd3+ compared to K+, Ba2+ and Bi3+ ions. The average particle size of the prepared phosphors was calculated by the SEM images from the histogram using the ImageJ software and these values were found to be 1.02, 2.19, 1.69, 1.77, 1.24, 1.73 and 1.66 μm for CaTiO3:2Sm3+, CaTiO3:2Sm3+/1.0Li+, CaTiO3:2Sm3+/5.0K+, CaTiO3:2Sm3+/5.0Mg2+, CaTiO3:2Sm3+/5.0Ba2+, CaTiO3:2Sm3+/0.5Gd3+ and CaTiO3:2Sm3+/5.0Bi3+ phosphor samples, respectively [see Fig. 3(a)–(g)]. Thus, the average particles size was found to increase in the presence of all the ions and it is maximum for CaTiO3:2Sm3+/1.0Li+ phosphors. The Li+/K+/Mg2+/Ba2+/Gd3+/Bi3+ ion actually acts as a surface modifier, which modifies the local crystal field around the Sm3+ ions in the CaTiO3 matrix, and therefore, improves the emission intensity in the presence of these modifier ions. It is found maximum in the case of Li+ ions.
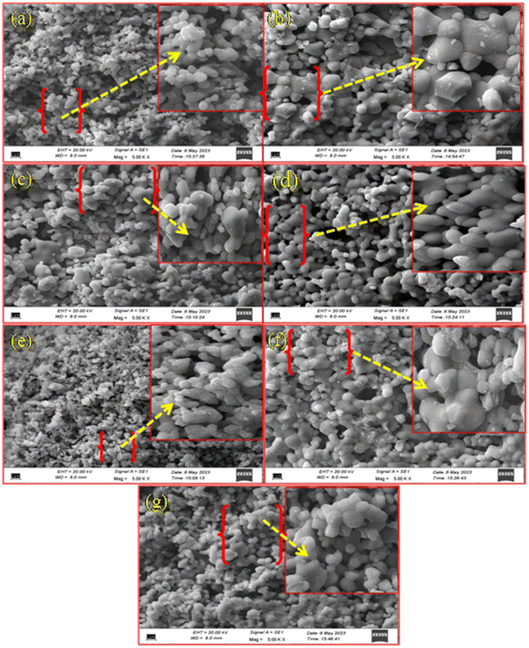 |
| Fig. 2 SEM images of (a) CaTiO3:2Sm3+, (b) CaTiO3:2Sm3+/1.0Li+, (c) CaTiO3:2Sm3+/5.0K+, (d) CaTiO3:2Sm3+/5.0Mg2+, (e) CaTiO3:2Sm3+/5.0Ba2+, (f) CaTiO3:2Sm3+/0.5Gd3+, and (g) CaTiO3:2Sm3+/5.0Bi3+ phosphor samples. | |
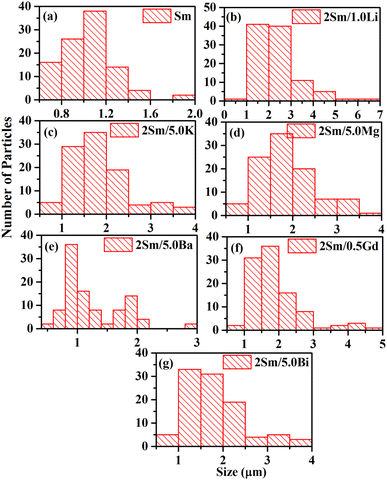 |
| Fig. 3 Particle size distribution of (a) CaTiO3:2Sm3+, (b) CaTiO3:2Sm3+/1.0Li+, (c) CaTiO3:2Sm3+/5.0K+, (d) CaTiO3:2Sm3+/5.0Mg2+, (e) CaTiO3:2Sm3+/5.0Ba2+, (f) CaTiO3:2Sm3+/0.5Gd3+ and (g) CaTiO3:2Sm3+/5.0Bi3+ phosphor samples. | |
Energy-dispersive X-ray spectroscopic (EDX) measurements were used to know the elements present in the prepared phosphor samples. The EDX patterns of CaTiO3:2Sm3+, CaTiO3:2Sm3+/1.0Li+, CaTiO3:2Sm3+/5.0K+, CaTiO3:2Sm3+/5.0Mg2+, CaTiO3:2Sm3+/5.0Ba2+, CaTiO3:2Sm3+/0.5Gd3+ and CaTiO3:2Sm3+/5.0Bi3+ phosphor samples are shown in Fig. 4(a)–(g). The spectra show the presence of Ca, Ti, O, Sm, K, Mg, Ba, Gd and Bi elements in the prepared phosphor samples except the Li element. Because of its very light nature, it was ionized in the SEM chamber during interaction with the incident beam, and therefore, it could not be seen in the spectrum.34 This confirmed that all the elements which were used in the sample preparation are present in the prepared samples.
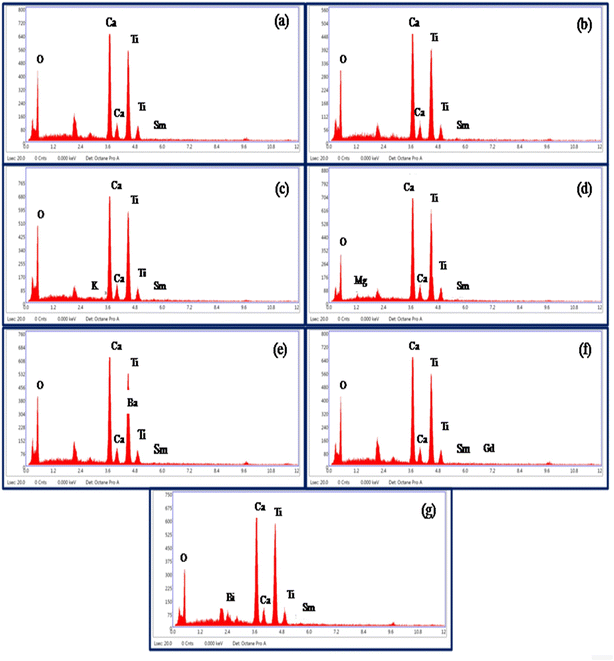 |
| Fig. 4 EDX spectra of (a) CaTiO3:2Sm3+, (b) CaTiO3:2Sm3+/1.0Li+, (c) CaTiO3:2Sm3+/5.0K+, (d) CaTiO3:2Sm3+/5.0Mg2+, (e) CaTiO3:2Sm3+/5.0Ba2+, (f) CaTiO3:2Sm3+/0.5Gd3+ and (g) CaTiO3:2Sm3+/5.0Bi3+ phosphor samples. | |
3.2. Optical characterization
3.2.1. FTIR studies. The Fourier transform infrared measurements were carried out to know the phonon frequencies (vibrational bands frequencies) of the phosphor samples. The FTIR spectra of CaTiO3:2Sm3+ and CaTiO3:2Sm3+/1.0Li+ phosphors in the spectral region 400–3000 cm−1 are shown in Fig. 5. The vibrational bands are observed at 430 and 545 cm−1 due to Ca–O and Ti–O groups.34,39
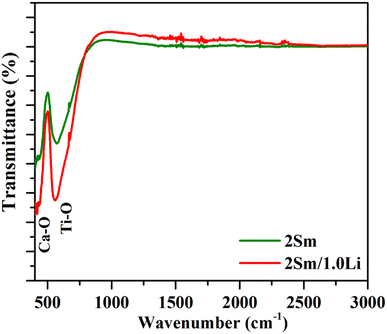 |
| Fig. 5 FTIR spectra of CaTiO3:2Sm3+ and CaTiO3:2Sm3+/1.0Li+ phosphors. | |
The spectra corresponding to modifier ions (Li+) are exactly identical except that there is a change in the intensity of the bands. The following conclusions could be drawn on the basis of these measurements. First, the phonon frequency of the phosphor materials is low, and hence, the non radiative relaxation process in this case will be poor. This means that the radiative emission in this case is expected to be high. Second, the vibrational frequency of the bands remains unchanged upon co-doping of the modifier. Only their intensity is affected due to scattering of photons with the modifier ion.
3.2.2. Photoluminescence excitation spectrum and emission spectra of CaTiO3:cSm3+ phosphors. The photoluminescence excitation spectrum (PLE) of CaTiO3:2Sm3+ phosphor sample was monitored with λem = 599 nm in the 350–525 nm region, as shown in Fig. 6(a). The spectrum of CaTiO3:2Sm3+ phosphor contained a number of peaks, situated at 365, 380, 407, 422, 440, 467 and 481 nm due to 6H5/2 → 4D3/2, 4D1/2, 4F7/2, 4P5/2, 4G9/2, 4I13/2 and 4I11/2 transitions of the Sm3+ ion, respectively.15–17 The peak at 407 nm shows maximum intensity and lies in the region of the n-UV LED chip. Hence, this is important for LED applications.
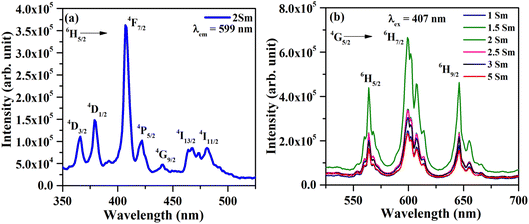 |
| Fig. 6 (a) Photoluminescence excitation (PLE) spectrum of the CaTiO3:2Sm3+ phosphor sample with λem = 599 nm and (b) photoluminescence emission (PL) spectra of CaTiO3:cSm3+ (where c = 1.0, 1.5, 2.0, 2.5, 3.0 and 5.0 mol%) phosphor samples with λex = 407 nm. | |
The photoluminescence emission (PL) spectra of CaTiO3:cSm3+ (where c = 1.0, 1.5, 2.0, 2.5, 3.0 and 5.0 mol%) phosphors with λex = 407 nm in the spectral region 525–700 nm and they are depicted in Fig. 6(b). In the PL spectra, we observed three intense emission peaks at 564, 599, and 646 nm due to 4G5/2 → 6H5/2, 6H7/2 and 6H9/2 transitions of Sm3+ ions.15–17 The most intense peak is situated at 599 nm (4G5/2 → 6H7/2). The peak at 564 nm is due to the magnetic dipole transition. The most intense emission peak at 599 nm appears partially due to magnetic and partially due to forced electric dipole transitions. However, the peak at 646 nm is found due to electric dipole transition.
The Sm3+ ions present in the ground state (6H5/2), are promoted to the 4F7/2 excited state by absorption of 407 nm photons. The Sm3+ ions from 4F7/2 excited state relax non-radiatively to the 4G5/2 excited state, which give multi-transition emission to different sublevels of the ground state, which lie in orange to red regions. The energy level diagram of Sm3+ ions is shown in Fig. 7(a). The photoluminescence emission intensity was found to increase with the concentrations of Sm3+ ions, and it was found optimum at 2 mol% in this host. The emission intensity was found to decrease for higher concentrations due to concentration quenching. The variation in emission intensity with different concentrations of Sm3+ ions is shown in Fig. 7(b). Two mechanisms are generally found to involve in concentration quenching. One is the exchange interaction and the other is the multipolar interaction. The two mechanisms depend on the critical distance between the activator ions. If the value of critical distance between the two ions is ≤5 Å, the concentration quenching would be due to exchange interaction. However, if it is ≥5 Å, it would be due to multipolar interaction.
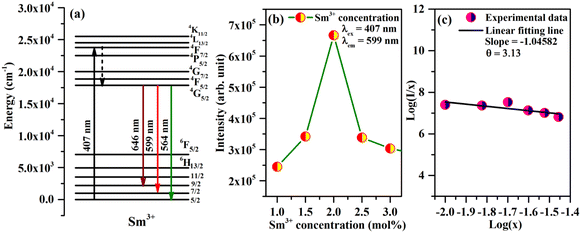 |
| Fig. 7 (a) Schematic energy level diagram of Sm3+ and the process involved in excitation and emission. (b) Variation of emission intensity with the concentration of Sm3+ ions. (c) Dual logarithmic plot between PL emission intensity per Sm3+ ion concentration [Log(I/x)] versus Sm3+ concentration [Log(x)]. | |
The value of critical distance between Sm3+–Sm3+ ions could be calculated using the following equation:34
|
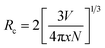 | (3) |
where
V is the volume of unit cell,
x is the optimum concentration of Sm
3+ ions for which the emission intensity is maximum and
N is the number of Ca
2+ sites occupied by Sm
3+ ions. In the present case, the value of
V = 224.0342 Å
3 and
x = 0.02. The number of Ca sites,
i.e. N = 4. This resulted in an
Rc value of 17.44 Å, a value much larger than 5 Å. Hence, the concentration quenching in CaTiO
3:
cSm
3+ phosphors was found to be multipolar interaction.
The multipolar interactions can be recognized using the following formula:34
|
 | (4) |
where
I is the PL emission intensity,
x is the Sm
3+ ion concentration,
k and
β are the constants for a given host. The value of
θ decides the actual nature of interaction between Sm
3+ ions. Depending on the value of
θ equal to 6, 8 or 10, the nature of interaction would be dipole–dipole (d–d), dipole–quadrupole (d–q) or quadrupole–quadrupole (q–q), respectively. A plot between Log(
I/
x)
versus Log(
x) for CaTiO
3:
cSm
3+ (where
c = 1.0, 1.5, 2.0, 2.5, 3.0 and 5.0 mol%) phosphors with
λex = 407 nm and
λem = 599 nm is shown in
Fig. 7(c). The slope value of the curve was found by fitting the plot Log(
I/
x)
versus Log(
x). The observed slope value (equal to −
θ/3) was found to be 1.04582, from which the value of
θ ∼ 3.13, close to 3. If the value of
θ is less than 6, the energy transfer is due to the interaction between the adjacent ions.
3.2.3. Effect of co-doping of singly, doubly and triply ionized ions on the photoluminescence emission intensity of CaTiO3:2Sm3+ phosphor. The PL emission spectra of CaTiO3:2Sm3+/xLi+ (where x = 0.5, 1.0 and 3.0 mol%), xK+ (where x = 1.0, 3.0, 5.0 and 7.0 mol%), yMg2+ (where y = 1.0, 3.0, 5.0 and 10 mol%), yBa2+ (where y = 3.0, 5.0 and 10 mol%), zGd3+ (where z = 0.1, 0.5 and 0.7 mol%) and zBi3+ (where z = 3.0, 5.0 and 10 mol%) phosphors were monitored under excitation at 407 nm, and they are given in Fig. 8(a)–(f). The emission intensity is maximum for 1.0 mol% Li+, 5.0 mol% K+, 5.0 mol% Mg2+, 5.0 mol% Ba2+, 0.5 mol% Gd3+ and 5.0 mol% Bi3+ co-doped CaTiO3:2Sm3+ phosphors. As mentioned earlier, we selected two types of ions: one with a smaller size (Li+/Mg2+/Gd3+ with singly, doubly and triply ionized states) and the other with a larger size (K+/Ba2+/Bi3+ with singly, doubly and triply ionized states), and monitored the photoluminescence emission spectra under the same conditions. It was found that the emission wavelengths of the Sm3+ ion are the same. However, the photoluminescence emission intensity is increased in the presence of all these modifier ions. Moreover, it is larger in the case of ions with smaller ionic radii. The enhancement in the intensity of Sm3+ ions follow the trend ILi+ > IMg2+ > IGd3+ > IK+ > IBi3+ > IBa2+. Several researchers have used these ions to improve the emission intensity of rare earth ions in different host matrices.23–27 For example, Wu et al. have studied the photoluminescence properties of Eu3+/Li+ co-doped CaTiO3 phosphors.37 They found that the emission intensity of Eu3+ ions is increased in the presence of Li+ ions. They have explained it to be the result of the increase in average particle size and charge compensation. Our group have also observed an enhancement in the emission intensity of Tm3+/Yb3+ co-doped ZnWO4 phosphors in the presence of Mg2+ ions due to the modification in the local crystal field.40 Linga et al. have reported an enhancement in the photoluminescence intensity of (Ca1−x−y,Lny)MoO4:xEu3+ (Ln = Y and Gd) phosphors in the presence of Y3+ and Gd3+ ions.41
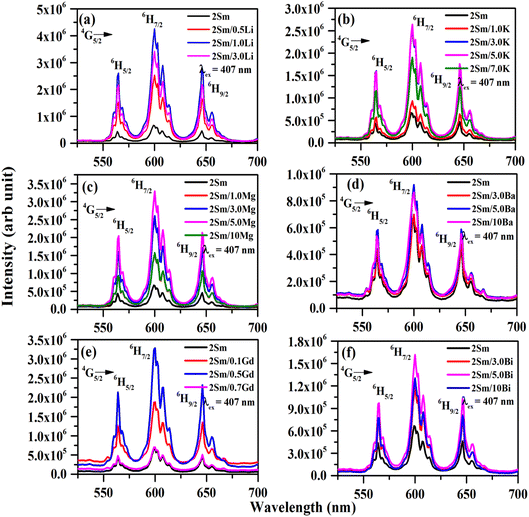 |
| Fig. 8 Photoluminescence emission (PL) spectra of (a) CaTiO3:2Sm3+/xLi+ (where x = 0, 0.5, 1.0 and 3.0 mol%), (b) CaTiO3:2Sm3+/xK+ (where x = 0, 1.0, 3.0, 5.0 and 7.0 mol%), (c) CaTiO3:2Sm3+/yMg2+ (where y = 0, 1.0, 3.0, 5.0 and 10 mol%), (d) CaTiO3:2Sm3+/yBa2+ (where y = 0, 3.0, 5.0 and 10 mol%), (e) CaTiO3:2Sm3+/zGd3+ (where z = 0, 0.1, 0.5 and 0.7 mol%) and (f) CaTiO3:2Sm3+/zBi3+ (where z = 0, 3.0, 5.0 and 10 mol%) phosphor samples with λex = 407 nm. | |
The emission intensity of CaTiO3:2Sm3+/1.0Li+, CaTiO3:2Sm3+/5.0K+, CaTiO3:2Sm3+/5.0Mg2+, CaTiO3:2Sm3+/5.0Ba2+, CaTiO3:2Sm3+/0.5Gd3+ and CaTiO3:2Sm3+/5.0Bi3+ phosphors was enhanced by 6.3, 4.0, 5.1, 1.4, 5.0 and 2.5 times (for 599 nm peak) compared to the CaTiO3:2Sm3+ phosphor.
These observations indicate that the ions with smaller size (ionic radii) are more effective in enhancing the emission intensity of Sm3+ ions. This is due to the reason that ions with a smaller ionic size will be able to reach closer to activator ions. The charge compensation will create a larger field to enhance the emission intensity. In the case of larger radius ions, whatever may be the ionization state (K+, Ba2+, and Bi3+), they will be struck out by the host atoms/ions before they reach closer to the activator ion (i.e. Sm3+) due to their larger size, and hence, the field created will be smaller. Thus, the ionic radii of Li+, Mg2+ and Gd3+ ions are 0.076, 0.072 and 0.093 nm, which are smaller than the Ca2+ ionic radii (0.100 nm) at which these ions are substituted, while the ionic radii of K+, Ba2+ and Bi3+ are 0.138, 0.135 and 0.103 nm, which are larger than the Ca2+ ionic radii. In all these, Li+, Mg2+ and Gd3+ ions produce larger enhancement and in that Li+ is the largest one. Another reason for the enhancement in emission intensity is if we compare the crystallite and particle size, they also follow the same trend, i.e. (Li+) > (Mg2+) > (Gd3+) > (K+) > (Bi3+) > (Ba2+). As larger the particle size, it contains a large number of activators which give large emission intensity. The increase in average crystallite and particle sizes in the presence of Li+/K+/Mg2+/Ba2+/Gd3+/Bi3+ ions improves the population of the 4G5/2 level. Therefore, the emission intensity is enhanced in the presence of Li+/K+/Mg2+/Ba2+/Gd3+/Bi3+ ions.42 The emission intensity is maximum in the case of CaTiO3:2Sm3+/1.0Li+ phosphor and it is due to its smaller ionic radii and used as a charge compensator as well as it has the largest average crystallite and particle size.
3.2.4. Color coordinates, color purity and correlated color temperature calculations. The CIE (Commission Internationale de I'Eclairage) diagram is an excellent tool to verify the color emitted from the phosphors. The values of CIE coordinates were calculated for different samples, and they are shown in Fig. 9 for CaTiO3:2Sm3+ (0.58, 0.42), CaTiO3:2Sm3+/1.0Li+ (0.60, 0.41), CaTiO3:2Sm3+/5.0Mg2+ (0.59, 0.41), CaTiO3:2Sm3+/0.5Gd3+ (0.59, 0.41), CaTiO3:2Sm3+/5.0K+ (0.58, 0.42), CaTiO3:2Sm3+/5.0Ba2+ (0.58, 0.42), and CaTiO3:2Sm3+/5.0Bi3+ (0.58, 0.42), phosphors. The values of CIE coordinates are nearly the same in the presence of different ions and they lie in the orange red region.
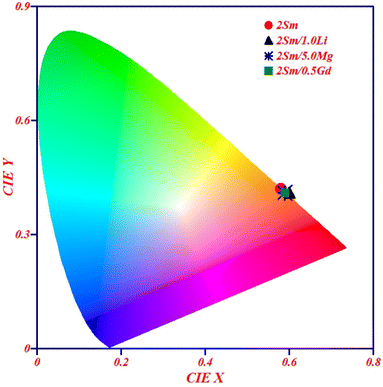 |
| Fig. 9 CIE diagram of CaTiO3:2Sm3+, CaTiO3:2Sm3+/1.0Li+, CaTiO3:2Sm3+/5.0Mg2+ and CaTiO3:2Sm3+/0.5Gd3+ phosphor samples upon 407 nm excitation. | |
The color purity of the phosphor materials is another important parameter to recognize phosphor as a good source of light for a particular color for solid-state lighting applications. The color purity of the light source can be calculated using the following relation:43
|
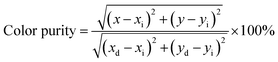 | (5) |
where (
x,
y) are the CIE coordinates of the phosphor, (
xd,
yd) are the CIE coordinates of the dominant wavelength and (
xi,
yi) are the CIE coordinates for the standard light source. The values of color purity in the present case were found to be 87.80% for CaTiO
3:2Sm
3+, CaTiO
3:2Sm
3+/5.0K
+, CaTiO
3:2Sm
3+/5.0Ba
2+ and CaTiO
3:2Sm
3+/5.0Bi
3+ phosphors and 89.88% for CaTiO
3:2Sm
3+/5.0Mg
2+ and CaTiO
3:2Sm
3+/0.5Gd
3+ phosphors. The color purity for the CaTiO
3:2Sm
3+/1.0Li
+ phosphor is 93%. The color purity was also found to improve in the presence of ions of smaller ionic radius (Li
+/Mg
2+/Gd
3+) co-doping and it is maximum for 1.0 mol% Li
+ co-doping in the CaTiO
3:2Sm
3+ phosphor.
We have also calculated the correlated color temperature (CCT) to evaluate the nature of emitted light. The CCT values for the different phosphor samples were evaluated using McCammy's equation as follows:43
|
T = −449(n3) + 3525(n2) − 6823.3(n) + 5520.33
| (6) |
where
n = (
x − 0.3320)/(
y − 0.1858) and (
x,
y) refer to CIE coordinates. The calculated CCT values were found to be 1975 K, for CaTiO
3:2Sm
3+, CaTiO
3:2Sm
3+/5.0K
+, CaTiO
3:2Sm
3+/5.0Ba
2+ and CaTiO
3:2Sm
3+/5.0Bi
3+ phosphors and 1880 K CaTiO
3:2Sm
3+/5.0Mg
2+ and CaTiO
3:2Sm
3+/0.5Gd
3+ phosphors. The CCT value is 1805 K for CaTiO
3:2Sm
3+/1.0Li
+, phosphors. Thus, the CCT values were found to lie in the warm orange-red light region. Therefore, Sm
3+-doped Sm
3+/Li
+/K
+/Mg
2+/Ba
2+/Gd
3+/Bi
3+ co-doped CaTiO
3 phosphor samples were found to be suitable for orange-red lighting devices.
3.2.5. Lifetime measurements. The lifetime of the 4G5/2 level of Sm3+ ions was measured using the 4G5/2 → 6H7/2 transition emitting at 599 nm under 407 nm excitation, and the decay curves are shown in Fig. 10(a)–(g). The decay curves of the 4G5/2 level of Sm3+ ions in different cases were found to fit well using a single exponential relation:38 |
I(t) = I0 exp(−t/τ)
| (7) |
where I0 and I(t) are the PL emission intensities at time zero and t seconds, respectively and ‘τ’ is the lifetime.
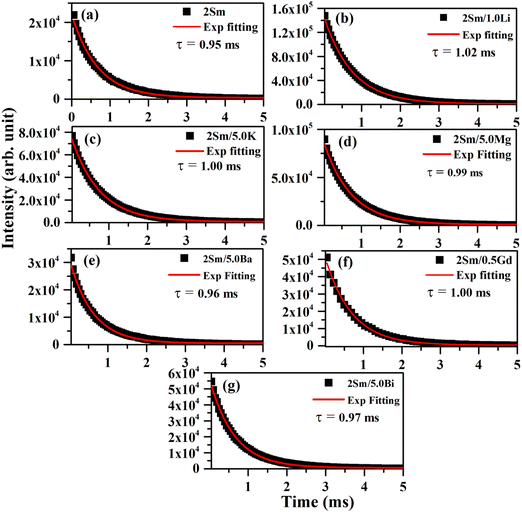 |
| Fig. 10 Decay curves for the 4G5/2 level of Sm3+ ions under λex = 407 nm and λem = 599 nm for (a) CaTiO3:2Sm3+, (b) CaTiO3:2Sm3+/1.0Li+, (c) CaTiO3:2Sm3+/5.0K+, (d) CaTiO3:2Sm3+/5.0Mg2+, (e) CaTiO3:2Sm3+/5.0Ba2+, (f) CaTiO3:2Sm3+/0.5Gd3+ and (g) CaTiO3:2Sm3+/5.0Bi3+ phosphors. | |
The value of lifetime for the 4G5/2 level of Sm3+ in the case of CaTiO3:2Sm3+, CaTiO3:2Sm3+/1.0Li+, CaTiO3:2Sm3+/5.0K+, CaTiO3:2Sm3+/5.0Mg2+, CaTiO3:2Sm3+/5.0Ba2+, CaTiO3:2Sm3+/0.5Gd3+ and CaTiO3:2Sm3+/5.0Bi3+ phosphors were found to be 0.95, 1.02, 1.00, 0.99, 0.96, 1.00 and 0.97 ms, respectively. From this, it is clear that the value of lifetime is increased in the presence of these modifiers (Li+/K+/Mg2+/Ba2+/Gd3+/Bi3+ ions) and it is optimum in the case of Li+ ions. The lifetime of the 4G5/2 level of Sm3+ ions follows the trend, i.e. τLi > τk ∼ τGd > τMg > τBi > τBa. The increase in the average crystallite and particle size and the modification in the crystal field around the activator Sm3+ ion in the presence of Li+/K+/Mg2+/Ba2+/Gd3+/Bi3+ ions improve the population in 4G5/2 levels, and therefore, the value of lifetime of 4G5/2 is increased.42 This might be one of the reasons for enhancement in the PL emission intensity.
4. Conclusions
Sm3+-doped and Sm3+/Li+/K+/Mg2+/Ba2+/Gd3+/Bi3+ co-doped CaTiO3 phosphors have been synthesized by a solid-state reaction method at 1473 K. The structural and morphological properties of the prepared samples were studied by XRD and SEM measurements. The average crystallite and particle sizes were found to increase in the presence of modifiers and they follow the trend Li+ > Mg2+ > Gd3+ > K+ > Bi3+ > Ba2+. EDX measurements were carried out to verify the elements present in the respective phosphor samples. The infrared measurements of the phosphors showed the presence of Ca–O and Ti–O vibrational bands at 430 and 545 cm−1, respectively, indicating that the phosphor has a low phonon frequency. The emission spectra of Sm3+ ions showed an intense emission peak at 599 nm due to 4G5/2 → 6H7/2 transition upon excitation at 407 nm wavelength and the emission intensity is maximum for 2 mol% of Sm3+ ion. The emission intensity is quenched for a higher concentration of Sm3+ ions. Therefore, the surface modifiers were used to further enhance the emission intensity. It was found that co-doping 1.0 mol% Li+, 5.0 mol% K+, 5.0 mol% Mg2+, 5.0 mol% Ba2+, 0.5 mol% Gd3+ and 5.0 mol% Bi3+ ions enhances the emission intensity by 6.3, 4.0, 5.1, 1.4, 5.0 and 2.5 times respectively as compared to the 2Sm3+-doped CaTiO3 phosphor. The increase in emission intensity is due to the modification of the crystal field around the Sm3+ ions in the CaTiO3 host as well as the increase in average crystallite and particle sizes in the presence of these ions. It was found that the size (ionic radii) and charge compensation of the ion together play a dominant role. The enhancement is more after co-doping with smaller radius ions (Li+, Mg2+ and Gd3+), among which Li+ shows the largest enhancement. This is because ions of smaller size will be able to go closer to the activator ion and the charge imbalance causes a larger field. The emission intensity is maximum in the case of the CaTiO3:2Sm3+/1.0Li+ phosphor due to its smaller ionic radii and used as a charge compensator as well as it has the largest average crystallite and particle size. The CIE color coordinates and correlated color temperature (CCT) showed the orange-red color with a color purity as high as 93% in the case of the CaTiO3:2Sm3+/1.0Li+ phosphor. The lifetime of the 4G5/2 level of Sm3+ ions was also found enhanced in the presence of Li+/K+/Mg2+/Ba2+/Gd3+/Bi3+ ions and it follows the trend τLi > τk ∼ τGd > τMg > τBi > τBa. From these studies, it is suggested that Sm3+-doped and Sm3+/Li+/K+/Mg2+/Ba2+/Gd3+/Bi3+ co-doped CaTiO3 samples may be suitable for display devices and for LEDs under n-UV excitation.
Conflicts of interest
This work does not have any conflict of interest.
Acknowledgements
Ms Priti Singh wishes to acknowledge University Grants Commission (UGC), India for providing research fellowship. Authors are also thankful to the Institute of Eminence (IOE), BHU -6031 grant for chemicals.
References
- Y. Pan, Q. Su, H. Xu, T. Chen, W. Ge, C. Yang and M. Wu, Synthesis and red luminescence of Pr3+ doped CaTiO3 nanophosphor from polymer precursor, J. Solid State Chem., 2003, 174, 69–73 CrossRef CAS.
- F. Li, X. Liu and T. He, Solid state synthesis of CaTiO3:Dy3+/Eu3+ phosphors towards white light emission, Chem. Phys. Lett., 2017, 686, 78–82 CrossRef CAS.
- N. Santha, I. N. Jawahar, P. Mohanan and M. T. Sebastian, Microwave dielectric properties of (1-x)CaTiO3-xSm(Mg1/2Ti1/2)O3 [0.1 ≤ x ≤ 1] ceramics, Mater. Lett., 2002, 54, 318–322 CrossRef CAS.
- R. P. Rao and D. J. Devine, RE-activated lanthanide phosphate phosphors for PDP applications, J. Lumin., 2000, 87–89, 1260–1263 CrossRef CAS.
- F. B. Xiong, S. X. Liu, H. F. Lin, X. G. Meng, S. Y. Lian and W. Z. Zhu, A novel white-light emission phosphor Dy3+-doped CaLaB4O13 under UV excitation, Opt. Laser Technol., 2018, 106, 29–33 CrossRef CAS.
- D. M. de. Leeuw, C. A. H. A. Mutsaers, H. Mulder and D. B. M. Klaassen, Blue Emitting Phosphors for Projection Cathode Ray Tubes:(La, Y)OBr:Ce and (La, Gd)OBr:Ce, J. Electrochem. Soc., 1988, 135, 1009 CrossRef.
- A. K. Choudhary, S. K. Singh, A. Dwivedi, A. Bahadur and S. B. Rai, Enhanced upconversion emission of Er3+/Yb3+ and Er3+/Yb3+/Zn2+ doped calcium aluminate for use in optical thermometry and laser induced optical heating, Methods Appl. Fluoresc., 2018, 6, 035014 CrossRef CAS.
- F. Wu, H. Su, X. Zhu, K. Wang, Z. Zhang and W.-K. Wong, Near-Infrared Emissive Lanthanide Hybridized Carbon Quantum Dots for Bioimaging Applications, J. Mater. Chem. B, 2016, 4, 6366 RSC.
- L. F. Johnson and H. Guggenheim, Infrared-Pumped Visible Laser, Appl. Phys. Lett., 1971, 19, 44–47 CrossRef CAS.
- B. M Van Der Ende, L. Aarts and A. Meijerink, Lanthanide ions as spectral converters for solar cells, Phys. Chem. Chem. Phys., 2009, 11, 11081–11095 RSC.
- F. Wang, Y. Han, C. S. Lim, Y. Lu, J. Wang, J. Xu, H. Chen, C. Zhang, M. Hong and X. Liu, Simultaneous phase and size control of upconversion nanocrystals through lanthanide doping, Nat, 2010, 463, 1061–1065 CrossRef CAS PubMed.
- R. S. Yadav, R. K. Verma and S. B. Rai, Intense white light emission in Tm3+/Er3+/Yb3+ co-doped Y2O3-ZnO nano-composite, J. Phys. D: Appl. Phys., 2013, 46, 275101 CrossRef.
- R. S. Yadav, Monika, E. Rai and S. B. Rai, Realizing enhanced downconversion photoluminescence and high color purity in Dy3+ doped MgTiO3 phosphor in presence of Li+ ion, J. Lumin., 2020, 217, 116810 CrossRef CAS.
- R. V. Yadav, R. S. Yadav, A. Bahadur, A. K. Singh and S. B. Rai, Enhanced Quantum Cutting via Li+ Doping from a Bi3+/Yb3+-Codoped Gadolinium Tungstate Phosphor, Inorg. Chem., 2016, 55, 10928–10935 CrossRef CAS PubMed.
- M. Xin, D. Tu, H. Zhu, W. Luo, Z. Liu, P. Huang, R. Li, Y. Cao and X. Chen, Single-Composition White-Emitting NaSrBO3:Ce3+, Sm3+, Tb3+ Phosphors for NUV Light-Emitting Diodes, J. Mater. Chem. C, 2015, 3, 7286–7293 RSC.
- R. Yu, H. Mi Noh, B. K. Moon, B. C. Choi, J. H. Jeong, H. S. Lee, K. Jang and S. S. Yi, Photoluminescence characteristics of Sm3+-doped Ba2CaWO6 as new orange-red emitting phosphors, J. Lumin., 2014, 152, 133–137 CrossRef CAS.
- D. Qingqing, Z. Guangjun, Z. Juan, Z. Haifeng, Z. Jie and Y. Zhongsen, Facile sol-gel combustion synthesis and photoluminescence enhancement of CaZrO3:Sm3+ nanophosphors via Gd3+ doping, J. Rare Earths, 2012, 30, 1000–1004 CrossRef.
- M. G. Ha, M. R. Byeon, T. E. Hong, J. S. Bae, Y. Kim, S. Park, H.-S. Yang and K. S. Hong, Sm3+-doped CaTiO3 phosphor: synthesis, structure, and photoluminescent properties, Ceram. Int., 2012, 38, 1365–1370 CrossRef CAS.
- M. Shivaram, H. Nagabhushana, S. C. Sharma, S. C. Prashantha, B. D. Prasad, N. Dhananjaya, R. H. Krishna, B. M. Nagabhushana, C. Shivakumara and R. P. S. Chakradhar, Synthesis and luminescence properties of Sm3+ doped CaTiO3 nanophosphor for application in white LED under NUV excitation, Spectrochim. Acta, Part A, 2014, 128, 891–901 CrossRef CAS PubMed.
- Z. Jieqiang, F. Yanwei, C. Zhaoyang, W. Junhua, Z. Pengjun and H. Bin, Enhancing the photoluminescence intensity of CaTiO3:Eu3+ red phosphors with magnesium, J. Rare Earths, 2015, 3, 1036–1039 Search PubMed.
- W. L. Feng, M. F. Zhao, J. Y. Xue and X. J. Tian, Photoluminescence properties of (Ba1-xEux)WO4 red synthesized by the coprecipitation/calcination method, J. Alloys Compd., 2012, 521, 146–149 CrossRef CAS.
- Z. Sun, Q. Zhang, Y. Li and H. Wang, Thermal stable La2Ti2O7:Eu3+ phosphors for blue-chip white LEDs with high color rendering index, J. Alloys Compd., 2010, 506, 338–342 CrossRef CAS.
- M. Jayachandiran and S. Masilla Moses Kennedy, Investigation of Alkali metals (A+ = K, Na, Li) co-doped with samarium ions in the eulytite-type phosphate based phosphors for the enhancement of luminescence properties, J. Lumin., 2020, 219, 116951 CrossRef CAS.
- R. Cao, J. Lina, B. Lana, F. Cheng, T. Chena, L. Li, R. Liu and J. Wang, Luminescence properties, tunable emission and energy transfer of Na3Sc2(PO4)3: Sm3+, Bi3+ phosphors, J. Mol. Struct., 2023, 1282, 135221 CrossRef CAS.
- R. Priya and O. P. Pandey, Spectroscopic analysis of alkaline-earth metal (Mg, Ca, Sr and Ba) co-doped Gd2O3:Eu phosphors synthesized via co-precipitation route, Spectrochim. Acta, Part A, 2020, 231, 118078 CrossRef CAS.
- Z. Lu, W. Zhang, J. Chen, S. Chen, J. Cao and H. Guo, Tunable photoemission and energy transfer of heavily Bi3+,Eu3+ co-doped Y4GeO8 phosphors, J. Lumin., 2021, 232, 117857 CrossRef CAS.
- K. N. Kumara, L. Vijayalakshmic and J. S. Kim, Enhanced red luminescence quantum yield from Gd3+/Eu3+: CaLa2ZnO5 phosphor spheres for photonic applications, Mater. Res. Bull., 2018, 103, 234–241 CrossRef.
- Z. Zhu, C. Tao, Z. Wang, Z. Yang and P. Li, Luminescence and energy transfer of warm white-emitting phosphor Mg2Y2Al2Si2O12:Dy3+,Eu3+ for white LEDs, RSC Adv., 2021, 11, 32707–32716 RSC.
- R. Cao, G. Chen, X. Yu, P. Tang, Z. Luo, S. Guo and G. Zheng, Enhanced photoluminescence of CaTiO3:Sm3+ red phosphors by Na+, H3BO3 added, Mater. Chem. Phys., 2016, 171, 222–226 CrossRef CAS.
- V. V. Shanbhag, S. C. Prashantha, H. Nagabhushana, D. M. Jnaneshwara, N. Basavaraju, H. P. Nagaswarup, K. M. Girishe and R. Naik, Mater. Res. Express, 2019, 6, 085037 CrossRef CAS.
- H. Pamuluri, M. Rathaiah, K. Linganna, C. K. Jayasankar, V. Lavınd and V. Venkatramu, Role of Dy3+- Sm3+ energy transfer in the tuning of warm to cold white light emission in Dy3+/Sm3+ co-doped Lu3Ga5O12 nano-garnets, New J. Chem., 2018, 42, 1260–1270 RSC.
- Z. Zhu, G. Fu, Y. Yang, Z. Yang and P. Li, Energy transfer, tunable luminescence, and thermal stability of Tb3+-Sm3+-codoped Na3Bi(PO4)2 phosphors, J. Mater. Sci., 2016, 51, 6944–6954 CrossRef CAS.
- N. Dhananjaya, H. Nagabhushana, B. M. Nagabhushana, B. Rudraswamy, C. Shivakumara, K. Narahari and R. P. S. Chakradhar, Enhanced photoluminescence of Gd2O3:Eu3+ nanophosphors with alkali (M = Li+, Na+, K+) metal ion co-doping, Spectrochim. Acta, Part A, 2012, 86, 8–14 CrossRef CAS PubMed.
- P. Singh, R. S. Yadav and S. B. Rai, Enhanced photoluminescence in a Eu3+ doped CaTiO3 perovskite phosphor via incorporation of alkali ions for white LEDs, J. Phys. Chem. Solids, 2021, 151, 109916 CrossRef CAS.
- D. Singh, S. Sheoran, V. Tanwar and S. Bhagwan, Optical characteristics of Eu(III) doped MSiO3 (M = Mg, Ca, Sr and Ba) nanomaterials for white light emitting applications, J. Mater. Sci.: Mater. Electron., 2017, 28, 3243–3253 CrossRef CAS.
- H. Wang and L. Tian, Luminescence properties of SrIn2O4:Eu3+ incorporated with Gd3+ or Sm3+ ions, J. Alloys Compd., 2011, 509, 2659–2662 CrossRef CAS.
- Y. F. Wu, Y. T. Nien, Y. J. Wang and I. G. Chen, Enhancement of photoluminescence and color purity of CaTiO3:Eu phosphor by Li doping, J. Am. Ceram. Soc., 2011, 95, 1360–1366 CrossRef.
- P. Singh, H. Mishra, P. C. Pandey and S. B. Rai, Structure, photoluminescence properties and energy transfer phenomenon in Sm3+/Eu3+ co-doped CaTiO3 phosphors, New J. Chem., 2023, 47, 1460–1471 RSC.
- P. Singh, R. S. Yadav, P. Singh and S. B. Rai, Upconversion and downshifting emissions of Ho3+-Yb3+ co-doped ATiO3 perovskite phosphors with temperature sensing properties in Ho3+-Yb3+ codoped BaTiO3 phosphor, J. Alloys Compd., 2021, 855, 157452 CrossRef CAS.
- R. S. Yadav, S. J. Dhoble and S. B. Rai, Enhanced photoluminescence in Tm3+, Yb3+, Mg2+ tri-doped ZnWO4 phosphor: three photon upconversion, laser induced optical heating and temperature sensing, Sens. Actuators, B, 2018, 273, 1425–1434 CrossRef CAS.
- Y. Yu-Linga, L. Xue-Minga, F. Wen-Lina, L. Wu-Lina and T. Chuan-Yi, Co-precipitation synthesis and photoluminescence properties of (Ca1-x-y,Lny)MoO4: xEu3+ (Ln = Y, Gd) red phosphors, J. Alloys Compd., 2010, 505, 239–242 CrossRef.
- Monika, R. S. Yadav, A. Bahadur and S. B. Rai, Concentration and pump power-mediated color tunability, optical heating and temperature sensing via TCLs of red emission in an Er3+/Yb3+/Li+ codoped ZnGa2O4 phosphor, RSC Adv., 2019, 9, 40092–40108 RSC.
- P. Singh, H. Mishra and S. B. Rai, Excitation wavelength dependent color tunability, white light generation and enhanced optical emission in mixed phase ZnO–CAZO:xEu3+ over single phase ZnO:xEu3+ phosphors: a comparative study, Phys. Scr., 2023, 98, 035402 CrossRef.
|
This journal is © The Royal Society of Chemistry 2023 |
Click here to see how this site uses Cookies. View our privacy policy here.