DOI:
10.1039/D3RA05912J
(Paper)
RSC Adv., 2023,
13, 28288-28298
Novel epoxy-terminated macromonomers and their polymerization for synthesis of bottle-brush type amphiphilic block copolymers†
Received
30th August 2023
, Accepted 21st September 2023
First published on 26th September 2023
Abstract
Architecture of polymers has vital implications for their physical properties and applications. In this study, synthesis of a series of novel epoxy-terminated macromonomers namely Ep-DEGMME, Ep-TEGMME, Ep-EGMEE, Ep-EGMBE, and Ep-EGMHE is reported. The synthesized macromonomers vary in number of ethylene oxide units and length of the alkyl group. These macromonomers are first homopolymerized by anionic ring-opening polymerization for synthesis of homopolymers of a molar mass range. Subsequently, these macromonomers with different lengths of two segments (alkyl group and ethylene oxide units) are copolymerized with other monomers for synthesis of bottle-brush type architectures. In the first case, di- and tri-block copolymers of Ep-EGMBE are synthesized while using MeO-PEG or PEG as a macroinitiator; the resulting block copolymers have hydrophilic handle and hydrophobic brush. On the same lines, block copolymers of Ep-TEGMME with ε-caprolactone have hydrophobic handle and hydrophilic brush. The synthesized block copolymers are comprehensively characterized by SEC and liquid chromatography at critical conditions. The analysis reveals the successful synthesis of block copolymers while providing information on relative total molar mass, and individual block lengths of the block copolymers, along with amount of unwanted homopolymers in the sample.
1. Introduction
Polymers can have different architectures, such as linear, branched, grafts, star, cross-linked, brushes, and so forth.1 Among various reported architectures of polymers, brushes are a promising class with special properties, for instance, rapid conformational changes under influence of external stimuli, such as pH, temperature, ionic strength, heat or light. Owing to low hydrodynamic radii of the branched polymers, their physical properties, both bulk and solution, are very different from similar molar masses of their linear analogues.2–4 Recently, an increased interest in polymer brushes has emerged in various fields such as tissue engineering, drug delivery, sensing, lubrication, etc.5–10 The performance properties of any material not only depend upon the parent constituents but also on their surfaces. Physical properties that are directly associated with the surfaces of materials include friction, wettability, adhesion, colloidal stability, lubrication, and biocompatibility.9,11–13
Polymer brushes are usually synthesized by means of physisorption or covalent attachment. Physisorption methodology often suffers from the solvent and thermal instability unlike covalent attachment. Covalent attachment is mainly accomplished through “grafting from” or “grafting to’’ methods.14,15 In “grafting to” approach, polymer bearing reactive end-functionality is synthesized that subsequently reacts with a complementary surface reactive group of the backbone. The major disadvantage of this strategy is low grafting density of the synthesized polymer brushes. The “grafting from’’ technique has developed as a versatile approach for the synthesis of high grafting density polymer brushes. This method involves an in situ polymerization of surface immobilized initiator layer with a monomer, and provides reliability, versatility and controllability. It can be employed with a variety of polymerization methods such as reversible addition fragmentation chain transfer (RAFT) polymerization, ring-opening polymerization (ROP), atom transfer radical polymerization (ATRP), and nitroxide-mediated polymerization (NMP).8,13,16–19 In this regard, ROP is a favorable method for synthesis of polymer brushes by using epoxides as a functional monomer.20 Epoxides have several advantages compared to other monomers, such as ease of accessibility, comparatively simple synthesis of monomers, possibility of different functional groups on the epoxy ring, and high ring-strain of the three membered cyclic ether.21,22
Glycidyl ethers have an epoxide ring that may be attached with different groups having distinct polar character. Their ROP subsequently produce polymer brushes with different pendant groups that lead to peculiar physical and chemical properties.23 Homopolymers of glycidyl ethers with different compositions of the pendant groups should have quite different thermal and morphological properties. Block copolymers of these epoxy-terminated macromonomers with other monomers such as ethylene oxide, lactones, higher epoxide etc. shall induce amphiphilicity resulting in synthesis of amphiphilic block copolymers. Controlled delivery of hydrophobic drugs by their improved solubilization and stabilization through amphiphilic block copolymers is one of the hot research domains of modern day science.24 A well-defined nano-sized, core/shell type architectures called micelles are formed by amphiphilic block copolymers in solvents having preferential solvation for one block compared to the other block. For instance, in water the hydrophilic segment self-assembles to make protective shell over the core of the hydrophobic block. This hydrophilic corona protected hydrophobic core of the micelles serves as a reservoir for transport of hydrophobic drugs to the target site.25,26 There are several variations that can directly impact the performance of amphiphilic block copolymers, such as overall molar mass, relative block length, and chemical composition.27–32
Anionic ROP of cyclic monomers is a feasible method of polymerization of monomers having an epoxide ring, such as glycidyl ethers, by employing metal salts of potassium, sodium, and cesium that generate alkoxide species by deprotonation of a hydroxy bearing compound. The generated alkoxide group ultimately attacks carbon atom of oxirane to effectively open the ring and creates another oxyanion for propagation of polymerization.33–38
Industrial surfactants and emulsifiers are generally low molar mass polymers synthesized by ring-opening polymerization of cyclic monomers. Ring-opening of epoxide is, by far, the most widely used approach in this context for their synthesis. Some of the important industrial products in this context are fatty acid ethoxylates, polysorbate ethoxylates, and poloxamers (EO–PO block copolymers) etc.39
In this study, we report synthesis of novel epoxy-terminated macromonomers containing different segments of varying polarity such as alkyl group of varying length and different number of ethylene oxide (EO) units. These groups are introduced by reaction of different glycol ethers namely DEGMME, TEGMME, EGMEE, EGMBE, and EGMHE with epichlorohydrin. The synthesized macromonomers are named as epoxy-terminated DEGMME, epoxy-terminated TEGMME, epoxy-terminated EGMEE, epoxy-terminated EGMBE, and epoxy-terminated EGMHE. The success of the synthesis of macromonomers is evaluated by 1H-NMR. The synthesized macromonomers are polymerized by anionic ROP using diethylene glycol (DEG) as an initiator and sodium hydride as a catalyst. Homopolymers are characterized by size exclusion chromatography, and 1H-NMR. Finally, di- and tri-block copolymers are synthesized by using PEG and MeO-PEG as a macro-initiator while using Ep-EGMBE as a macromonomer. These block copolymers have a bottle-brush type structure having hydrophilic handle with hydrophobic brush. Tri-block copolymer synthesized by using PEG as macroinitiator have a dumbbell type structure with hydrophilic shaft in the center and bulky side groups. On the same lines, sequential anionic polymerization of Ep-TEGMME and ε-caprolactone resulted in bottle-brush type architecture with hydrophobic handle and hydrophilic brush, Fig. 1. As typical examples, we focus on TEGMME and EGMBE for synthesis of block copolymers. Size exclusion chromatography is employed for relative molar mass analysis of the block copolymers. Moreover, liquid chromatography at critical conditions is employed at chromatographic critical point (CCP) of PCL and P(Ep-TEGMME) for determination of unwanted critical homopolymers in the sample along with individual block length of the non-critical block in the block copolymer.
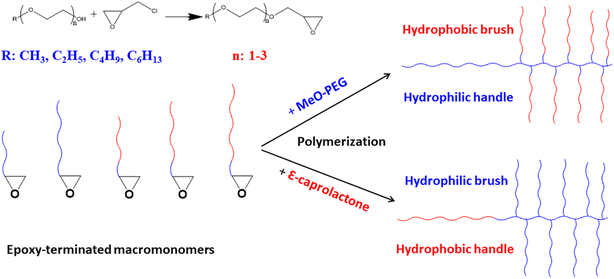 |
| Fig. 1 Variety of epoxy-terminated macromonomers and their polymerization for synthesis of bottle-brush type architectures. | |
2. Experimental section
2.1. Materials
Diethylene glycol (DEG, Scharlau), sodium hydride (NaH, Alfa Aesar), sodium hydroxide (NaOH, Merck), and epichlorohydrin (ECH) (Daejung) were used without further purification. Diethylene glycol monomethyl ether (DEGMME, Daejung), triethylene glycol monomethyl ether (TEGMME, Daejung), ethylene glycol monoethyl ether (EGMEE, Daejung), ethylene glycol monobutyl ether (EGMBE, Daejung), ethylene glycol monohexyl ether (EGMHE, Leap Chem) were used as received. Polyethylene glycol (PEG400 Sigma-Aldrich, Germany), methoxy-polyethylene glycol (MeO-PEG550, Sigma-Aldrich, Germany) were dried by azeotropic distillation over toluene prior to use. ε-Caprolactone (ε-CL, TCI, Japan) was dried over CaH2 and distilled under reduced pressure. HPLC grade chloroform, acetone, and acetonitrile were purchased from Daejung, Korea.
2.2. Synthesis of epoxy-terminated macro-monomer
Glycol ethers (DEGMME, TEGMME, EGMEE, EGMBE, and EGMHE) and NaOH were placed in a three-neck round-bottom flask equipped with a bubbler, a thermometer, and a dropping funnel. The mixture was stirred to dissolve NaH. After a pre-decided time-interval, an excess amount of epichlorohydrin (ECH) was added drop-wise. The temperature in the reaction flask was kept below 40 °C by putting flask in cold water. After the addition of whole ECH stirring continued for two hours. Thereafter, the product mixture was dissolved in acetonitrile followed by filtration under vacuum to remove excess NaOH and NaCl. The solvent (CH3CN) and unreacted ECH were removed on rotary evaporator under reduced pressure. The reaction of the synthesis of epoxy-terminated macromonomer is demonstrated in Scheme 1. The synthesized macromonomers vary in the length of EO block in a range of 1–3 units and the alkyl group (CH3, C2H5, C4H9, and C6H13) depending upon the used glycol ether. Several different macromonomers are successfully synthesized namely epoxy-terminated DEGMME, epoxy-terminated TEGMME, epoxy-terminated EGMEE, epoxy-terminated DGMBE, and epoxy-terminated EGMHE.
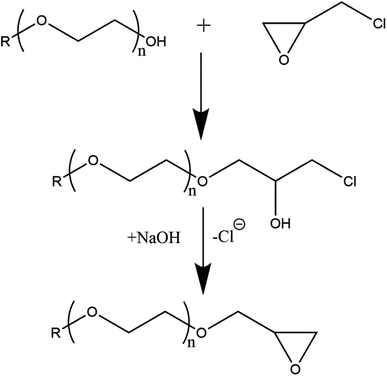 |
| Scheme 1 Synthesis of epoxy terminated macromonomers; R: CH3, C2H5, C4H9, C6H13, and n: 1–3. | |
2.3. Polymerizations
The synthesis of homopolymers by anionic ring-opening polymerization was conducted in a round bottom flask containing DEG as an initiator and sodium hydride as a catalyst along with respective epoxy-terminated macromonomer. For instance, calculated amounts of DEG, NaH, and Ep-TEGMME or Ep-EGMBE were taken in flask that is subjected to three evacuation and nitrogen purging cycles followed by heating to 200 °C for synthesis of homopolymer brushes with different target molar masses. The amounts of the reagents used in the synthesis of different target molar masses of homopolymers are given in Table S1-ESI Material.† After polymerization, active chain-end was neutralized by adding equimolar amount of acetic acid.
First series of block copolymers was synthesized by anionic ROP of Ep-EGMBE using MeO-PEG550 and PEG400 as a macroinitiator and NaH as a catalyst. Calculated amounts of the reagents were added in a round bottom flask that was subjected to three evacuation and nitrogen purging cycles. All the polymerizations were carried out at 200 °C in an inert atmosphere. After, polymerization, active chain-end was neutralized by adding equimolar amount of acetic acid. The mass ratio of the macroinitiators and epoxy-terminated macromonomers was varied as per target length of the hydrophobic block while keeping same length of hydrophilic block in di- and tri-block copolymers, Table S2-ESI Material.†
For second series of block copolymers, different molar masses of the first block were prepared in a range of 500–3000 g mol−1 by varying ratios of initiator and monomer as shown in Table S1-ESI Material.† In this case, TEGMME was used as initiator. P(Ep-TEGMME)2K was selected for polymerization of ε-caprolactone. The synthesized P(Ep-TEGMME)2K serves as a macroinitiator and initial ratios of the macroinitiator and ε-caprolactone (monomer) were varied in order to have different block length of the hydrophobic polycaprolactone while having same length of P(Ep-TEGMME), Table S3-ESI Material.† After completion of polymerization of Ep-TEGMME, the temperature of flask was reduced to 140 °C and calculated amount of ε-caprolactone was added. Three different block copolymers were synthesized having same length of the hydrophilic P(Ep-TEGMME) block (2000 g mol−1) and different length of the PCL block. After, polymerization, active chain end was neutralized by adding equimolar amount of acetic acid. The products are named as P(Ep-TEGMME)2K-b-PCL (3–1), P(Ep-TEGMME)2K-b-PCL (1–1), P(Ep-TEGMME)2K-b-PCL (1–3), where number in parenthesis represent the initial mass ratio of the macro-initiator [P(Epoxy-TEGMME)2K] and the monomer (ε-caprolactone).
2.4. Size exclusion chromatography (SEC)
An Agilent SEC instrument (infinity 1200 series), equipped with an isocratic pump, a manual injector, a column oven and a RI detector, was used for SEC analysis. A set of three PLgel columns (300 × 7.5 mm, 5 μm, Mixed D) connected in front with a PLgel guard column (50 × 7.5 mm) were placed in a column oven maintained at 30 °C. Chloroform (HPLC grade) was used as an eluent at a flow rate of 1.0 mL min−1. The SEC system was calibrated with narrow molar mass polystyrene standards (ranging from 165 g mol−1 to 3 × 106 g mol−1) procured from Polymer Standard Services (Germany). Therefore, all the molar mass values obtained by SEC analysis are relative to linear PS. The data was processed by Agilent OpenLAB ChemStation. Sample concentrations were kept between 3.0 and 5.0 mg mL−1, and 100 μL of sample solution was injected.
2.5. Nuclear magnetic resonance spectroscopy (NMR)
1H-NMR spectra of epoxy-terminated macromonomers and homopolymer were recorded using Bruker AVANCE AN-500 MHz spectrometer. Tetramethylsilane was used as an internal standard and analytes were dissolved in CDCl3. TopSpin 3.6.1 software was used for data processing.
2.6. Liquid chromatography at critical conditions (LCCC)
Liquid chromatographic analyses at critical conditions were performed at an Agilent technologies chromatograph (infinity 1200 series). The system is equipped with a binary pump, a column oven, an auto-sampler, and an evaporative light scattering detector. The column oven was maintained at 30 °C. The temperature of ELSD was set at 40 °C and nitrogen was used as carrier gas at a pressure of 50 psi. The mobile phase was delivered at 0.5 mL min−1. Sample concentrations were kept between 3.0 and 10.0 mg mL−1, and 20 μL of sample solution was injected. The data were processed by Agilent Open LAB ChemStation for LC system. Following columns were used in this study.
• ZORBAX ODS, pore size 70 Å, particle size 5 mm, and column dimensions 250 × 4.6 mm (Phenomenex, USA).
• Ultisil diol column, pore size 120 Å, particle size 5 mm, and column dimensions 250 × 4.6 mm (Welch, China).
HPLC grade acetone, acetonitrile, and double deionized water were used for preparation of mobile phases. Solvents were mixed by volume and vacuum degassed.
3. Results & discussion
Macromonomers with epoxide ring attached to different groups varying in number of EO repeat units and length of alkyl chains induce peculiar properties to their homo- and block copolymers. First target in this study was the development of a method for synthesis of macromonomers that allows to tailor their structure for different lengths of the variable segments.
3.1. Epoxidation of glycol ethers
Epoxide ring is introduced to a series of glycol ethers namely DEGMME, TEGMME, EGMEE, EGMBE, and EGMHE through their reaction with epichlorohydrin in order to synthesize a novel library of macromonomers, Scheme 1. 1H-NMR spectroscopy is employed for the confirmation of formation of epoxy-terminated macromonomer. As a typical example, 1H-NMR spectrum of Ep-TEGMME is presented in Fig. 2. The peaks in a range of 2.5–3.2 ppm (peaks d, e, and f) correspond to the protons of the epoxide ring. It is pertinent to mention that protons “e” and “f” have same chemical environment but they are showing different chemical shifts because they are diastereotopic protons and have different chiral environment. A peak at 3.3 and 3.7 ppm corresponds to methyl proton (a) and protons of three EO repeat units (b), respectively. The low intensity peak at 3.6 ppm corresponds to the proton attached to the epoxide group. Hence, all the peaks are justified and indicate successful synthesis of Ep-terminated macromonomers. It is pertinent to mention here that yields in all cases were above 90%.
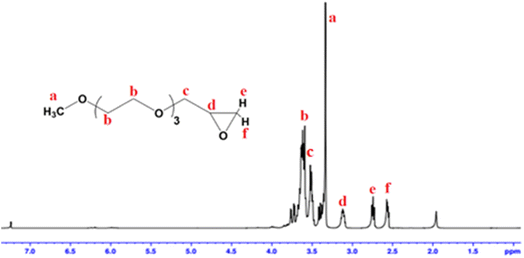 |
| Fig. 2 1H-NMR spectrum of Ep-TEGMME. | |
3.2. Homopolymers of epoxy-terminated macromonomers
Polymerization of the synthesized macromonomers is conducted to make homopolymers having peculiar properties. As mentioned earlier sodium ion generate alkoxide species by deprotonation terminal hydroxyl groups of DEG or any other compound containing terminal hydroxyl group. The generated alkoxide group ultimately attacks carbon atom of oxirane to effectively open the ring and creates another oxyanion for propagation of polymerization.33–38 A general scheme of polymerization of the synthesized epoxy-terminated macromonomers is presented in Scheme 2. In the scheme R and n correspond to the alkyl group and number of ethylene oxide units in the macromonomer while m refers to the degree of polymerization. Generally, ROP is employed for synthesis of low molar mass polymers and achieving very high molar masses is practically not possible. Size exclusion chromatography (SEC), the primary technique for analysis of molar mass and its distributions, is employed for analysis of the synthesized polymers.40,41 Different target molar masses of homopolymers are synthesized by varying the molar feed ratio of monomer-to-initiator (M/I). SEC elugrams of three products of P(Ep-TEGMME) of different target molar masses, i.e., 1000 g mol−1 (1 K), 2000 g mol−1 (2 K), and 3000 g mol−1 (3 K) are shown in Fig. 3A. Separation in SEC is based on the hydrodynamic size of the polymer molecules in dilute solution, the elution volumes are subsequently compared with polymer standards of known molar mass in order to obtain relative molar mass of the analyzed polymers. Retention volumes of the synthesized polymers decrease with increase in the molar mass as per expectation based on the initial monomer-to-initiator ratio. Molar mass data of the homopolymers as obtained by SEC using PS calibration is given in Table S4, ESI Material.† Moreover, 1H-NMR spectroscopy confirmed the synthesis of homopolymers through disappearance of signals around 2.6, 2.8, and 3.2 ppm that are attributed to the protons of the oxirane ring, Fig. 3B.
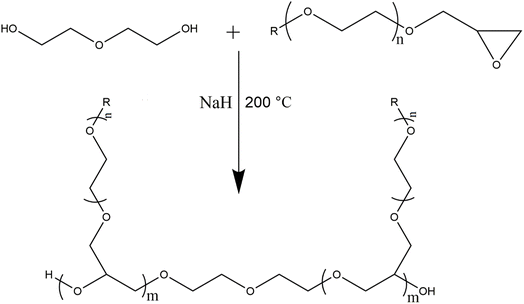 |
| Scheme 2 General reaction scheme of polymerization of epoxy-terminated macromonomers where R: CH3, C2H5, C4H9, C6H13, n: 1–3, and m: degree of polymerization. | |
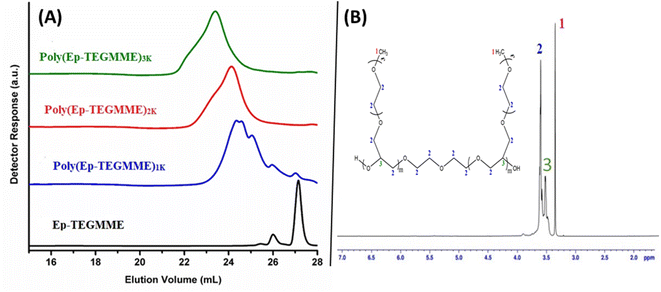 |
| Fig. 3 P(Ep-TEGMME) (A) SEC chromatograms of homopolymers with different target molar masses on a set of three PLgel columns (300 × 7.5 mm, 5 μm, Mixed D) and PLgel guard column (50 × 7.5 mm) in CHCl3 at flow rate of 1.0 mL min−1; (B) 1H-NMR spectrum of P(Ep-TEGMME)2K. | |
Different experimental parameters such as amount of catalyst, monomer-to-initiator ratio, and polymerization temperature are optimized. At the optimized conditions, the augmentation in the molar mass as a function of time at various temperatures for P(Ep-TEGMME) is presented in Fig. 4. The molar mass of the P(Ep-TEGMME) increased with polymerization times at different rates while polymerizing at different temperatures. A reasonable propagation in the molar mass is achieved for reaction at 200 °C. Same polymerization temperature is found to be rational for synthesis of P(Ep-EGMBE).
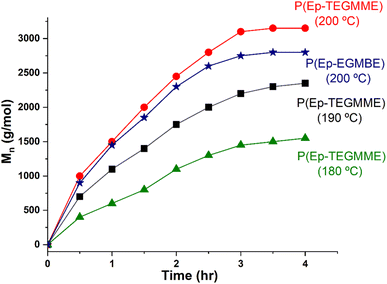 |
| Fig. 4 Propagation of molar mass as a function of time at different polymerization temperatures. | |
3.3. Block copolymers of epoxy-terminated macromonomers
Sequential polymerization of epoxy-terminated macromonomers with other monomers produces block copolymers with unique architectures. Herein, we synthesized two types of block copolymers rendering two unique bottle-brush type architectures. The first type has hydrophilic handle and hydrophobic brush while the second type has hydrophobic handle and hydrophilic brush.
3.3.1. Block copolymers having hydrophilic handle and hydrophobic brush. In the first series of block copolymers, PEG400 and MeO-PEG550 are used as macroinitiator while polymerizing Ep-EGMBE. Di- and tri-block copolymers are synthesized having a bottle-brush like architecture with hydrophilic handle and hydrophobic brush. The reaction scheme for synthesis of diblock copolymer initiated with MeO-PEG550 is presented in Scheme 3. Triblock copolymers synthesized by using PEG400 would have similar reaction scheme as presented for homopolymer (Scheme 2). The only difference would be higher number of ethylene oxide units in the center. The synthesized triblock copolymers have a dumbbell type architecture. Synthesized di- and tri-block copolymers of Ep-EGMBE are characterized by size exclusion chromatography (SEC) for augmentation in molar mass. SEC elugrams of di- and tri-block copolymers having different ratios of monomer-to-macroinitiator are demonstrated in Fig. 5. As can be noticed, elution volume of the block copolymers decreased with increase in the monomer (EGMBE) content in the initial feed. This is an expected behavior and indicates the formation of the block copolymers. Molar mass averages and dispersity (Đ) of the synthesized di- and tri-block copolymers using PS calibration curve are listed in Tables S5 and S6-ESI Material.†
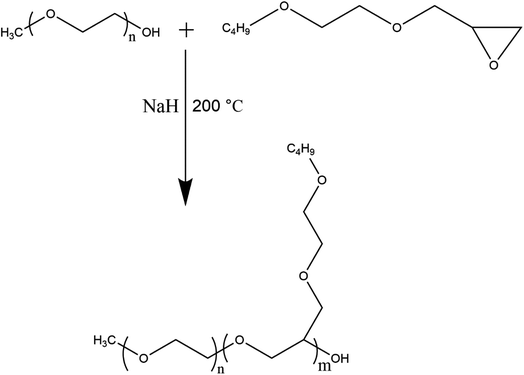 |
| Scheme 3 Reaction scheme for synthesis of MeO-PEG550-b-P(Ep-EGMBE). | |
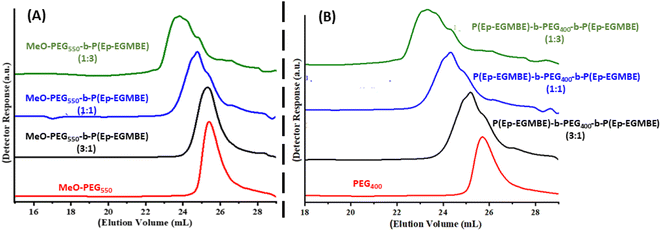 |
| Fig. 5 Size exclusion chromatography on a set of three PLgel columns (300 × 7.5 mm, 5 μm, Mixed D) and PLgel guard column (50 × 7.5 mm) in chloroform (CHCl3) at flow rate of 1.0 mL min−1, (A) MeO-PEG550-b-P(Ep-EGMBE), (B) P(Ep-EGMBE)-b-PEG400-b-P(Ep-EGMBE); numbers in parenthesis represent initial feed ratios of macro-initiator and monomer in mass. | |
3.3.2. Block copolymers having hydrophobic handle and hydrophilic brush. The second series of block copolymer with bottle-brush like architecture have hydrophobic handle and hydrophilic brush, synthesized through sequential addition of monomers. Epoxy-terminated triethylene glycol monomethyl ether (Ep-TEGMME) is first polymerized to achieve different molar masses in a range of 500–3000 g mol−1 at the optimized polymerization conditions using TEGMME as an initiator. Different molar masses of P(Ep-TEGMME) are used for establishment of the chromatographic critical point (CCP) of P(Ep-TEGMME). P(Ep-TEGMME)2K is selected as a macroinitiator for synthesis of its block copolymer with ε-caprolactone, Scheme 4. The mass ratios of macroinitiator and ε-caprolactone are varied systematically to have different block lengths of the hydrophobic PCL block attached to the same length of the hydrophilic block [P(Ep-TEGMME)2K]. SEC elution volume of the block copolymers decreased systematically compared to macroinitiator with an increase in the ε-caprolactone content of the initial feed, Fig. 6. SEC elution order was P(Ep-TEGMME)2K-b-PCL (1–3), P(Ep-TEGMME)2K-b-PCL (1–1), and P(Ep-TEGMME)2K-b-PCL (3–1) followed by P(Ep-TEGMME)2K (the macroinitiator), as expected. Molar mass averages and dispersity (Đ) of the synthesized P(Ep-TEGMME)2K-b-PCL using PS calibration curve are listed in Table S7-ESI Material.†
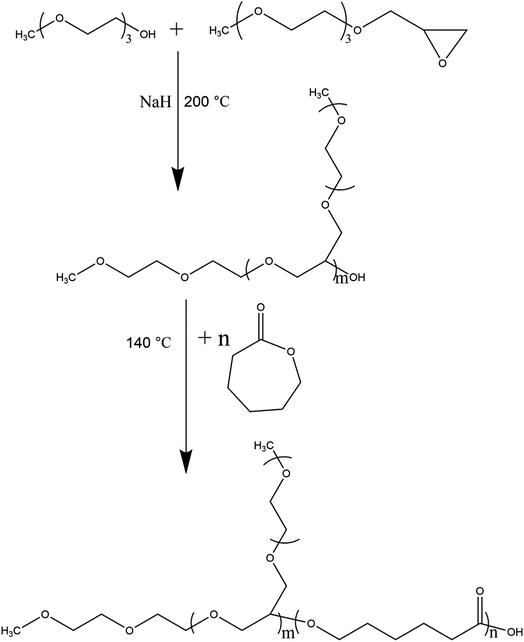 |
| Scheme 4 Reaction scheme for synthesis of P(Ep-TEGMME)2K-b-PCL by sequential addition of monomers. | |
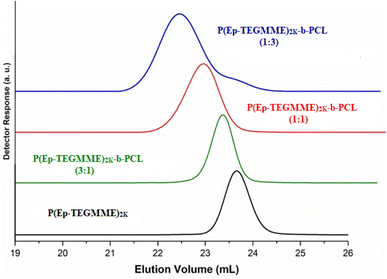 |
| Fig. 6 Size exclusion chromatography of P(Ep-TEGMME)2K-b-PCL on a set of three PLgel columns (300 × 7.5 mm, 5 μm, Mixed D) and PLgel guard column (50 × 7.5 mm) in chloroform (CHCl3) at flow rate of 1.0 mL min−1; numbers in parenthesis represent initial feed ratios of macro-initiator and monomer in mass. | |
The next relevant question for any block copolymer is the connectivity of two blocks to each other and the individual block lengths. Moreover, determination of presence/absence of any unwanted homopolymers in the sample is imperative which can impede the final application properties. Liquid chromatography at critical conditions (LCCC) is literally the only technique that can reveal this important information.36,42–51 The approach requires establishment of chromatographic critical point (CCP) of each block that allows exclusion of the non-critical block.52–54
In this context, CCP of PCL is established on a RP ODS column in a mobile phase (MP) constituting ACN and THF. In the ACN/THF MP, 1% of triethylamine (TEA) is added in order to avoid unwanted polar interactions of the polar groups in the polymer chains with free silanol groups of the stationary phase (SP).46 The eluotropic strength of THF is higher on RP columns compared to ACN. In a MP composition of ACN/THF/TEA: 75/25/01 (V%), PCL eluted in order of decreasing molar mass, a typical SEC regime (Fig. 7A). A decrease of THF content to 19% resulted in a shift of elution volumes to higher value, however, elution order remained the same. An additional decrease in the THF content of MP to 15% not only shifted elution volume further but also reversed the elution order, PCL eluted in order of increasing molar mass in a typical IC regime. There must be a MP composition between two opposing elution orders where all PCL should elute as a sharp peak near the void volume of the column. This is indeed the case in a MP composition of ACN/THF/TEA: 82/17/01 (V%), so-called CCP of PCL on RP column under current conditions. Under these conditions, P(Ep-TEGMME) eluted in order of decreasing molar mass earlier than the void volume of the column, Fig. 7B. Hence, the elution volume of P(Ep-TEGMME)2K-b-PCL represents the block length of P(Ep-TEGMME) with minimal effect of the PCL block. Furthermore, PCL homopolymers get separated from the rest of the sample at its CCP, Fig. 8. Same macroinitiator P(Ep-TEGMME)2K is used for synthesis of block copolymers, therefore, elution of block copolymer under conditions of LCCC-PCL is not affected and all three block copolymers under study have the similar elution volume indicating the same length of P(Ep-TEGMME) block. However, the content of unwanted PCL homopolymers has increased with the increase in the ε-caprolactone content of the initial feed. The increase in the initial monomer content (ε-caprolactone) will effectively increase the length of PCL block of block copolymer whose effect is nullified at its CCP. Hence, analysis at LCCC-PCL reveal similar length of P(Ep-TEGMME) block and an increase in the PCL homopolymer content of the products with higher monomer ratio in the initial feed. It is pertinent to mention that free P(Ep-TEGMME) coelute with the block copolymers and analysis at LCCC-P(Ep-TEGMME) is required for its determination.
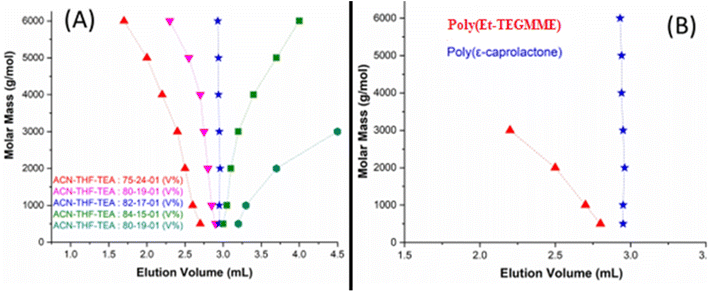 |
| Fig. 7 (A) Elution behavior of different molar masses of PCL as a function of the MP composition (ACN/THF/TEA); (B) elution behavior of P(Ep-TEGMME) at the CCP of PCL: mobile phase: ACN/THF/TEA: 82/17/01(V%), column: ZORBAX ODS, pore size 70 Å, particle size 5 mm, and column dimensions 250 × 4.6 mm (Phenomenex, USA), detector: ELS, column temperature: 30 °C. | |
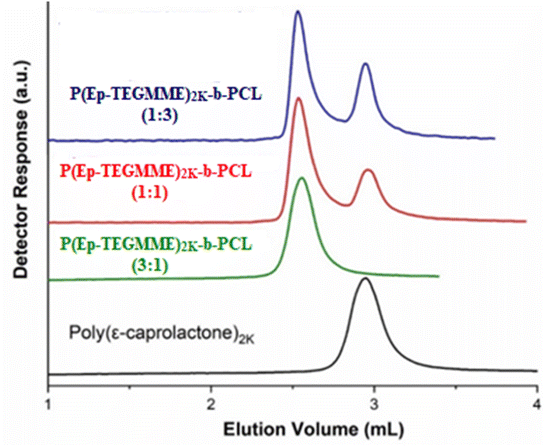 |
| Fig. 8 P(Ep-TEGMME)2K-b-PCL at LCCC-PCL, mobile phase ACN/THF/TEA: 82/17/01 (V%), column: ZORBAX ODS, pore size 70 Å, particle size 5 mm, and column dimensions 250 × 4.6 mm (Phenomenex, USA). | |
The analysis of P(Ep-TEGMME)2K-b-PCL samples by size exclusion chromatography showed an expected increase in the hydrodynamic volume that matches well with the amount of initial ε-caprolactone content. Moreover, analysis at LCCC-PCL on RP column indicate the similar length of P(Ep-TEGMME) block along with the PCL homopolymer content of the sample. It is however not possible to unequivocally decide about presence of free macroinitiator, P(Ep-TEGMME)2K, in the sample and the individual lengths of the PCL block. LCCC at CCP of P(Ep-TEGMME) is required to obtain this information. P(Ep-TEGMME) is a polar polymer compared to PCL, therefore, at its CCP on a NP column PCL should be excluded from the pores of the SP.45
On a NP SP, more polar component of the MP has higher eluotropic strength compared to less polar component. In this case, a binary mixture of acetone and water is used.55 Obviously, the eluotropic strength of water is higher compared to acetone. In a MP composed of 75% acetone in water, P(Ep-TEGMME) have stronger interaction with the SP and eluted in order of increasing molar mass after the void volume of the column, Fig. 9A. The interaction strength decreased considerably while using 70% acetone but the elution order remained the same, higher molar masses eluted later than lower molar masses. Further decrease of acetone content to 65% resulted in reversal of the elution order, higher molar masses eluted earlier than the lower molar masses in a typical SEC order. In between these two opposing elution orders, there is a point at 68% acetone where P(Ep-TEGMME) of a molar mass range 500–3000 eluted at the same elution volume irrespective of its molar mass. This is the CCP of P(Ep-TEGMME) under current conditions. At the CCP of P(Ep-TEGMME), PCL eluted in a typical SEC regime earlier than the void volume of the column, Fig. 9B. These conditions can be used for estimation of the individual block length of PCL in P(Ep-TEGMME)-b-PCL along with separation of free P(Ep-TEGMME) from the rest of the sample. The analysis of the synthesized P(Ep-TEGMME)-b-PCL at LCCC of P(Ep-TEGMME) is demonstrated in Fig. 10. The elution volume of block copolymers decreased with the increase in the initial ε-caprolactone content which indicates an increase in the individual PCL block length. The elution volumes correspond well with the calibration curve of PCL under same conditions. It is important to note that generally not much free P(Ep-TEGMME)2K are present in the block copolymer samples. A small amount of free P(Ep-TEGMME)2K is noticed in P(Ep-TEGMME)2K-b-PCL (3–1) which may be attributed to excess of P(Ep-TEGMME)2K compared to ε-caprolactone in the initial feed.
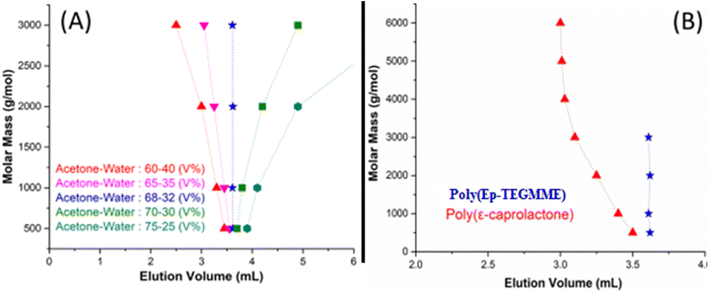 |
| Fig. 9 (A) Elution behavior of different molar masses of P(Ep-TEGMME) as a function of the MP composition (acetone/water); (B) elution behavior of PCL at the CCP of P(Ep-TEGMME), MP: acetone/water: 68/32 (V%), column: Ultisil diol column, 120 Å,5 μm, 250 × 4.6 mm (Welch, China), detector: ELS, column temperature: 30 °C. | |
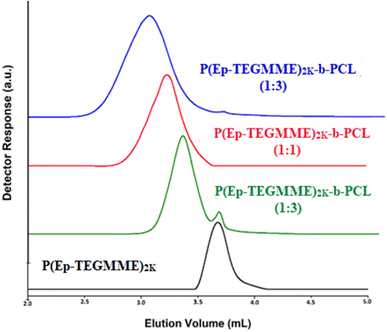 |
| Fig. 10 P(Ep-TEGMME)2K-b-PCL at LCCC-P(Ep-TEGMME), MP: acetone/water: 68/32 (V%), column: Ultisil diol column, 120 Å,5 μm, 250 × 4.6 mm (Welch, China), detector: ELS, column temperature: 30 °C. | |
Hence, the analysis of P(Ep-TEGMME)2K-b-PCL (3–1) by SEC, LCCC-PCL, LCCC-P(Ep-TEGMME) revealed total relative molar mass of macroinitiator and the block copolymers, length of P(Ep-TEGMME) and PCL blocks in the block copolymers along with the content of free homopolymers of both types in the sample. The physical properties and applications of these fascinating architectures of amphiphilic block copolymers will be topic of our next publication of this series.
4. Conclusion
In this study, a facile and convenient method of tailoring of epoxy-terminated macromonomers is reported. 1H-NMR analysis of macromonomers confirm the successful synthesis of the macromonomers. Size exclusion chromatography of the synthesized homopolymers of novel epoxy-terminated macromonomers indicate the success of polymerization by increase in the hydrodynamic volume. Moreover, absence of protons of oxirane ring in 1H-NMR spectrum of the polymers confirm the ring-opening polymerization. Amphiphilic block copolymer brushes with hydrophilic handle and hydrophobic brush as well as with hydrophobic handle and hydrophilic brush are synthesized by copolymerization of epoxy-terminated macromonomers with other appropriate monomers. The analysis of bottle-brush like architectures of amphiphilic block copolymers by liquid chromatography at critical conditions revealed individual block lengths and homopolymer content of the samples.
Data availability
Data will be made available on request.
Conflicts of interest
There are no conflicts to declare.
Acknowledgements
The research is supported by Higher Education Commission of Pakistan under “National Research Program for Universities” against project # 5735.
References
- L. Y. Qiu and Y. H. Bae, Pharm. Res., 2006, 23, 1–30 CrossRef CAS PubMed
. - M. W. Neiser, S. Muth, U. Kolb, J. R. Harris, J. Okuda and M. Schmidt, Angew. Chem., Int. Ed., 2004, 43, 3192–3195 CrossRef CAS PubMed
. - H.-i. Lee, J. Pietrasik, S. S. Sheiko and K. Matyjaszewski, Prog. Polym. Sci., 2010, 35, 24–44 CrossRef CAS
. - A. L. Liberman-Martin, C. K. Chu and R. H. Grubbs, Macromol. Rapid Commun., 2017, 38, 1700058 CrossRef PubMed
. - C. Feng and X. Huang, Acc. Chem. Res., 2018, 51, 2314–2323 CrossRef CAS PubMed
. - T. Chen, I. Amin and R. Jordan, Chem. Soc. Rev., 2012, 41, 3280–3296 RSC
. - S. Peng and B. Bhushan, RSC Adv., 2012, 2, 8557–8578 RSC
. - N. D. Ogbonna, M. Dearman, C.-T. Cho, B. Bharti, A. J. Peters and J. Lawrence, JACS Au, 2022, 2, 898–905 CrossRef CAS PubMed
. - R. Verduzco, X. Li, S. L. Pesek and G. E. Stein, Chem. Soc. Rev., 2015, 44, 2405–2420 RSC
. - A. Chremos and P. E. Theodorakis, ACS Macro Lett., 2014, 3, 1096–1100 CrossRef CAS PubMed
. - W. J. Brittain and S. Minko, J. Polym. Sci., Part A: Polym. Chem., 2007, 45, 3505–3512 CrossRef CAS
. - O. Azzaroni, J. Polym. Sci., Part A: Polym. Chem., 2012, 50, 3225–3258 CrossRef CAS
. - W.-L. Chen, R. Cordero, H. Tran and C. K. Ober, Macromolecules, 2017, 50, 4089–4113 CrossRef CAS
. - J. A. Johnson, Y. Y. Lu, A. O. Burts, Y. Xia, A. C. Durrell, D. A. Tirrell and R. H. Grubbs, Macromolecules, 2010, 43, 10326–10335 CrossRef CAS PubMed
. - J. A. Johnson, Y. Y. Lu, A. O. Burts, Y.-H. Lim, M. G. Finn, J. T. Koberstein, N. J. Turro, D. A. Tirrell and R. H. Grubbs, J. Am. Chem. Soc., 2011, 133, 559–566 CrossRef CAS PubMed
. - T. Hirai, M. Sato, T. Kato, H. Shimamoto, K. Uno, N. Otozawa and A. Takahara, Polym. J., 2018, 50, 931–939 CrossRef CAS
. - R. Ducker, A. Garcia, J. Zhang, T. Chen and S. Zauscher, Soft Matter, 2008, 4, 1774–1786 RSC
. - S. S. Sheiko, B. S. Sumerlin and K. Matyjaszewski, Prog. Polym. Sci., 2008, 33, 759–785 CrossRef CAS
. - Y. L. Kamble, D. J. Walsh and D. Guironnet, Macromolecules, 2022, 55, 10255–10263 CrossRef CAS
. - C. G. Rodriguez, R. C. Ferrier, A. Helenic and N. A. Lynd, Macromolecules, 2017, 50, 3121–3130 CrossRef CAS
. - Y. Xia and J. Zhao, Polymer, 2018, 143, 343–361 CrossRef CAS
. - M. I. Childers, J. M. Longo, N. J. Van Zee, A. M. LaPointe and G. W. Coates, Chem. Rev., 2014, 114, 8129–8152 CrossRef CAS PubMed
. - P. Verkoyen, T. Johann, J. Blankenburg, C. Czysch and H. Frey, Polym. Chem., 2018, 9, 5327–5338 RSC
. - P. Verkoyen and H. Frey, Macromol.
Rapid Commun., 2020, 41, 2000225 CrossRef CAS PubMed
. - G. Gaucher, M.-H. Dufresne, V. P. Sant, N. Kang, D. Maysinger and J.-C. Leroux, J. Controlled Release, 2005, 109, 169–188 CrossRef CAS PubMed
. - K. Kataoka, A. Harada and Y. Nagasaki, Adv. Drug Delivery Rev., 2001, 47, 113–131 CrossRef CAS PubMed
. - S. B. Darling, Prog. Polym. Sci., 2007, 32, 1152–1204 CrossRef CAS
. - I. W. Hamley, Block Copolymers in Solution: Fundamentals and Applications, John Wiley & Sons, Ltd, Chichester, England, 2005 Search PubMed
. - S. Zhou, X. Deng and H. Yang, Biomaterials, 2003, 24, 3563–3570 CrossRef CAS PubMed
. - A. S. Mikhail and C. Allen, Biomacromolecules, 2010, 11, 1273–1280 CrossRef CAS PubMed
. - X. Shuai, H. Ai, N. Nasongkla, S. Kim and J. Gao, J. Controlled Release, 2004, 98, 415–426 CrossRef CAS PubMed
. - M. L. Adams, A. Lavasanifar and G. S. Kwon, J. Pharm. Sci., 2003, 92, 1343–1355 CrossRef CAS PubMed
. - S. Paul, Y. Zhu, C. Romain, R. Brooks, P. K. Saini and C. K. Williams, Chem. Commun., 2015, 51, 6459–6479 RSC
. - A.-L. Brocas, C. Mantzaridis, D. Tunc and S. Carlotti, Prog. Polym. Sci., 2013, 38, 845–873 CrossRef CAS
. - M. I. Malik, B. Trathnigg and C. O. Kappe, Eur. Polym. J., 2009, 45, 899–910 CrossRef CAS
. - M. I. Malik, B. Trathnigg and C. O. Kappe, J. Chromatogr. A, 2009, 1216, 1167–1173 CrossRef CAS PubMed
. - M. I. Malik, B. Trathnigg and C. O. Kappe, Eur. Polym. J., 2008, 44, 144–154 CrossRef CAS
. - M. I. Malik, B. Trathnigg and C. O. Kappe, Macromol. Chem. Phys., 2007, 208, 2510–2524 CrossRef CAS
. - J. Herzberger, K. Niederer, H. Pohlit, J. Seiwert, M. Worm, F. R. Wurm and H. Frey, Chem. Rev., 2016, 116, 2170–2243 CrossRef CAS PubMed
. - A. M. Striegel, Multiple detection in size-exclusion chromatography, ACS Publications, 2004 Search PubMed
. - A. Lederer and J. Brandt, in Molecular Characterization of Polymers, ed. M. I. Malik, J. Mays and M. R. Shah, Elsevier, 2021, pp. 61–96, DOI:10.1016/B978-0-12-819768-4.00012-9
. - M. I. Malik and H. Pasch, in Molecular Characterization of Polymers, ed. M. I. Malik, J. Mays and M. R. Shah, Elsevier, 2021, pp. 1–59, DOI:10.1016/B978-0-12-819768-4.00007-5
. - M. I. Malik, Chromatographia, 2021, 84, 1089–1094 CrossRef CAS
. - T. Ahmad, S. S. Durrani, F. J. Iftikhar, S. G. Musharraf and M. I. Malik, Eur. Polym. J., 2021, 142, 110161 CrossRef CAS
. - M. I. Malik, J. Chromatogr. A, 2020, 1609, 460440 CrossRef CAS PubMed
. - R. Khatoon, S. Rahim, R. Abdul-Karim, S. G. Musharraf and M. I. Malik, Macromolecules, 2019, 52, 7688–7695 CrossRef CAS
. - M. I. Malik and H. Pasch, Prog. Polym. Sci., 2014, 39, 87–123 CrossRef CAS
. - M. I. Malik, G. W. Harding and H. Pasch, Anal. Bioanal. Chem., 2012, 403, 601–611 CrossRef CAS PubMed
. - M. I. Malik, G. W. Harding, M. E. Grabowsky and H. Pasch, J. Chromatogr. A, 2012, 1244, 77–87 CrossRef CAS PubMed
. - M. I. Malik, B. Trathnigg, K. Bartl and R. Saf, Anal. Chim. Acta, 2010, 658, 217–224 CrossRef CAS PubMed
. - M. I. Malik, B. Trathnigg and R. Saf, J. Chromatogr. A, 2009, 1216, 6627–6635 CrossRef CAS PubMed
. - M. A. Bashir and W. Radke, J. Chromatogr. A, 2012, 1225, 107–112 CrossRef CAS PubMed
. - M. A. Bashir and W. Radke, J. Chromatogr. A, 2007, 1163, 86–95 CrossRef CAS PubMed
. - M. A. Bashir and W. Radke, J. Chromatogr. A, 2006, 1131, 130–141 CrossRef CAS PubMed
. - H. Ahmed, B. Trathnigg, C. O. Kappe and R. Saf, Eur. Polym. J., 2009, 45, 2338–2347 CrossRef CAS
.
|
This journal is © The Royal Society of Chemistry 2023 |
Click here to see how this site uses Cookies. View our privacy policy here.