DOI:
10.1039/D2SC05841C
(Edge Article)
Chem. Sci., 2023,
14, 1286-1290
A Ni-catalyzed asymmetric C(sp)–P cross-coupling reaction for the synthesis of P-stereogenic alkynylphosphines†
Received
21st October 2022
, Accepted 6th January 2023
First published on 7th January 2023
Abstract
Due to the high reactivity of the triple bond, P-stereogenic alkynylphosphines could be easily derivatized, serving as universal building blocks for structurally diverse phosphine compounds. However, the synthesis of alkynylphosphines via direct P–C bond formation was unprecedented. Here, we report an efficient method for the synthesis of P-stereogenic alkynylphosphines with high enantioselectivity via a Ni-catalyzed asymmetric cross-coupling reaction. The reaction could tolerate a variety of functional groups, affording products that can be converted into useful phosphine derivatives.
Introduction
Enantioenriched P-stereogenic phosphines have been applied to a wide range of asymmetric transformations as ligands for metal catalysts or directly as organocatalysts.1 Compared with phosphine ligands with backbone stereogenicities, P-stereogenic phosphines may exhibit a unique enantioselective induction because the stereogenic center of the catalyst is closer to the active site. In particular, P-stereogenic ligands with large differences in the bulkiness of the substituents on the phosphorus atom have been introduced as a key motif into ligands because of their ability to induce high enantioselectivity in asymmetric catalysis. And the best among them is t-butyl-substituted P-stereogenic ligands,2 such as QuinoxP,2a DuanPhos,2b Buchwald ligand,2c and WingPhos,2d which have found broad applications in both academic investigations and industrial applications.
Alkynyl groups are considered to have the minimum stereobulkiness due to their linear topology. Therefore, the incorporation of both the small alkynyl and the large tert-butyl groups on the phosphorus atom would create the largest bulkiness differentiation and presumably exhibit profound stereogenic discrimination. In this regard, several interesting alkynyl chiral phosphine ligands have been developed, showing enhanced catalytic excellent performance in reactions, such as catalytic asymmetric hydrogenation and allylic substitution reactions (Scheme 1A).3,4 Furthermore, alkynylphosphine oxides have been found to have attractive biological activities in medicinal chemistry,5 as well as photoluminescent materials.6
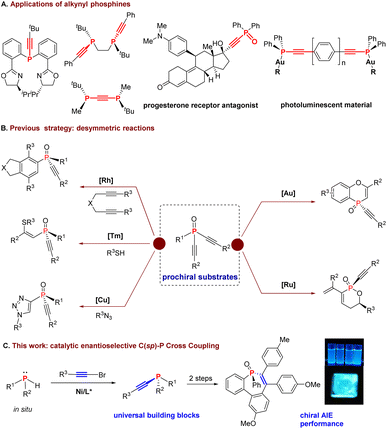 |
| Scheme 1 P-stereogenic alkynylphosphines. | |
However, the strategy for the synthesis of P-stereogenic alkynylphosphines is limited.7 Generally, most P-stereogenic alkynylphosphines were synthesized from stoichiometric amounts of chiral substrates via nucleophilic substitution reactions.3,8 And transition metal-catalyzed dehydrogenative coupling of stereogenic secondary phosphine oxides or secondary phosphine boranes with terminal alkynes provided an alternative method with retention of the P-stereogenicity.9,10 Despite better atom economy, stoichiometric stereogenic phosphine starting materials were still required in this method. As a more efficient strategy, a plethora of catalytic desymmetrization reactions have been reported recently with prostereogenic dialkynylphosphines through [2 + 2 + 2] cycloaddition, 1,4-addition reactions, etc. (Scheme 1B).11 In brief, previous methods suffer from limited substrate scope or tedious preparation of starting materials and are thus lacking in broad applications of the products. The development of catalytic asymmetric reactions by direct P–C bond formation to prepare P-stereogenic alkynylphosphines remains unexplored.
Recently, a transition metal-catalyzed enantioselective C(sp2)–P and C(sp3)–P coupling reaction was reported an effective strategy for the construction of P-stereogenic centers.12 However, enantioselective C(sp)–P coupling has not been reported. In addition, the synthesis of chiral P(III) phosphines is challenging in transition metal catalyzed asymmetric reactions because of the strong poisoning effect of both the P(III) starting material and the product.12j,t–v Besides, the previously reported Ni-catalyzed asymmetric syntheses of P-stereogenic phosphines do not work for bulky P-nucleophiles, e.g. phosphines with a tert-butyl or mesityl substituent.12m–o Herein, we disclosed our finding in the catalytic asymmetric cross-coupling reaction of secondary phosphines with bromoalkynes, in which excellent results could be obtained with bulky secondary phosphines (Scheme 1C).
Results and discussion
The cross-coupling reaction was carried out with secondary phosphine 1a, which was reduced in situ from bench-stable secondary phosphine oxides and (bromoethynyl)benzene 2a (Table 1). At the outset of the investigation, we were aware that the high reactivity of the bromoalkyne may lead to an uncatalyzed background reaction, resulting in diminished ee. As expected, when the reaction was performed without a catalyst, a significant background reaction occurred to afford the desired product 3aa in 20% yield (please see the ESI†). Therefore, a highly active catalyst is required to alleviate such a problem. Low temperature is beneficial to reduce the background reaction, although a long reaction time is required for the completion of the reaction.
Table 1 Initial evaluation of the cross-coupling reaction
After extensive evaluation of the reaction parameters, we were able to obtain the optimal conditions as follows. The secondary phosphine was formed in situ from 1 (0.2 mmol) and PhSiH3 (0.2 mmol) followed by the addition of substrate 2a (0.1 mmol), Ni(COD)2 (5 mol%), (S,S)-Et-Duphos (6 mol%), NaOAc (0.25 mmol), and mesitylene (1 mL) at 0 °C. Preliminary evaluation suggested that the stereohindrance of the substituents on the P atom also showed a profound effect on the enantioselectivity (Table 1). The bulky t-butyl phenyl secondary phosphine gave the best results with 86% ee and 72% overall isolated yield of product 3ba, whose absolute configuration was determined to be sp by comparison with known compounds (please see the ESI†).
The scope of the Ni-catalysed asymmetric C(sp)–P cross coupling reaction was investigated with respect to both the P-substituents and the bromoalkynes under the optimized conditions obtained above (Table 2). For the convenience of isolation and purification, the tertiary alkynylphosphine products were protected either as a borane derivative (3ea′), sulfides or oxides. The method could tolerate a broad range of secondary phosphines with various substituents at the aryl group. Products with substituents at the para position of the P-aryl group were obtained in 63–78% overall yields and 76–90% ee (3fa–3ka). Substrates with meta-substituents (1l, 1m) also reacted smoothly, affording products 3la and 3ma in 75% ee and 64% yield and 82% ee and 70% yield, respectively. In addition, substrate 1n, bearing an o-methylphenyl group, could give the desired product with better enantioselectivity (3na, 90% ee) than substrates 1o and 1p (3oa, 76% ee and 3pa, 86% ee).
1 (0.2 mmol), PhSiH3 (0.2 mmol), NaOAc (0.25 mmol), 2 (0.1 mmol), Ni(COD)2 (5 mol%), (S,S)-Et-Duphos (6 mol%), 1 mL mesitylene, 0 °C, and 72 h. Isolated yields. dr was determined by 31P NMR analyses of crude reaction mixtures.
−10 °C and 96 h.
1 (0.4 mmol), PhSiH3 (0.4 mmol), NaOAc (0.5 mmol), Ni(COD)2 (10 mol%), (S,S)-Et-Duphos (12 mol%), 0 °C, and 120 h. dr was determined by HPLC analyses.
Ni(COD)2 (10 mol%), (S,S)-Et-Duphos (12 mol%), 10 °C, and 96 h.
|
|
The current process was highly reliable and scalable, as demonstrated by the gram-scale reaction where 1n (5 mmol, 0.98 g) and 2a proceeded smoothly to generate 3na with 87% ee and 54% yield. Furthermore, secondary phosphines with other aromatic groups were also competent substrates, generating the corresponding products with 80% ee and 60% yield, and 72% ee and 41% yield (3qa and 3ra). To verify the effect of the o-methylphenyl group on the enantioselectivity, substrates 1s and 1t were tested. Both substrates gave corresponding products 3sa and 3ta in 90% ee and 92% ee. Notably, isopropyl-substituted (1u) and mesityl isopropyl (1v) secondary phosphines were also amenable to the protocol to give the desired products with 88% ee and 68% yield and 80% ee and 50% yield, respectively.
The scope of bromoalkynes was then examined (Table 2). Aromatic bromoalkynes with a variety of functional groups (2a–2i) were all amenable to the reaction, affording phosphine oxides in 84–93% ee and 51–64% yields (3na-O–3ni). Substrates with electron-donating groups at the para position gave better enantioselectivities than substrates with electron-withdrawing groups (3nbvs.3nc, 3nd, and 3ne). A similar trend was observed for meta-substituted aryl groups (3nfvs.3ng). This result may be due to the lower electrophilicity of electron-rich bromoalkynes, which results in minimal background reactions. In addition, substrates with electron-donating groups (OMe) at the ortho position also gave high enantioselectivity in 90% ee (3nh). The reactions of bromoalkynes bearing naphthyl and thienyl groups also gave the products with high ee (81% and 78%) and moderate yield (3ni and 3nj). Notably, the reaction of 1,3-bis(bromoethynyl) benzene 2k with 1n gave the double cross-coupling product 3nk in 97% ee, 5
:
1 dr and 33% overall yield. Then, the reaction of alkyl bromoalkynes was tested. Thus, substrate 1n was selected to react with three representative alkyl bromoalkynes. All these substrates gave the corresponding products in 93%, 90% and 84% ee, respectively, albeit with diminished overall yields (3nl–3nn). The application of this reaction in a biologically relevant molecule was then investigated. And L-phenylalanine derivative 2o gave corresponding products 3no in 9
:
1 dr and 32% yield under standard conditions.
In addition, the P-stereogenic alkynylphosphine products could be easily elaborated to structurally diverse P-stereogenic molecules through simple transformations. P-stereogenic alkynylphosphine oxide 3na-O could undergo a hydration reaction under palladium catalysis to give β-keto-phosphine derivative 4, an analog of β-lactamase inhibitors13a,b in 84% ee and 73% yield (Fig. 1A). In addition, 3ba could also be transformed into π-conjugated benzo[b]phosphole oxides 5 in 86% ee, 58% yield and 3
:
1 dr by a radical-initiated tandem addition/cyclization reaction (Fig. 1B).13c Phosphine derivatives have recently attracted broad attention in photoluminescent materials; however, the chiroptical properties of these materials with a P-stereogenic center have rarely been reported.14 To probe the potential value of enantioenriched P-stereogenic phosphines in luminescent materials, seven-membered phosphepine 6 was synthesized from 3wp (64% ee and 53% yield) in two steps (99% ee after recrystallization, Fig. 1C).15 And the compound exhibited promising CPL photoluminescent properties in addition to the originally reported AIE effect of racemic 6 (Fig. 1D and E). The compound also represents a rare example of a CPL material with a single P-stereogenic centre stereogenicity, which merits in-depth investigation.
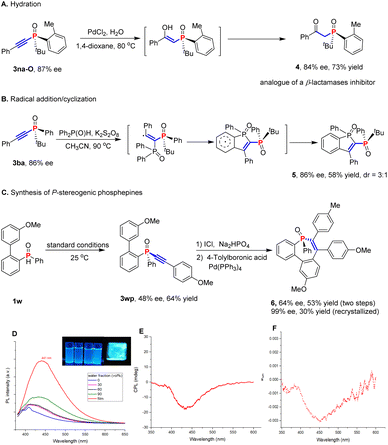 |
| Fig. 1 (A) Hydration. (B) Radical addition cyclization. (C) Synthesis of P-stereogenic phosphepine. (D) PL spectra of 6 in THF/water mixtures with different water fractions and 6 films under 365 nm. (E) CPL spectra of 6 films. (F) glum value spectra of 6 films. | |
Conclusions
In summary, we developed an efficient method for the synthesis of P-stereogenic alkynylphosphines via a Ni-catalyzed asymmetric cross coupling reaction. The P-stereogenic alkynylphosphines could be converted to structurally diverse P-stereogenic compounds via simple transformations. The discovery may inspire new applications of alkynylphosphines in asymmetric catalysis and materials chemistry.
Data availability
The experimental details, characterization data, NMR spectra, and HPLC chromatograms associated with this article are provided in the ESI.†
Author contributions
Q. Z. conceived the project and B. Z. initiated the optimization studies and conducted the experiments. W. Z., X. L. and Y. S. helped with a part of the substrate scope. B. Z and Q. Z. wrote the manuscript together. All authors have given approval to the final version of the manuscript.
Conflicts of interest
There are no conflicts to declare.
Acknowledgements
We acknowledge financial support from the NSFC (21901235 and 22071224) and the USTC Research Funds of the Double First-Class Initiative (YD2060002010). Dr Shan Jin and Prof. Manzhou Zhu of Anhui University, and Dr Pengcai Liu of the State Key Laboratory of Elemento-Organic Chemistry, College of Chemistry, Nankai University, are acknowledged for helpful discussions about optical data measurement.
Notes and references
- For the selected examples, see:
(a) B. D. Vineyard, W. S. Knowles, M. J. Sabacky, G. L. Bachman and D. J. Weinkauff, J. Am. Chem. Soc., 1977, 99, 5946–5952 CrossRef CAS;
(b) W. S. Knowles, Adv. Synth. Catal., 2003, 345, 3–13 CrossRef CAS;
(c) T. Imamoto, Yuki Gosei Kagaku Kyokaishi, 2007, 65, 1060–1069 CrossRef CAS;
(d) M. Dutartre, J. Bayardon and S. Juge, Chem. Soc. Rev., 2016, 45, 5771–5794 RSC;
(e) T. Imamoto, Chem. Rec., 2016, 16, 2659–2673 CrossRef PubMed;
(f) Y. J. Xiang, Q. Y. Ge, S. L. Wu, Y. Zheng and Z. H. Yang, RSC Adv., 2022, 10, 9563–9578 RSC;
(g) A. Cabre, A. Riera and X. Verdaguer, Acc. Chem. Res., 2022, 53, 676–689 CrossRef PubMed.
- For the selected recent examples, see:
(a) T. Imamoto, K. Sugita and K. Yoshida, J. Am. Chem. Soc., 2005, 127, 11934–11935 CrossRef CAS PubMed;
(b) H. Geng, W. C. Zhang, J. Chen, G. Hou, L. Zhou, Y. Zou, W. Wu and X. Zhang, Angew. Chem., Int. Ed., 2009, 48, 6052–6054 CrossRef CAS PubMed;
(c) A. Taylor, R. A. Altman and S. L. Buchwald, J. Am. Chem. Soc., 2009, 131, 9900–9901 CrossRef CAS PubMed;
(d) G. Liu, X. Liu, Z. Cai, G. Jiao, G. Xu and W. Tang, Angew. Chem., Int. Ed., 2013, 52, 4235–4238 CrossRef CAS PubMed;
(e) P. J. González-Liste, F. Leon, I. Arribas, M. Rubio, S. E. Garcia-Garrido, V. Cadierno and A. Pizzano, ACS Catal., 2016, 6, 3056–3060 CrossRef;
(f) B. Li, J. Chen, Z. Zhang, I. D. Gridnev and W. Zhang, Angew. Chem., Int. Ed., 2019, 58, 7329–7334 CrossRef CAS PubMed;
(g) W. Tang, N. Patel, G. Xu, X. Xu, J. Savoie, S. Ma, M. Hao, S. Keshipeddy, A. G. Capacci, X. Wei, Y. Zhang, J. J. Gao, W. Li, S. Rodriguez, B. Lu, N. K. Yee and C. H. Senanayake, Org. Lett., 2012, 14, 2258–2261 CrossRef CAS PubMed;
(h) N. Hu, G. Zhao, Y. Zhang, X. Liu, G. Li and W. Tang, J. Am. Chem. Soc., 2015, 137, 6746–6749 CrossRef CAS PubMed;
(i) Y.-S. Jang, L. Woz'niak, J. Pedroni and N. Cramer, Angew. Chem., Int. Ed., 2018, 57, 12901–12905 CrossRef CAS PubMed;
(j) L. Li, Y.-C. Liu and H. Shi, J. Am. Chem. Soc., 2021, 143, 4154–4161 CrossRef CAS PubMed.
- T. Imamoto, Y. Saitoh, A. Koide, T. Ogura and K. Yoshida, Angew. Chem., Int. Ed., 2007, 46, 8636–8639 CrossRef CAS PubMed.
- W.-B. Xu, M. Sun, M. Shu and C. Li, J. Am. Chem. Soc., 2021, 143, 8255–8260 CrossRef CAS PubMed.
- For the selected recent examples, see:
(a) W. Jiang, J. J. Fiordeliso, O. Linton, P. Tannenbaum, J. Xu, P. Zhu, J. Gunnet, K. Demarest, S. Lundeen and Z. Sui, Steroids, 2006, 71, 949–954 CrossRef CAS PubMed;
(b) D. G. Salomon, S. M. Grioli, M. Buschiazzo, E. Mascaro, C. Vitale, G. Radivoy, M. Perez, Y. Fall, E. A. Mesri, A. C. Curino and M. M. Facchinetti, ACS Med. Chem. Lett., 2011, 2, 503–508 CrossRef CAS PubMed;
(c) M. Horiba, T. Yamaguchi and S. Obika, J. Org. Chem., 2022, 85, 1794–1801 CrossRef PubMed;
(d) M. Pujadas and L. Rodriguez, Coord. Chem. Rev., 2022, 408, 213179 CrossRef.
- For the selected recent examples, see:
(a) S. Zhu, Y. Zhang, P. Li, W. Bi, X. Chen and Y. Zhao, Phosphorus Sulfur Silicon Relat. Elem., 2017, 192, 1–8 CrossRef CAS;
(b) A. Gupta and B. Flynn, J. Org. Chem., 2016, 81, 4012–4019 CrossRef CAS PubMed;
(c) B. O. Ashburn, R. G. Carter and L. N. Zakharov, J. Am. Chem. Soc., 2007, 129, 9109–9116 CrossRef CAS PubMed;
(d) G. Nishida, K. Noguchi, M. Hirano and K. Tanaka, Angew. Chem., Int. Ed., 2007, 46, 3951–3954 CrossRef CAS PubMed;
(e) K. Kondoh, H. Yorimitsu and K. Oshima, J. Am. Chem. Soc., 2007, 129, 4099–4104 CrossRef PubMed;
(f) S. Sasaki, K. Adachi and M. Yoshifuji, Org. Lett., 2007, 9, 1729–1732 CrossRef CAS PubMed;
(g) J. Mo, D. Kang, D. Eom, S. H. Kim and P. H. Lee, Org. Lett., 2003, 15, 26–29 CrossRef PubMed;
(h) D. Lecercle, M. Sawicki and F. Taran, Org. Lett., 2006, 8, 4283–4285 CrossRef CAS PubMed;
(i) J. M. Kee, B. Villani, L. R. Carpenter and T. W. Muir, J. Am. Chem. Soc., 2010, 132, 14327–14329 CrossRef CAS PubMed;
(j) A. Kondoh and H. Y. K. Oshima, Chem. – Asian J., 2010, 5, 398–409 CrossRef CAS PubMed.
-
(a) A. Ochida, H. Ito and M. Sawamura, J. Am. Chem. Soc., 2006, 128, 16486–16487 CrossRef CAS PubMed;
(b) A. Ochida and M. Sawamura, Chem. – Asian J., 2007, 2, 609–618 CrossRef CAS PubMed.
- L.-J. Liu, W.-M. Wang, L. Yao, F.-J. Meng, Y.-M. Sun, H. Xu, Z. Y. Xu, Q. Li and C.-Q. Zhao, J. Org. Chem., 2017, 82, 11990–12002 CrossRef CAS PubMed.
- J. Yang, T. Chen, Y. Zhou, S.-F. Yin and L.-B. Han, Organometallics, 2015, 34, 5095–5098 CrossRef CAS.
- K. Jouvin, R. Veillard, C. Theunissen, C. Alayrac, A.-C. Gaumont and G. Evano, Org. Lett., 2013, 15, 4592–4595 CrossRef CAS PubMed.
-
(a) G. Nishida, K. Noguchi, M. Hirano and K. Tanaka, Angew. Chem., Int. Ed., 2008, 47, 3410–3413 CrossRef CAS PubMed;
(b) J. S. Harvey, G. T. Giuffredi and V. Gouverneur, Org. Lett., 2010, 12, 1236–1239 CrossRef CAS PubMed;
(c) Y. Zheng, L. Guo and W. Zi, Org. Lett., 2018, 20, 7039–7043 CrossRef CAS PubMed;
(d) Y. Zhang, F. Zhang, L. Chen, J. Xu, X. Liu and X. Feng, ACS Catal., 2009, 9, 4834–4840 CrossRef;
(e) R. Y. Zhu, L. Chen, X. S. Hu, F. Zhou and J. Zhou, Chem. Sci., 2020, 11, 97–106 RSC.
- For the selected recent examples, see:
(a) J. R. Moncarz, N. F. Laritcheva and D. S. Glueck, J. Am. Chem. Soc., 2002, 124, 13356–13357 CrossRef CAS PubMed;
(b) D. S. Glueck, Synlett, 2007, 48, 2627–2634 CrossRef;
(c) D. S. Glueck, Chem. – Eur. J., 2008, 14, 7108–7117 CrossRef CAS PubMed;
(d) Q. Dai, L. Liu and J. L. Zhang, Angew. Chem., Int. Ed., 2021, 60, 27247–27252 CrossRef CAS PubMed;
(e) Q. Dai, W. B. Li, Z. M. Lo and J. L. Zhang, J. Am. Chem. Soc., 2019, 141, 20556–20564 CrossRef CAS PubMed;
(f) A. Mondal, N. O. Thiel, R. Dorel and B. L. Feringa, Nat. Catal., 2022, 5, 10–19 CrossRef CAS;
(g) N. F. Blank, K. C. McBroom, D. S. Glueck and W. S. Kassel, Organometallics, 2006, 25, 1742–1748 CrossRef CAS;
(h) C. Korff and G. Helmchen, Chem. Commun., 2004, 5, 530–531 RSC;
(i) Y. L. Li, X. Jin, P. Liu, H. Zhang, X. Yu, Y. Liu, B. Liu and W. Yang, Angew. Chem., Int. Ed., 2022, e202117093 CAS;
(j) V. S. Chan, M. Chiu, R. G. Bergman and F. D. Toste, J. Am. Chem. Soc., 2009, 131, 6021–6032 CrossRef CAS PubMed;
(k) B. J. Anderson, S. C. Reynolds, M. A. Guinoo, Z. Xu and D. S. Glueck, ACS Catal., 2016, 6, 8106–8114 CrossRef CAS;
(l) R. Beaud, R. J. Phipps and M. J. Gaunt, J. Am. Chem. Soc., 2016, 138, 13183–13186 CrossRef CAS PubMed;
(m) X.-T. Liu, Y.-Q. Zhang, X.-Y. Han, S.-P. Sun and Q.-W. Zhang, J. Am. Chem. Soc., 2019, 141, 16584–16589 CrossRef CAS PubMed;
(n) Q. Zhang, X.-T. Liu, Y. Wu and Q.-W. Zhang, Org. Lett., 2021, 23, 8683–8687 CrossRef CAS PubMed;
(o) W.-Q. Cai, Q. Wei and Q. W. Zhang, Org. Lett., 2022, 24, 1258–1262 CrossRef CAS PubMed;
(p) C. Li, W. Li, S. Xu and W.-L. Duan, Chinese J. Org. Chem., 2013, 33, 799–802 CrossRef CAS;
(q) Z.-H. Wu, A.-Q. Cheng, M. Yuan, Y.-X. Zhao, H.-L. Yang, L.-H. Wei, H.-Y. Wang, T. Wang, Z. Zhang and W. L. Duan, Angew. Chem., Int. Ed., 2021, 60, 27241–27246 CrossRef CAS PubMed;
(r) H. Zhou, Z.-L. Li, Q.-S. Gu and X.-Y. Liu, ACS Catal., 2021, 11, 7978–7986 CrossRef CAS;
(s) C. Luan, C.-J. Yang, L. Liu, Q.-S. Gu and X.-Y. Liu, Chem. Catal., 2022, 2, 2876–2888 CrossRef;
(t) D. Ji, J. Jing, Y. Wang, Z. Qi, F. Wang, X. Zhang, Y. Wang and X. Li, Chem. Catal., 2022, 2, 3346–3362 Search PubMed;
(u) Y.-B. Li, H. Tian and L. Yin, J. Am. Chem. Soc., 2020, 142, 20098–20106 CrossRef CAS PubMed;
(v) V. S. Chan, L. C. Stewart, R. G. Bergman and F. D. Toste, J. Am. Chem. Soc., 2006, 128, 2786–2787 CrossRef CAS PubMed.
-
(a) S. K. Perumal, A. A. Adediran and R. F. Pratt, Bioorg. Med. Chem., 2008, 16, 6987–6994 CrossRef CAS PubMed;
(b) X. Li, G. Hu, P. Luo, G. Tang, Y. Gao, P. Xu and Y. Zhao, Adv. Synth. Catal., 2012, 354, 2427–2432 CrossRef CAS;
(c) D. Ma, W. Chen, G. Hu, Y. Zhang, Y. Gao, Y. Yin and Y. Zhao, Green Chem., 2016, 18, 3522–3526 RSC.
-
(a) R. Mokrai, A. Mocanu, M. P. Duffy, T. Vives, E. Caytan, V. Dorcet, T. Roisnel, L. Nyulaszi, Z. Benko, P.-A. Bouit and M. Hissler, Chem. Commun., 2021, 57, 7256–7259 RSC;
(b) S. Nishigaki, K. Murayama, Y. Shibata and K. Tanaka, Mater. Chem. Front., 2018, 2, 585–590 RSC.
- D. Wu, D. Wu, C. Hu, L. Qiu, Z. Duan and F. Mathey, Eur. J. Org. Chem., 2019, 6369–6637 CrossRef CAS.
|
This journal is © The Royal Society of Chemistry 2023 |
Click here to see how this site uses Cookies. View our privacy policy here.