DOI:
10.1039/D3SC00107E
(Edge Article)
Chem. Sci., 2023,
14, 3523-3530
Supramolecular photosensitizers using extended macrocyclic hosts for photodynamic therapy with distinct cellular delivery†
Received
9th January 2023
, Accepted 28th February 2023
First published on 8th March 2023
Abstract
The photosensitizers (PSs) for photodynamic therapy (PDT) mostly possess conjugated skeletons that are over-sized and poorly water-soluble to be encapsulated by conventional macrocyclic receptors. Herein, we report that two fluorescent hydrophilic cyclophanes, AnBox·4Cl and ExAnBox·4Cl, can effectively bind hypocrellin B (HB), a pharmaceutically active natural PS for PDT, with binding constants of the 107 level in aqueous solutions. The two macrocycles feature extended electron-deficient cavities and can be facilely synthesized through photo-induced ring expansions. The corresponding supramolecular PSs (HB⊂AnBox4+ and HB⊂ExAnBox4+) exhibit desirable stability, biocompatibility, and cellular delivery, as well as excellent PDT efficiency against cancer cells. In addition, living cell imaging results indicate that HB⊂AnBox4+ and HB⊂ExAnBox4+ have different delivery effects at the cellular level.
Introduction
Facilitating drug delivery is crucial to achieve optimal therapeutic effects to increase drug hydrophilicity, reduce side effects, and improve bioavailability.1,2 In particular, most photosensitizers (PSs) for photodynamic therapy (PDT) have large conjugated skeletons that are poorly soluble in water, thereby making cellular delivery of PSs an active area of investigation.3–7 For example, the natural perylenequinonoid PS hypocrellin B (HB) is an active pharmaceutical ingredient in commercialized Chinese medicines for PDT treatment of skin diseases,8,9 but is for external uses only due to its extremely low water solubility. In addition, insufficient solubility would lead to undesired aggregation which not only limits the drug delivery for biomedical applications, but also complicates the investigation to monitor and optimize the in situ photodynamic performance at the cellular level.10
Macrocycles represent a key molecular basis for supramolecular chemistry and essential resources for non-covalent interactions, thereby providing an excellent platform for drug delivery with proven success.11–19 Compared with nanomaterial-based drug delivery strategies,20–26 macrocyclic supramolecular receptors could form discrete, monodisperse host–guest complexes with drug molecules, featuring advantages including user-friendly operations, molecular-level precision, and guest-specific affinity.27 Moreover, due to the reversible and dynamic host–guest interactions, macrocycles have emerged as powerful components for smart and environment-responsive drug delivery systems.28–30
Because of the limited cavity sizes, single molecules of conventional macrocycles, such as crown ethers, cyclodextrins, calixarenes, pillararenes, and cucurbiturils, become unsuitable to encapsulate large guest molecules with diameters of more than 1 nm like HB.31,32 Thus, the suitable macrocyclic receptors for HB delivery should have strong guest binding to avoid drug leakage, while be able to release the PS in the cellular environment. The resulting supramolecular PS would prevent aggregate formation and multi-step chemical modifications of the natural PS.33–38
Here we report two water-soluble cationic cyclophanes, AnBox·4Cl and ExAnBox·4Cl (Fig. 1), as the first macrocyclic supramolecular receptors of HB (the natural form without chemical modifications). Both macrocycles could be facilely synthesized using an improved strategy through photo-induced ring expansions.39–42 They feature electron-deficient hydrophobic cavities with elongated shapes to accommodate the oversized electron-rich PS and show high affinity to HB by forming 1
:
1 host–guest complexes with binding constants of the 107 level in aqueous solutions. Notably, spatiotemporal monitoring of the HB delivery in the cellular environment reveals that the introduction of the two macrocycles leads to high PDT efficiency against cancer cells (IC50 as low as 0.35 μM) with distinct delivery effects. As shown in Fig. 1, the supramolecular PS, HB⊂AnBox4+, can enter the cells directly, while HB⊂ExAnBox4+ leaves the macrocyclic vehicle at the cell membrane and unloads HB into the cells.
 |
| Fig. 1 Macrocycles AnBox·4Cl and ExAnBox·4Cl as supramolecular receptors of photosensitizer HB with distinct delivery effects. | |
Results and discussion
Macrocycle design and synthesis
Because of notable features including water solubility, modifiable cavities, and electron-deficient π-conjugations, pyridinium-derived tetracationic cyclophanes43 are promising candidates as macrocyclic hosts for the hydrophobic conjugated HB. In addition, we envisioned that the incorporation of polycyclic aromatic moieties (e.g., 2,6-disubstituted anthracene) into the cyclophane scaffolds would not only introduce elongated cavities and fluorescent properties, but also provide extra anchors to bind HB through enhanced π–π interactions.44,45 Nonetheless, conventional syntheses of the elongated cyclophanes involve consecutive nucleophilic substitution reactions (Fig. 2, the routes with grey arrows), which commonly suffer from low cyclization yields due to entropy-driven polymerization by-products. To overcome the cyclization challenges, the underexplored anthracene [4 + 4] photodimer46–49 could serve as an X-shaped template to facilitate efficient dual cyclizations to form figure-of-eight macrocyclic intermediates, which would undergo late-stage ring expansion via photo-induced cycloreversion.39–42 With this approach, the anthracene moieties can be re-installed through reversible [4 + 4] photodimerization, at the same time affording tetracationic cyclophanes with extended cavities (Fig. 2, the routes with red arrows).
 |
| Fig. 2 Syntheses of AnBox·4Cl and ExAnBox·4Cl through photo-induced ring expansions. | |
Accordingly, the ring expansion approach toward AnBox4+, which had been first prepared by Stoddart through consecutive nucleophilic substitutions,50 commenced with [4 + 4]-photocycloaddition of bis(pyridyl)anthracene 1 (Fig. 2, top). The head-to-head product 4-HH was obtained as the predominant stereoisomer likely due to the π-stacking tendency of 1. Nucleophilic substitution between 4-HH and bis(benzyl bromide) 2 generated the figure-of-eight intermediate 5, forming two macrocycles in one step with 46% yield (82% yield per bond formation). Next, the photo-induced cycloreversion took place under 254 nm UV light irradiation and cleanly provided the hexafluorophosphate salt of AnBox4+ with 90% isolated yield. Notably, although this ring expansion approach involves one more step than the Stoddart's route,50 the higher overall yield reflects user-friendly purifications and improved synthetic efficiency.
Moreover, the other stereoisomer of the photodimer, the head-to-tail 4-HT, could be amenable to the ring expansion approach toward a more extended cyclophane ExAnBox4+ (Fig. 2, bottom). Because of the more flexible skeleton, synthetic attempts toward ExAnBox4+ through consecutive nucleophilic substitutions merely led to a minimal isoable macrocyclization product. As such, the advantages of the ring expansion approach have been further demonstrated. Under photo-induced [4 + 4]-cycloaddition conditions, the pinacol borate-derived anthracene was preferentially converted into the head-to-tail photodimer 8 due to steric effects of the bulky substitutions. Palladium-catalyzed Suzuki–Miyaura coupling between 8 and 4-bromopyridine smoothly afforded 4-HT. Compared to those in 4-HH, the pyridine moieties in 4-HT became more apart, thereby serving as matching reactive sites for longer cyclization fragments, namely the extended bis(benzyl bromide) 6. Dual macrocyclization between 4-HT and 6 afforded the figure-of-eight compound 9 with 40% yield (80% yield per bond formation). Likewise, the photo-induced cycloreversion of 9 generated the hexafluorophosphate salt of ExAnBox4+ with 95% isolated yield. Finally, anion exchange of the hexafluorophosphate salts of AnBox4+ and ExAnBox4+ provided the corresponding chlorides AnBox·4Cl and ExAnBox·4Cl, respectively, as water-soluble macrocycles.
Supramolecular host–guest complexes with HB
The facile preparations through the ring expansion approach secured rapid access to sufficient amounts of both macrocycles for supramolecular characterization. Indeed, due to the extended, electron-deficient cavities, both cationic macrocycles were identified as excellent hosts for HB which contains oversized π-conjugation as a guest molecule. In organic solvents such as acetonitrile, proton NMR signals displayed significant shifts upon mixing HB and AnBox4+ or ExAnBox4+ hexafluorophosphate (Fig. 3). For the guest complexation of each macrocycle, one set of broadened signals were observed, indicating fast exchange of host–guest equilibrium on the NMR time scale. Specifically, the resonances of central protons of both macrocycles (positions 1–5 of AnBox4+; positions 1′–5′ of ExAnBox4+) were considerably shifted toward the upfield direction likely due to the shielding effect of the HB guest, whereas the protons at the corners of the macrocycles (positions 6, 7, and a of AnBox4+; positions 6′–8′, a′, and b′ of ExAnBox4+) were just slightly affected by guest inclusion. Likewise, evident upfield shifts were observed for the resonances of the aromatic protons of HB, with less extent of changes for those of the peripheral methoxy groups. These results are in good accordance with the inclusion of HB inside the macrocyclic cavities of AnBox4+ and ExAnBox4+.
 |
| Fig. 3 Comparison of 1H NMR spectra (400 MHz, CD3CN, and 25 °C). | |
Based on the HB-binding properties of the two macrocycles in acetonitrile, we speculated that the corresponding water-soluble macrocycles containing chloride anions would interact with HB more tightly in aqueous solutions because of hydrophobic effects (cf. Table S1†).51 Indeed, further evidence obtained from UV-vis absorption in water suggested host–guest complex formation driven by strong non-covalent interactions, which considerably enhanced the water-solubility of HB. Upon addition of equimolar HB in the solid form to an aqueous solution of AnBox4+ (10 μM, Fig. 4a), the color of the solution changed from orange-yellow to dark red, and HB was completely dissolved to form a uniform solution. In parallel, the absorption of AnBox4+ at 455 nm was red-shifted to 465 nm, along with the appearance of a weak and broad band expanding to ca. 700 nm. By comparison, the solubility of neat HB is negligible in water, with the supernatant exhibiting minimal UV-vis absorption even after extended sonication (Fig. S23†). Mixing HB with AnBox4+ also significantly quenched the macrocycle's fluorescence intensity, which was utilized to quantitatively measure the guest binding stoichiometry and constants (Fig. 4b and c). According to fluorescence titration experiments, the mole ratio plot indicated that each AnBox4+ could bind one HB molecule, with a calculated binding constant of (1.73 ± 0.01) × 107 M−1 based on duplicate experiments. Similar results with regard to the UV-vis absorption and fluorescence changes were also observed for the other macrocycle ExAnBox4+ bearing a more extended cavity, exhibiting 1
:
1 host–guest stoichiometry and a comparable binding constant of (1.96 ± 0.40) × 107 M−1 in aqueous solution (Fig. 5a–c). In addition, the host–guest complexes for both macrocycles with HB were optimized by DFT calculations. Consistent with the NMR and photophysical characterization, the optimized structures showed that the flat HB molecule is surrounded by belt-like macrocycles with evident π–π interactions between the electron-rich conjugated guest and the electron-deficient central subunits of the macrocyclic hosts (Fig. 4d and 5d).
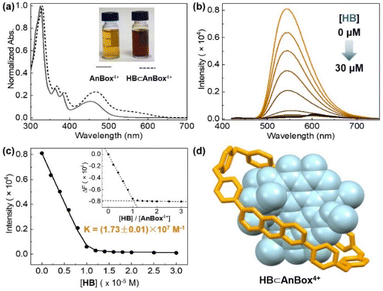 |
| Fig. 4 Photophysical and HB-binding properties of AnBox4+. (a) UV-vis absorption of AnBox4+ (10 μM in water) in the absence and presence of equimolar HB at 25 °C. (b) Fluorescence titration of AnBox4+ (10 μM in 20 : 80 DMSO/H2O, and λex = 350 nm) with HB at 25 °C. (c) Binding constant of HB⊂AnBox4+. (Inset) Mole ratio plot indicating a 1 : 1 stoichiometry. (d) Computationally optimized host–guest complex of HB⊂AnBox4+ at the B3LYP/6-31+G* level. | |
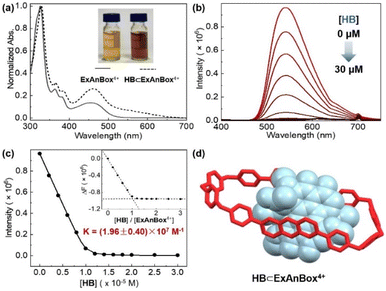 |
| Fig. 5 Photophysical and HB-binding properties of ExAnBox4+. (a) UV-vis absorption of ExAnBox4+ (10 μM in water) in the absence and presence of equimolar HB at 25 °C. (b) Fluorescence titration of ExAnBox4+ (10 μM in 20 : 80 DMSO/H2O and λex = 350 nm) with HB at 25 °C. (c) Binding constant of HB⊂ExAnBox4+. (Inset) Mole ratio plot indicating a 1 : 1 stoichiometry. (d) Computationally optimized host–guest complex of HB⊂ExAnBox4+ at the B3LYP/6-31+G* level. | |
Photoactivity test and stability of supramolecular photosensitizers HB⊂AnBox4+ and HB⊂ExAnBox4+
The chemical trapping method was applied to determine the reactive oxygen species (ROS) generation by the HB⊂AnBox4+ and HB⊂ExAnBox4+ complexes in response to light irradiation, employing 1,3-diphenylisobenzofuran (DPBF) and dihydrorhodamine 123 (DHR123) as singlet oxygen (1O2) and superoxide radical (O2˙−) trappers, respectively. As shown in Fig. 6a, in the presence of either HB⊂AnBox4+ or HB⊂ExAnBox4+, the absorbance of DPBF at 415 nm significantly decreased at steady rates under 532 nm laser irradiation, indicating DPBF degradation as a result of 1O2 generation. Furthermore, 532 nm laser irradiation could also induce evident fluorescence enhancement of DHR123 by 8.5 and 9.9 times in the presence of HB⊂AnBox4+ and HB⊂ExAnBox4+, respectively (Fig. 6b), which suggested effective production of O2˙−. These results indicated that the two supramolecular PSs can produce both 1O2 and O2˙−. Next, the stability evaluation showed that after continuous irradiation with a 532 nm laser (0.1 W cm−2 for 60 min), 96% and 98% of the absorbance intensities remained for HB⊂AnBox4+ and HB⊂ExAnBox4+, respectively, suggesting excellent photo-stability for both supramolecular PSs in aqueous solutions (Fig. 6c). Moreover, the aqueous solutions of HB⊂AnBox4+ and HB⊂ExAnBox4+ can be kept under ambient conditions for at least 7 days with more than 90% of their original absorbance intensities retained (Fig. 6d), indicating negligible aggregation or degradation after extended storage.
 |
| Fig. 6 (a) Normalized absorbance of DPBF at 415 nm as a function of irradiation time in the presence of AnBox4+, HB⊂AnBox4+, ExAnBox4+, and HB⊂ExAnBox4+ (20 μM in 50 : 50 DMSO/H2O) under 532 nm laser irradiation. (b) O2˙− detection of AnBox4+, HB⊂AnBox4+, ExAnBox4+, and HB⊂ExAnBox4+ (10 μM in water) using DHR123. (c) The photo-stability of HB⊂AnBox4+ and HB⊂ExAnBox4+ (10 μM in water) detected under 532 nm laser irradiation via absorbance spectra. (d) The stability of HB⊂AnBox4+ and HB⊂ExAnBox4+ (10 μM in water) detected via absorbance spectra. | |
In vitro imaging
To explore the cell uptake of the two supramolecular PSs, in vitro fluorescence imaging experiments using HeLa cells were performed. As control experiments, when the cells were treated with AnBox4+ or ExAnBox4+ in the absence of HB, obvious fluorescence signals were recorded at the cell membranes and became more intense during an extended incubation time (from 10 min to 30 min, Fig. 7a and b). At the same time, the cytoplasmic staining was not pronounced. The observed enrichment of the cationic macrocycles is consistent with their electrostatic interactions with the anionic cell surfaces. Interestingly, HB⊂AnBox4+ and HB⊂ExAnBox4+ exhibited different cellular uptake behaviors. The confocal images of HeLa cell incubated with HB⊂AnBox4+ show fluorescence throughout the cytoplasm (Fig. 7c), indicating that HB⊂AnBox4+ can easily permeate the cell membranes. Such observation was in stark contrast to the control experiments without HB (Fig. 7a), suggesting that the interactions between AnBox4+ and HB should be superior to those of AnBox4+ and the cell membranes. In parallel experiments, after the cells were incubated with HB⊂ExAnBox4+, the fluorescence of cell membranes in the TRITC channel (mainly contributed by the signals of the macrocycles) was stronger than that in the cytoplasm, while the opposite fluorescence intensities were observed in the Cy5 channel (mainly contributed by the signals of HB). This result indicated that when HB⊂ExAnBox4+ reaches cell surfaces, HB can be released from ExAnBox4+ and efficiently taken up into the cytoplasm, leaving ExAnBox4+ enriched in the cell membranes (Fig. 7d). The cell imaging results showed that the two supramolecular PSs have different cellular delivery behaviors for HB when entering the cells: AnBox4+ can enter the cells together with HB, whereas ExAnBox4+ can release HB at the cell membrane.
 |
| Fig. 7 Confocal images of HeLa cells incubated with (a) AnBox4+, (b) ExAnBox4+, (c) HB⊂AnBox4+, and (d) HB⊂ExAnBox4+. All concentrations are 10 μM in PBS. Scale bar: 20 μm. | |
In vitro photodynamic therapy
To detect the production of ROS in HeLa cells, the fluorescence probe of dichlorofluorescein diacetate (DCFH-DA) was used. The presence of ROS can transform the weakly fluorescent DCFH-DA into its oxidized form dichlorofluorescein (DCF) with strong green fluorescence. Upon laser irradiation, the DCFH-DA + HB⊂AnBox4+ and DCFH-DA + HB⊂ExAnBox4+ groups showed green fluorescence signals in HeLa cells, while free DCFH-DA, free HB⊂AnBox4+, and free HB⊂ExAnBox4+ as controls cannot induce green fluorescence (Fig. 8a). The above results suggested that HB can be efficiently carried by both macrocycles and effect photodynamic ROS generation.
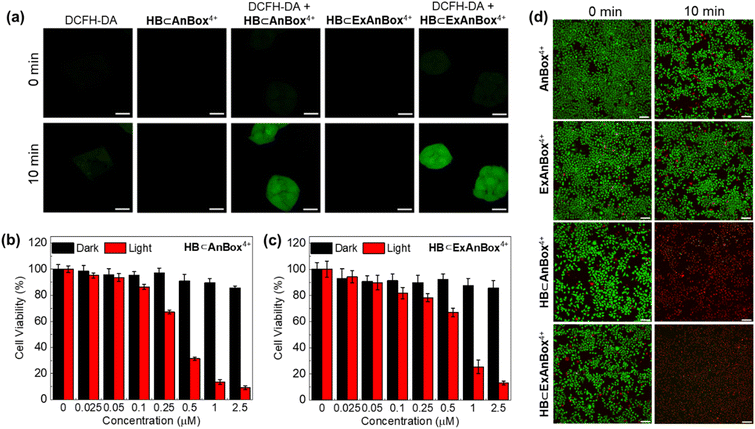 |
| Fig. 8 (a) Fluorescence images of ROS production detected by DCFH-DA in HeLa cells. Scale bar: 20 μm. Relative viability of HeLa cells incubated with (b) HB⊂AnBox4+ and (c) HB⊂ExAnBox4+ in the culture medium in the dark or under 532 nm laser irradiation (0.1 W cm−2). (d) Fluorescence images of the calcein AM and propidium iodide (PI) incubated with 2.5 μM AnBox4+, ExAnBox4+, HB⊂AnBox4+, and HB⊂ExAnBox4+, respectively, in the dark or under 532 nm laser irradiation (0.1 W cm−2). Scale bar: 100 μm. | |
Next, the biocompatibility and photo-toxicity properties of AnBox4+, ExAnBox4+, HB⊂AnBox4+, and HB⊂ExAnBox4+ against HeLa cells were evaluated by the 3-(4,5-dimethylthiazolyl-2)-2,5-diphenyltetrazolium bromide (MTT) assay. As shown in Fig. S35,† both AnBox4+ and ExAnBox4+ exhibited minimal cytotoxicity, demonstrating their high biocompatibility and low photo-toxicity under 532 nm laser irradiation. Furthermore, after incubation with HB⊂AnBox4+ in the dark, the cell viability remains over 80% at 2.5 μM (Fig. 8b). Upon 532 nm laser irradiation, the photo-toxicity of HB⊂AnBox4+ becomes evident, with the cell viability reduced to 15% at 2.5 μM. The half maximal inhibitory concentration (IC50) of HB⊂AnBox4+ was determined to be 0.35 μM, and that of HB⊂ExAnBox4+ (IC50 = 0.64 μM) is slightly higher (Fig. 8c). Calcein AM and propidium iodide (PI) co-staining assay was further used to verify the PDT efficacy by imaging live (green fluorescence) and dead (red fluorescence) cells. When irradiated with a 532 nm laser alone or treated with HB⊂AnBox4+ or HB⊂ExAnBox4+ in the dark, the cells retained a high survival rate (Fig. 8d and S36†). However, all cells died in the presence of either supramolecular PS under 532 nm laser irradiation for 10 min, which is consistent with the MTT experimental results. All of the above results indicated that AnBox4+ and ExAnBox4+ as HB carriers can achieve excellent photodynamic properties in living cells.
Conclusions
In summary, two fluorescent and hydrophilic cyclophanes, AnBox·4Cl and ExAnBox·4Cl, have been facilely synthesized employing a photo-induced ring expansion strategy, featuring extended macrocyclic cavities as supramolecular hosts for HB, an over-sized conjugated PS. Both macrocycles can make the hydrophobic HB soluble in water through host–guest complexation with excellent binding constants of the 107 level in aqueous solutions. The resulting supramolecular PSs show low toxicity in the dark, good stability, and notable photodynamic properties in living cancer cells by efficiently generating ROS under light irradiation. The fluorescent properties of the two macrocycles allow real-time monitoring of the PS delivery processes, revealing different cellular uptake behaviors of HB⊂AnBox4+ and HB⊂ExAnBox4+. We believe that this work showcases macrocyclic carriers of over-sized PSs for effective cellular delivery and ROS generation and would inspire future development for supramolecular photodynamic therapy.
Data availability
All experimental procedures, characterization data, photodynamic therapy related cell experiments, and computational data are available in the ESI.†
Author contributions
H. C., P. W., and W. L. conceived and directed the project. X. Z., S.-N. L., Z. G., and X. D. designed and carried out the experiments. H. X. performed DFT calculations. H. C., W. L., X. Z., P. W., C.-H. T., and L.-Z. W. analyzed data and prepared the manuscript with inputs from all authors.
Conflicts of interest
There are no conflicts to declare.
Acknowledgements
Financial support was provided by the National Natural Science Foundation of China (21922113, 61720106014, 22088102, 21988102, and 22071257), the Chinese Academy of Sciences (XDB17000000), and TIPC Director's Fund.
Notes and references
- J. Shi, P. W. Kantoff, R. Wooster and O. C. Farokhzad, Nat. Rev. Cancer, 2017, 17, 20–37 CrossRef CAS PubMed.
- M. T. Manzari, Y. Shamay, H. Kiguchi, N. Rosen, M. Scaltriti and D. A. Heller, Nat. Rev. Mater., 2021, 6, 351–370 CrossRef CAS PubMed.
- D. E. J. G. J. Dolmans, D. Fukumura and R. K. Jain, Nat. Rev. Cancer, 2003, 3, 380–387 CrossRef CAS PubMed.
- X. Zheng, J. Ge, J. Wu, W. Liu, L. Guo, Q. Jia, Y. Ding, H. Zhang and P. Wang, Biomaterials, 2018, 185, 133–141 CrossRef CAS PubMed.
- C. Ji, W. Cheng, Q. Yuan, K. Mullen and M. Yin, Acc. Chem. Res., 2019, 52, 2266–2277 CrossRef CAS PubMed.
- P. C. A. Swamy, G. Sivaraman, R. N. Priyanka, S. O. Raja, K. Ponnuvel, J. Shanmugpriya and A. Gulyani, Coord. Chem. Rev., 2020, 411, 213233 CrossRef.
- X. Zhao, J. Liu, J. Fan, H. Chao and X. Peng, Chem. Soc. Rev., 2021, 50, 4185–4219 RSC.
- Q. Xiao, J. Wu, X. Pang, Y. Jiang, P. Wang, A. W. Leung, L. Gao, S. Jiang and C. Xu, Curr. Med. Chem., 2018, 25, 839–860 CrossRef CAS PubMed.
- J. Wu, J. Sha, C. Zhang, W. Liu, X. Zheng and P. Wang, View, 2020, 1, 20200090 CrossRef.
- Y. N. Konan, R. Gurny and E. Allémann, J. Photochem. Photobiol., B, 2002, 66, 89–106 CrossRef CAS PubMed.
- X. Ma and Y. Zhao, Chem. Rev., 2015, 115, 7794–7839 CrossRef CAS PubMed.
- J. Zhou, G. Yu and F. Huang, Chem. Soc. Rev., 2017, 46, 7021–7053 RSC.
- M. J. Webber and R. Langer, Chem. Soc. Rev., 2017, 46, 6600–6620 RSC.
- H. Zhang, Z. Liu and Y. Zhao, Chem. Soc. Rev., 2018, 47, 5491–5528 RSC.
- P. Li, Y. Chen and Y. Liu, Chin. Chem. Lett., 2019, 30, 1190–1197 CrossRef CAS.
- J. Wankar, N. G. Kotla, S. Gera, S. Rasala, A. Pandit and Y. A. Rochev, Adv. Funct. Mater., 2020, 30, 1909049 CrossRef CAS.
- Y.-C. Pan, X.-Y. Hu and D.-S. Guo, Angew. Chem., Int. Ed., 2021, 60, 2768–2794 CrossRef CAS PubMed.
- W. Li, W. Xu, S. Zhang, J. Li, J. Zhou, D. Tian, J. Cheng and H. Li, J. Agric. Food Chem., 2022, 70, 12746–12759 CrossRef CAS PubMed.
- Z. Wang, C. Sun, K. Yang, X. Chen and R. Wang, Angew. Chem., Int. Ed., 2022, 61, e202206763 CAS.
- T. Sun, Y. S. Zhang, B. Pang, D. C. Hyun, M. Yang and Y. Xia, Angew. Chem., Int. Ed., 2014, 53, 12320–12364 CAS.
- H. Cabral, K. Miyata, K. Osada and K. Kataoka, Chem. Rev., 2018, 118, 6844–6892 CrossRef CAS PubMed.
- N. Panwar, A. M. Soehartono, K. K. Chan, S. Zeng, G. Xu, J. Qu, P. Coquet, K. T. Yong and X. Chen, Chem. Rev., 2019, 119, 9559–9656 CrossRef CAS PubMed.
- J. Gao, K. Dutta, J. Zhuang and S. Thayumanavan, Angew. Chem., Int. Ed., 2020, 59, 23466–23470 CrossRef CAS PubMed.
- M. Manzano and M. Vallet-Regí, Adv. Funct. Mater., 2020, 30, 1902634 CrossRef CAS.
- N. Rohaizad, C. C. Mayorga-Martinez, M. Fojtu, N. M. Latiff and M. Pumera, Chem. Soc. Rev., 2021, 50, 619–657 RSC.
- M. J. Mitchell, M. M. Billingsley, R. M. Haley, M. E. Wechsler, N. A. Peppas and R. Langer, Nat. Rev. Drug Discovery, 2021, 20, 101–124 CrossRef CAS PubMed.
- D. Ma, G. Hettiarachchi, D. Nguyen, B. Zhang, J. B. Wittenberg, P. Y. Zavalij, V. Briken and L. Isaacs, Nat. Chem., 2012, 4, 503–510 CrossRef CAS PubMed.
- T.-X. Zhang, Z.-Z. Zhang, Y.-X. Yue, X.-Y. Hu, F. Huang, L. Shi, Y. Liu and D.-S. Guo, Adv. Mater., 2020, 32, 1908435 CrossRef CAS PubMed.
- Y.-M. Zhang, Y.-H. Liu and Y. Liu, Adv. Mater., 2020, 32, 1806158 CrossRef CAS PubMed.
- W.-C. Geng, J. L. Sessler and D.-S. Guo, Chem. Soc. Rev., 2020, 49, 2303–2315 RSC.
- F. Schmitt, J. Freudenreich, N. P. Barry, L. Juillerat-Jeanneret, G. Suss-Fink and B. Therrien, J. Am. Chem. Soc., 2012, 134, 754–757 CrossRef CAS PubMed.
- I. Roy, S. Bobbala, R. M. Young, Y. Beldjoudi, M. T. Nguyen, M. M. Cetin, J. A. Cooper, S. Allen, O. Anamimoghadam, E. A. Scott, M. R. Wasielewski and J. F. Stoddart, J. Am. Chem. Soc., 2019, 141, 12296–12304 CrossRef CAS PubMed.
- K. Liu, Y. Liu, Y. Yao, H. Yuan, S. Wang, Z. Wang and X. Zhang, Angew. Chem., Int. Ed., 2013, 52, 8285–8289 CrossRef CAS PubMed.
- J. Gao, J. Li, W.-C. Geng, F.-Y. Chen, X. Duan, Z. Zheng, D. Ding and D.-S. Guo, J. Am. Chem. Soc., 2018, 140, 4945–4953 CrossRef CAS PubMed.
- X. Li, S. Lee and J. Yoon, Chem. Soc. Rev., 2018, 47, 1174–1188 RSC.
- H.-T. Feng, Y. Li, X. Duan, X. Wang, C. Qi, J. W. Y. Lam, D. Ding and B. Z. Tang, J. Am. Chem. Soc., 2020, 142, 15966–15974 CrossRef CAS PubMed.
- K. Yang, Z. Zhang, J. Du, W. Li and Z. Pei, Chem. Commun., 2020, 56, 5865–5876 RSC.
- N. Kwon, H. Kim, X. Li and J. Yoon, Chem. Sci., 2021, 12, 7248–7268 RSC.
- Z.-A. Huang, C. Chen, X.-D. Yang, X.-B. Fan, W. Zhou, C.-H. Tung, L.-Z. Wu and H. Cong, J. Am. Chem. Soc., 2016, 138, 11144–11147 CrossRef CAS PubMed.
- Z. Zhao, Q. Zhu, Z. Wang, J. Lu, Z. Jin and H. Liu, Macromolecules, 2017, 50, 8907–8915 CrossRef CAS.
- W. Xu, X.-D. Yang, X.-B. Fan, X. Wang, C.-H. Tung, L.-Z. Wu and H. Cong, Angew. Chem., Int. Ed., 2019, 58, 3943–3947 CrossRef CAS PubMed.
- Y. Ueda, K. Suzuki and K. Ohmori, Org. Lett., 2020, 22, 2002–2006 CrossRef CAS PubMed.
- X.-Y. Chen, H. Chen and J. F. Stoddart, Angew. Chem., Int. Ed., 2023, 62, e202211387 CAS.
- E. J. Dale, N. A. Vermeulen, M. Juricek, J. C. Barnes, R. M. Young, M. R. Wasielewski and J. F. Stoddart, Acc. Chem. Res., 2016, 49, 262–273 CrossRef CAS.
- I. Roy, A. H. G. David, P. J. Das, D. J. Pe and J. F. Stoddart, Chem. Soc. Rev., 2022, 51, 5557–5605 RSC.
- H. D. Becker, Chem. Rev., 1993, 93, 145–172 CrossRef CAS.
- H. Bouas-Laurent, J.-P. Desvergne, A. Castellan and R. Lapouyade, Chem. Soc. Rev., 2001, 30, 248–263 RSC.
- C. Yang and Y. Inoue, Chem. Soc. Rev., 2014, 43, 4123–4143 RSC.
- W. Liu, L. Guo, Y. Fan, Z. Huang and H. Cong, Chin. J. Org. Chem., 2017, 37, 543–554 CrossRef CAS.
- A. Garci, Y. Beldjoudi, M. S. Kodaimati, J. E. Hornick, M. T. Nguyen, M. M. Cetin, C. L. Stern, I. Roy, E. A. Weiss and J. F. Stoddart, J. Am. Chem. Soc., 2020, 142, 7956–7967 CrossRef CAS PubMed.
- A. Gu and N. J. Wheate, J. Inclusion Phenom. Macrocyclic Chem., 2021, 100, 55–69 CrossRef CAS.
Footnotes |
† Electronic supplementary information (ESI) available: General information, synthesis and characterization, host–guest chemistry, photodynamic therapy related cell experiments, and DFT calculations. See DOI: https://doi.org/10.1039/d3sc00107e |
‡ These authors contributed equally. |
|
This journal is © The Royal Society of Chemistry 2023 |
Click here to see how this site uses Cookies. View our privacy policy here.