DOI:
10.1039/D3SC01006F
(Edge Article)
Chem. Sci., 2023,
14, 5141-5147
ItOct (ItOctyl) – pushing the limits of ItBu: highly hindered electron-rich N-aliphatic N-heterocyclic carbenes†
Received
22nd February 2023
, Accepted 15th April 2023
First published on 18th April 2023
Abstract
ItBu (ItBu = 1,3-di-tert-butylimidazol-2-ylidene) represents the most important and most versatile N-alkyl N-heterocyclic carbene available in organic synthesis and catalysis. Herein, we report the synthesis, structural characterization and catalytic activity of ItOct (ItOctyl), C2-symmetric, higher homologues of ItBu. The new ligand class, including saturated imidazolin-2-ylidene analogues has been commercialized in collaboration with MilliporeSigma: ItOct, 929
298; SItOct, 929
492 to enable broad access of the academic and industrial researchers within the field of organic and inorganic synthesis. We demonstrate that replacement of the t-Bu side chain with t-Oct results in the highest steric volume of N-alkyl N-heterocyclic carbenes reported to date, while retaining the electronic properties inherent to N-aliphatic ligands, such as extremely strong σ-donation crucial to the reactivity of N-alkyl N-heterocyclic carbenes. An efficient large-scale synthesis of imidazolium ItOct and imidazolinium SItOct carbene precursors is presented. Coordination chemistry to Au(I), Cu(I), Ag(I) and Pd(II) as well as beneficial effects on catalysis using Au(I), Cu(I), Ag(I) and Pd(II) complexes are described. Considering the tremendous importance of ItBu in catalysis, synthesis and metal stabilization, we anticipate that the new class of ItOct ligands will find wide application in pushing the boundaries of new and existing approaches in organic and inorganic synthesis.
Introduction
ItBu (ItBu = 1,3-di-tert-butylimidazol-2-ylidene) is the most useful and most general bulky N-alkyl N-heterocyclic carbene in organic synthesis and catalysis (Fig. 1A, 1).1–3 The importance of ItBu is reflected by the numerous applications in transition-metal-catalysis using an entire palette of metals and transformations. The extraordinary high utility of ItBu stems from the large steric volume (%Vbur = 39.6%; %Vbur = %buried volume, [Au(ItBu)Cl]) provided by the bulky t-Bu group at the N-wingtip.4 Simultaneously, the electron-donating N-alkyl groups engender the ligand with strong σ-donation (TEP, 2049 cm−1, [Rh(ItBu)(CO)2Cl]) and high π-acceptance (77Se NMR, δSe, 183 ppm, [Se(ItBu)]), which supersede the values observed for N-aromatic NHCs.5 Overall, this results in a unique NHC scaffold that has become an indispensable part of the synthetic, organometallic and inorganic toolbox, while providing direct access to novel reactivity, and is now routinely utilized in metal stabilization, reaction screening and optimization. ItBu imidazolium precursor is now commercially available from several suppliers as Cl or BF4 salts (CAS: 157197-54-1; CAS: 263163-17-3).6,7
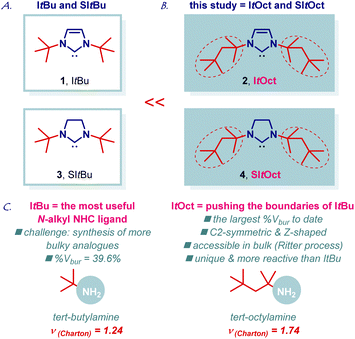 |
| Fig. 1 (A–C) Pushing the limits of sterically-demanding N-alkyl-heterocyclic carbenes: moving beyond ItBu. ItOct and SItOct are commercially available from MilliporeSigma: ItOct, 929 298; SItOct, 929 492. | |
As part of our program in transition-metal-catalysis,8,9 herein we report the synthesis, structural characterization, and catalytic activity of ItOct (ItOctyl) class of ligands, which are C2-symmetric, higher homologues of ItBu (Fig. 1B, 2). The new ligand class, including saturated imidazolin-2-ylidene analogue, has been commercialized in collaboration with MilliporeSigma: ItOct, 929
298; SItOct, 929
492, to enable broad access of academic and industrial researchers.10 We demonstrate that ItBu to ItOct exchange results in the highest steric volume reported to date for N-alkyl N-heterocyclic carbenes (%Vbur = 44.7%), while retaining electronic properties inherent to N-alkyl ligands, such as extremely strong σ-donation and π-acceptance.4,5 Notably, the steric volume of ItOct matches the values observed for the archetypal N-aromatic NHC ligands for the first time (IPr, %Vbur = 45.4%; IMes, %Vbur = 36.5%, [Au(NHC)Cl]). ItOct features a unique C2-symmetric and Z-shape scaffold.11 The saturated congener, SItOct (Fig. 1B, 4), is homologous to SItBu (Fig. 1A, 3). Large scale synthesis, coordination chemistry to Au(I), Cu(I), Ag(I) and Pd(II), structure and electronic properties of the carbene center as well as beneficial effects on catalysis using Au(I), Cu(I), Ag(I) and Pd(II) complexes are described. Considering the tremendous importance and utility of ItBu in catalysis, synthesis and metal stabilization1–3 we anticipate that the new class of ItOct ligands will find wide application in pushing the limits of N-alkyl N-heterocyclic carbenes in organic and inorganic synthesis.
Results and discussion
The chemistry of N-bulky NHC ligands has been studied in a wide array of contexts, including catalysis, coordination chemistry and stabilization of reactive metal centers.11,12 We reasoned that an increase in sterics as measured by the Charton parameter (t-Bu, ν = 1.24; t-Oct, ν = 1.74, Fig. 1C) would result in an attractive new class of N-aliphatic bulky NHC ligands. As a key element of our design, we recognized that tert-octylamine is considerably cheaper than other bulky amines and readily available on kg scale by the Ritter process of the isomeric 2,2,4-trimethylpentenes,13,14 which are commercially produced from isobutene feedstock.
We initiated our studies by developing a flexible and robust synthesis of ItOct imidazolium precursor using the readily available tert-octylamine13–15 as the starting material (Scheme 1A). As shown in Scheme 1, the optimized synthesis of ItOct precursor proceeds in cost-effective, chromatography-free, and straightforward manner. Thus, condensation of tert-octylamine with glyoxal at room temperature and cyclization of the diimine using a combination of HCl/(CH2O)n in toluene at 60 °C delivered the desired ItOct as HCl salt after simple filtration, allowing for a routine preparation of gram quantities of the product (step 1: 98% yield, 61 mmol scale; step 2: 73% yield, 10 mmol scale). The synthesis of ItOct as HBF4 salt was optimized to proceed in 82% yield (Scheme 1B), while a one-step procedure was developed using the combination of tert-octylamine, HBF4/(CH2O)n and glyoxal in 58% yield (see ESI†). The synthesis of SItOct as HCl salt was accomplished by the reduction of diimine 7 to the diamine using NaBH4 in MeOH/THF at room temperature (91% yield, 18 mmol scale) and cyclization to the imidazolinium SItOct salt using a combination of HC(OEt)3/HCO2H at 125 °C (77% yield, 5 mmol scale) (Scheme 1C). It should be noted that the synthesis is highly practical and allows for the isolation of ItOct·HCl, ItOct·HBF4 and SItOct·HCl by simple filtration and recrystallization from the reaction mixtures.
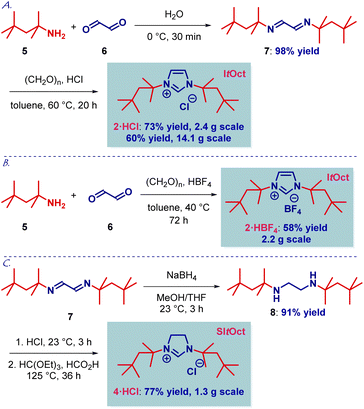 |
| Scheme 1 Synthesis of ItOct, SItOct and precursors. Conditions: (A) 5 (1.0 equiv.), (CHO)2 (40%, aq. 0.5 equiv.), H2O, 0 °C, then (CH2O)n (1.0 equiv.), HCl (4.0 M, dioxane, 1.0 equiv.), toluene, 60 °C. (B) 7 (1.0 equiv.), (CH2O)n (1.0 equiv.), HBF4 (48% aq. 1.0 equiv.), toluene, 40 °C; one-step: 5 (1.2 equiv.), (CH2O)n (1.0 equiv.), HBF4 (48% aq. 1.0 equiv.), toluene, 40 °C. (C) 7 (1.0 equiv.), NaBH4 (8.0 equiv.), MeOH/THF, 23 °C, then HCl, 23 °C, HC(OEt)3 (10.0 equiv.), HCO2H, 125 °C. | |
With facile access to ItOct in hand, we next focused on comprehensive evaluation of steric and electronic properties of this novel NHC ligand (Scheme 2A). As shown in Scheme 2, the gold complex [Au(ItOct)Cl] (9) was prepared using LiHMDS/THF, while the method using K2CO3/acetone gave lower yields.16 Moreover, [Ag(ItOct)Cl] (10) and [Cu(ItOct)Cl] (11) were prepared using Ag2O/CuCl and K2CO3 in 1,4-dioxane at 80 °C.17 The selenium adduct [Se(ItOct)] (12) was synthesized using selenium/K2CO3 at 80 °C,18 while the Pd(II) complexes [Pd(ItOct)(allyl)Cl] (13) and [Pd(ItOct)(3-Cl-py)Cl2] (14) were prepared from the palladium allyl dimer [{Pd(allyl)(μ-Cl)}2] and PdCl2/3-Cl-py in the presence of LiHMDS and K2CO3, respectively.19,20 It should be noted that NHC salts (2) and (4) as well as all products 9–14 were found to be stable to air and moisture. Complexes 9–12 and 14 were fully characterized by X-ray crystallography (Fig. 2, 3, and ESI†).21
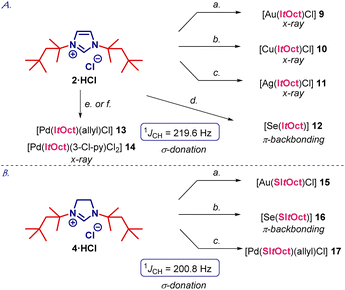 |
| Scheme 2 Synthesis of ItOct and SItOct complexes. Conditions: (A) (a) AuCl·Me2S (1.0 equiv.), LiHMDS (1.1 equiv.), THF, 23 °C, 15 h, 84%. (b) CuCl (2.0 equiv.), K2CO3 (3.0 equiv.), dioxane, 80 °C, 15 h, 76%. (c) Ag2O (2.0 equiv.), K2CO3 (3.0 equiv.), dioxane, 80 °C, 15 h, 80%. (d) Se (2.0 equiv.), K2CO3 (3.0 equiv.), dioxane, 80 °C, 15 h, 75%. (e) LiHMDS (1.1 equiv.), [Pd(allyl)Cl]2 (1.0 equiv.), THF, 23 °C, 15 h, 89%. (f) PdCl2 (1.0 equiv.), K2CO3 (3.0 equiv.), 3-Cl-py, 80 °C, 15 h, 76%. (B) (a) AuCl·Me2S (1.0 equiv.), LiHMDS (1.1 equiv), THF, 23 °C, 15 h, 81%. (b) Se (2.0 equiv.), KOt-Bu (3.0 equiv.), THF, 23 °C, 15 h, 69%. (c) LiHMDS (1.1 equiv.), [Pd(allyl)Cl]2 (1.0 equiv.), THF, 23 °C, 15 h, 74%. | |
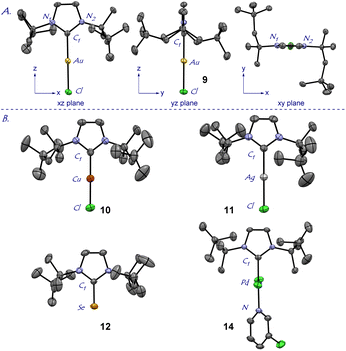 |
| Fig. 2 X-ray crystal structures of complexes 9–12, 14. (A) 9: Views along three axes are shown. (B) 10–12, 14. Hydrogen atoms have been omitted for clarity. See ESI† for bond lengths [Å], angles and expanded structures. Crystallographic data have been deposited with the Cambridge Crystallographic Data Center. CCDC 2239373 (9); CCDC 2239374 (10); CCDC 2239375 (11); CCDC 2239376 (12); CCDC 2239377 (14). | |
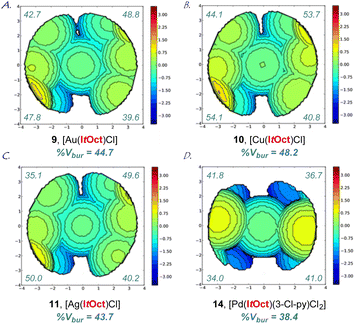 |
| Fig. 3 (A–D) Topographical steric maps of [Au(ItOct)Cl] (9), [Cu(ItOct)Cl] (10), [Ag(ItOct)Cl] (11) and [Pd(ItOct)(3-Cl-py)Cl2] (14) showing %Vbur per quadrant. See ESI† for additional details. | |
The X-ray structure of [Au(ItOct)Cl] (9) revealed a unique C2-symmeric and Z-shape arrangement of N-alkyl substituents with a linear (C–Au–Cl, 179.9°; C–Au, 2.007 Å) geometry (Fig. 2A). The % buried volume (%Vbur) of [Au(ItOct)Cl] is 44.7%. Crucially, [Au(ItOct)Cl] represents the most bulky N-alkyl NHC ligand reported to date.4,5 This value can be compared with the (%Vbur) of 39.6% determined for [Au(ItBu)Cl].4b Furthermore, it should be noted that the gem-Me2 substitution22 of the longer tert-Oct side-chain places the metal within the pocket formed by the alkyl side chain. The steric mapping of the metal center23 in [Au(ItOct)Cl] is shown in Fig. 3 (see Fig. 5 and ESI† for comparison between [M(ItOct)X] and [M(ItBu)X]). It should be noted that ItOct is large and flexible, while the %Vbur determined by XRD represent local minima in terms of energies.
Complexes [Ag(ItOct)Cl] (10), [Cu(ItOct)Cl] (11), [Se(ItOct)] (12) and [Pd(ItOct)(3-Cl-py)Cl2] (14) were also fully characterized by X-ray crystallography (Fig. 2, 3 and ESI†). The summary of structural parameters is presented in the ESI.† Importantly, the %buried volume (%Vbur) of linear [Ag(ItOct)Cl] (10), [Cu(ItOct)Cl] (11), and [Se(ItOct)] (12) of 43.7%, 48.2% and 44.1%, attests to the immense steric impact of the ItOct substitution. The (%Vbur) of square planar [Pd(ItOct)(3-Cl-py)Cl2] (14) is lower of 38.4%, which demonstrates the capacity of the tert-octyl side chains to adjust to the steric impact of the metal center (see ESI†).11
The selenourea adduct [Se(ItOct)] (12) permits to gauge π-backbonding of ItOct from the 77Se NMR spectra.18 The δSe value of 216.7 ppm for [Se(ItOct)] (CDCl3) indicates that ItOct is more π-accepting than ItBu (δSe, 183 ppm, CDCl3). Furthermore, 1JCH coupling constant from the 13C satellites of 1H NMR spectra of 219.60 Hz for ItOct·HCl (CDCl3) gives an accurate indication of σ-donation,24 and indicates that this ligand is more strongly donating than N-aryl ligands, such as IPr (1JCH = 223.70 Hz; cf.ItBu: 1JCH = 219.35 Hz).
We also performed the synthesis of representative complexes using the imidazolinium precursor SItOct (Scheme 2B). The synthesis of [Au(SItOct)Cl] (15), [Se(SItOct)] (16) and [Pd(ItOct)(allyl)Cl] (17) proceeded smoothly under the conditions developed for ItOct·HCl (Scheme 2A). The δSe value of 298.2 ppm and the 1JCH value of 200.80 Hz indicate an increased π-acceptance and σ-donation of the saturated imidazolin-2-ylidene SItOct, as expected.4,5
From the outset, we proposed that the increased steric bulk of the ItOct ligand would be beneficial on transition-metal-catalysis. To demonstrate the effect of increased steric substitution, we performed several representative reactions in Au(I), Cu(I), Ag(I) and Pd(0) catalysis (Scheme 3). For direct comparison, the corresponding [ItBu–M] complexes were prepared and tested in parallel. As shown, the performance of [Au(ItOct)Cl], [Cu(ItOct)Cl], [Ag(ItOct)Cl], [Pd(ItOct)(3-Cl-py)Cl2] in Au(I)-catalyzed hydration,25 Cu(I)-catalyzed hydroboration,26 Ag(I)-catalyzed hydroboration,27 Cu(I)-catalyzed C–O coupling28 and Pd(0)-catalyzed C–C and C–N coupling29 supersede the analogous [ItBu–M] complexes.
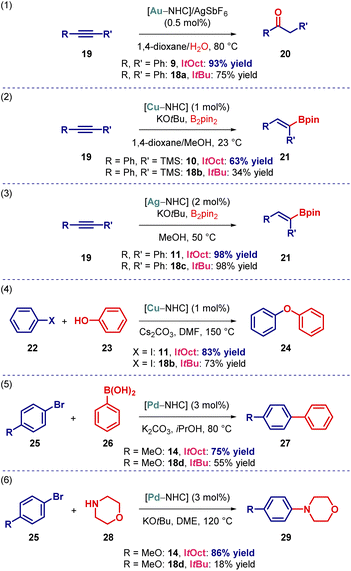 |
| Scheme 3 Activity of [ItOct–M] in catalysis. See ESI† for additional details. | |
These highly promising preliminary studies provide a strong support for the routine addition of the ItOct class of ligands to the toolbox for reaction screening. Further, it is expected that the ItOct to ItBu replacement will have an even greater effect on stabilizing reactive metal centers by metal shielding.1–3 Studies in this direction are currently underway and will be reported in due course.
To gain further insight into the electronic structure of the ItOct class of ligands, we determined HOMO and LUMO energy levels at the B3LYP 6-311++g(d,p) level (Fig. 4 and ESI†). It is well established that computed HOMO and LUMO provide the most accurate estimation of nucleophilicity and electrophilicity of NHC ligands.5,6 The HOMO of ItOct (−5.68 eV) is in the same range as ItBu (−5.67 eV), which is much higher than for the archetypal IPr30 (−6.01 ev). The HOMO of SItOct is even higher (−5.50 eV), which can be compared with SItBu (−5.46 eV). The π-accepting orbital (LUMO+2 due to required symmetry) of ItOct (0.06 eV) and SItOct (−0.04 eV) are comparable to ItBu (0.36 eV) and SItBu (0.14 eV), which could be compared with IPr (−0.48 eV). Overall, these results confirm ItOct as strongly σ-nucleophilic and sterically-bulky ligands with electronics matching those of ItBu.
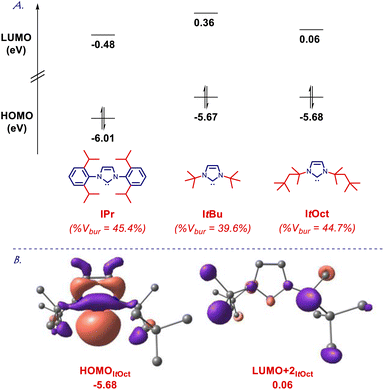 |
| Fig. 4 (A) HOMO and LUMO energy levels (eV). (B) HOMO and LUMO+2 (eV) of ItOct calculated at B3LYP 6-311++g(d,p). See ESI.† | |
Furthermore, to eliminate impact from steric packing, we have determined the (%Vbur) for the linear [Cu(NHC)Cl] complexes at the B3LYP 6-311++g(d,p) level (NHC = ItOct, ItBu, SItOct, SItBu, Fig. 5 and ESI†). The accurate determination of the computed linear geometry obviates effects from crystal packing.5,6 [Cu(I)–NHC] complexes were selected to facilitate computations. The %Vbur of ItOct (45.1%), ItBu (41.0%), SItOct (47.1%), SItBu (41.7%) confirm the effects observed in the X-ray analysis and clearly demonstrate the increased steric demand and unique C2-symmetric Z-shape of ItOct ligands.
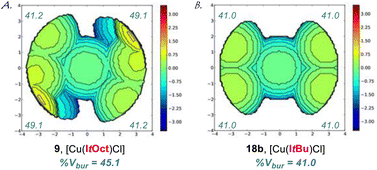 |
| Fig. 5 (A and B) Topographical steric maps of [Cu(ItOct)Cl] (9) and [Cu(ItBu)Cl] (18b) showing %Vbur per quadrant determined at the B3LYP 6-311++g(d,p) level. See ESI† for additional details. Note the steric difference between the ItOct and ItBu ligands. | |
Interestingly, we found that there is a very good linear correlation between the (%Vbur) and the steric Charton parameter (ν)31 (Fig. 6) using linear [Au(NHC)Cl] complexes. This finding further establishes ItOct as the most sterically-demanding N-alkyl NHC ligands. The present correlation appears to be general and can be used for the future determination of steric impact of N-alkyl-substituted NHC ligands. Finally, it should be noted that the expensive yet extremely useful bulky adamantyl (IAd)32 is much smaller in volume than ItOct (%Vbur = 39.8%, IAd vs. 44.7%, ItOct).
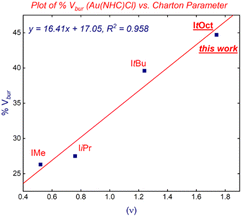 |
| Fig. 6 Plot of %Vburvs. Charton parameter in [Au(NHC)Cl] complexes. Note that ItOct is the most sterically-demanding N-alkyl-NHC to date. | |
Conclusions
In summary, we have reported ItOct (ItOctyl) class of ligands that push the limits of ItBu, which is the most useful N-alkyl NHC ligand developed to date in various facets of organic and inorganic synthesis. The ItOct class of ligands is characterized by the highest steric volume reported to date for N-aliphatic NHC ligands, while exploiting extremely strong σ-donating electronic properties inherent to N-alkyl N-heterocyclic carbenes. The facile preparation of ItOct has been developed using tert-octylamine as a product of downstream conversion of feedstock isobutene, which allows for rapid and cost-effective synthesis of ItOct ligands. This route enables routine access and commercial availability. Further, the ItOct class of ligands feature a unique C2-symmetric Z-shaped steric architecture, making it attractive for future development of strongly σ-donating carbenes. Considering the tremendous importance of N-aliphatic ligands and the commercial availability of the ItOct ligands (MilliporeSigma: ItOct, 929
298; SItOct, 929
492),10 we anticipate that ItOct ligands will find wide application in pushing the boundaries of new and existing approaches in organic and inorganic synthesis.
Data availability
Experimental procedures, characterization data, crystallographic and computational details are available in the ESI.†
Author contributions
M. R. developed the ligands and performed the experiments. G. M. was involved in ligand design. E. B. and B. D. provided crystallographic analysis. R. L. was involved in crystallographic studies. R. S. performed DFT computations. M. S. conceived and directed the project and wrote the manuscript.
Conflicts of interest
The authors declare the following competing financial interests: Rutgers University has filed patents on ligands and precatalysts described in this manuscript (US 63/155492, Mar 2, 2021).
Acknowledgements
We thank Rutgers University (M. S.), the NSF (CAREER CHE-1650766, M. S.), and the NIH (R35GM133326, M. S.) for generous financial support. The Bruker 500 MHz spectrometer used in this study was supported by the NSF-MRI grant (CHE-1229030). Additional support was provided by the Rutgers Graduate School in the form of Dean’s Dissertation Fellowship (M. R. and G. M.). Supplement funding for this project was provided by the Rutgers University – Newark Chancellor's Research Office. We thank the Wroclaw Center for Networking and Supercomputing (grant number WCSS159). E. B. acknowledges Narodowe Centrum Nauki (grant no. 2019/35/D/ST4/00806, E. B.) for generous financial support.
Notes and references
-
Science of Synthesis: N-Heterocyclic Carbenes in Catalytic Organic Synthesis, ed. S. P. Nolan and C. S. J. Cazin, Thieme, Stuttgart, 2017 Search PubMed.
-
(a) M. N. Hopkinson, C. Richter, M. Schedler and F. Glorius, Nature, 2014, 510, 485–496 CrossRef CAS PubMed;
(b)
N-Heterocyclic Carbenes, ed. S. P. Nolan, Wiley, Weinheim, 2014 Search PubMed;
(c) G. C. Fortman and S. P. Nolan, Chem. Soc. Rev., 2011, 40, 5151–5169 RSC;
(d) S. Diez-Gonzalez, N. Marion and S. P. Nolan, Chem. Rev., 2009, 109, 3612–3676 CrossRef CAS PubMed;
(e)
N-Heterocyclic Carbenes: From Laboratory Curiosities to Efficient Synthetic Tools, ed. S. Diez-Gonzalez, RSC, Cambridge, 2016 Search PubMed;
(f)
H. V. Huynh, The Organometallic Chemistry of N-Heterocyclic Carbenes, Wiley, Hoboken, 2017 CrossRef;
(g)
N-Heterocyclic Carbenes in Transition Metal Catalysis, ed. C. S. J. Cazin, Springer, New York, 2011 Search PubMed;
(h) F. Glorius, Top. Organomet. Chem., 2007, 21, 1–231 CrossRef CAS PubMed;
(i) S. Würtz and F. Glorius, Acc. Chem. Res., 2008, 41, 1523–1533 CrossRef PubMed;
(j) E. A. B. Kantchev, C. J. O. O'Brien and M. G. Organ, Angew. Chem., Int. Ed., 2007, 46, 2768–2813 CrossRef CAS PubMed;
(k) W. A. Hermann, Angew. Chem., Int. Ed., 2002, 41, 1290–1309 CrossRef;
(l) E. Peris, Chem. Rev., 2018, 118, 9988–10031 CrossRef CAS PubMed;
(m) G. Sipos and R. Dorta, Coord. Chem. Rev., 2018, 375, 13–68 CrossRef CAS;
(n) M. Iglesias and L. A. Oro, Chem. Soc. Rev., 2018, 47, 2772–2808 RSC;
(o) D. Munz, Organometallics, 2018, 37, 275–289 CrossRef CAS;
(p) A. A. Danopoulos, T. Simler and P. Braunstein, Chem. Rev., 2019, 119, 3730–3961 CrossRef CAS PubMed;
(q) Q. Zhao, G. Meng, S. P. Nolan and M. Szostak, Chem. Rev., 2020, 120, 1981–2048 CrossRef CAS PubMed.
-
(a) G. Pisano and C. S. J. Cazin, Green Chem., 2020, 22, 5253–5256 RSC;
(b) T. Scattolin, N. V. Tzouras, L. Falivene, L. Cavallo and S. P. Nolan, Dalton Trans., 2020, 49, 9694–9700 RSC;
(c) K. B. Smith and M. K. Brown, J. Am. Chem. Soc., 2017, 139, 7721–7724 CrossRef CAS PubMed;
(d) K. Kubota, M. Uesugi, S. Osaki and H. Ito, Org. Biomol. Chem., 2019, 17, 5680–5683 RSC;
(e) R. Guo, X. Huang, M. Zhao, Y. Lei, Z. Ke and L. Kong, Inorg. Chem., 2019, 58, 13370–13375 CrossRef CAS PubMed;
(f) B. Hupp, J. Nitsch, T. Schmitt, R. Bertermann, K. Edkins, F. Hirsch, I. Fischer, M. Auth, A. Sperlich and A. Steffen, Angew. Chem., Int. Ed., 2018, 57, 13671–13675 CrossRef CAS PubMed;
(g) Z. C. Cao, Q. Y. Luo and Z. J. Shi, Org. Lett., 2016, 18, 5978–5981 CrossRef CAS PubMed;
(h) J. Zhang, J. Xu, Y. Xu, H. Sun, Q. Shen and Y. Zhang, Organometallics, 2015, 34, 5792–5800 CrossRef CAS;
(i) Y. Hoshimoto, Y. Hayashi, H. Suzuki, M. Ohashi and S. Ogoshi, Angew. Chem., Int. Ed., 2012, 51, 10812–10815 CrossRef CAS PubMed;
(j) M. McGraw and E. Y. X. Chen, ACS Catal., 2018, 8, 9877–9887 CrossRef CAS;
(k) Y. Zhang, M. Schmitt, L. Falivene, L. Caporaso, L. Cavallo and E. Y. X. Chen, J. Am. Chem. Soc., 2013, 135, 17925–17942 CrossRef CAS PubMed;
(l) A. T. Papastavrou, M. Pauze, E. Gómez-Bengoa and G. C. Vougioukalakis, ChemCatChem, 2019, 11, 5379–5386 CrossRef CAS;
(m) S. Li, Z. Tang, Y. Wang, D. Wang, Z. Wang, C. Yu, T. Li, D. Wei and C. Yao, Org. Lett., 2019, 21, 1306–1310 CrossRef CAS PubMed;
(n) M. K. Denk, N. S. Milutinovic, K. M. Marczenko, N. M. Sadowski and A. Paschos, Chem. Sci., 2017, 8, 1883–1887 RSC;
(o) G. Tintori, P. Nabokoff, R. Buhaibeh, D. Bergé-Lefranc, S. Redon, J. Broggi and P. Vanelle, Angew. Chem., Int. Ed., 2018, 57, 3148–3153 CrossRef CAS PubMed.
-
(a) H. Clavier and S. P. Nolan, Chem. Commun., 2010, 46, 841–861 RSC;
(b) A. Gomez-Suarez, D. J. Nelson and S. P. Nolan, Chem. Commun., 2017, 53, 2650–2660 RSC.
-
(a) S. Diez-Gonzalez and S. P. Nolan, Coord. Chem. Rev., 2007, 251, 874–883 CrossRef CAS;
(b) H. Jacobsen, A. Correa, A. Poater, C. Costabile and L. Cavallo, Coord. Chem. Rev., 2009, 253, 687–703 CrossRef CAS;
(c) T. Dröge and F. Glorius, Angew. Chem., Int. Ed., 2010, 49, 6940–6952 CrossRef PubMed;
(d) D. J. Nelson and S. P. Nolan, Chem. Soc. Rev., 2013, 42, 6723–6753 RSC;
(e) H. V. Huynh, Chem. Rev., 2018, 118, 9457–9492 CrossRef CAS PubMed.
-
(a) D. Martin, M. Melaimi, M. Soleilhavoup and G. Bertrand, Organometallics, 2011, 30, 5304–5313 CrossRef CAS PubMed;
(b) M. Melaimi, M. Soleihavoup and G. Bertrand, Angew. Chem., Int. Ed., 2010, 49, 8810–8849 CrossRef CAS PubMed;
(c) M. Soleilhavoup and G. Bertrand, Acc. Chem. Res., 2015, 48, 256–266 CrossRef CAS PubMed;
(d) U. S. D. Paul and U. Radius, Eur. J. Inorg. Chem., 2017, 3362–3375 CrossRef CAS;
(e) J. Cheng, L. Wang and L. Deng, Chem. Rev., 2018, 118, 9930–9987 CrossRef CAS PubMed;
(f) V. M. Chernyshev, E. A. Denisova, D. B. Eremin and V. P. Ananikov, Chem. Sci., 2020, 11, 6957–6977 RSC;
(g) M. Soleilhavoup and G. Bertrand, Chem, 2020, 6, 1275–1282 CrossRef CAS.
-
(a) L. Benhamou, E. Chardon, G. Lavigne, S. Bellemin-Laponnaz and V. Cesar, Chem. Rev., 2011, 111, 2705–2733 CrossRef CAS PubMed;
(b) D. Nelson, Eur. J. Inorg. Chem., 2015, 2012–2027 CrossRef CAS;
(c) T. Scattolin and S. P. Nolan, Trends Chem., 2020, 2, 721–736 CrossRef CAS.
-
(a) T. Zhou, S. Ma, F. Nahra, A. M. C. Obled, A. Poater, L. Cavallo, C. S. J. Cazin, S. P. Nolan and M. Szostak, iScience, 2020, 23, 101377 CrossRef CAS PubMed;
(b) S. Shi, S. P. Nolan and M. Szostak, Acc. Chem. Res., 2018, 51, 2589–2599 CrossRef CAS PubMed;
(c) P. Lei, G. Meng and M. Szostak, ACS Catal., 2017, 7, 1960–1965 CrossRef CAS;
(d) P. Lei, G. Meng, S. Shi, Y. Ling, J. An, R. Szostak and M. Szostak, Chem. Sci., 2017, 8, 6525–6530 RSC;
(e) Q. Zhao, G. Meng, G. Li, C. Flach, R. Mendelsohn, R. Lalancette, R. Szostak and M. Szostak, Chem. Sci., 2021, 12, 10583–10589 RSC;
(f) Q. Xia, S. Shi, P. Gao, R. Lalancette, R. Szostak and S. Szostak, J. Org. Chem., 2021, 86, 15648–15657 CrossRef CAS PubMed;
(g) J. Zhang, X. Li, T. Li, G. Zhang, K. Wan, Y. Ma, R. Fang, R. Szostak and M. Szostak, ACS Catal., 2022, 12, 15323–15333 CrossRef CAS;
(h) J. Zhang, T. Li, X. Li, A. Lv, X. Li, Z. Wang, R. Wang, Y. Ma, R. Fang, R. Szostak and M. Szostak, Commun. Chem., 2022, 5, 60 CrossRef CAS PubMed;
(i) P. Gao, J. Xu, T. Zhou, Y. Liu, E. Bisz, B. Dziuk, R. Lalancette, R. Szostak, D. Zhang and M. Szostak, Angew. Chem., Int. Ed., 2023, 62, e202218427 CrossRef CAS PubMed.
-
(a) G. Li, S. Ma and M. Szostak, Trends Chem., 2020, 2, 914–928 CrossRef CAS;
(b) G. Meng, S. Shi, R. Lalancette, R. Szostak and M. Szostak, J. Am. Chem. Soc., 2018, 140, 727–734 CrossRef CAS PubMed;
(c) S. Shi, G. Meng and M. Szostak, Angew. Chem., Int. Ed., 2016, 55, 6959–6963 CrossRef CAS PubMed;
(d) G. Meng and M. Szostak, Angew. Chem., Int. Ed., 2015, 54, 14518–14522 CrossRef CAS PubMed.
-
(a)
M. Szostak and M. Rahman, Sterically Hindered N-Aliphatic N-Heterocyclic Carbene Catalysts and Methods Using Same, US Pat., 63/155492, 2021;
(b)
https://sigmaladrich.com/US/en/product/aldrich/929298, accessed on Feb 22, 2023;
(c)
https://sigmaladrich.com/US/en/product/aldrich/929492, accessed on Feb 22, 2023.
-
(a) F. Izquierdo, S. Manzini and S. P. Nolan, Chem. Commun., 2014, 50, 14926–14937 RSC;
(b) G. Altenhoff, R. Goddard, C. W. Lehmann and F. Glorius, J. Am. Chem. Soc., 2004, 126, 15195–15201 CrossRef CAS PubMed;
(c) V. Lavallo, Y. Canac, C. Präsang, B. Donnadieu and G. Bertrand, Angew. Chem., Int. Ed., 2005, 44, 5705 CrossRef CAS PubMed;
(d) M. Yamashita, K. Goto and T. Kawashima, J. Am. Chem. Soc., 2005, 127, 7294–7295 CrossRef CAS PubMed;
(e) G. Berthon-Gelloz, M. A. Siegler, A. L. Spek, B. Tinant, J. N. H. Reek and I. E. Marko, Dalton Trans., 2010, 39, 1444–1446 RSC;
(f) Y. Wei, B. Rao, X. Cong and X. Zeng, J. Am. Chem. Soc., 2015, 137, 9250–9253 CrossRef CAS PubMed;
(g) S. Okumura, S. Tang, T. Saito, K. Semba, S. Sakaki and Y. Nakao, J. Am. Chem. Soc., 2016, 138, 14699–14704 CrossRef CAS PubMed;
(h) M. P. Wiesenfeldt, Z. Nairoukh, W. Li and F. Glorius, Science, 2017, 357, 908–912 CrossRef CAS PubMed;
(i) N. I. Saper, A. Ohgi, D. W. Small, K. Semba, Y. Nakao and J. F. Hartwig, Nat. Chem., 2020, 12, 276–283 CrossRef CAS PubMed.
-
(a) V. Nesterov, D. Reiter, P. Bag, P. Frisch, R. Holzner, A. Porzelt and S. Inoue, Chem. Rev., 2018, 118, 9678–9842 CrossRef CAS PubMed;
(b) A. Doddi, M. Peters and M. Tamm, Chem. Rev., 2019, 119, 6994–7112 CrossRef CAS PubMed;
(c) A. Vivancos, C. Segarra and M. Albrecht, Chem. Rev., 2018, 118, 9493–9586 CrossRef CAS PubMed;
(d) N. M. Scott and S. P. Nolan, Eur. J. Inorg. Chem., 2005, 10, 1815–1828 CrossRef;
(e)
Ligand Design in Metal Chemistry: Reactivity and Catalysis, ed. M. Stradiotto and R. J. Lundgren, Wiley, Hoboken, 2016 Search PubMed;
(f) D. Janssen-Müller, C. Schlepphorst and F. Glorius, Chem. Soc. Rev., 2017, 46, 4845–4854 RSC.
-
P. Roose, K. Eller, E. Henkes, R. Rossbacher and H. Höke, Amines, Aliphatic, Ullmann’s Encyclopedia of Industrial Chemistry, 2015, pp. 1–50 Search PubMed.
-
(a) J. J. Ritter and P. P. Minieri, J. Am. Chem. Soc., 1948, 70, 4045–4048 CrossRef CAS PubMed;
(b)
K. Mundinger and R. Schneider, Process for the preparation of primary amines, DE Pat., DE19632107, 1998 Search PubMed.
- R. H. Archer, S. I. Zones and M. E. Davis, Microporous Mesoporous Mater., 2010, 130, 255–265 CrossRef CAS.
- A. Collado, A. Gomez-Suarez, A. R. Martin, A. M. Z. Slawin and S. P. Nolan, Chem. Commun., 2013, 49, 5541–5543 RSC.
- P. de Frémont, N. M. Scott, E. D. Stevens, T. Ramnial, O. C. Lightbody, C. L. B. Macdonald, J. A. C. Clyburne, C. D. Abernethy and S. P. Nolan, Organometallics, 2005, 24, 6301–6309 CrossRef.
-
(a) S. V. C. Vummaleti, D. J. Nelson, A. Poater, A. Gomez-Suarez, D. B. Cordes, A. M. Z. Slawin, S. P. Nolan and L. Cavallo, Chem. Sci., 2015, 6, 1895–1904 RSC;
(b) A. Liske, K. Verlinden, H. Buhl, K. Schaper and C. Ganter, Organometallics, 2013, 32, 5269–5272 CrossRef CAS;
(c) O. Back, M. Henry-Ellinger, C. D. Martin, D. Martin and G. Bertrand, Angew. Chem., Int. Ed., 2013, 52, 2939–2943 CrossRef CAS PubMed.
- N. Marion and S. P. Nolan, Acc. Chem. Res., 2008, 41, 1440–1449 CrossRef CAS PubMed.
- R. D. J. Froese, C. Lombardi, M. Pompeo, R. P. Rucker and M. G. Organ, Acc. Chem. Res., 2017, 50, 2244–2253 CrossRef CAS PubMed.
- Crystallographic data have been deposited with the Cambridge Crystallographic Data Center.
- M. E. Jung and G. Piizzi, Chem. Rev., 2005, 105, 1735–1766 CrossRef CAS PubMed.
- L. Falivene, Z. Cao, A. Petta, L. Serra, A. Poater, R. Oliva, V. Scarano and L. Cavallo, Nat. Chem., 2019, 11, 872–879 CrossRef CAS PubMed.
- G. Meng, L. Kakalis, S. P. Nolan and M. Szostak, Tetrahedron Lett., 2019, 60, 378–381 CrossRef CAS.
- N. Marion, R. S. Ramon and S. P. Nolan, J. Am. Chem. Soc., 2009, 131, 448–449 CrossRef CAS PubMed.
- Y. D. Bidal, F. Lazreg and C. S. J. Cazin, ACS Catal., 2014, 4, 1564–1569 CrossRef CAS.
- H. Yoshida, I. Kageyuki and K. Takaki, Org. Lett., 2014, 16, 3512–3515 CrossRef CAS PubMed.
- S. Paul, B. P. Joy, R. Rajendran and V. B. Gudimetla, ChemistrySelect, 2019, 4, 7181–7186 CrossRef CAS.
- O. Navarro, H. Kaur, P. Mahjoor and S. P. Nolan, J. Org. Chem., 2004, 69, 3173–3180 CrossRef CAS PubMed.
- J. Huang and S. P. Nolan, J. Am. Chem. Soc., 1999, 121, 9889–9890 CrossRef CAS.
- M. Charton, J. Am. Chem. Soc., 1975, 97, 1552–1556 CrossRef CAS.
-
(a) K. A. Agnew-Francis and C. M. Williams, Adv. Synth. Catal., 2016, 358, 675–700 CrossRef CAS;
(b) M. B. Dinger, P. Nieczypor and J. C. Mol, Organometallics, 2003, 22, 5291–5296 CrossRef CAS;
(c) S. Kronig, E. Theuergarten, D. Holschumacher, T. Bannenberg, C. G. Daniliuc, P. G. Jones and M. Tamm, Inorg. Chem., 2011, 50, 7344–7359 CrossRef CAS PubMed;
(d) J. He, M. Wasa, K. S. L. Chan and J. Q. Yu, J. Am. Chem. Soc., 2013, 135, 3387–3390 CrossRef CAS PubMed;
(e) M. Tobisu, T. Morioka, A. Ohtsuki and N. Chatani, Chem. Sci., 2015, 6, 3410–3414 RSC.
|
This journal is © The Royal Society of Chemistry 2023 |
Click here to see how this site uses Cookies. View our privacy policy here.