Emerging consensus on net energy paves the way for improved integrated assessment modeling
Received
10th March 2023
, Accepted 20th November 2023
First published on 28th November 2023
Abstract
Extracting, processing, and delivering energy requires energy itself, which reduces the net energy available to society and yields considerable socioeconomic implications. Yet, most mitigation pathways and transition models overlook net energy feedbacks, specifically related to the decline in the quality of fossil fuel deposits, as well as energy requirements of the energy transition. Here, we summarize our position across 8 key points that converge to form a prevailing understanding regarding EROI (Energy Return on Investment), identify areas of investigation for the Net Energy Analysis community, discuss the consequences of net energy in the context of the energy transition, and underline the issues of disregarding it. Particularly, we argue that reductions in net energy can hinder the transition if demand-side measures are not implemented and adopted to limit energy consumption. We also point out the risks posed for the energy transition in the Global South, which, while being the least responsible for climate change, may be amongst the most impacted by both the climate crisis and net energy contraction. Last, we present practical avenues to consider net energy in mitigation pathways and Integrated Assessment Models (IAMs), emphasizing the necessity of fostering collaborative efforts among our different research communities.
Broader context
The transition from fossil fuels to low-carbon energy is made difficult by several factors. One of which is the energy investments required by the transition, often examined through the lens of the EROI (Energy Return on Investment) metric. Although the concept of EROI is simple, its application has proven to be challenging due to theoretical and practical difficulties. To address this situation, we summarize our position with 8 key points, which approximate an emerging consensus around EROI, and identify key areas under investigation for the Net Energy Analysis research community. Our summary uncovers how net energy is critical for the assessment of equitable and feasible transition scenarios, and yet how it remains marginally addressed in the current use of Integrated Assessment Models. We therefore suggest avenues for improvements to make sure that energy-economy feedbacks are internally consistent in mitigation pathways.
|
Introduction
On April 4 2022, IPCC Working Group III finalized its contribution to the Sixth Assessment Report. Reviewing progress and commitments for climate change mitigation, the report calls for more sustainable consumption habits and a shift away from fossil fuels towards low-carbon energy systems.1 This transition nevertheless requires significant energy investments for the alternative low-carbon energy system, which can be examined through the lens of the Energy Return on (Energy) Invested or ERO(E)I metric.2,3 Recent developments in the Net Energy Analysis (NEA) research community have highlighted EROI implications for socio-economic scenarios,4–8 in particular regarding the practical challenges of the low-carbon transition. Yet, in part due to a lack of formal methodology prior to the 2010s9,10 and to a delay in the emergence of robust results, such studies have failed to influence transition scenarios. To remedy this situation, we provide an overview of the net energy approach, summarize the claimed emerging consensus around EROI, address how it relates to the low-carbon transition, and suggest ways to better integrate net energy in Integrated Assessment Models (IAMs).
The net energy analysis approach
Net energy, i.e. the energy supplied to society in the form of energy carriers after subtracting the energy invested for the production and distribution of those energy carriers, is a fundamental prerequisite to allow the production and exchange of goods and services. For a given amount of net energy, a key metric of the energy system is the EROI – defined as the ratio between the total energy returned (Ereturned) and the total energy invested to accomplish the conversion (Einvested) over the entire life cycle of the system under study, i.e. EROI = Ereturned/Einvested.
As with all analyses that can be performed at the macro (economy-wide) and micro (technology-specific) scales, EROI can have slightly different interpretations.11–13 For example, at the scale of the global economy, the EROI has a minimum of 0 based on the first law of thermodynamics. When analyzing a single technology or energy subsystem that produces a final energy carrier, the EROI ratio can be less than one to one (1
:
1) (e.g., in Fig. 1, Einvested,2 is greater than Ereturned,2). Such systems can still be locally or temporarily useful when they have compelling properties, for example delivering a specific energy carrier that is in particular demand, e.g., the industrial food system, but they cannot be a main supplier of energy for society. Although the equations involved are simple, their application entails theoretical and practical difficulties that call for a rigorous definition of the systems boundaries.14,15
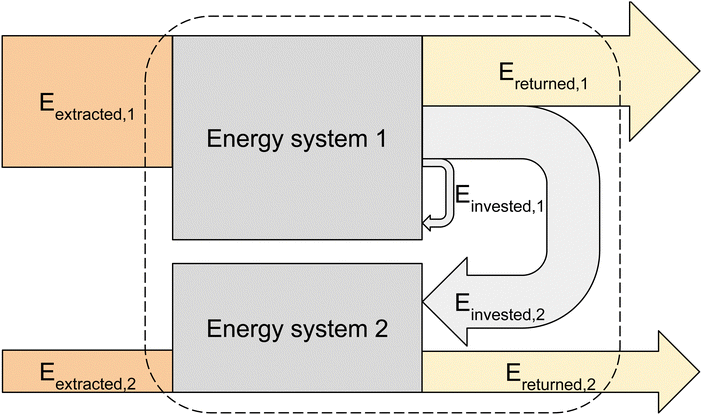 |
| Fig. 1 Returned energy as a function of the extracted and the invested energy for two systems. EROI (global) = (Ereturned,1 + Ereturned,2)/(Einvested,1 + Einvested,2). EROI (energy system 2) = (Ereturned,2)/(Einvested,2). Energy losses are omitted for clarity. | |
The “standard” (or primary stage) EROI accounts for the energy used in the extraction process only. It is useful for studying the energy demand of a primary energy extraction sector or technology.
The point-of-use (or final stage) EROI includes the energy used in not only extracting, but also processing and delivering an energy carrier. Therefore, for a given energy carrier, the point-of-use EROI is substantially lower than the standard EROI since additional energy inputs are considered. Focusing on the point of use is gaining in importance, as: (i) the energy requirements of processing, refining and other downstream processes for fossil fuels may be larger than that for their extraction, and (ii) most renewable energy systems directly deliver final energy carriers, i.e., typically electricity, making the analysis at the final energy stage essential to compare renewable and fossil fuel energy systems like for like.†
The dynamic EROI of the full energy system corresponds to the energy delivered by a countrys (or the entire worlds) energy system divided by its energy consumption at a given time, and is in that respect a Power Return on Investment (PROI) as the calculation is performed for a delimited time interval (one year usually).12,16
Emerging consensus on net energy
As researchers in the field of NEA, we summarize our position with the following 8 key points, which approximate an emerging consensus around EROI:
1. The standard EROI of oil is usually lower than that of gas, which is lower than that of most coal.17
2. Conventional fossil fuels (crude oil, natural gas liquids, etc.) may have lower standard EROIs than tight gas and oil produced from fracking18,19 but higher than other unconventional fuels (tar sands, mined shale oil, coal bed methane, etc.).6,20,21
3. The standard EROI of new fossil energy resources is expected to improve initially as technology develops, before decreasing due to a decline in the quality of the extracted resource.22,23 For instance, the standard EROI of oil sands-derived crude has been increasing since the first bitumen-producing mines became operational.24 On the contrary, many major conventional oil fields have already seen marked decreases in their standard EROI due to the requirements for enhanced recovery25 and global resource depletion, as evidenced by the decline in the quantity of the remaining “2P” (proven and probable) reserves.26,27
4. The aggregate EROI of fossil fuels at the point of use declines over time, albeit at a slower pace than at the point of extraction, since the largest investment (at the denominator of the EROI ratio) is not the energy required for extraction (that increases over time as resource quality decreases) but the subsequent energy required for processing and delivery (which is generally not much affected by the quality of the resource over the long-term).28
5. Today, the EROI of fossil-fueled electricity at point of end-use is often found to be lower than those of PV, wind and hydro electricity, even when the latter include the energy inputs for short-term (e.g., 8 h) storage‡ technologies.29,30 Average EROI values however hide strong regional variability, particularly for solar and wind technologies.31,32
6. The EROI of nuclear and hydropower have historically been high, however, the former is constrained by slow deployment times, the latter is limited in terms of availability of suitable locations, and both face many environmental considerations.
7. The point of use EROI for thermal fuels is usually low, specifically for liquid fuels (gasoline, biodiesel, bioethanol, etc.) compared to solid (coal, woodchips, etc.) or gaseous fuels.30
8. A rapid large-scale deployment of renewable electricity and associated infrastructure will likely temporarily reduce the dynamic EROI (i.e., PROI) of the energy system as it requires a significant up-front energy investment embodied in infrastructure.7,8,33–36
In parallel to this emerging consensus, several areas are under investigation, such as the future EROI trends of wind and solar. On one hand, their EROI might be negatively affected by the increase in energy requirements per unit of valuable mineral extracted due to geological depletion,37 whereby the quality of mineral deposits extracted (e.g., in terms of ore grade) decreases as a function of cumulative production. On the other hand, technological improvements may favorably affect the EROI of wind and solar PV.38 The same is true for increasing the recycling capacity of renewable energy technologies, but the delay is significant because of the time required to build up a stock of materials suitable for recycling.
Another area under scrutiny is the extension of the analysis to the useful stage of energy use, i.e. at the stage when energy is actually exchanged for energy services39 (see Fig. 2) as some energy carriers may be used for similar end-uses with very different final-to-useful efficiencies.40 For example, electricity might fuel a car at a lower EROIpoint-of-use than gasoline, but an electric vehicle motor has a considerably higher final-to-useful efficiency in converting its fuel input into mechanical drive when compared to a traditional internal combustion engine, such that an electric vehicle can have higher EROI at the useful stage. Of particular interest is the use of net energy analysis at the useful stage for a comprehensive understanding of the rebound effect at different geographic and time scales. More precisely, this approach can help explain why global data shows energy use continuing to increase as individual technologies become more efficient, suggesting it is difficult to disprove that, to date, increased efficiency has enabled increased energy use. Models that attempt to quantify rebound show large rebound effects (typical economy-wide estimates are over 50%).41
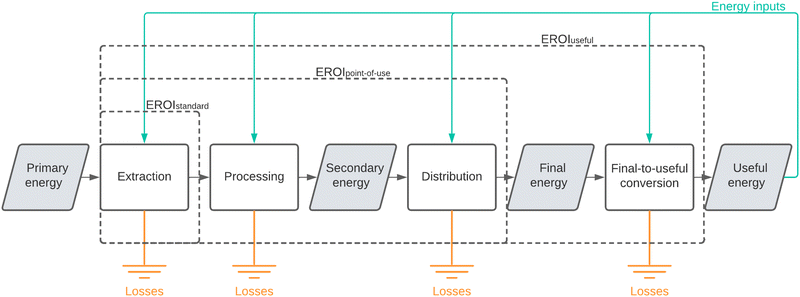 |
| Fig. 2 Standard, or primary stage EROI (EROIstandard), point-of-use or final stage EROI (EROIpoint-of-use) and useful EROI (EROIuseful). | |
Implications for the low-carbon transition
The net energy approach provides an enhanced understanding of the role of energy in economic processes, and as such, the EROI concept is increasingly used to model the energy-economy nexus. This growing modeling effort highlights two main net energy aspects which have implications for the low-carbon transition. On the one hand, the decline in the standard EROI of oil and gas may entail a rise in emissions per unit of net energy supplied to society,42 and long-term energy price increases,43,44 leading to periods of unfavorable growth or recession, especially for slow transition scenarios. On the other hand, the-perhaps only temporary-reduction in net energy available for society in rapid transition scenarios may result in a high investment share and employment rate in low-emissions technologies, which could altogether generate inflation,7,45,46 and thus raise questions of socio-political acceptance.
The pace of transition is bounded at the upper limit by the energy needed to sustain society without disruption (additional supply bottlenecks aside), and at the lower limit by the minimum speed required to meet climate targets (see Fig. 3). Both limits are expected to move closer to each other as the transition is delayed, reducing the window of opportunity for a global transition compatible with ambitious climate targets. On one hand, the upper limit is likely to become more restrictive over time due to the geological depletion of fossil fuels, the fact that a more rapid low-carbon investment consumes a higher proportion of energy, and that more high-carbon investment needs replacement or becomes stranded. On the other hand, the lower limit will become more pressing because, trivially, the longer the transition delays, the less likely it is to comply with ambitious climate targets. The implementation of demand-side policies47 to reduce discretionary energy use, as highlighted by IPCC WG III,1 is becoming increasingly relevant in this regard. Moving away from unnecessary uses and switching to more efficient conversion chains (e.g., from gasoline-powered to electric cars or bicycles) helps reduce discretionary energy use as long as rebound effects are mitigated. Further, recent research suggests that a decent life for all can be sustained at much lower levels of final energy use than at present within wealthy nations.48–50
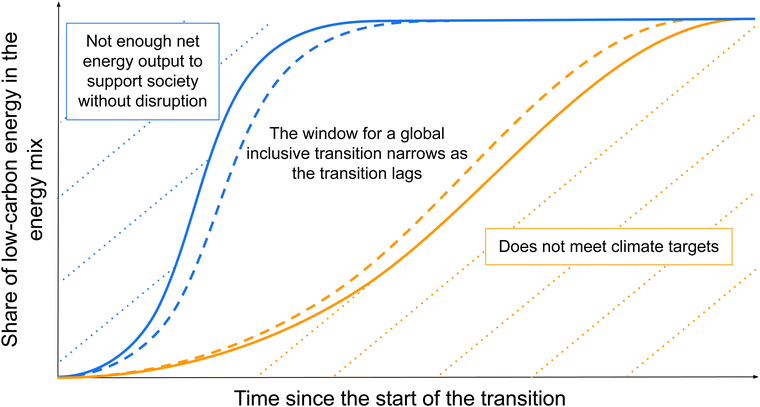 |
| Fig. 3 Sketch of principle of an evolving window of opportunity for the global inclusive transition to low-carbon energy as a function of normalized time at the start of the transition. The solid lines delineate the current window of opportunity. The dashed lines represent a future window in which action has not been taken quickly enough such that climate and net energy increasingly constrain the window of opportunity. | |
The energy transition has implications for equity. In particular, the upcoming reductions in net energy will necessarily amplify energy transition costs due to fossil fuel inflation and rapid low-carbon investment. Such reductions will in turn exacerbate competition for the energy and material resources necessary for the transition, a competition in which low-income countries are already at a clear disadvantage.51 Every Northern country that delays action thus risks compromising its ability to complete a transition and maintain or achieve high levels of material well-being, both for itself and other countries. This political situation raises inequity issues as countries from the Global North are likely to make their transition first. In this context, countries of the Global South are susceptible to lack access to energy, to the risk of getting slowed down – or even trapped – in their progress towards modern low-carbon energy, while being among the least responsible for and most affected by climate change.52–56 Accelerating the energy transition for the Global South is therefore a major stumbling block to a “just” transition, and requires massive financial support and technology transfers.57–60
Proper consideration of net energy is required in mitigation pathways
While significant progress has been made in research on mitigation pathways, net energy has been addressed only marginally. The latest IPCC report,1 for instance, mentions EROI issues in one paragraph (ch. 6, p. 44) and leaves out the evolution of the related literature, in part because the 8 key points developed earlier have only recently emerged. This situation results in insufficient discussion on the consequences of a decrease in the EROI of the energy system. The overlooking of net energy is also apparent in Integrated Assessment Models (IAMs), the main tools used to produce global, regionally disaggregated mitigation pathways.61
First, most IAMs merely characterize exhaustible fossil fuel resources through cost-supply curves, whose limitations in terms of modeling and parameterization lead to significant loss of robustness for mitigation pathways. On one hand, these curves operate under the assumption that the supply of fossil fuel resources depends purely on economic criteria, which means that production fluctuates with price, but in reality prices increase also because production does not increase fast enough. They furthermore assume that the most economically viable reserves will be exploited first, regardless of the complex interplay of other socio-geopolitical factors that shape the reality of producing companies,62 countries,63 and regions.64,65 On the other hand, typical upward sloping cost-supply curves are subject to criticism for potentially outdated, simplistic and over-optimistic assumptions in the recoverability of fossil resources.5,66–85 For instance, the MESSAGE86 and IMACLIM-R87 (partly) models continue to depend on the data provided by Rogner et al.88 for global fossil fuel reserves and resources, while the EPPA model89 includes simple recursive endogenous resource supply functions. The use of cost-supply curves also impedes the analysis of the economic consequences of a plateau or decline in oil production90-for example left out in the EMF2791 and RoSE82,92–94 intercomparison exercises. The main problem of using technically simplistic and methodologically questionable cost-supply curves is not only overestimating the plausibility of high-emission scenarios, but also making fossil fuels more attractive than they would be if depletion feedback effects were properly considered. This point of view is supported by the evaluation of AR5 scenarios against consistent growth rates of emissions from the fossil fuels industry,95,96 and the analysis of the GCAM-MAGICC integrated assessment model's sensitivity to revised cost-supply curves.83 It is also backed by the comparison of WoLiM78,97 or MEDEAS5 energy-constrained model results with scenarios from the literature, the incorporation of thorough oil production profiles in IMACLIM-R,98,99 and the examination of various fossil resource availabilities in the RoSE exercise.
Another critical modeling assumption is the fact that the energy used by the industry for a given scenario is not calculated in relation to the demand for the raw materials necessary for the completion of that scenario. To be able to calculate the raw material requirements, IAMs would have to represent the stocks of all infrastructures, combined with data on lifetimes and material intensities. The potential inconsistency between the industrial energy calculated from elasticities in IAMs and the industrial energy that would be calculated using a stock and raw materials approach adds further uncertainty to the net energy requirements.100,101
Moreover, IAMs dismiss comprehensive energy-economic feedbacks. They indeed assume that decreasing (net) energy supply, or increasing energy costs, do not influence economic growth whatsoever, as in the IMAGE,102 GCAM103 or POLES104 models, or have minimal impact when the output is recursively calculated, for instance using nested constant elasticity of substitution (CES) production functions found in models like EPPA, GTEM-C,105 REMIND, and WITCH.106 These functions have indeed faced criticism for their inability to accurately align with historically observed patterns in the dynamics of energy transition.107,108 We find this lack of energy-economic feedbacks particularly troublesome as the decrease in the EROI of the energy system will influence the impact of make demand-side measures in mitigation scenarios. The lack is even more problematic since some authors have found that IAMs favor Bioenergy with Carbon Capture and Storage (BECCS) over the use of renewable energy, notably by underestimating the cost reduction potential of renewables and especially PV,109–114 while in fact bioenergy and CCS technologies result in a significant decline in net energy.115,116 The importance of the net energy-economy feedback becomes even more apparent when considering the substantial energy requirements associated with the deployment of Direct Air Carbon Capture and Storage (DACCS), which are estimated to consume up to 300 EJ per year by 2100 in some scenarios.§
117
A considerable exception to current IAMs is the MEDEAS model5,118,119-now developed as the WILIAM model in the scope of the LOCOMOTION project–which appears to be the sole multi-scale¶ IAM that explores, from a heterodox perspective,120 the implications that the energy required for the transition may have on the energy system and the economy.121 Unlike other IAMs, MEDEAS includes an energy-economy feedback that allows energy availability to limit GDP growth in the event that it falls short of demand.120 When compared with AR5 business-as-usual scenarios, the results obtained with MEDEAS show a larger primary energy intensity of GDP, as well as lower CO2 intensity of primary energy, GDP per capita, and temperature change over pre-industrial levels.5 Such a modeling approach not only enables the characterization of the interaction between energy and the economy, such as the rebound effect,122 in a more historically consistent way,123 but also allows the user to assess the probability of GHG scenarios taken from other IAMs,124 as well as degrowth scenarios.120,122,125
The reasons why net energy is not comprehensively accounted for in IAMs are multiple and, in our view, fall primarily into three categories.
First, most IAMs lack proper representation of the energy and material flows of the goods and services provided,126–129 making them structurally unable to consider the industrial energy embodied in the infrastructures, and thus the energy-economy linkages brought to the fore by net energy analysis. Overlooking these flows may lead to an overestimation of the potential for reducing energy intensity (thus assuming possibly unrealistic decoupling rates between GHG and energy/material use), a greater focus on supply-side solutions for mitigating climate change,130 and underestimating the impact of rebound effects on energy demand.41,122,131–133
Second, most IAMs – either energy system models coupled with macroeconomic growth models or multi-sectorial Computable General Equilibrium (CGE) models – still utilize optimal growth theory from neoclassical economics. However, in these models, increases in energy costs cannot significantly affect GDP growth, either because GDP or technological change are assumed to be exogenous, or because the cost share of energy (as a percentage of GDP) is assumed to have negligible feedback on GDP. Thus, the current crop of IAMs not only downplays the contribution of energy and exergy in economic processes,134–136 but also sets aside its interaction with money and the financial sector, as these are both largely unmodeled in IAMs.137–140 This omission further precludes any attempt to understand how high levels of debt, which can increase financial instability risks, can be associated with net energy constraints or high energy costs (e.g. the global financial crisis of 2007–2008).141
Third, the current climate change scenarios framework (illustrated by the Shared Socioeconomic Pathways, SSP142) nurtures a simplistic and technocratic vision of the economy, that assumes little in the way of interdependence among population, economic growth, and other socio-economic parameters such as net-energy.143–149 This lack of explicit interdependence hinders existing climate mitigation scenarios from adequately assessing societal transformations,150 (in)justice151–153 including large-scale shifts in energy use between Global North and South,56 and systemic risks.154 The roots of the aforementioned limitations can be found in a lack of reflexivity, imaginative flexibility, plurality, transparency and transdisciplinarity within the IAM community, as acknowledged by some of its own members,127,155–164 but also in the gradual erosion of IAMs' neutrality165,166 due to political influence, and the communitys interest in playing an increasingly normative role in climate governance and policy-making.167–169
Avenues of improvement to consider net energy in IAMs
Several initiatives are underway to better account for industrial energy, and represent the interactions between energy and the economy in macroeconomic models and/or IAMs.170 Some IAMs, for instance, have adopted the use of more reliable data pertaining to fossil fuel energy resources, as exemplified by the incorporation of a comprehensive bottom-up dataset from Rystad Energy in E3ME-FTT-GENIE171,172 or the construction of detailed field-level analysis supply curves in TIAM-UCL.173 Dynamic constraints on extraction rates have also been introduced, as in REMIND174 or TIAM-UCL175 models, as well as specific rules trying to mimic the behavior of swing producers, as in IMACLIM-R or IMAGE, albeit in a very simplified way and mostly for the oil market.176,177 In an attempt to bridge the gap with Industrial Ecology (IE), several IAMs (notably REMIND and MESSAGE) have explored the implications of incorporating life cycle assessment coefficients from input–output (I–O) tables such as THEMIS178–180 or EXIOBASE,181 highlighting the potential for sustainability research areas such as the energy-industry nexus and post-growth scenarios.182 Efforts are also underway for examining the contribution of improved Energy System Models (ESM).183,184 Still, most IAMs operate within the neoclassical equilibrium framework, and thus do not properly capture the feedback from the energy system on the economy. For instance, Pehl et al. (2017)178 integrate a life-cycle assessment perspective in the REMIND model, and find that “fully considering life-cycle greenhouse gas emissions has only modest effects on the scale and structure of power production in cost-optimal mitigation scenarios”. However, the authors rely on a model that uses a CES production function, with limited feedback from the energy system (including its energy requirements) on the economy, and focus exclusively on the power sector.
In an attempt to remedy this situation, we highlight six avenues for improving IAMs:
1. the integration of Industrial Ecology methods (e.g. Material Flow Analysis) or modules such as DyMEMDS,185–187 ODYM-RECC,188,189 or QTDIAN,190 in order to better capture stocks and flows of energy and materials associated with the industrial subsector;
2. the adoption of a multi-sectoral energy framework, for instance relying on primary-final-useful (PFU) energy databases191–194 and consistent energy services narratives;
3. the use of an ecological macroeconomic framework (as in HARMONEY195,196 or TranSim46) or ESM which deal with environmental and biophysical indicators197 in a more comprehensive way, such as ENBIOS,198 EnergyScope199 or the one developed by Crownshaw;200
4. a common reporting template to include the energy consumption of the energy sector as well as useful energy in mitigation scenarios;8,201,202
5. an explicit modelling of energy-economy feedbacks (including rebound effects) using net energy, at least with the aim of understanding how a net energy feedback would affect IAMs results to assess to what extent energy and economic feedbacks are internally (in)consistent across mitigation pathways;202
6. the exploration of new mitigation pathways achieving high wellbeing levels with low resource use,203 limiting the deployment of energy intensive carbon dioxide removal,204–208 and equitable low-growth209 and post-growth scenarios.210–217
However, if these measures are to be properly implemented, they must be carried out simultaneously and without neoclassical economics theories,218,219 which we see as incompatible. This point particularly addresses the IAM communitys appeal that “further studies should at least aim at better reflecting the plurality of the visions of the economy”170 and take advantage of the robust development of heterodox economics,220 especially ecological macroeconomics.221,222
As countries seek to develop new nationally determined contributions (NDCs) and the IPCC currently considers reforming itself to produce more relevant knowledge for climate action,223–225 current momentum is towards the development of a new generation of IAMs and scenarios.226–233 In this regard, we believe that fostering collaborative efforts among our different research communities is timely, and could help improve integrated assessment modeling, with these dynamics being all the more supported by the convergence of views on demand-side measures and alternative economic pathways.
Conclusion
Consideration of net energy is crucial to assess and design comprehensive and coherent climate mitigation scenarios. Yet, in part due to the late emergence of robust results in the EROI literature, such consideration has not yet spread beyond the Net Energy Analysis community. Here we try to address this issue by outlining the emerging EROI consensus, exploring key areas under investigation, and identifying further work.
Our summary underlines that, in a fossil fuel dominated world, the initial energy investment to power the transition to a low-carbon future will inevitably come from fossil fuels. This does not mean, though, that renewables cannot eventually support themselves. However, net energy constraints may still limit the energy available to invest in energy infrastructure and the energy available for discretionary uses, absent more sustainable production and consumption habits. This situation may be particularly destabilizing for industrializing countries, which might stay at the doorstep of the energy transition, unable to increase their reliance on modern low-carbon energy, while being among the least responsible and among the most impacted by climate change. These dynamics should not be ignored in transition scenarios, and we therefore call on fellow researchers to integrate net energy into Integrated Assessment Models using theories outside of the neoclassical economics paradigm. In this respect, we believe that fostering collaborative efforts among our different research communities could prove decisive.
Author contributions
Louis Delannoy: conceptualization, writing – original draft, writing – review & editing, visualization, project administration. Matthieu Auzanneau: conceptualization, writing – review & editing. Baptiste Andrieu: writing – review & editing. Olivier Vidal: writing – review & editing. Pierre-Yves Longaretti: writing – review & editing, supervision, funding acquisition. Emmanuel Prados: writing – review & editing, supervision, funding acquisition. David J. Murphy: writing – review & editing Roger W. Bentley: writing – review & editing, supervision. Michael Carbajales-Dale: writing – review & editing. Marco Raugei: writing – review & editing. Mikael Höök: writing – review & editing. Victor Court: writing – review & editing. Carey W. King: writing – review & editing. Florian Fizaine: writing – review & editing. Pierre Jacques: writing – review & editing. Matthew Kuperus Heun: writing – review & editing Andrew Jackson: writing – review & editing. Charles Guay-Boutet: writing – review & editing. Emmanuel Aramendia: writing – review & editing. Jianliang Wang: writing – review & editing. Hugo Le Boulzec: writing – review & editing. Charles A. S. Hall: writing – review & editing.
Conflicts of interest
The authors declare that they have no known competing financial interests or personal relationships that could have appeared to influence the work reported in this paper.
Acknowledgements
This work benefited from the careful reading of Paul Brockway, Jarmo Kikstra and Loïc Giaccone. We also thank Iñigo Capellán-Pérez for comments on a previous version of the manuscript. Louis Delannoy, Pierre-Yves Longaretti and Emmanuel Prados acknowledge the support of the French National Institute for Research in Digital Science and Technology (INRIA). Victor Court acknowledges the support of the Chair Energy and Prosperity, under the aegis of La Fondation du Risque.
Notes and references
- IPCC, Climate Change 2022: Mitigation of Climate Change, Contribution of Working Group III to the Sixth Assessment Report of the Intergovernmental Panel on Climate Change, Technical report, IPCC, Cambridge, UK and New York, NY, USA, 2022.
-
C. A. S. Hall. Energy Return on Investment. Springer International Publishing, 2017 Search PubMed.
- H. Haberl, D. Wiedenhofer, S. Pauliuk, F. Krausmann, D. B. Müller and M. Fischer-Kowalski, Contributions of sociometabolic research to sustainability science, Nat. Sustainable, 2019, 2(3), 173–184 CrossRef.
- L. C. King and J. C. van den Bergh, Implications of net energy-return-on-investment for a low-carbon energy transition, Nat. Energy, 2018, 3(4), 334–340 CrossRef CAS.
- I. Capellán-Pérez, I. de Blas, J. Nieto, C. de Castro, L. J. Miguel, Ó. Carpintero, M. Mediavilla, L. F. Lobejón, N. Ferreras-Alonso, P. Rodrigo, F. Frechoso and D. Álvarez-Antelo, MEDEAS: a new modeling framework integrating global biophysical and socioeconomic constraints, Energy Environ. Sci., 2020, 13(3), 986–1017 RSC.
- L. Delannoy, P.-Y. Longaretti, D. J. Murphy and E. Prados, Assessing global long-term EROI of gas: A net-energy perspective on the energy transition, Energies, 2021, 14(16), 5112 CrossRef CAS.
- P. Jacques, L. Delannoy, B. Andrieu, D. Yilmaz, H. Jeanmart and A. Godin, Assessing the economic consequences of an energy transition through a biophysical stock-flow consistent model, Ecol. Econ., 2023, 209, 107832 CrossRef.
- A. Slameršak, G. Kallis and D. W. O’Neill, Energy requirements and carbon emissions for a low-carbon energy transition, Nat. Commun., 2022, 13(1), 6932 CrossRef PubMed.
- E. White and G. J. Kramer, The changing meaning of energy return on investment and the implications for the prospects of post-fossil civilization, One Earth, 2019, 1(4), 416–422 CrossRef.
- R. L. Rana, M. Lombardi, P. Giungato and C. Tricase, Trends in Scientific Literature on Energy Return Ratio of Renewable Energy Sources for Supporting Policymakers, Adm. Sci., 2020, 10(2), 21 CrossRef.
- A. R. Brandt and M. Dale, A general mathematical framework for calculating systems-scale efficiency of energy extraction and conversion: Energy return on investment (EROI) and other energy return ratios, Energies, 2011, 4(8), 1211–1245 CrossRef.
- C. King, J. Maxwell and A. Donovan, Comparing world economic and net energy metrics, part 1: Single technology and commodity perspective, Energies, 2015, 8(11), 12949–12974 CrossRef.
- C. King, J. Maxwell and A. Donovan, Comparing world economic and net energy metrics, part 2: Total economy expenditure perspective, Energies, 2015, 8(11), 12975–12996 CrossRef.
- D. J. Murphy and C. A. S. Hall, Energy return on investment, peak oil, and the end of economic growth, Ann. N. Y. Acad. Sci., 2011, 1219(1), 52–72 CrossRef PubMed.
- M. Raugei, Net energy analysis must not compare apples and oranges, Nat. Energy, 2019, 4(2), 86–88 CrossRef.
- M. Carbajales-Dale, When is EROI not EROI?, Biophys. Econ. Resour. Qual., 2019, 4(4), 16 CrossRef.
- V. Court and F. Fizaine, Long-term estimates of the energy-return-on-investment (EROI) of coal, oil, and gas global productions, Ecol. Econ., 2017, 138, 145–159 CrossRef.
- A. R. Brandt, T. Yeskoo and K. Vafi, Net energy analysis of Bakken crude oil production using a well-level engineering-based model, Energy, 2015, 93, 2191–2198 CrossRef.
- D. Moeller and D. Murphy, Net energy analysis of gas production from the marcellus shale, Biophys. Econ. Resour. Qual., 2016, 1(1), 5 CrossRef.
- A. R. Brandt, Y. Sun, S. Bharadwaj, D. Livingston, E. Tan and D. Gordon, Energy return on investment (EROI) for forty global oilfields using a detailed engineering-based model of oil production, PLoS One, 2015, 10(12), e0144141 CrossRef PubMed.
- L. Delannoy, P.-Y. Longaretti, D. J. Murphy and E. Prados, Peak oil and the low-carbon energy transition: A net-energy perspective, Appl. Energy, 2021, 304, 117843 CrossRef.
-
M. Dale. Global Energy Modelling: a Biophysical Approach (GEMBA), PhD thesis, University of Canterbury, 2011 Search PubMed.
- M. S. Masnadi and A. R. Brandt, Energetic productivity dynamics of global super-giant oilfields, Energy Environ. Sci., 2017, 10(6), 1493–1504 RSC.
- C. Guay-Boutet, Estimating the disaggregated standard EROI of canadian oil sands extracted via open-pit mining, 1997–2016, Biophys. Econ. Sustainability, 2023, 8(1), 2 CrossRef.
- V. S. Tripathi and A. R. Brandt, Estimating decades-long trends in petroleum field energy return on investment (EROI) with an engineering-based model, PLoS One, 2017, 12(2), e0171083 CrossRef PubMed.
- The Shift Project. The European Union is likely to face strong constraints on oil supplies between now and 2030 - Prudential prospective analysis, Technical report, The Shift Project, 2020.
- J. Laherrère, C. A. S. Hall and R. Bentley, How much oil remains for the world to produce? comparing assessment methods, and separating fact from fiction, Curr. Res. Environ. Sustainable, 2022, 4, 100174 CrossRef.
- P. E. Brockway, A. Owen, L. I. Brand-Correa and L. Hardt, Estimation of global final-stage energy-return-on-investment for fossil fuels with comparison to renewable energy sources, Nat. Energy, 2019, 4(7), 612–621 CrossRef CAS.
- M. Raugei, E. Leccisi and V. M. Fthenakis, What are the energy and environmental impacts of adding battery storage to photovoltaics? a generalized life cycle assessment, Energy Technol., 2020, 8(11), 1901146 CrossRef.
- D. J. Murphy, M. Raugei, M. Carbajales-Dale and B. R. Estrada, Energy return on investment of major energy carriers: Review and harmonization, Sustainability, 2022, 14(12), 7098 CrossRef CAS.
- E. Dupont, R. Koppelaar and H. Jeanmart, Global available wind energy with physical and energy return on investment constraints, Appl. Energy, 2018, 209, 322–338 CrossRef.
- E. Dupont, R. Koppelaar and H. Jeanmart, Global available solar energy under physical and energy return on investment constraints, Appl. Energy, 2020, 257, 113968 CrossRef.
- M. Dale and S. M. Benson, Energy balance of the global photovoltaic (PV) industry - is the PV industry a net electricity producer?, Environ. Sci. Technol., 2013, 47(7), 3482–3489 CrossRef CAS PubMed.
- S. Sgouridis, D. Csala and U. Bardi, The sower's way: quantifying the narrowing net-energy pathways to a global energy transition, Environ. Res. Lett., 2016, 11(9), 094009 CrossRef.
- I. Capellán-Pérez, C. de Castro, L. Javier and M. González, Dynamic energy return on energy investment (EROI) and material requirements in scenarios of global transition to renewable energies, Energy Strategy Rev., 2019, 26, 100399 CrossRef.
- A. Fabre, Evolution of EROIs of electricity until 2050: Estimation and implications on prices, Ecol. Econ., 2019, 164, 106351 CrossRef.
- F. Fizaine and V. Court, Renewable electricity producing technologies and metal depletion: A sensitivity analysis using the EROI, Ecol. Econ., 2015, 110, 106–118 CrossRef.
- B. Steffen, D. Hischier and T. S. Schmidt, Historical and projected improvements in net energy performance of power generation technologies, Environ. Sci. Technol., 2018, 11(12), 3524–3530 Search PubMed.
- E. Aramendia, P. E. Brockway, M. Pizzol and M. K. Heun., Moving from final to useful stage in energy-economy analysis: A critical assessment, Appl. Energy, 2021, 283, 116194 CrossRef.
-
E. Aramendia. Exploring the useful energy impacts of the global energy transition: a net energy perspective. PhD thesis, University of Leeds, 2023.
- P. E. Brockway, S. Sorrell, G. Semieniuk, M. Kuperus Heun and V. Court, Energy efficiency and economy-wide rebound effects: A review of the evidence and its implications, Renewable Sustainable Energy Rev., 2021, 141, 110781 CrossRef.
- M. Manfroni, S. G. F. Bukkens and M. Giampietro, The declining performance of the oil sector: Implications for global climate change mitigation, Appl. Energy, 2021, 298, 117210 CrossRef.
- C. W. King and C. A. S. Hall, Relating financial and energy return on investment, Sustainability, 2011, 3(10), 1810–1832 CrossRef.
- M. K. Heun and M. de Wit, Energy return on (energy) invested (EROI), oil prices, and energy transitions, Energy Policy, 2012, 40, 147–158 CrossRef.
- L. Režný and V. Bureš, Energy transition scenarios and their economic impacts in the extended neoclassical model of economic growth, Sustainability, 2019, 11(13), 3644 CrossRef.
- A. Jackson and T. Jackson, Modelling energy transition risk: The impact of declining energy return on investment (EROI), Ecol. Econ., 2021, 185, 107023 CrossRef.
- F. Creutzig, J. Roy, W. F. Lamb, I. M. L. Azevedo, W. Bruine de Bruin, H. Dalkmann, O. Y. Edelenbosch, F. W. Geels, A. Grubler, C. Hepburn, E. G. Hertwich, R. Khosla, L. Mattauch, J. C. Minx, A. Ramakrishnan, N. D. Rao, J. K. Steinberger, M. Tavoni, D. Ürge Vorsatz and E. U. Weber, Towards demand-side solutions for mitigating climate change, Nat. Clim. Change, 2018, 8(4), 260–263 CrossRef.
- J. M. Cullen, J. M. Allwood and E. H. Borgstein, Reducing Energy Demand: What Are the Practical Limits?, Environ. Sci. Technol., 2011, 45(4), 1711–1718 CrossRef CAS PubMed.
- J. Millward-Hopkins, J. K. Steinberger, N. D. Rao and Y. Oswald, Providing decent living with minimum energy: A global scenario, Global Environ. Change., 2020, 65, 102168 CrossRef.
- J. S. Kikstra, A. Mastrucci, J. Min, K. Riahi and N. D. Rao, Decent living gaps and energy needs around the world, Environ. Res. Lett., 2021, 16(9), 095006 CrossRef.
-
C. A. S. Hall, R. Powers and W. Schoenberg. Peak oil, EROI, investments and the economy in an uncertain future. in Biofuels, Solar and Wind as Renewable Energy Systems, ed. D. Pimentel, Springer, Netherlands, pp. 109–132, 2008 Search PubMed.
- Y. Oswald, A. Owen and J. K. Steinberger, Large inequality in international and intranational energy footprints between income groups and across consumption categories, Nat. Energy, 2020, 5(3), 231–239 CrossRef.
- S. Carley and D. M. Konisky, The justice and equity implications of the clean energy transition, Nat. Energy, 2020, 5(8), 569–577 CrossRef CAS.
- J. Hickel, D. W. O'Neill, A. L. Fanning and H. Zoomkawala., National responsibility for ecological breakdown: a fair-shares assessment of resource use, 1970–2017, Lancet Planet. Health, 2022, 6(4), e342–e349 CrossRef PubMed.
- J. Hickel, C. Dorninger, H. Wieland and I. Suwandi, Imperialist appropriation in the world economy: Drain from the global south through unequal exchange, 1990–2015, Global Environ. Change., 2022, 73, 102467 CrossRef.
- J. Hickel and A. Slamersak, Existing climate mitigation scenarios perpetuate colonial inequalities, Lancet Planet. Health, 2022, 6(7), e628–e631 CrossRef PubMed.
- M. M. V. Cantarero, Of renewable energy, energy democracy, and sustainable development: A roadmap to accelerate the energy transition in developing countries, Energy Res. Soc. Sci., 2020, 70, 101716 CrossRef.
- P. Newell, S. Srivastava, L. O. Naess, G. A. T. Contreras and R. Price, Toward transformative climate justice: An emerging research agenda, Wiley Interdiscip. Rev.: Clim. Change., 2021, 12(6), e733 Search PubMed.
- M. Poblete-Cazenave, S. Pachauri, E. Byers, A. Mastrucci and B. van Ruijven, Global scenarios of household access to modern energy services under climate mitigation policy, Nat. Energy, 2021, 6(8), 824–833 CrossRef.
- A. L. Fanning and J. Hickel, Compensation for atmospheric appropriation, Nat. Sustainable, 2023, 6, 1077–1086 CrossRef.
- L. van Beek, M. Hajer, P. Pelzer, D. van Vuuren and C. Cassen, Anticipating futures through models: the rise of integrated assessment modelling in the climate science-policy interface since 1970, Global Environ. Change., 2020, 65, 102191 CrossRef.
- R. Heede and N. Oreskes, Potential emissions of co2 and methane from proved reserves of fossil fuels: An alternative analysis, Global Environ. Change., 2016, 36, 12–20 CrossRef.
- F. Johnsson, J. Kjärstad and J. Rootzén, The threat to climate change mitigation posed by the abundance of fossil fuels, Clim. Policy, 2018, 19(2), 258–274 CrossRef.
- A. Verbruggen and T. Van de Graaf, Peak oil supply or oil not for sale?, Futures, 2013, 53, 74–85 CrossRef.
- N. Norouzi, M. Fani and Z. K. Ziarani, The fall of oil age: A scenario planning approach over the last peak oil of human history by 2040, J. Pet. Sci. Eng., 2020, 188, 106827 CrossRef CAS.
- R. J. Brecha, Emission scenarios in the face of fossil-fuel peaking, Energy Policy, 2008, 36(9), 3492–3504 CrossRef.
- P. A. Kharecha and J. E. Hansen, Implications of “peak oil” for atmospheric co2 and climate, Global Biogeochem. Cycles, 2008, 22, GB3012 CrossRef.
- W. P. Nel and C. J. Cooper, Implications of fossil fuel constraints on economic growth and global warming, Energy Policy, 2009, 37(1), 166–180 CrossRef.
- M. Höök, A. Sivertsson and K. Aleklett, Validity of the fossil fuel production outlooks in the IPCC emission scenarios, Nat. Resour. Res., 2010, 19(2), 63–81 CrossRef.
- A. Verbruggen and M. Al Marchohi, Views on peak oil and its relation to climate change policy, Energy Policy, 2010, 38(10), 5572–5581 CrossRef.
- L. Chiari and A. Zecca, Constraints of fossil fuels depletion on global warming projections, Energy Policy, 2011, 39(9), 5026–5034 CrossRef.
- M. Höök, Future coal production outlooks in the IPCC emission scenarios: Are they plausible?, Energy Environ., 2011, 22(7), 837–857 CrossRef.
- M. Dale, S. Krumdieck and P. Bodger, Global energy modelling—a biophysical approach (GEMBA) part 2: Methodology, Ecol. Econ., 2012, 73, 158–167 CrossRef.
- J.-F. Mercure and P. Salas, An assessement of global energy resource economic potentials, Energy, 2012, 46(1), 322–336 CrossRef.
- P. Berg and A. Boland, Analysis of ultimate fossil fuel reserves and associated co2 emissions in IPCC scenarios, Nat. Resour. Res., 2013, 23(1), 141–158 CrossRef.
- M. Höök and X. Tang, Depletion of fossil fuels and anthropogenic climate change—a review, Energy Policy, 2013, 52, 797–809 CrossRef.
- J. W. Murray and J. Hansen, Peak oil and energy independence: Myth and reality, Trans., Am. Geophys. Union, 2013, 94(28), 245–246 CrossRef.
- I. Capellán-Pérez, M. Mediavilla, C. de Castro, Ó. Carpintero and L. J. Miguel, Fossil fuel depletion and socio-economic scenarios: An integrated approach, Energy, 2014, 77, 641–666 CrossRef.
- I. Chapman, The end of Peak Oil? Why this topic is still relevant despite recent denials, Energy Policy, 2014, 64, 93–101 CrossRef.
- S. H. Mohr, J. Wang, G. Ellem, J. Ward and D. Giurco, Projection of world fossil fuels by country, Fuel, 2015, 141, 120–135 CrossRef CAS.
- J. W. Murray, Limitations of oil production to the IPCC scenarios: The new realities of US and global oil production, Biophys. Econ. Resour. Qual., 2016, 1(2), 1–13 Search PubMed.
- N. Bauer, J. Hilaire, R. J. Brecha, J. Edmonds, K. Jiang, E. Kriegler, H.-H. Rogner and F. Sferra, Assessing global fossil fuel availability in a scenario framework, Energy, 2016, 111, 580–592 CrossRef.
- I. Capellán-Pérez, I. Arto, J. M. Polanco-Martínez, M. González-Eguino and B. N. Marc, Likelihood of climate change pathways under uncertainty on fossil fuel resource availability, Energy Environ. Sci., 2016, 9(8), 2482–2496 RSC.
- J. Ritchie and H. Dowlatabadi, Why do climate change scenarios return to coal?, Energy, 2017, 140, 1276–1291 CrossRef.
- J. Wang, L. Feng, X. Tang, Y. Bentley and M. Höök, The implications of fossil fuel supply constraints on climate change projections: A supply-side analysis, Futures, 2017, 86, 58–72 CrossRef.
- IIASA, MESSAGE documentation, International Institute for Applied Systems Analysis, 2020.
- H. Waisman, C. Guivarch, F. Grazi and J. C. Hourcade, The imaclim-r model: infrastructures, technical inertia and the costs of low carbon futures under imperfect foresight, Clim. Change, 2012, 114(1), 101–120 CrossRef.
- H.-H. Rogner, An Assessment Of World Hydrocarbon Resources, Annu. Rev. Energy, 1997, 22(1), 217–262 CrossRef.
- Y.-H. H. Chen, S. Paltsev, A. Gurgel, J. M. Reilly and J. Morris, A multisectoral dynamic model for energy, economic, and climate scenario analysis, Low Carbon Econ., 2022, 13(02), 70–111 CrossRef.
- R. W. Bentley, M. Mushalik and J. Wang, The resource-limited plateau in global conventional oil production: Analysis and consequences, Biophys. Econ. Sustainability, 2020, 5(2), 10 CrossRef.
- D. McCollum, N. Bauer, K. Calvin, A. Kitous and K. Riahi, Fossil resource and energy security dynamics in conventional and carbon-constrained worlds, Clim. Change, 2013, 123(3–4), 413–426 Search PubMed.
- A. Cherp, J. Jewell, V. Vinichenko, N. Bauer and E. D. Cian, Global energy security under different climate policies, GDP growth rates and fossil resource availabilities, Clim. Change, 2016, 136(1), 83–94 CrossRef.
- E. D. Cian, F. Sferra and M. Tavoni, The influence of economic growth, population, and fossil fuel scarcity on energy investments, Clim. Change, 2016, 136(1), 39–55 CrossRef.
- E. Kriegler, I. Mouratiadou, G. Luderer, N. Bauer, R. J. Brecha, K. Calvin, E. De Cian, J. Edmonds, K. Jiang, M. Tavoni and O. Edenhofer, Will economic growth and fossil fuel scarcity help or hinder climate stabilization?, Clim. Change, 2016, 136(1), 7–22 CrossRef.
- M. G. Burgess, J. Ritchie, J. Shapland and R. Pielke, IPCC baseline scenarios have over-projected co2 emissions and economic growth, Environ. Res. Lett., 2020, 16(1), 014016 CrossRef.
- R. Pielke Jr, M. G. Burgess and J. Ritchie, Plausible 2005–2050 emissions scenarios project between 2 °C and 3 °C of warming by 2100, Environ. Res. Lett., 2022, 17(2), 024027 CrossRef.
- I. Capellán-Pérez, M. Mediavilla, C. de Castro, Ó. Carpintero and L. J. Miguel, More growth? an unfeasible option to overcome critical energy constraints and climate change, Sustainability Sci., 2015, 10(3), 397–411 CrossRef.
- J. Rozenberg, S. Hallegatte, A. Vogt-Schilb, O. Sassi, C. Guivarch, H. Waisman and J.-C. Hourcade, Climate policies as a hedge against the uncertainty on future oil supply, Clim. Change, 2010, 101(3–4), 663–668 CrossRef.
- H. Waisman, J. Rozenberg, O. Sassi and J.-C. Hourcade, Peak oil profiles through the lens of a general equilibrium assessment, Energy Policy, 2012, 48, 744–753 CrossRef.
- O. Y. Edelenbosch, K. Kermeli, W. Crijns-Graus, E. Worrell, R. Bibas, B. Fais, S. Fujimori, P. Kyle, F. Sano and D. P. van Vuuren, Comparing projections of industrial energy demand and greenhouse gas emissions in long-term energy models, Energy, 2017, 122, 701–710 CrossRef.
- C. Bataille, L. J. Nilsson and F. Jotzo, Industry in a net-zero emissions world: New mitigation pathways, new supply chains, modelling needs and policy implications, Energy Clim. Change, 2021, 2, 100059 CrossRef CAS.
-
E. Stehfest, D. van Vuuren, T. Kram, L. Bouwman, R. Alkemade, M. Bakkenes, H. Biemans, A. Bouwman, M. den Elzen, J. Janse, P. Lucas, J. van Minnen, C. Muller and A. Prins, Integrated assessment of global environmental change with IMAGE 3.0: Model description and policy applications, 2014 Search PubMed.
- K. Calvin, P. Patel, L. Clarke, G. Asrar, B. Bond-Lamberty, R. Y. Cui, A. D. Vittorio, K. Dorheim, J. Edmonds, C. Hartin, M. Hejazi, R. Horowitz, G. Iyer, P. Kyle, S. Kim, R. Link, H. McJeon, S. J. Smith, A. Snyder, S. Waldhoff and M. Wise, GCAM v5.1: representing the linkages between energy, water, land, climate, and economic systems, Geosci. Model Dev., 2019, 12(2), 677–698 CrossRef CAS.
- European Commission Joint Research Centre, POLES-JRC model documentation: 2018 update, Publications Office, 2018.
- Y. Cai, D. Newth, J. Finnigan and D. Gunasekera, A hybrid energy-economy model for global integrated assessment of climate change, carbon mitigation and energy transformation, Appl. Energy, 2015, 148, 381–395 CrossRef CAS.
- V. Bosetti, C. Carraro, M. Galeotti, E. Massetti and M. Tavoni, A world induced technical change hybrid model, Energy J., 2006, 01, 13–37 CrossRef.
- M. Heun, J. Santos, P. Brockway, R. Pruim, T. Domingos and M. Sakai, From theory to econometrics to energy policy: Cautionary tales for policymaking using aggregate production functions, Energies, 2017, 10(2), 203 CrossRef.
- A. Kaya, D. Csala and S. Sgouridis, Constant elasticity of substitution functions for energy modeling in general equilibrium integrated assessment models: a critical review and recommendations, Clim. Change, 2017, 145(1–2), 27–40 CrossRef.
- F. Creutzig, P. Agoston, J. C. Goldschmidt, G. Luderer, G. Nemet and R. C. Pietzcker, The underestimated potential of solar energy to mitigate climate change, Nat. Energy, 2017, 2(9), 17140 CrossRef.
- M. Victoria, N. Haegel, I. M. Peters, R. Sinton, A. Jäger-Waldau, C. del Cañizo, C. Breyer, M. Stocks, A. Blakers, I. Kaizuka, K. Komoto and A. Smets, Solar photovoltaics is ready to power a sustainable future, Joule, 2021, 5(5), 1041–1056 CrossRef CAS.
- N. Grant, A. Hawkes, T. Napp and A. Gambhir, Cost reductions in renewables can substantially erode the value of carbon capture and storage in mitigation pathways, One Earth, 2021, 4(11), 1588–1601 CrossRef.
- T. M. L. Wigley, S. Hong and B. W. Brook, Value-added diagnostics for the assessment and validation of integrated assessment models, Renewable Sustainable Energy Rev., 2021, 152, 111605 CrossRef CAS.
- M. Xiao, T. Junne, J. Haas and M. Klein, Plummeting costs of renewables - are energy scenarios lagging?, Energy Strategy Rev., 2021, 35, 100636 CrossRef.
- R. Way, M. C. Ives, P. Mealy and J. D. Farmer., Empirically grounded technology forecasts and the energy transition, Joule, 2022, 6(9), 2057–2082 CrossRef.
- M. Fajardy and N. M. Dowell., The energy return on investment of BECCS: is BECCS a threat to energy security?, Energy Environ. Sci., 2018, 11(6), 1581–1594 RSC.
- J. Sekera and A. Lichtenberger, Assessing carbon capture: Public policy, science, and societal need, Biophys. Econ. Sustainability, 2020, 5(3), 14 CrossRef.
- G. Realmonte, L. Drouet, A. Gambhir, J. Glynn, A. Hawkes, A. C. Köberle and M. Tavoni, An inter-model assessment of the role of direct air capture in deep mitigation pathways, Nat. Commun., 2019, 10(1), 3277 CrossRef CAS PubMed.
- R. Samsó, I. de Blas, I. Perissi, G. Martelloni and J. Solé, Scenario analysis and sensitivity exploration of the MEDEAS europe energy-economy-environment model, Energy Strategy Rev., 2020, 32, 100582 CrossRef.
- J. Solé, R. Samsó, E. García-Ladona, A. García-Olivares, J. Ballabrera-Poy, T. Madurell, A. Turiel, O. Osychenko, D. Álvarez, U. Bardi, M. Baumann, K. Buchmann, Í. Capellán-Pérez, M. Cerný, Ó. Carpintero, I. de Blas, C. De Castro, J.-D. De Lathouwer, C. Duce, L. Eggler, J. M. Enríquez, S. Falsini, K. Feng, N. Ferreras, F. Frechoso, K. Hubacek, A. Jones, R. Kaclíková, C. Kerschner, C. Kimmich, L. F. Lobejón, P. L. Lomas, G. Martelloni, M. Mediavilla, L. J. Miguel, D. Natalini, J. Nieto, A. Nikolaev, G. Parrado, S. Papagianni, I. Perissi, C. Ploiner, L. Radulov, P. Rodrigo, L. Sun and M. Theofilidi, Modelling the renewable transition: Scenarios and pathways for a decarbonized future using pymedeas, a new open-source energy systems model, Renewable Sustainable Energy Rev., 2020, 132, 110105 CrossRef.
- J. Nieto, Ó. Carpintero, L. F. Lobejón and L. J. Miguel, An ecological macroeconomics model: The energy transition in the EU, Energy Policy, 2020, 145, 111726 CrossRef.
- S. Hafner, A. Anger-Kraavi, I. Monasterolo and A. Jones, Emergence of new economics energy transition models: A review, Ecol. Econ., 2020, 177, 106779 CrossRef.
- I. de Blas, M. Mediavilla, I. Capellán-Pérez and C. Duce, The limits of transport decarbonization under the current growth paradigm, Energy Strategy Rev., 2020, 32, 100543 CrossRef.
- I. de Blas, L. J. Miguel and I. Capellán-Pérez, Modelling of sectoral energy demand through energy intensities in MEDEAS integrated assessment model, Energy Strategy Rev., 2019, 26, 100419 CrossRef.
- D. Huard, J. Fyke, I. Capellán-Pérez, H. D. Matthews and A.-I. Partanen, Estimating the likelihood of GHG concentration scenarios from probabilistic integrated assessment model simulations, Earths Future, 2022, 10(10), e2022EF002715 CrossRef.
- D. Pulido-Sánchez, I. Capellán-Pérez, C. de Castro and F. Frechoso, Material and energy requirements of transport electrification, Energy Environ. Sci., 2022, 15(12), 4872–4910 RSC.
- S. Pauliuk, A. Arvesen, K. Stadler and E. G. Hertwich, Industrial ecology in integrated assessment models, Nat. Clim. Change, 2017, 7(1), 13–20 CrossRef.
- J. T. S. Pedersen, D. van Vuuren, J. Gupta, F. D. Santos, J. Edmonds and R. Swart, IPCC emission scenarios: How did critiques affect their quality and relevance 1990–2022?, Global Environ. Change., 2022, 75, 102538 CrossRef.
- H. Desing, R. Widmer, U. Bardi, A. Beylot, R. G. Billy, M. Gasser, M. Gauch, D. C. Monfort, D. Beat Müller, M. Raugei, K. Remmen, V. Schenker, H. Schlesier, S. Valdivia and P. Wäger, Mobilizing materials to enable a fast energy transition: a conceptual framework, Resour., Conserv. Recycl., 2024, 200, 107314 CrossRef.
- E. Aramendia, P. B. Brockway, P. G. Taylor and J. Norman, Global energy consumption of the mineral mining industry: Exploring the historical perspective and future pathways to 2060, Global Environmental Change, 2023, 83, 102745, DOI:10.1016/j.gloenvcha.2023.102745.
- K. Scott, C. J. Smith, J. A. Lowe and L. Garcia-Carreras., Demand vs supply-side approaches to mitigation: What final energy demand assumptions are made to meet 1.5 and 2 targets?, Global Environ. Change., 2022, 72, 102448 CrossRef.
- B. Andrieu, O. Vidal, H. L. Boulzec, L. Delannoy and F. Verzier, Energy intensity of final consumption: the richer, the poorer the efficiency, Environ. Sci. Technol., 2022, 56(19), 13909–13919 CrossRef CAS PubMed.
- G. Semieniuk, L. Taylor, A. Rezai and D. K. Foley, Plausible energy demand patterns in a growing global economy with climate policy, Nat. Clim. Change, 2021, 11(4), 313–318 CrossRef.
- A. Gambhir, L. Drouet, D. McCollum, T. Napp, D. Bernie, A. Hawkes, O. Fricko, P. Havlik, K. Riahi, V. Bosetti and J. Lowe, Assessing the feasibility of global long-term mitigation scenarios, Energies, 2017, 10(1), 89 CrossRef.
- R. Kümmel and D. Lindenberger, How energy conversion drives economic growth far from the equilibrium of neoclassical economics, New J. Phys., 2014, 16(12), 125008 CrossRef.
- J. Santos, T. Domingos, T. Sousa and M. S. Aubyn, Useful exergy is key in obtaining plausible aggregate production functions and recognizing the role of energy in economic growth: Portugal 1960–2009, Ecol. Econ., 2018, 148, 103–120 CrossRef.
- J. H. Spangenberg and L. Polotzek, Like blending chalk and cheese - the impact of standard economics in IPCC scenarios, Real-World Econ. Rev., 2019, 87, 196–212 Search PubMed.
- H. Pollitt and J.-F. Mercure, The role of money and the financial sector in energy-economy models used for assessing climate and energy policy, Clim. Policy, 2017, 18(2), 184–197 CrossRef.
- E. Espagne, Money, finance and climate: The elusive quest for a truly integrated assessment model, Comp. Econ. Stud., 2018, 60(1), 131–143 CrossRef.
- M. Sanders, A. Serebriakova, P. Fragkos, F. Polzin, F. Egli and B. Steffen, Representation of financial markets in macro-economic transition models—a review and suggestions for extensions, Environ. Res. Lett., 2022, 17(8), 083001 CrossRef.
- Gaël Giraud and Paul Valcke, Macrodynamics and climate: a reformulation, Oxford Open Economics, 2023.
- R. Svartzman, D. Dron and E. Espagne, From ecological macroeconomics to a theory of endogenous money for a finite planet, Ecol. Econ., 2019, 162, 108–120 CrossRef.
- K. Riahi, D. P. van Vuuren, E. Kriegler, J. Edmonds, B. C. ONeill, S. Fujimori, N. Bauer, K. Calvin, R. Dellink, O. Fricko, W. Lutz, A. Popp, J. Crespo Cuaresma, S. Kc, M. Leimbach, L. Jiang, T. Kram, S. Rao, J. Emmerling, K. Ebi, T. Hasegawa, P. Havlik, F. Humpenöder, L. Aleluia Da Silva, S. Smith, E. Stehfest, V. Bosetti, J. Eom, D. Gernaat, T. Masui, J. Rogelj, J. Strefler, L. Drouet, V. Krey, G. Luderer, M. Harmsen, K. Takahashi, L. Baumstark, J. C. Doelman, M. Kainuma, Z. Klimont, G. Marangoni, H. Lotze-Campen, M. Obersteiner, A. Tabeau and M. Tavoni, The Shared Socioeconomic Pathways and their energy, land use, and greenhouse gas emissions implications: An overview, Global Environ. Change., 2017, 42, 153–168 CrossRef.
-
K. Kuhnhenn, Economic growth in mitigation scenarios: A blind spot in climate science global scenarios from a growth-critical perspective, Heinrich Böll Foundation, 2018.
- H. Buhaug and J. Vestby, On growth projections in the shared socioeconomic pathways, Glob. Environ. Polit., 2019, 19(4), 118–132 CrossRef.
- S. Asefi-Najafabady, L. Villegas-Ortiz and J. Morgan, The failure of integrated assessment models as a response to ‘climate emergency' and ecological breakdown: the emperor has no clothes, Globalizations, 2020, 18(7), 1178–1188 CrossRef.
-
B. Purvis, Modelling global futures: a comparison of 'limits to growth' and the use of integrated assessment models within the climate literature, Conference of the System Dynamics Society, 2021.
- V. Court and F. McIsaac, A representation of the world population dynamics for integrated assessment models, Environ. Model. Assess., 2020, 25(5), 611–632 CrossRef.
- R. Pielke and J. Ritchie, Distorting the view of our climate future: The misuse and abuse of climate pathways and scenarios, Energy Res. Soc. Sci., 2021, 72, 101890 CrossRef.
- R. Pielke and J. Ritchie, How climate scenarios lost touch with reality, Issues Environ. Sci. Technol., 2021, 37, 74–83 Search PubMed.
- E. Trutnevyte, L. F. Hirt, N. Bauer, A. Cherp, A. Hawkes, O. Y. Edelenbosch, S. Pedde and D. P. van Vuuren., Societal transformations in models for energy and climate policy: The ambitious next step, One Earth, 2019, 1(4), 423–433 CrossRef.
- N. D. Rao, P. Sauer, M. Gidden and K. Riahi, Income inequality projections for the shared socioeconomic pathways (ssps), Futures, 2019, 105, 27–39 CrossRef.
- N. R. Rivadeneira and W. Carton, (in)justice in modelled climate futures: A review of integrated assessment modelling critiques through a justice lens, Energy Res. Soc. Sci., 2022, 92, 102781 CrossRef.
- S. Pachauri, S. Pelz, C. Bertram, S. Kreibiehl, N. D. Rao, Y. Sokona and K. Riahi, Fairness considerations in global mitigation investments, Science, 2022, 378(6624), 1057–1059 CrossRef CAS PubMed.
- J. Rising, M. Tedesco, F. Piontek and D. A. Stainforth, The missing risks of climate change, Nature, 2022, 610(7933), 643–651 CrossRef CAS PubMed.
- H. Doukas, A. Nikas, M. González-Eguino, I. Arto and A. Anger-Kraavi, From Integrated to Integrative: Delivering on the Paris Agreement, Sustainability, 2018, 10(7), 2299 CrossRef.
- K. Anderson and J. Jewell, Debating the bedrock of climate-change mitigation scenarios, Nature, 2019, 573(7774), 348–349 CrossRef CAS PubMed.
- G. Foster, Concrete utopianism in integrated assessment models: Discovering the philosophy of the shared socioeconomic pathways, Energy Res. Soc. Sci., 2020, 68(101533), 101533 CrossRef.
- L. F. Hirt, G. Schell, M. Sahakian and E. Trutnevyte, A review of linking models and socio-technical transitions theories for energy and climate solutions, Environ. Innov. Soc. Transit., 2020, 35, 162–179 CrossRef.
- B. C. O'Neill, T. R. Carter, K. Ebi, P. A. Harrison, E. Kemp-Benedict, K. Kok, E. Kriegler, B. L. Preston, K. Riahi, J. Sillmann, B. J. van Ruijven, D. van Vuuren, D. Carlisle, C. Conde, J. Fuglestvedt, C. Green, T. Hasegawa, J. Leininger, S. Monteith and R. Pichs-Madruga, Achievements and needs for the climate change scenario framework, Nat. Clim. Change, 2020, 10(12), 1074–1084 CrossRef PubMed.
- P. Raskin and R. Swart, Excluded futures: the continuity bias in scenario assessments, Sustainable Earth, 2020, 3(1), 8 CrossRef.
- S. Robertson, Transparency, trust, and integrated assessment models: An ethical consideration for the intergovernmental panel on climate change, Wiley Interdiscip. Rev.: Clim. Change., 2020, 12(1), e676 Search PubMed.
- J. Skea, P. Shukla, A. A. Khourdajie and D. McCollum, Intergovernmental panel on climate change: Transparency and integrated assessment modeling, Wiley Interdiscip. Rev.: Clim. Change., 2021, 12(5), e727 Search PubMed.
- S. Sgouridis, C. Kimmich, J. Solé, M. Cerný, M.-H. Ehlers and C. Kerschner, Visions before models: The ethos of energy modeling in an era of transition, Energy Res. Soc. Sci., 2022, 88, 102497 CrossRef.
- K. Koasidis, A. Nikas and H. Doukas, Why integrated assessment models alone are insufficient to navigate us through the polycrisis, One Earth, 2023, 6(3), 205–209 CrossRef.
- S. Ellenbeck and J. Lilliestam, How modelers construct energy costs: Discursive elements in energy system and integrated assessment models, Energy Res. Soc. Sci., 2019, 47, 69–77 CrossRef.
- L. van Beek, J. Oomen, M. Hajer, P. Pelzer and D. van Vuuren, Navigating the political: An analysis of political calibration of integrated assessment modelling in light of the 1.5 °C goal, Environ. Sci. Policy, 2022, 133, 193–202 CrossRef.
- S. Beck and M. Mahony, The politics of anticipation: the ipcc and the negative emissions technologies experience, Glob. Sustainability, 2018, 1, e8 CrossRef.
- L. van Beek, M. Hajer, P. Pelzer, D. van Vuuren and C. Cassen, Anticipating futures through models: the rise of integrated assessment modelling in the climate science-policy interface since 1970, Global Environ. Change., 2020, 65, 102191 CrossRef.
- S. Beck and J. Oomen, Imagining the corridor of climate mitigation - what is at stake in ipccs politics of anticipation?, Environ. Sci. Policy, 2021, 123, 169–178 CrossRef.
- I. Keppo, I. Butnar, N. Bauer, M. Caspani, O. Edelenbosch, J. Emmerling, P. Fragkos, C. Guivarch, M. Harmsen, J. Lefèvre, T. Le Gallic, M. Leimbach, W. McDowall, J.-F. Mercure, R. Schaeffer, E. Trutnevyte and F. Wagner, Exploring the possibility space: taking stock of the diverse capabilities and gaps in integrated assessment models, Environ. Res. Lett., 2021, 16(5), 053006 CrossRef.
- J.-F. Mercure, P. Salas, P. Vercoulen, G. Semieniuk, A. Lam, H. Pollitt, P. B. Holden, N. Vakilifard, U. Chewpreecha, N. R. Edwards and J. E. Vinuales, Reframing incentives for climate policy action, Nat. Energy, 2021, 6(12), 1133–1143 CrossRef.
- G. Semieniuk, P. B. Holden, J.-F. Mercure, P. Salas, H. Pollitt, K. Jobson, P. Vercoulen, U. Chewpreecha, N. R. Edwards and J. E. Viñuales, Stranded fossil-fuel assets translate to major losses for investors in advanced economies, Nat. Clim. Change, 2022, 12(6), 532–538 CrossRef.
- D. Welsby, J. Price, S. Pye and P. Ekins, Unextractable fossil fuels in a 1.5 °C world, Nature, 2021, 597(7875), 230–234 CrossRef CAS PubMed.
- L. Baumstark, N. Bauer, F. Benke, C. Bertram, S. Bi, C. Chris Gong, J. Philipp Dietrich, A. Dirnaichner, A. Giannousakis, J. Hilaire, D. Klein, J. Koch, M. Leimbach, A. Levesque, S. Madeddu, A. Malik, A. Merfort, L. Merfort, A. Odenweller, M. Pehl, R. C. Pietzcker, F. Piontek, S. Rauner, R. Rodrigues, M. Rottoli, F. Schreyer, A. Schultes, B. Soergel, D. Soergel, J. Strefler, F. Ueckerdt, E. Kriegler and G. Luderer., REMIND2.1: transformation and innovation dynamics of the energy-economic system within climate and sustainability limits, Geosci. Model Dev., 2021, 14(10), 6571–6603 CrossRef CAS.
-
S. Pye, I. Butnar, J. Cronin, D. Welsby, J. Price, O. Dessens, B. S. Rodríguez, M. Winning, G. Anandarajah, D. Scamman and I. Keppo, The TIAM-UCL Model (Version 4.1.1) Documentation, 2020 Search PubMed.
- T. Faehn, G. Bachner, R. Beach, J. Chateau, S. Fujimori, M. Ghosh, M. Hamdi-Cherif, E. Lanzi, S. Paltsev, T. Vandyck, B. Cunha, R. Garaffa and K. Steininger, Capturing key energy and emission trends in CGE models: Assessment of status and remaining challenges, J. Glob. Econ. Anal., 2020, 5(1), 196–272 CrossRef.
- J. Foure, A. Aguiar, R. Bibas, J. Chateau, S. Fujimori, J. Lefevre, M. Leimbach, L. Rey-Los-Santos and H. Valin, Macroeconomic drivers of baseline scenarios in dynamic CGE models: review and guidelines proposal, J. Glob. Econ. Anal., 2020, 5(1), 28–62 CrossRef.
- M. Pehl, A. Arvesen, F. Humpenöder, A. Popp, E. G. Hertwich and G. Luderer, Understanding future emissions from low-carbon power systems by integration of life-cycle assessment and integrated energy modelling, Nat. Energy, 2017, 2(12), 939–945 CrossRef CAS.
- A. Arvesen, G. Luderer, M. Pehl, B. L. Bodirsky and E. G. Hertwich, Deriving life cycle assessment coefficients for application in integrated assessment modelling, Environ Model Softw, 2018, 99, 111–125 CrossRef.
- G. Luderer, M. Pehl, A. Arvesen, T. Gibon, B. L. Bodirsky, H. Sytze de Boer, O. Fricko, M. Hejazi, F. Humpenöder, G. Iyer, S. Mima, I. Mouratiadou, R. C. Pietzcker, A. Popp, M. van den Berg, D. van Vuuren and G. H. Edgar, Environmental co-benefits and adverse side-effects of alternative power sector decarbonization strategies, Nat. Commun., 2019, 10(1), 5229 CrossRef PubMed.
- M. Budzinski, R. Wood, B. Zakeri, V. Krey and A. H. Strømman, Coupling energy system models with multi-regional input–output models based on the make and use framework – insights from MESSAGEix and EXIOBASE, Econ. Syst. Res., 2023, 1–19 CrossRef.
- J. Lefèvre, Integrated assessment models and input-output analysis: bridging fields for advancing sustainability scenarios research, Econ. Syst. Res., 2023, 1–24 CrossRef.
- K. Huang and M. J. Eckelman, Appending material flows to the national energy modeling system (NEMS) for projecting the physical economy of the united states, J. Ind. Ecol., 2020, 26(1), 294–308 CrossRef.
- F. Kullmann, P. Markewitz, D. Stolten and M. Robinius, Combining the worlds of energy systems and material flow analysis: a review, Energy Sustainable Soc., 2021, 11(1), 13 CrossRef.
- O. Vidal, H. L. Boulzec, B. Andrieu and F. Verzier, Modelling the Demand and Access of Mineral Resources in a Changing World, Sustainability, 2021, 14(1), 11 CrossRef.
- H. L. Boulzec, L. Delannoy, B. Andrieu, F. Verzier, O. Vidal and S. Mathy, Dynamic modeling of global fossil fuel infrastructure and materials needs: Overcoming a lack of available data, Appl. Energy, 2022, 326, 119871 CrossRef.
- H. L. Boulzec, S. Mathy, F. Verzier, B. Andrieu, D. Monfort-Climent and O. Vidal, Material requirements and impacts of the building sector in the shared socioeconomic pathways, J. Cleaner Prod., 2023, 428, 139117 CrossRef.
- S. Pauliuk and N. Heeren, ODYM—an open software framework for studying dynamic material systems: Principles, implementation, and data structures, J. Ind. Ecol., 2019, 24(3), 446–458 CrossRef.
- S. Pauliuk, T. Fishman, N. Heeren, P. Berrill, Q. Tu, P. Wolfram and E. G. Hertwich, Linking service provision to material cycles: A new framework for studying the resource efficiency–climate change (RECC) nexus, J. Ind. Ecol., 2020, 25(2), 260–273 CrossRef.
- D. Süsser, H. Rakouki and J. Lilliestam, The QTDIAN modelling toolbox-quantification of social drivers and constraints of the diffusion of energy technologies. deliverable 2.3. sustainable energy transitions laboratory (sentinel) project. Technical report, Institute for Advanced Sustainability, Studies (IASS), 2021 Search PubMed.
- M. K. Heun, Z. Marshall, E. Aramendia and P. E. Brockway, The energy and exergy of light with application to societal exergy analysis, Energies, 2020, 13(20), 5489 CrossRef.
- P. Steenwyk, M. K. Heun, P. Brockway, T. Sousa and S. Henriques, The contributions of muscle and machine work to land and labor productivity in world agriculture since 1800, Biophys. Econ. Sustainability, 2022, 7, 2 CrossRef.
- R. Pinto, S. T. Henriques, P. E. Brockway, M. K. Heun and T. Sousa, The rise and stall of world electricity efficiency:1900–2017, results and insights for the renewables transition, Energy, 2023, 269, 126775 CrossRef.
-
Z. Marshall, M. Heun, P. Brockway, E. Aramendia, P. Steenwyk, T. Relph, M. Widjanarko, J. Kim, A. Sainju and J. Iturbe, Ta multi-regional primary-final-useful (mr-pfu) energy and exergy database v1.0, Technical Report, University of Leeds, 2023 Search PubMed.
- C. W. King, An integrated biophysical and economic modeling framework for long-term sustainability analysis: the HARMONEY model, Ecol. Econ., 2020, 169, 106464 CrossRef.
- C. W. King, Interdependence of growth, structure, size and resource consumption during an economic growth cycle, Biophys. Econ. Sustainability, 2021, 7, 1 Search PubMed.
- J. Sherwood, M. Carbajales-Dale and B. R. Haney, Putting the biophysical (back) in economics: A taxonomic review of modeling the earth-bound economy, Biophys. Econ. Sustainability, 2020, 5, 4 CrossRef.
- N. Martin, C. Madrid-López, G. Villalba-Méndez and L. Talens-Peiró, Overlooked factors in predicting the transition to clean electricity, Environmental Research: Infrastructure and Sustainability, 2022, 2(2), 021005 Search PubMed.
- G. Limpens, S. Moret, H. Jeanmart and F. Maréchal, EnergyScope TD: A novel open-source model for regional energy systems, Appl. Energy, 2019, 255, 113729 CrossRef.
-
T. J. H. Crownshaw. A modelling framework for evaluating the dynamic metabolic feasibility of energy transition scenarios. Working paper SSRN, 2023 Search PubMed.
- C. Neumeyer and R. Goldston, Dynamic EROI assessment of the IPCC 21st century electricity production scenario, Sustainability, 2016, 8(5), 421 CrossRef.
- G. Palmer, A biophysical perspective of IPCC integrated energy modelling, Energies, 2018, 11(4), 839 CrossRef.
- C. Wilson, A. Grubler, G. Nemet, S. Pachauri, S. Pauliuk and D. Wiedenhofer, The 'high-with-low' scenario narrative: Key themes, cross-cutting linkages, and implications for modelling, IIASA Working Paper, 2023 Search PubMed.
- R. Martin, Sers. Ecological macroeconomic assessment of meeting a carbon budget without negative emissions, Glob. Sustainability, 2022, 5, e6 CrossRef.
- D. P. van Vuuren, E. Stehfest, D. E. H. J. Gernaat, M. van den Berg, D. L. Bijl, H. Sytze de Boer, V. Daioglou, J. C. Doelman, O. Y. Edelenbosch, M. Harmsen, A. F. Hof and M. A. E. van Sluisveld, Alternative pathways to the 1.5 °C target reduce the need for negative emission technologies, Nat. Clim. Change, 2018, 8(5), 391–397 CrossRef.
- S. Pye, O. Broad, C. Bataille, P. Brockway, H. E. Daly, R. Freeman, A. Gambhir, O. Geden, F. Rogan, S. Sanghvi, J. Tomei, I. Vorushylo and J. Watson, Modelling net-zero emissions energy systems requires a change in approach, Clim. Policy, 2020, 21(2), 222–231 CrossRef.
- M. Diesendorf., Scenarios for mitigating co2 emissions from energy supply in the absence of co2 removal, Clim. Policy, 2022, 22(7), 882–896 CrossRef.
- S. Hollnaicher, On economic modeling of carbon dioxide removal: values, bias, and norms for good policy-advising modeling, Glob. Sustainability, 2022, 5, e18 CrossRef.
- M. G. Burgess, R. E. Langendorf and J. D. Moyer, Ashley Dancer, Barry B. Hughes, and David Tilman. Multidecadal dynamics project slow 21st-century economic growth and income convergence, Commun. Earth Environ., 2023, 4, 220 CrossRef.
- J. Floyd, S. Alexander, M. Lenzen, P. Moriarty, G. Palmer, S. Chandra-Shekeran, B. Foran and L. Keyßer., Energy descent as a post-carbon transition scenario: How ‘knowledge humility' reshapes energy futures for post-normal times, Futures, 2020, 122, 102565 CrossRef.
- I. Otero, K. N. Farrell, S. Pueyo, G. Kallis, L. Kehoe, H. Haberl, C. Plutzar, P. Hobson, J. G. Márquez, B. R. Labajos, J. L. Martin, K. H. Erb, S. Schindler, J. Nielsen, T. Skorin, J. Settele, F. Essl, E. G. Baggethun, L. Brotons, W. Rabitsch, F. Schneider and G. Peer, Biodiversity policy beyond economic growth, Conserv. Lett., 2020, 13(4), e12713 CrossRef PubMed.
- L. T. Keyßer and M. Lenzen, 1.5 °C degrowth scenarios suggest the need for new mitigation pathways, Nat. Commun., 2021, 12(1), 2676 CrossRef PubMed.
- L. Warszawski, E. Kriegler, T. M. Lenton, O. Gaffney, D. Jacob, D. Klingenfeld, R. Koide, M. M. Costa, D. Messner, N. Nakicenovic, H. J. Schellnhuber, P. Schlosser, K. Takeuchi, S. Van Der Leeuw, G. Whiteman and J. Rockström., All options, not silver bullets, needed to limit global warming to 1.5 °C: a scenario appraisal, Environ. Res. Lett., 2021, 16(6), 064037 CrossRef CAS.
- J. Hickel, P. Brockway, G. Kallis, L. Keyßer, M. Lenzen, A. Slameršak, J. Steinberger and D. Ü. Vorsatz, Urgent need for post-growth climate mitigation scenarios, Nat. Energy, 2021, 6(8), 766–768 CrossRef.
-
J. S. Kikstra, M. Li, P. Brockway, J. Hickel, L. Keysser, A. Malik, J. Rogelj, B. Van Ruijven and M. Lenzen, Towards degrowth in integrated assessment models [preprint v1.1], 2023.
- M. Li, L. Keyßer, J. S. Kikstra, J. Hickel, P. E. Brockway, N. Dai, A. Malik and M. Lenzen, Integrated assessment modelling of degrowth scenarios for australia, Econ. Syst. Res., 2023, 1–31 CrossRef.
- J. D. Moyer, Modeling
transformational policy pathways on low growth and negative growth scenarios to assess impacts on socioeconomic development and carbon emissions, Sci. Rep., 2023, 13, 15996 CrossRef CAS PubMed.
- L. Brand-Correa, A. Brook, M. Büchs, P. Meier, Y. Naik and D. W. O'Neill., Economics for people and planet—moving beyond the neoclassical paradigm, Lancet Planet. Health, 2022, 6(4), e371–e379 CrossRef PubMed.
- N. Stern, J. Stiglitz and C. Taylor, The economics of immense risk, urgent action and radical change: towards new approaches to the economics of climate change, J. Econ. Methodol., 2022, 29(3), 181–216 CrossRef.
- J. Christopher Proctor, Expanding the possible: exploring the role for heterodox economics in integrated climate-economy modeling, Review of Evolutionary Political Economy, 2023 Search PubMed.
- R. Cattan and F. McIsaac, A macroeconomic critique of integrated assessment environmental models: the case of Brazil, Rev. Keynes. Econ., 2021, 9(2), 204–231 Search PubMed.
-
J. Althouse. Ecological Macroeconomics for a Shared Planet: towards a Global Political Ecology of Money, Finance and Production. PhD thesis, Université Sorbonne Paris Nord, 2022 Search PubMed.
- S. Asayama, K. De Pryck, S. Beck, B. Cointe, P. N. Edwards, H. Guillemot, K. M. Gustafsson, F. Hartz, H. Hughes, B. Å. Lahn, O. Leclerc, R. Lidskog, J. E. Livingston, I. Lorenzoni, J. Petrasek MacDonald, M. Mahony, J. C. H. Miguel, M. Monteiro, J. OReilly, W. Pearce, A. Petersen, B. Siebenhüner, T. Skodvin, A. Standring, G. Sundqvist, R. Taddei, B. van Bavel, M. Vardy, Y. Yamineva and M. Hulme, Three institutional pathways to envision the future of the ipcc, Nat. Clim. Change, 2023, 13(9), 877–880 CrossRef.
- E. A. T. Hermansen, E. L. Boasson and G. P. Peters, Climate action post-paris: how can the ipcc stay relevant?, npj Clim. Action, 2023, 2, 30 CrossRef.
- I. Noy, Economists are not engaged enough with the ipcc, npj Clim. Action, 2023, 2, 33 CrossRef.
- D. P. Jiesper Strandsbjerg Tristan Pedersen, B. A. van Vuuren, R. Aparício and J. Swart, Gupta, and Filipe Duarte Santos. Variability in historical emissions trends suggests a need for a wide range of global scenarios and regional analyses, Commun. Earth Environ., 2020, 1(1), 41 CrossRef.
- A. Gambhir, G. Ganguly and S. Mittal, Climate change mitigation scenario databases should incorporate more non-IAM pathways, Joule, 2022, 6(12), 2663–2667 CrossRef.
- J. S. T. Pedersen, C. M. Gomes, J. Gupta, D. van Vuuren, F. D. Santos and R. Swart, The policy-relevance of emission scenarios: Policymakers require simpler, relevant, and more communicative scenarios, SSRN Electron. J., 2022 DOI:10.2139/ssrn.4073175.
-
A. Ranjan, T. Kanitkar and T. Jayaraman, A new scenario framework for equitable and climate-compatible futures, 2023 Search PubMed.
- I. Savin and J. van den Bergh, Tired of climate targets? shift focus of ipcc scenarios from emission and growth targets to policies, Ann. N. Y. Acad. Sci., 2022, 1517(1), 5–10 CrossRef PubMed.
-
M. Meinshausen, C.-F. Schleussner, K. Beyer, G. Bodeker, O. Boucher, J. G. Canadell, J. S. Daniel, A. Diongue-Niang, F. Driouech, E. Fischer, P. Forster, M. Grose, G. Hansen, Z. Hausfather, T. Ilyina, J. S. Kikstra, J. Kimutai, A. King, J.-Y. Lee, C. Lennard, T. Lissner, A. Nauels, G. P. Peters, A. Pirani, G.-K. Plattner, H. Pörtner, J. Rogelj, M. Rojas, J. Roy, B. H. Samset, B. M. Sanderson, R. Séférian, S. Seneviratne, C. J. Smith, S. Szopa, A. Thomas, D. Urge-Vorsatz, G. J. M. Velders, T. Yokohata, T. Ziehn and Z. Nicholls, A perspective on the next generation of earth system model scenarios: towards representative emission pathways (reps), 2023.
- G. P. Peters, A. Al Khourdajie, I. Sognnaes and B. M. Sanderson, Ar6 scenarios database: an assessment of current practices and future recommendations, npj Clim. Action, 2023, 2, 31 CrossRef.
-
K. Szetey, E. Moallemi, S. Chakori and B. A. Bryan, Improving the relevance of the shared socioeconomic pathways for sustainability science, 2023 Search PubMed.
- G. Luderer, Z. Vrontisi, C. Bertram, O. Y. Edelenbosch, R. C. Pietzcker, J. Rogelj, H. Sytze De Boer, L. Drouet, J. Emmerling, O. Fricko, S. Fujimori, P. Havlík, G. Iyer, K. Keramidas, A. Kitous, M. Pehl, V. Krey, K. Riahi, B. Saveyn, M. Tavoni, D. P. Van Vuuren and E. Kriegler, Residual fossil CO2 emissions in 1.5–2 °C pathways, Nat. Clim. Change, 2018, 8(7), 626–633 CrossRef CAS.
-
H. U. Sverdrup, A. H. Olafsdottir and K. V. Ragnarsdottir. Development of a biophysical economics module for the global integrated assessment model WORLD7, Feedback Economics, Springer International Publishing, 2021, pp. 247–283 Search PubMed.
Footnotes |
† Discussion is still on-going in the research community about some subtle methodological issues on exactly how EROI at point of use should be formulated, but these do not affect the main argument being made here, i.e., that in order to be meaningful, all such comparisons should in fact be made at point of use. |
‡ The inclusion of storage devices in the system boundary (rather than at the level of an individual power generation technology), however, is more relevant at the country, regional, or grid level, because each technology, if deployed in isolation, would require some storage capacity to successfully keep up with the pattern of electricity demand. |
§ In this regard, it is worth noting that it has been estimated that, due to residual fossil emissions, 640-950 GtCO2 carbon dioxide removal (CDR), i.e., BECCS, DACCS and afforestation, will be required for a likely chance of limiting end-of-century warming to 1.5 °C, when strengthened pre-2030 mitigation action is combined with very stringent long-term policies.234 |
¶ The SFCIO-IAM204 and WORLD7235 are for instance only global models. |
|
This journal is © The Royal Society of Chemistry 2024 |
Click here to see how this site uses Cookies. View our privacy policy here.