Exploring sustenance: cereal legume combinations for vegan meat development
Received
15th May 2023
, Accepted 23rd October 2023
First published on 26th October 2023
Abstract
The rapidly increasing global population reached 8 billion in November 2022, which is further projected to reach 9.7 billion by 2050. The question of how to sustainably feed the growing population has become a major concern for many countries. In terms of the global protein supply, animal-based protein sources continue to play a dominant role, particularly in developed countries. However, there is growing interest in plant-based protein sources, particularly in the form of meat analogues, as a way to provide a more sustainable and ethical source of protein. To ensure an adequate supply of protein for the world, it's important to promote sustainable and equitable food systems that provide a variety of nutritious and affordable food options, including both animal and plant-based sources of protein. Additionally, investment in research and development of new plant-based protein sources, as well as new technologies to improve the efficiency and sustainability of animal agriculture, can help to ensure a secure and healthy protein supply for the world's growing population. Cereal and legume combinations play a critical role in the development of meat analogues because they provide an important source of protein that can be used to mimic the taste, texture, and nutritional properties of meat. When combined, cereal and legume products can provide a complete source of protein that is comparable to that found in animal-based products. In terms of developing meat analogues, cereal and legume combinations can be used to make products such as veggie burgers, meatless meatballs, and other plant-based meat alternatives. These products can be made using a variety of techniques, including grinding, texturizing, and extruding, to create a product that mimics the taste, texture, and nutritional properties of meat. The current article revolves around the theme of the potential of cereal legume combinations, current practices, challenges faced, novel ingredients and technological practices in developing sustainable meat analogues.
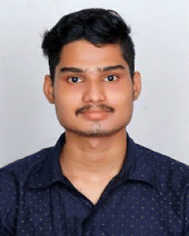 Kannan Vignesh | Mr Kannan Vignesh obtained his M.Sc. (Food Processing and Nutrition Science) from the Indian Institute of Engineering Science and Technology, Shibpur. He is currently working as a Junior Research Fellow in the Grain Science and Technology Division at the DRDO-Defence Food Research Laboratory, Mysore and pursuing his PhD (Food Science and Technology) at the University of Mysore, Mysuru. His contribution to the present study is preparation, creation and/or presentation of the published work, specifically writing the initial draft (including substantive translation), ideas and formulation or evolution of overarching research goals and aims. |
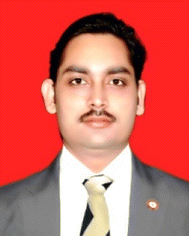 Dev Kumar Yadav | Mr Dev Kumar Yadav received his M.Sc. (Food Technology) from the University of Allahabad, Allahabad. He is presently working as Scientist ‘E’ at the Grain Science and Technology (GST) Division, Defence Food Research Laboratory (DRDO-DFRL), Mysore. He has published and presented his research findings in various platforms/journals of national and international repute (51). He is a recipient of prestigious awards viz. Laboratory Scientist of the Year by DRDO, Technology Group award (three times) from DRDO, Low-Cost Technology award from the Association of Food Scientists and Technologists of India – AFSTI, Mysore and Lucid Hydrocolloid award from the Association of Carbohydrate Chemistry & Technologists of India (ACCTI), Dehradun. His research areas include modification of starches for specific food applications, development of microwave-based disinfestation processes, instant and ready-to-eat foods based on cereals, pulses and millets, development of imitation milk products from decorticated sesame seeds, and design and development of ration storage systems for service-specific applications. His contribution to the present study is preparation, creation and/or presentation of the published work by those from the original research group, specifically critical review, commentary or revision – including pre- or post-publication stages. |
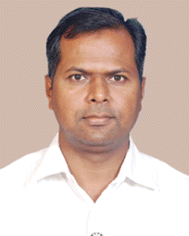 D. D. Wadikar | Dr Dadasaheb D. Wadikar obtained his B.Tech and M.Tech degrees from the College of Food Technology, VNMKV, Parbhani-Maharashtra and PhD (Food Science) from the University of Mysore, Mysore. He is currently working as Scientist-‘F’ at the DRDO-Defence Food Research Laboratory, Mysore. He has transferred 35 product technologies to different industries. He is the recipient of the AFSTI Young Scientist Award-2011 and the Laboratory Scientist of the Year award – 2017. His area of expertise is food technologies pertaining to grains including millets, oilseeds and spices. His contribution to the present study is oversight and leadership responsibility for the research activity planning and execution, including mentorship external to the core team. |
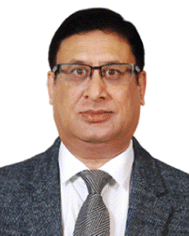 A. D. Semwal | Dr A. D. Semwal obtained his PhD (Chemistry) from the University of Mysore. He is currently working as the Director, DRDO-Defence Food Research Laboratory, Mysore. He has significantly contributed to the development of convenience foods and extrusion technology and extensively worked on the factors affecting the stability of various precooked dehydrated foods. His contribution includes management and coordination responsibility for the research activity planning and execution. |
Sustainability spotlight
In a world where the demand for sustainable food options continues to rise, the pressing concern lies in the unsustainable practices associated with conventional meat production. Recognizing the urgency of the matter, this research delves into the development of plant-based meat alternatives, focusing on the synergy of cereal and legume combinations. The sustainable advancement within this work is twofold. Firstly, it offers a viable solution to mitigate the environmental impacts of traditional meat production. By exploring the potential of cereal and legume-based vegan meats, this research paves the way for an eco-friendlier, resource-efficient, and lower-emission protein source. Secondly, it aligns explicitly with the United Nations' Sustainable Development Goals (SDGs), notably Goal 2 (zero hunger) and Goal 13 (climate action).
|
1. Introduction
For ages, proteins have been a vital constituent of human nutrition involved in innumerable structural and functional attributes of human metabolism. Traditionally, humans have relied on animal-based products especially meat as a primary source of high-quality proteins owing to its availability, organoleptic properties, minimal processing, and lesser concerns for antinutritional factors.1,2 However, fostering livestock animals for meat production is associated with extensive use of land and water, resulting in increased greenhouse gas emissions, thereby contributing to negative environmental impact.3,4 Protein supply is nutritionally and environmentally crucial in terms of carbon footprinting; in particular, meat consumption has the highest carbon footprint of 3.3 tons, while the vegan diet has the lowest at less than half of it.5–7 Besides, there are other prominent challenges related to meat consumption such as high-risk health illnesses and the detrimental impact on terrestrial and aquatic biodiversity. Furthermore, animal-based diets may increase the risk of zoonosis, exposure to veterinary antibiotics, and deadly ailments such as colorectal cancer and cardiovascular disease.8 The exponential population rise of the world, unforced limitations from animal-based products, and the ubiquitous trend of vegan communities led to an increase in the demand for the production of sustainable plant-based foods for mankind with sensorial attributes similar to animal meat.9 To counter these environmental concerns and alleviate public health concerns, plenty of plant-based sources that are alternatives to conventional meat products are being explored. Such sustainable foods are termed plant-based meat analogues (PBMAs), also known by various other terms such as mock meat, meat replacers, imitation meat, faux meat, meatless meat, and mimic animal meat across the globe.10–12 According to the results of a life cycle assessment, meat analogues have a significantly reduced carbon footprint and can thus provide environmentally lucrative alternatives to animal-derived meat.13,14 The plant-based diet has displayed several benefits, including lowering obesity, the presence of a high amount of nutritional ingredients such as vitamins, micro- and and macro-elements, blood pressure and cholesterol control, positive psychological outlook towards animal welfare.9,15,16 Conventional mock meats are produced using extrusion, but other novel techniques have also emerged to produce meat analogues such as the spinning technique, 3D printing, shear cell technology, and freeze structuring technique.17,18 The widespread use of meat across cuisines in the market holds a strong demand irrespective of the season. Consequently, PBMAs can be potentially exploited among a wide array of recipes across cuisines and diets.19 Since the past decade, there has been a bustle among the scientific community in investigating the potential vegan sources to mimic meat concerning its organoleptic, texture, and nutritional attributes.
Over time, various sources of cereal and/or legume combinations have been employed in developing PBMAs. Legumes, though rich in protein content lack methionine. Among them, soy has been the predominant legume source of interest in this regard. With its remarkable protein content and amino acid profile, soy has been serving as a potent ingredient in developing PBMAs followed by pea protein.20,21 Cereal proteins have been under-utilized in making PBMAs due to their incomplete amino acid content with regard to the Recommended Dietary Allowance, such as lysine. One exception among cereal proteins is wheat gluten, which has been used in combination with other protein isolates due to its elastic properties like meat.22,23 The current article intends to provide a review of the essence, constraints faced by current and novel ingredients, and technological applications in developing meat analogues using cereal legume combinations.
2. Importance of cereal legume combinations
Since time immemorial, the use of cereal legume combinations (CLCs) has been a part of the staple diet, depicting their invaluable significance to the human race.24 CLCs have been a quintessential part of global nutrition with their wide array of nutrients, especially in economically budding countries such as India where the consumption of nutritious food has often been a challenge.25 Thus, CLCs have always provided a cheaper nutritive alternative by supplying adequate nutrients to humans.26 In terms of production, global cereal and legume production have been increasing in recent years, driven by improvements in agricultural technology, increased investment in research and development, and a growing demand for food to meet the needs of a growing global population. In terms of consumption, the trend towards plant-based diets and growing interest in sustainable food options are likely to drive increased demand for cereals and legumes in the future. This, combined with the recognition of the health benefits of these foods, is likely to result in increased production and consumption of these crops in the years to come. The global production of cereal grains for the year 2022 as per the forecast of the Food and Agricultural Organization was 2756 million tonnes and consumption of 2777 million tonnes, respectively.27 The statistics portray the eminence of the consumption of cereal and legumes worldwide, provoking the research brains to keep their focus on this, in developing newer products and technologies. Cereals such as rice, wheat and its products have been eaten for ages but there is a rapid change in the trend of their consumption with the concept of whole cereal grains, which could potentially provide added nutrients such as dietary fibre and phytochemicals.28,29 Recently a few underutilized kinds of cereal such as oats and barley have gained interest owing to their health-promoting compounds viz. β-glucan.30,31 The idea of breakfast cereals is a common scenario in every household especially in Western countries, explaining its importance. Conversely, legumes are called poor man's meat and are consumed extravagantly across the globe due to their adequate protein, dietary complex carbohydrates, and B vitamins despite some anti-nutritional concerns.32,33 Legumes such as green gram, black gram, red gram, green peas, and groundnuts have been consumed extensively by hundreds of millions of the world population.34,35 Legumes due to the presence of anti-nutritional factors require additional processing steps viz. tannins, phytates, and cyanogens, whereas cereals require less complex mechanical processing methods to make them fit for consumption.36–38 Present-day society is more engrossed in the quality of protein being consumed than its quantity. The quality of protein depends on the digestibility of the protein and amino acid composition and is measured in terms of Protein Digestibility Corrected Amino Acid Score (PDCAAS) and Digestible Indispensable Amino Acid Score (DIAAS).39–41 It is well known that neither cereals nor legumes are balanced with all nine essential amino acids, with lysine and methionine as their limiting essential amino acids, respectively. Therefore, the imbalance in amino acid patterns can be compensated for by consuming a cereal–legume combination diet.23 Therefore, CLCs could serve as a potential source to target for the development of various sustainable foods such as meat analogues and the products can be established even more in a sustainable way by the implementation of green processing technologies.
3. Meat analogues based on cereal legume combinations
3.1. Extrusion technology
Extrusion technology has been a go-to technology for the development of meat analogues for decades by the virtue of its robustness, versatility, high scalability, low cost and employment of temperature, pressure, shear force, mixing, shaping, expansion, and cooling along with various physicochemical changes viz. denaturation, cross-linking, aggregation, fragmentation, complexation of proteins, denaturation and gelatinization of starch, reduction of anti-nutritional factors, increasing the digestibility and bioavailability of nutrients, and idiosyncratic flavour conferment due to the Maillard reaction.42–44 Nevertheless, extrusion technology has been in the routine of development of meat analogues particularly based on cereal legume combinations. Extrusion conditions were broadly classified into high-moisture extrusion (>40%) and low-moisture extrusion (<40%), based on the moisture content of the final extruded meat analogue.45,46 For the past half a decade, various new combinations of cereals and/or legume proteins were assessed for their potential in developing vegan meat using extrusion cooking, viz., oat-pea blend,47–51 soy-gluten,52–55 soy-gluten-corn starch,56–58 pea-gluten,59,60 pea-soy-gluten,61–65 soy-green gram-peanut-pea-gluten,45 rice-soy,66–68 soy-gluten-whey,69 faba bean-pea,70–72 peas-lentils-faba beans,73,74 pea-chickpea,75 soy-surimi,76 and rapeseed-yellow pea.77 Though possessing innumerable advantages, extrusion technology accounts for some drawbacks such as the degradation of vitamins and acrylamide concerns, thereby necessitating the emergence of novel technologies.
3.2. Other novel advanced methods and technological advancements
Although proactive research is being carried out on extrusion technology for meat analogues, there is a meagre shift in the focus on the development of innovative technologies keeping future needs in the picture. The effectiveness of novel advanced techniques in the development of meat analogues compared to traditional methods like extrusion technology is a multidimensional consideration. Traditional extrusion technology has been overexploited in industry, owing to its effectiveness in creating meat analogues with familiar textures and shapes. Its capability of scaling up to meet the demands of mass production efficiently makes it still the most predominantly used technology for the development of meat analogues. However, the prospects of meat analogues are evolving rapidly, and novel advanced techniques are pushing the boundaries to meet perfection. These techniques, such as micro-compounding and 3D printing, offer an attractive level of customization. They enable fine-tuned control over ingredient distribution at a micro-level, thereby offering innovative textures, flavours, and nutritional profiles that can closely mimic real meat. This is particularly valuable for catering to diverse consumer preferences and dietary requirements. Nevertheless, there are concerns about its employment. The cost of implementing advanced techniques, with their specialized equipment and research requirements, can be discouraging for many manufacturers. Scaling up from lab-scale experiments to commercial production presents challenges related to consistency, efficiency, and cost-effectiveness. The acceptance of novel meat analogues, which may differ in texture and appearance from traditional meat, requires consumer education and adaptation. Moreover, the success of these advanced techniques often relies on the availability of suitable ingredients, which may not be universally accessible. One such emerging technology with the widest research in developing meat analogues is 3D printing. The 3D printing technology involves the layer-by-layer deposition of material based on the consumers' desirability (Fig. 1). One of the most significant advantages of using 3D printing is its customization of appearance such as the shape and fibrous structure, thereby increasing the consumers' acceptability.78 Other advantages also include efficiency, complex shapes, and sustainability. The technology aligns with sustainability goals by reducing the environmental impact associated with meat production. Though possessing numerous benefits, 3D printing has its limitations such as scalability, cost, and speed. The production of 3D-printed meat analogues is a mammoth task for a larger population, thereby limiting its usage to the laboratory level.79 3D printing has been employed in the development of reduced-fat meat analogues from emulsion gels,80 textured soft hybrid meat analogues,81 application of additives viz. hydrocolloids such as gelatin, alginates,82–84 methylcellulose,85 xyloses,86 transglutaminase,87 and cocoa butter88 in improving the physicochemical properties of 3D printed meat analogues, and artificial muscle fibre insertion using coaxial nozzle assisted 3D printing.89 In another distinctive study, Baik et al.90 incorporated Gryllus bimaculatus powder into a soy-based 3D printed analogue. Woo Choi et al.91 developed a novel technology for high moisture texture soy protein using a vacuum packaging and pasteurized heat (vacuum-autoclaving) treatment. The treatment was observed to increase the disulfide bonds, α-helix, and β-sheets, despite decreasing the texturization index and hardness of the product. Krintiras et al.92 employed simple shear and heat in a Couette cell to develop a soy-gluten-based meat analogue and were successful in the development of fibrous anisotropic structures that mimicked meat tissue. The Couette cell consists of two concentric cylinders, typically made of glass or metal. The inner cylinder is solid and stationary, while the outer cylinder is rotatable. The space between these cylinders is filled with the fluid or material under investigation, in this case, a meat analogue mixture. When the outer cylinder is rotated, it imparts a shear force to the fluid between the cylinders. This shear force causes the material to flow in concentric layers, with the innermost layer moving at a lower speed (closer to the stationary inner cylinder) and the outermost layer moving at a higher speed (closer to the rotating outer cylinder). This creates a gradient of shear rates within the material. Like 3D printing, the technique is useful for laboratory-scale research, but scaling up the findings to industrial production can be challenging. Achieving the same texture and properties in large batches may require additional adjustments and considerations. Besides, using the Couette cell requires specialized equipment and expertise, which may not be readily available to all researchers or food manufacturers, thereby limiting its accessibility. Micro-compounding is another recently emerged novel technology, found to be proficient in the texturization of pea protein isolate. The process is more or less similar to the extrusion principle and can be regarded as miniaturized extrusion owing to its usage of a smaller quantity of samples (5–15 ml), which is almost impossible in the case of conventional extrusion, temperature, shear mixing and pressure. One of the strengths of micro-compounding is the ability to customize the ingredient mix. This allows food technologists to fine-tune the texture, flavour, and nutritional profile of the meat analogue to closely resemble real meat. The potential benefits include texture precision, flavour customization, uniformity, and reduced allergenicity. The limitations of the technology include equipment costs, ingredient sourcing, processing time, scalability, and restriction to flavour and taste.93 Chantanuson et al.94 developed a meat analogue based on soy-protein-based food gels by the freezing technique and evaluated its rheology and fibrous structure. In the freeze-structuring technique, the protein emulsion of the desired source is subjected to freezing to provide a more fibrous structure resembling meat. Furthermore, the ice crystals in the emulsion are removed to leave a more porous and fibrous structure, which mimics the fibrillar protein network of real meat (Fig. 2). Despite exhibiting high yield and product desirability, the technique possesses the same limitations as its co-evolving emerging techniques (Couette cell technique, 3D printing, and micro-compounding) such as scalability, high production cost, slow production rates.59
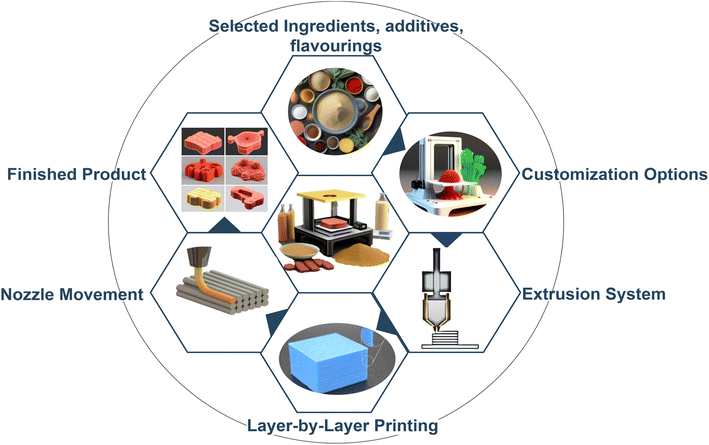 |
| Fig. 1 Salient features and process flow of 3D printing. | |
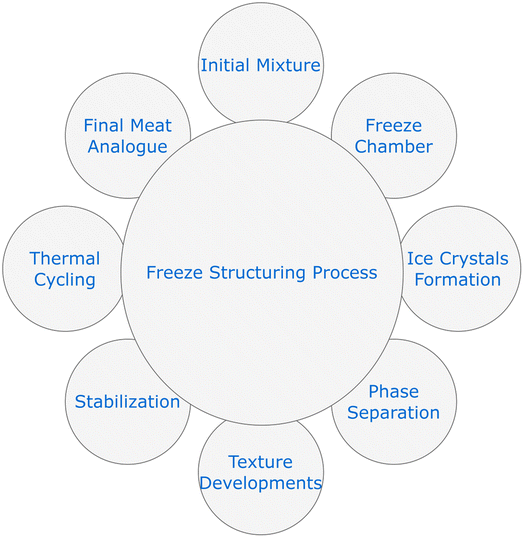 |
| Fig. 2 Various process steps of the freeze structuring technique. | |
4. Novel ingredients and additive combinations
Human psychology always tends to try out newer sources and look out for a change in the choice of ingredients that are used for the development of any product, which is also applicable in the case of meat analogues.95 This pushes the scientific community to put extra effort into searching for unexplored commodities. Apart from cereals and/or legume combinations, certain novel ingredients have been explored in developing meat analogues. These include the by-products obtained in the production of other value-added products viz. rice bran,96,97 sunflower meal98 and rapeseed meal77 by a conventional extrusion process. This approach reduces the procurement cost of the ingredient and the burden on the industries in the disposal of unnecessary by-products, which could be further exploited for the development of other value-added products. Another emerging commodity in the manufacture of vegan meat is microalgae amidst the speculations that agricultural activities of cereals/legume sources could also play a role in carbon footprinting. Microalgae are one of the noteworthy sources of protein with tremendous protein quality and quantity owing to their immaculate essential amino acid score (EAAI) (>100; the standard value of casein is 100).99 The EAAI of Chlorella sp. and Arthrospira platensis is 107.5 and 102.6 respectively, showing them to be a potentially prominent ingredient in this regard.100 Microalgae also possess an extensive range of pigments with health-benefiting nutraceutical activity, viz. β-carotene, astaxanthin, fucoxanthin, and phycocyanin.101,102 Despite having a resounding protein profile and other bioactive compounds, microalgae have certain constraints, which limit their employment in the development of PBMAs. The production process is energy-intensive and requires the attention of well-trained supervision.103 The acceptability of microalgae is highly challenging due to its objectionable earthy/fishy odour, which requires prospective technological intervention to make it suitable for consumption.104,105 Xia et al.106 studied the structural and rheological properties of pea protein-based meat analogues incorporated with Haematococcus pluvialis residues using high moisture extrusion. In a similar type of study, soy protein-based meat analogues were extruded with heterotrophically cultivated microalgae called Auxenochlorella protothecoides.107 Jin et al.108 developed a beef jerky analogue using a transglutaminase-treated yuba film, which significantly affected the cross-linking of soy proteins, thereby imparting the soy-based jerky with enhanced cohesiveness, cutting strength and texture. Kim et al.109 developed surimi-based crab meat with a potato starch solution layer analogue using coaxial extrusion 3D printing. Besides the exploration of novel ingredients, there were a plethora of efforts to improve the functional and nutritional properties of existing ingredients with the help of certain additives. Cho and Ryu110 studied the effect of the addition of mealworm larva (Tenebrio molitor) on a physicochemical soy-protein-based extruded meat analogue and observed that the DPPH radical scavenging activity, sulfur amino acid and glutamic acid content of the meat analogue increased with increase in the mealworm composition. Wen et al.86 evaluated the influence of the addition of xyloses on the physical characteristics (colour, texture, rheological, printing properties, and texture) of 3D-printed green gram protein-based meat analogues and witnessed that xylose significantly increased the shear modulus and printing behaviour of the protein gels used for 3D printing extrusion. Palanisamy et al.111 examined the changes in the properties of a soya protein meat analogue added with iota carrageenan and concluded that the additive improved the texturization properties of the meat analogue more than the control. In a similar type of study, Dou et al.112 evaluated the effect of a mixture of gums (iota carrageenan, carboxymethylcellulose sodium and sodium alginate) on the fibre formation properties of a high moisture extruded soy-based meat analogue. Peng et al.113 evaluated the effect of the addition of L-cysteine on the fibrous structure formation of pea protein and witnessed a decrease in fibrous formation when the additive concentration exceeded 0.09%. The influence of oyster mushroom addition on the physicochemical properties of a full fat soy-based analogue extruded burger patty and physical and microstructure properties of low-grade soy proteins, thereby indicating the potential of mushrooms in improving the quality of meat analogues.114–117 Palanisamy et al.118 included various ratios of spirulina/lupin protein mixtures and observed a significant increase in the properties such as in vitro protein digestibility, total phenolic content, and total flavonoid content. Xia et al.119 and Jeon et al.56 incorporated yeast into a soy protein-based meat analogue and concluded that yeast increased the fibrous structure of the products besides making them brighter and whiter. Similarly, Jeon et al.57 evaluated the influence of the addition of brewers' yeast on the quality characteristics of high-moisture extruded meat analogues. Mandliya et al.120 investigated the effect of the addition of mycelium (Pleurotus eryngii) in a pea-protein-based low moisture extruded meat analogue on the rehydration, physicochemical and functional properties. Bakhsh et al.121 studied the synergistic effect of lactoferrin and red yeast rice on the physicochemical properties of pea and soy protein-based-meat analogues. Szpicer et al.75 attempted to develop a meat analogue with enhanced antioxidant activity with the addition of micro-encapsulated anthocyanins and optimized the conditions to the inclusion of 2.74% of anthocyanins. Moll et al.122 compared the binding characteristics of a laccase-treated pea-protein-sugar beet pectin mixture with methylcellulose treated in a bacon-type meat analogue.
5. Consumer attitude towards cereal legume based meat analogues
Consumer behaviour towards meat analogues made using cereal legume combinations is influenced by various factors, including health concerns, environmental concerns, personal values and beliefs, and taste preferences.123 In recent years, there has been a growing trend towards plant-based diets, as consumers become more conscious of the environmental impact of animal-based products and the health benefits of consuming more plant-based foods.124 This has led to increased interest in meat analogues made using cereal legume combinations, as consumers look for sustainable and healthy alternatives to traditional animal-based products.125,126 However, taste remains an important factor for many consumers, and some may be hesitant to try meat analogues if they do not believe that the products will taste good.127 To overcome this, food manufacturers are working to improve the taste and texture of meat analogues, and many have been successful in creating products that are indistinguishable from traditional meat products.128 Additionally, there are also cultural and personal factors that may influence consumer behaviour towards meat analogues.129 For example, some consumers may be hesitant to try meat analogues due to a lack of familiarity with these products, while others may be more likely to try them due to personal values and beliefs.130 In conclusion, consumer behaviour towards meat analogues made using cereal legume combinations is influenced by a complex interplay of factors, including health and environmental concerns, personal values and beliefs, and taste preferences. To ensure that these products are widely adopted, it will be important for food manufacturers to continue to improve the taste and texture of meat analogues, while also educating consumers about the benefits of these products and addressing any misconceptions they may have.
6. Bottlenecks in the development of vegan meat
Though there is fast moving trend in society about the consumption of meat analogues, a wide range of challenges are being faced by researchers and industries from the selection of ingredients, complexity involved in the processing, palatability with the local cuisine, and consumer acceptability. Although cereal and legume combinations possess lots of essential nutritional dimensions, there are a few limitations faced by the researchers in employing this commodity for the development of meat analogues (Fig. 3). Cereals and legumes possess a wide range of anti-nutritional factors, thereby requiring additional processing steps to remove them.37,131,132 Among legumes, soybeans in the form of soy protein isolates have been widely employed due to their good protein quality and better functional properties followed by pea protein and chickpea protein.23 Unlike legumes, cereals, except for wheat, do not possess an adequate quantity of protein with the functional properties thus posing additional challenges in their utilization and failing to grab the interest of scientific and corporate communities in developing plant-based meat analogues due to their poor protein functionalities for large scale usage.133 Currently, soy protein isolates and vital wheat gluten are predominantly used, which are obtained after plenty of processing and purification stages inevitably increasing the production cost. In contrast, the quality of animal meat is relatively too high in comparison, thereby throwing a challenge to the research community to explore the technologies and resources that could match the protein quality of meat analogues to conventional meat without increasing the production cost.134 Besides their exemplary usage, soy and gluten have been two of the most common allergic concerns across the world. Approximately 3–10% of adults and 8% of children suffer from soy allergy and celiac disease worldwide, which shows the need to explore other potent ingredients for the development of vegan meat.135–137 The identification and procurement of raw materials that have the potential to replace meat tissue and fibre in terms of the texture and nutrition have always been a hurdle because there is not any such single source on this planet that is cheap and mimics meat in its physicochemical and organoleptic attributes.126 Therefore, an array of ingredients are being used in combination including cereal and legume sources making the preparation and processing stages complex. A commercially available plant-based analogue uses an average of 25–30 ingredients with different moisture contents, particle sizes, flow behaviours and solidity of the ingredient through various automated processes (conveyer and hopper).138 To ensure uninterrupted production flow, a crucial step called premixing of ingredients is another critical step in this regard. The ingredients involve a lot of soluble and insoluble fractions for meat structure construction, and some of them have to be distributed properly to avoid complications, thereby making the manufacturers look beyond the continuous process.139 On account of a lot of hurdles, manufacturers are being forced to choose the batch process, which decreases the productivity rate and increases the production cost. Another challenge in developing vegan meat is the consumer's acceptability in considering it as a convincing alternative to meat. Factors such as the appearance, flavour, taste, and texture that make the consumer perceive meat have always been a challenging task.140 The appearance (colour) is the first one to catch any person's interest in any food.141 The hurdle in this context is that cereal and legume-based ingredients normally used in the flour form vary from a white to a beige colour, which turns yellowish due to the Maillard reaction when cooking. Meat analogues should mimic conventional meat with red inside and brown outside when cooked.142 Another hurdle is the development of meat flavour. Cereal and legume origin ingredients range from sweet to beany flavour sometimes owing to the lipoxygenase activity on unsaturated fatty acids making their excess utilization limited.81 The process of meat flavour induction in the product is more tedious than the other perception factors. This is due to the presence of volatile flavour compounds that are particular to the natural meat tissue.143 A few compounds can be used in creating typical meat flavours viz. glutamates and ribonucleotides that in synchronization with the Maillard reaction develop an idiosyncratic flavour, thereby increasing the chances of consumers’ acceptability.144–146 The processing challenges of meat analogues have been minimal with the advent of twin-screw extruders, where proper mixing and uniform heat distribution have enabled their reliability in developing high-quality meat analogues with greater acceptance rates.
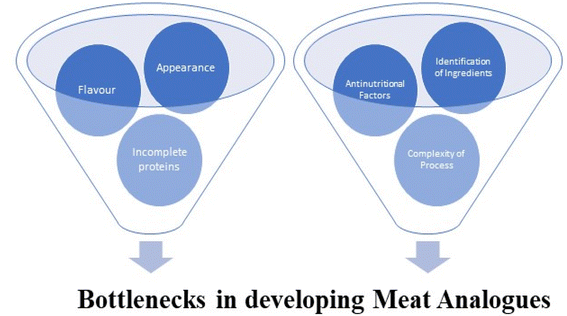 |
| Fig. 3 Challenges encountered during the development of vegan meat. | |
Addressing the complex challenges in developing meat analogues based on cereal and legume combinations requires a multifaceted approach. First, in tackling the presence of anti-nutritional factors in these ingredients, researchers should invest in advanced processing techniques to effectively remove these undesirable components, thereby streamlining the production process. Additionally, the issue of low protein content in cereals can be mitigated by combining them with legumes, creating a balanced protein profile for meat analogues. Research into isolating and enhancing the functionality of proteins from less-utilized cereal sources can also yield promising results.
The cost of production and quality comparison with animal meat pose significant hurdles. To address this, it's crucial to invest in ongoing research to discover cost-effective and sustainable protein sources that can match the quality of animal meat without inflating production costs. Continuous optimization of the production process is essential to strike a balance between quality and affordability. Furthermore, addressing allergic concerns related to soy and gluten necessitates exploration into alternative protein sources that are hypoallergenic and can effectively replace these ingredients. Ingredient complexity can be streamlined by standardizing combinations and investing in automation for ingredient handling and mixing, ensuring consistent product quality.
Consumer acceptance is a pivotal aspect of success in this industry. Achieving the desired appearance, flavour, and texture is crucial. Natural colourants can be utilized to mimic the appearance of conventional meat. Extensive research into flavour development using compounds like glutamates and ribonucleotides, in tandem with the Maillard reaction, can create distinctive meat-like flavours. Texture improvement through ingredient selection and process refinement is an ongoing endeavour.
7. Carbon footprint of vegan foods and adoption of green processing
Greenhouse gas (GHG) emission has been a human concern for decades contributing to global warming and an increase in sea level causing a threat to seashore areas in the near future. Food products right from the stages of their manufacture (growing, farming, and processing), transport, storage, consumption, and wastage have been inevitable stakeholders in deteriorating environmental health.147 Recently, there has been an inclusion of another term called carbon footprinting, which gives a clear indication of what scale of the effect of food products' impact on global environmental concerns can be measured.148 The carbon footprint is defined as activity pertaining to any individual, organization, product, or commodity that plays a role in releasing and accumulating carbon dioxide into the atmosphere.149,150 At present, the food supply chain holds a moderate stake of 30% of global carbon emissions (13.87 billion metric tons of carbon dioxide equivalents per annum) by human actions, which is expected to increase further with the increase of the world population and food requirements.27 Nevertheless, it is a two-way entanglement with the negative impact of climatic change on food supply causing massive agricultural loss.151 The recent trend of veganism gaining pace with the concept of being sustainable intrigues researchers in developing foods having smaller carbon footprinting making the food supply chain sustainable. Sustainability is a complicated term, which addresses the requirements of the existing generation without putting future generations at stake to meet their needs.152 Among the global GHG emissions of food products (per kilogram of food product), 5 out of the top 10 highest contributors belong to meat commodities (Fig. 4), with beef meat (beef herd) (99 kg CO2e) and lamb and mutton (39 kg CO2e) ranking first and third, respectively, indicating how crucial their contribution is to carbon footprinting. In contrast, cereals and legumes account for less than 4.5 kg CO2e, thereby making them a potential source in developing novel products by providing similar characteristics to meat in terms of nutrition and organoleptic attributes.153 Though it is slightly easier to opt for sustainable dietary habits among individuals seeking change in lifestyle preferences, it is quite unrealistic to expect a drastic shift in the dietary pattern of a large set of the population, especially where meat has been a part of the traditional cuisine.154,155 As mentioned before, apart from food consumption, food processing also extends a significant amount of contribution to global carbon footprinting.
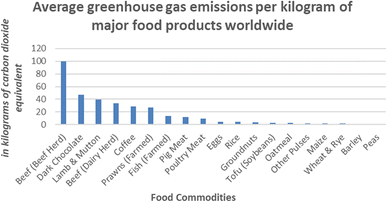 |
| Fig. 4 Carbon footprint comparison of meat with cereal and legumes (source: food product GHG emissions per kilogram worldwide153). | |
Therefore, the adoption of green processing could make the current worsening condition slightly better. Earlier, food processing was all about maintaining food safety, complying with regulatory stipulations, meeting consumer requirements, and profitability, whereas environmentally friendly practices have always been the least thing to bother about.156 Nowadays stringent compliance regulations from food safety and environment protection agencies make processing sustainability-oriented rather than profit-oriented.157 Green food processing technologies make use of minimal water and energy usage, providing high-quality products that are safe for disposal to the environment. Emerging green technologies include high hydrostatic pressure processing, pulsed electric field processing, plasma processing, the controlled pressure drop process, and supercritical fluid extraction.158,159 Thus food industries must step forward in choosing ingredients of a lower environmental impact without compromising nutrition and adopting green processing techniques for the development of vegan meat that could reduce the burden on the environment.
8. Missing links in the development of sustainable meat analogues
Developing sustainable meat analogues is an exciting area of research, but there are still some important research gaps and missing links that need to be addressed. One of the biggest challenges is achieving the right texture, as plant-based proteins often lack the fibrous texture of meat. Additionally, while plant-based proteins are often rich in protein, fibre, and micronutrients, they may not contain all of the essential amino acids found in meat, requiring more research to optimize the nutritional profile of meat analogues. The flavour is also a challenge, as meat analogues often lack the complex flavour profile of the meat. Cost is another issue, as sustainable meat analogues can be more expensive than conventional meat, which can limit their accessibility and adoption. Finally, despite growing interest in sustainable meat analogues, consumer acceptance remains a challenge, and more research is needed to understand consumer preferences and develop strategies for increasing consumer adoption. While interest in plant-based and sustainable foods is growing, consumers still have certain expectations around taste, texture, and nutritional value that need to be met. Researchers need to understand these preferences and develop strategies for improving the consumer acceptance of sustainable meat analogues, such as using marketing and education to increase the awareness of the benefits of these products and partnering with chefs and food companies to develop appealing and innovative product offerings. Addressing these research gaps and missing links will require continued investment in research and development, collaboration between industry and academia, and a focus on creating products that are not only sustainable but also appealing to consumers.
9. Conclusion and future prospects
The demand for sustainable foods calls for the exploration of every potential resource either in terms of ingredients or technologies leaving no stone unturned. The trend is driven by consumers seeking a healthy lifestyle change besides concerns about environmental issues. Meat analogues made up of cereal–legume combinations are cost-effective protein sources with negligible portions of saturated fat and cholesterol, providing health-benefiting compounds such as dietary fibres and phytochemicals. Nonetheless, the availability of meat analogues in the market is very scarce when compared with the market of meat products. The market prospects of meat analogues also require improvement owing to the consumer's attachment to conventional meat. It is difficult to know the key reason affecting the increase in consumption of meat analogues over meat. It is believed that the choice of ingredients plays a very crucial role in the consumer's acceptability. The marketing of a meat analogue has always been a tough job for food entrepreneurs due to the inclusion of the term meat. There were quite a few appeals to impose a ban on the use of the term “meat” in developing meat analogues, which is felt to be deceptive by some, but the European Parliament in the early 2020s ruled this plea in favour of the usage. There is a clear communication and research gap in knowing the requirements of meat analogues that the consumer is expecting apart from being a good protein source. The consumer is more likely to anticipate the extra added nutritional benefits viz. dietary fibre, fat-soluble vitamins as in meat, and phytochemicals.
In conclusion, the use of cereal and legume combinations for the development of sustainable meat analogues is a promising area for the future of food production. By combining these plant-based ingredients, it is possible to create products that mimic the taste, texture, and nutritional properties of meat, while providing a more sustainable and ethical alternative to traditional animal-based products.
Looking ahead, there is a significant opportunity for continued research and development in this area, including the development of new and improved plant-based protein sources, as well as the use of innovative processing techniques to improve the taste and texture of meat analogues. Additionally, as consumer demand for sustainable and ethical food options continues to grow, there is a clear need for continued investment in the development of meat analogues as a key part of a more sustainable and equitable food system.
To ensure the success of this field, it will be important to work closely with farmers, processors, and food manufacturers to ensure that plant-based protein sources are produced in a way that is both environmentally sustainable and economically viable. Additionally, collaboration with government, industry, and academic partners will be key to advancing the science and technology of meat analogue production and ensuring that these products are widely available and affordable for consumers.
In summary, the future of sustainable meat analogues made using cereal and legume combinations is bright, and there is great potential for continued innovation and growth in this field. By working together to create products that are delicious, nutritious, and environmentally friendly, it is possible to help build a more sustainable and equitable food system for future generations to make the world a better place to live.
Author contributions
Mr Kannan Vignesh: preparation, creation and/or presentation of the published work, specifically writing the initial draft (including substantive translation), ideas and formulation or evolution of overarching research goals and aims. Mr Dev Kumar Yadav: supervision of the planned work including review and commentary of the manuscript. Dr D. D. Wadikar: planning, guidance and assigning responsibility to the research activity. Dr A. D. Semwal: management and coordination responsibility of the research activity.
Conflicts of interest
There are no conflicts to declare.
References
- C. J. Bryant, Plant-based animal product alternatives are healthier and more environmentally sustainable than animal products, Future Foods, 2022, 6, 100174, DOI:10.1016/j.fufo.2022.100174.
- M. Henchion, M. Hayes, A. M. Mullen, M. Fenelon and B. Tiwari, Future Protein Supply and Demand: Strategies and Factors Influencing a Sustainable Equilibrium, Foods, 2017, 6, 53, DOI:10.3390/foods6070053.
- B. Machovina, K. J. Feeley and W. J. Ripple, Biodiversity conservation: The key is reducing meat consumption, Sci. Total Environ., 2015, 536, 419–431, DOI:10.1016/j.scitotenv.2015.07.022.
- M. Singh, N. Trivedi, M. K. Enamala, C. Kuppam, P. Parikh, M. P. Nikolova and M. Chavali, Plant-based meat analogue (PBMA) as a sustainable food: a concise review, Eur. Food Res. Technol., 2021, 247, 2499–2526, DOI:10.1007/s00217-021-03810-1.
- J. Poore and T. Nemecek, Reducing food's environmental impacts through producers and consumers, Science, 2018, 360, 987–992, DOI:10.1126/science.aaq0216.
- Q. Cao, J. Song, C. Liu and W. Yang, Evolving water, energy and carbon footprints in China's food supply chain, J. Cleaner Prod., 2023, 423, 138716, DOI:10.1016/j.jclepro.2023.138716.
- E. Röös, B. Bajželj, P. Smith, M. Patel, D. Little and T. Garnett, Protein futures for Western Europe: potential land use and climate impacts in 2050, Reg. Environ. Change, 2017, 17, 367–377, DOI:10.1007/s10113-016-1013-4.
- S. S. Anand, C. Hawkes, R. J. De Souza, A. Mente, M. Dehghan, R. Nugent, M.A. Zulyniak, T. Weis, A.M. Bernstein, R.M. Krauss, D. Kromhout, D.J.A Jenkins, V. Malik, M.A. Martinez-Gonzalez, D Mozaffarian, S. Yusuf, W.C. Willett and B.M. Popkin, Food Consumption and its Impact on Cardiovascular Disease: Importance of Solutions Focused on the Globalized Food System A Report from the Workshop Convened by the World Heart Federation, J. Am. Coll. Cardiol., 2015, 66(14), 1590–1614, DOI:10.1016/j.jacc.2015.07.050.
- S. van Vliet, F. D. Provenza and S. L. Kronberg, Health-Promoting Phytonutrients Are Higher in Grass-Fed Meat and Milk, Front. Sustain. Food Syst., 2021, 4 DOI:10.3389/fsufs.2020.555426.
- A. Bakhsh, S.-J. Lee, E.-Y. Lee, Y.-H. Hwang and S.-T. Joo, Traditional Plant-based Meat Alternatives, Current, and Future Perspective: A Review, Journal of Agriculture & Life Sciences, 2021, 55, 1–11, DOI:10.14397/JALS.2021.55.1.1.
- I. Ismail, Y. H. Hwang and S. T. Joo, Meat analog as future food: A review, J. Anim. Sci. Technol., 2020, 62, 111–120, DOI:10.5187/jast.2020.62.2.111.
- M. J. Sadler, Meat alternatives - Market developments and health benefits, Trends Food Sci. Technol., 2004, 15(5), 250–260, DOI:10.1016/j.tifs.2003.09.003.
- A. Detzel, M. Krüger, M. Busch, I. Blanco-Gutiérrez, C. Varela, R. Manners, J. Bez and E. Zannini, Life cycle assessment of animal-based foods and plant-based protein-rich alternatives: an environmental perspective, J. Sci. Food Agric., 2022, 102, 5098–5110, DOI:10.1002/jsfa.11417.
- S. Smetana, A. Mathys, A. Knoch and V. Heinz, Meat alternatives: life cycle assessment of most known meat substitutes, Int. J. Life Cycle Assess., 2015, 20, 1254–1267, DOI:10.1007/s11367-015-0931-6.
- V. Bouvard, D. Loomis, K. Z. Guyton, Y. Grosse, F. E. Ghissassi, L. Benbrahim-Tallaa, N. Guha, H. Mattock and K. Straif, Carcinogenicity of consumption of red and processed meat, Lancet Oncol., 2015, 16(16), 1599–1600, DOI:10.1016/S1470-2045(15)00444-1.
- B. L. Dekkers, R. M. Boom and A. J. van der Goot, Structuring processes for meat analogues, Trends Food Sci. Technol., 2018, 81, 25–36, DOI:10.1016/j.tifs.2018.08.011.
- J. Anu Bhushani and C. Anandharamakrishnan, Electrospinning and electrospraying techniques: Potential food based applications, Trends Food Sci. Technol., 2014, 38, 21–38, DOI:10.1016/j.tifs.2014.03.004.
- G. A. Krintiras, J. Gadea Diaz, A. J. Van Der Goot, A. I. Stankiewicz and G. D. Stefanidis, On the use of the Couette Cell technology for large scale production of textured soy-based meat replacers, J. Food Eng., 2016, 169, 205–213, DOI:10.1016/j.jfoodeng.2015.08.021.
- A. C. Hoek, P. A. Luning, P. Weijzen, W. Engels, F. J. Kok and C. de Graaf, Replacement of meat by meat substitutes. A survey on person- and product-related factors in consumer acceptance, Appetite, 2011, 56, 662–673, DOI:10.1016/j.appet.2011.02.001.
- C. Sun, J. Ge, J. He, R. Gan and Y. Fang, Processing, Quality, Safety, and Acceptance of Meat Analogue Products, Engineering, 2021, 7, 674–678, DOI:10.1016/j.eng.2020.10.011.
- A. Iqbal, I. A. Khalil, N. Ateeq and M. Sayyar Khan, Nutritional quality of important food legumes, Food Chem., 2006, 97, 331–335, DOI:10.1016/j.foodchem.2005.05.011.
- D. Anwar and G. El-Chaghaby, Nutritional quality, amino acid profiles, protein digestibility corrected amino acid scores and antioxidant properties of fried tofu and seitan, Journal of Faculty of Food Engineering, 2019, XVIII, 176–190 Search PubMed.
- P. Vasantha Kumari and N. Sangeetha, Nutritional significance of cereals and legumes based food mix- A review, Int. J. Agric. Sci., 2000, 3(1), 115–122, DOI:10.22573/spg.ijals.017.s12200075.
- V. V. Agte, M. K. Gokhale and S. A. Chiplonkar, Effect of natural fermentation on in vitro zinc bioavailability in cereal-legume mixtures, Int. J. Food Sci. Technol., 1997, 32(1), 29–32, DOI:10.1046/j.1365-2621.1997.00372.x.
- K. D. Kaur, A. Jha, L. Sabikhi and A. K. Singh, Significance of coarse cereals in health and nutrition: A review, J. Food Sci. Technol., 2014, 51, 1429–1441, DOI:10.1007/s13197-011-0612-9.
-
Y. Maphosa, V. A. Jideani, Y. Maphosa and V. A. Jideani, The Role of Legumes in Human Nutrition, IntechOpen, 2017, DOI:10.5772/intechopen.69127.
-
M. Torero, New FAO Analysis Reveals Carbon Footprint of Agri-Food Supply Chain, UN News, 2021 Search PubMed.
- I. Joye, Protein Digestibility of Cereal Products, Foods, 2019, 8, 199, DOI:10.3390/foods8060199.
- L. Dykes and L. W. Rooney, Sorghum and millet phenols and antioxidants, J. Cereal Sci., 2006, 44, 236–251, DOI:10.1016/j.jcs.2006.06.007.
- M. Henrion, C. Francey, K.-A. Lê and L. Lamothe, Cereal B-Glucans: The Impact of Processing and How It Affects Physiological Responses, Nutrients, 2019, 11, 1729, DOI:10.3390/nu11081729.
- M. Schmidt, Cereal beta-glucans: an underutilized health endorsing food ingredient, Crit. Rev. Food Sci. Nutr., 2022, 62, 3281–3300, DOI:10.1080/10408398.2020.1864619.
- M. D. Kristensen, N. T. Bendsen, S. M. Christensen, A. Astrup and A. Raben, Meals based on vegetable protein sources (beans and peas) are more satiating than meals based on animal protein sources (veal and pork) – a randomized cross-over meal test study, Food Nutr. Res., 2016, 60 DOI:10.3402/fnr.v60.32634.
- R. N. Tharanathan and S. Mahadevamma, Grain legumes—a boon to human nutrition, Trends Food Sci. Technol., 2003, 14, 507–518, DOI:10.1016/j.tifs.2003.07.002.
- S. S. Arya, A. R. Salve and S. Chauhan, Peanuts as functional food: a review, J. Food Sci. Technol., 2016, 53, 31–41, DOI:10.1007/s13197-015-2007-9.
- S. Langyan, P. Yadava, F. N. Khan, R. Bhardwaj, K. Tripathi, V. Bhardwaj, R. Bhardwaj, R. K. Gautam and A. Kumar, Nutritional and Food Composition Survey of Major Pulses Toward Healthy, Sustainable, and Biofortified Diets, Front. Sustain. Food Syst., 2022, 6, 878269, DOI:10.3389/fsufs.2022.878269.
-
S. Ram, S. Narwal, O. Gupta, V. Pandey and G. Singh, Anti-nutritional Factors and Bioavailability: Approaches, Challenges, and Opportunities, 2020, pp. 101–28, DOI:10.1016/B978-0-12-818444-8.00004-3.
- M. Samtiya, R. E. Aluko and T. Dhewa, Plant food anti-nutritional factors and their reduction strategies: an overview, Food Prod., Process. Nutr., 2020, 2(6) DOI:10.1186/s43014-020-0020-5.
- G. Sarwar Gilani, C. Wu Xiao and K. A. Cockell, Impact of antinutritional factors in food proteins on the digestibility of protein and the bioavailability of amino acids and on protein quality, Br. J. Nutr., 2012, 108(suppl 2), 315–332, DOI:10.1017/S0007114512002371.
- R. M. C. Ariëns, S. Bastiaan-Net, D. B. P. M. van de Berg-Somhorst, K. El Bachrioui, A. Boudewijn, R. T. M. van den Dool, G.A.H de Jong, H.J. Wichers and J.J. Mes, Comparing nutritional and digestibility aspects of sustainable proteins using the INFOGEST digestion protocol, J. Funct. Foods, 2021, 87(104748), 104748, DOI:10.1016/j.jff.2021.104748.
- G. Schaafsma, The protein digestibility-corrected amino acid score, J. Nutr., 2000, 130, 1865–1867, DOI:10.1093/jn/130.7.1865S.
- R. Sousa, R. Portmann, I. Recio, S. Dubois and L. Egger, Comparison of in vitro digestibility and DIAAR between vegan and meat burgers before and after grilling, Food Res. Int., 2023, 112569, DOI:10.1016/j.foodres.2023.112569.
- A. Sun, L. Chen, W. Wu, O. P. Soladoye, Y. Zhang and Y. Fu, The potential meat flavoring generated from Maillard reaction products of wheat gluten protein hydrolysates-xylose: Impacts of different thermal treatment temperatures on flavor, Food Res. Int., 2023, 165, 112512, DOI:10.1016/j.foodres.2023.112512.
- N. Gandhi, B. Singh, S. Sharma and S. Kapoor, Extrusion Process Optimization of Corn Starch to Develop Instant Vegetable Soup Mix, Int. J. Curr. Microbiol. Appl. Sci., 2018, 7(2) DOI:10.20546/ijcmas.2018.702.352.
-
M. A. Emin, 7 - Key technological advances of extrusion processing, in Food Engineering Innovations across the Food Supply Chain, ed. Juliano P., Buckow R., Nguyen M. H., Knoerzer K. and Sellahewa J., Academic Press, 2022, pp. 131–48, DOI:10.1016/B978-0-12-821292-9.00005-4.
- S. Samard and G.-H. Ryu, Physicochemical and functional characteristics of plant protein-based meat analogs, J. Food Process. Preserv., 2019, 43, e14123, DOI:10.1111/jfpp.14123.
-
F. Wild, Manufacture of Meat Analogues Through High Moisture Extrusion, Reference Module in Food Science, 2016, pp. 1–9, DOI:10.1016/B978-0-08-100596-5.03281-9.
- J. M. Ramos Diaz, K. Kantanen, J. M. Edelmann, H. Suhonen, T. Sontag-Strohm, K. Jouppila and V. Piironen, Fibrous meat analogues containing oat fiber concentrate and pea protein isolate: Mechanical and physicochemical characterization, Innovative Food Sci. Emerging Technol., 2022, 77(102954) DOI:10.1016/j.ifset.2022.102954.
- M. Immonen, A. Chandrakusuma, J. Sibakov, M. Poikelispää and T. Sontag-Strohm, Texturization of a Blend of Pea and Destarched Oat Protein Using High-Moisture Extrusion, Foods, 2021, 10, 1517, DOI:10.3390/foods10071517.
- A. Kaleda, K. Talvistu, H. Vaikma, M. L. Tammik, S. Rosenvald and R. Vilu, Physicochemical, textural, and sensorial properties of fibrous meat analogs from oat-pea protein blends extruded at different moistures, temperatures, and screw speeds, Future Foods, 2021, 4, 100092, DOI:10.1016/j.fufo.2021.100092.
- D. De Angelis, A. Kaleda, A. Pasqualone, H. Vaikma, M. Tamm, M.-L. Tammik, G. Squeo and C. Summo, Physicochemical and Sensorial Evaluation of Meat Analogues Produced from Dry-Fractionated Pea and Oat Proteins, Foods, 2020, 9(12), 1754, DOI:10.3390/foods9121754.
- A. Kaleda, K. Talvistu, M. Tamm, M. Viirma, J. Rosend, K. Tanilas, M. Kriisa, N. Part and M. Tammik, Impact of Fermentation and Phytase Treatment of Pea-Oat Protein Blend on Physicochemical, Sensory, and Nutritional Properties of Extruded Meat Analogs, Foods, 2020, 9(8), 1059, DOI:10.3390/foods9081059.
- D. Chen, D. Rocha-Mendoza, S. Shan, Z. Smith, I. García-Cano, J. Prost, R. Jimenez-Flores and O. Campanella, Characterization and Cellular Uptake of Peptides Derived from In Vitro Digestion of Meat Analogues Produced by a Sustainable Extrusion Process, J. Agric. Food Chem., 2022, 70(26), 8124–8133, DOI:10.1021/acs.jafc.2c01711.
- Z. Guo, F. Teng, Z. Huang, B. Lv, X. Lv, O. Babich, W. Yu, Y. Li, Z. Wang and L. Jiang, Effects of material characteristics on the structural characteristics and flavor substances retention of meat analogs, Food Hydrocolloids, 2020, 105, 105752, DOI:10.1016/j.foodhyd.2020.105752.
- J. H. Chiang, S. M. Loveday, A. K. Hardacre and M. E. Parker, Effects of soy protein to wheat gluten ratio on the physicochemical properties of extruded meat analogues, Food Struct., 2019, 19, 100102, DOI:10.1016/j.foostr.2018.11.002.
- B. L. Dekkers, M. A. Emin, R. M. Boom and A. J. van der Goot, The phase properties of soy protein and wheat gluten in a blend for fibrous structure formation, Food Hydrocolloids, 2018, 79, 273–281, DOI:10.1016/j.foodhyd.2017.12.033.
- Y.-H. Jeon, B.-J. Gu and G.-H. Ryu, Effect of Yeast Content on the Physicochemical Properties of Low-Moisture Extruded Meat Analog, J. Korean Soc. Food Sci. Nutr., 2022, 51(3), 271–277, DOI:10.3746/jkfn.2022.51.3.271.
- Y.-H. Jeon, B.-J. Gu and G.-H. Ryu, Effects of Brewer’s Spent Yeast Content on the Physicochemical Properties of Extruded High-Moisture Meat Analog, J. Korean Soc. Food Sci. Nutr., 2022, 51(10), 1084–1090, DOI:10.3746/jkfn.2022.51.10.1084.
- T.-T. Maung, B.-Y. Gu and G.-H. Ryu, Influence of extrusion process parameters on specific mechanical energy and physical properties of high-moisture meat analog, Int. J. Food Eng., 2021, 17, 149–157, DOI:10.1515/ijfe-2020-0042.
- O. Yuliarti, T. J. Kiat Kovis and N. J. Yi, Structuring the meat analogue by using plant-based derived composites, J. Food Eng., 2021, 288, 110138, DOI:10.1016/j.jfoodeng.2020.110138.
- H.-G. Zhu, H.-Q. Tang, Y.-Q. Cheng, Z.-G. Li and L.-T. Tong, Potential of preparing meat analogue by functional dry and wet pea (Pisum sativum) protein isolate, LWT--Food Sci. Technol., 2021, 148, 11702, DOI:10.1016/j.lwt.2021.111702.
- Y. Zhang and G.-H. Ryu, Effects of pea protein content and extrusion types on physicochemical properties and texture characteristic of meat analogs, JSFA Reports, 2023, 3, 30–40, DOI:10.1002/jsf2.97.
- A. Bakhsh, E.-Y. Lee, A. M. Bakry, D. Rathnayake, Y.-M. Son, S.-W. Kim, Y.-H. Hwang and S.-T. Joo, Synergistic effect of lactoferrin and red yeast rice on the quality characteristics of novel plant-based meat analog patties, LWT--Food Sci. Technol., 2022, 171, 114095, DOI:10.1016/j.lwt.2022.114095.
- S. J. E. Snel, Y. Bellwald, A. J. van der Goot and M. Beyrer, Novel rotating die coupled to a twin-screw extruder as a new route to produce meat analogues with soy, pea and gluten, Innovative Food Sci. Emerging Technol., 2022, 81, 03152, DOI:10.1016/j.ifset.2022.103152.
- C. Sun, J. Fu, Y. Chang, S. Li and Y. Fang, Structure Design for Improving the Characteristic Attributes of Extruded Plant-Based Meat Analogues, Food Biophys., 2022, 17, 137–149, DOI:10.1007/s11483-021-09692-w.
- F. K. G. Schreuders, B. L. Dekkers, I. Bodnár, P. Erni, R. M. Boom and A. J. van der Goot, Comparing structuring potential
of pea and soy protein with gluten for meat analogue preparation, J. Food Eng., 2019, 261, 32–39, DOI:10.1016/j.jfoodeng.2019.04.022.
- J.-W. Kim, B.-J. Gu and G.-H. Ryu, Effects of Isolated Rice Protein Addition on the Physicochemical Characteristics of Extruded Low-Moisture Meat Analog, J. Korean Soc. Food Sci. Nutr., 2022, 51(9), 976–984, DOI:10.3746/jkfn.2022.51.9.976.
- J. S. Lee, I. Choi and J. Han, Construction of rice protein-based meat analogues by extruding process: Effect of substitution of soy protein with rice protein on dynamic energy, appearance, physicochemical, and textural properties of meat analogues, Food Res. Int., 2022, 161, 111840, DOI:10.1016/j.foodres.2022.111840.
- J.-S. Lee, H. Oh, I. Choi, C. S. Yoon and J. Han, Physico-chemical characteristics of rice protein-based novel textured vegetable proteins as meat analogues produced by low-moisture extrusion cooking technology, LWT--Food Sci. Technol., 2022, 157, 113056, DOI:10.1016/j.lwt.2021.113056.
- P. Wittek, H. P. Karbstein and M. A. Emin, Blending proteins in high moisture extrusion to design meat analogues: Rheological properties, morphology development and product properties, Foods, 2021, 10(7) DOI:10.3390/foods10071509.
- K. Kantanen, A. Oksanen, M. Edelmann, H. Suhonen, T. Sontag-Strohm, V. Piironen, J. M. R. Diaz and K. Jouppila, Physical Properties of Extrudates with Fibrous Structures Made of Faba Bean Protein Ingredients Using High Moisture Extrusion, Foods, 2022, 11(9), 1280, DOI:10.3390/foods11091280.
- F. Ferawati, I. Zahari, M. Barman, M. Hefni, C. Ahlström, C. Witthöft and K. Östbring, High-moisture meat analogues produced from yellow pea and faba bean protein isolates/concentrate: Effect of raw material composition and extrusion parameters on texture properties, Foods, 2021, 10(4) DOI:10.3390/foods10040843.
- C. do Carmo, S. H. Knutsen, G. Malizia, T. Dessev, A. Geny, H. Zobel, K. S. Myhrer, P. Varela and S. Sahlstrom, Meat analogues from a faba bean concentrate can be generated by high moisture extrusion, Future Foods, 2021, 3, 100014, DOI:10.1016/j.fufo.2021.100014.
- T. Kim, R. Miller, H. Laird and M. N. Riaz, Beef flavor vegetable hamburger patties with high moisture meat analogs (HMMA) with pulse proteins-peas, lentils, and faba beans, Food Sci. Nutr., 2021, 9, 4048–4056, DOI:10.1002/fsn3.2172.
- T. Kim, M. N. Riaz, J. Awika and T. F. Teferra, The effect of cooling and rehydration methods in high moisture meat analogs with pulse proteins-peas, lentils, and faba beans, J. Food Sci., 2021, 86, 1322–1334, DOI:10.1111/1750-3841.15660.
- A. Szpicer, A. Onopiuk, M. Barczak and M. Kurek, The optimization of a gluten-free and soy-free plant-based meat analogue recipe enriched with anthocyanins microcapsules, LWT--Food Sci. Technol., 2022, 168, 113849, DOI:10.1016/j.lwt.2022.113849.
- Y. Zhang, J. Zhang, Q. Chen, N. He and Q. Wang, High-Moisture Extrusion of Mixed Proteins from Soy and Surimi: Effect of Protein Gelling Properties on the Product Quality, Foods, 2022, 11(10), 1397, DOI:10.3390/foods11101397.
- I. Zahari, F. Ferawati, J. K. Purhagen, M. Rayner, C. Ahlström, A. Helstad and K. Östbring, Development and Characterization of Extrudates Based on Rapeseed and Pea Protein Blends Using High-Moisture Extrusion Cooking, Foods, 2021, 10, 2397, DOI:10.3390/foods10102397.
- I. Dankar, A. Haddarah, F. El Omar, F. Sepulcre and M. Pujola, 3D printing technology: The new era for food customization and elaboration, Trends Food Sci. Technol., 2018, 75, 231–242, DOI:10.1016/j.tifs.2018.03.018.
- K. Ramachandraiah, Potential development of sustainable 3d-printed meat analogues: A review, Sustainability, 2021, 13(2) DOI:10.3390/su13020938.
- M. Shahbazi, H. Jäger, J. Chen and R. Ettelaie, Construction of 3D printed reduced-fat meat analogue by emulsion gels. Part II: Printing performance, thermal, tribological, and dynamic
sensory characterization of printed objects, Food Hydrocolloids, 2021, 121, 107054, DOI:10.1016/j.foodhyd.2021.107054.
- Y. Wang and C. Jian, Sustainable plant-based ingredients as wheat flour substitutes in bread making, npj Science of Food, 2022, 6(49) DOI:10.1038/s41538-022-00163-1.
- S. Leelapunnawut, L. Ngamwonglumlert, S. Devahastin, A. Derossi, R. Caporizzi and N. Chiewchan, Effects of Texture Modifiers on Physicochemical Properties of 3D-Printed Meat Mimics from Pea Protein Isolate-Alginate Gel Mixture, Foods, 2022, 11, 3947, DOI:10.3390/foods11243947.
- Y. Chen, M. Zhang and B. Bhandari, 3D Printing of Steak-like Foods Based on Textured Soybean Protein, Foods, 2021, 10, 2011, DOI:10.3390/foods10092011.
- J. Chen, T. Mu, D. Goffin, C. Blecker, G. Richard, A. Richel and E. Haubruge, Application of soy protein isolate and hydrocolloids based mixtures as promising food material in 3D food printing, J. Food Eng., 2019, 261, 76–86, DOI:10.1016/j.jfoodeng.2019.03.016.
- H. W. Kim, H. Bae and H. J. Park, Classification of the printability of selected food for 3D printing: Development of an assessment method using hydrocolloids as reference material, J. Food Eng., 2017, 215, 23–32, DOI:10.1016/j.jfoodeng.2017.07.017.
- Y. Wen, H. W. Kim and H. J. Park, Effect of xylose on rheological, printing, color, texture, and microstructure characteristics of 3D-printable colorant-containing meat analogs based on mung bean protein, Food Res. Int., 2022, 160, 111704, DOI:10.1016/j.foodres.2022.111704.
- Y. Wen, H. W. Kim and H. J. Park, Effects of transglutaminase and cooking method on the physicochemical characteristics of 3D-printable meat analogs, Innovative Food Sci. Emerging Technol., 2022, 81, 103114, DOI:10.1016/j.ifset.2022.103114.
- S. Wang and S. Liu, 3D Printing of Soy Protein- and Gluten-Based Gels Facilitated by Thermosensitive Cocoa Butter in a Model Study, ACS Food Sci. Technol., 2021, 1, 1990–1996, DOI:10.1021/acsfoodscitech.1c00311.
- H. J. Ko, Y. Wen, J. H. Choi, B. R. Park, H. W. Kim and H. J. Park, Meat analog production through artificial muscle fiber insertion using coaxial nozzle-assisted three-dimensional food printing, Food Hydrocolloids, 2021, 120, 106898, DOI:10.1016/j.foodhyd.2021.106898.
- W. Baik, J. Kim, G. Yoon, G. Kim, D. Kim and Y. Jeong, Textural and Sensory Characteristics of Soy Meat with the Addition of Gryllus bimaculatus Powder Produced Using a 3D Food Printer, J. Korean Soc. Food Sci. Nutr., 2022, 51(12), 1364–1369, DOI:10.3746/jkfn.2022.51.12.1364.
- H. Woo Choi, C. Ryoo, J. Hahn and Y. J. Choi, Development of a novel technology for high-moisture textured soy protein using a vacuum packaging and pressurized heat (vacuum-autoclaving) treatment, Food Chem., 2023, 399, 133887, DOI:10.1016/j.foodchem.2022.133887.
- G. A. Krintiras, J. Göbel, A. J. van der Goot and G. D. Stefanidis, Production of structured soy-based meat analogues using simple shear and heat in a Couette Cell, J. Food Eng., 2015, 160, 34–41, DOI:10.1016/j.jfoodeng.2015.02.015.
- C. F. Tingle, K. McClintic, A. J. Zervoudakis, B. J. Muhialdin and J. Ubbink, Texturization of pea protein isolate by micro compounding, Food Res. Int., 2023, 163, 112250, DOI:10.1016/j.foodres.2022.112250.
- R. Chantanuson, S. Nagamine, T. Kobayashi and K. Nakagawa, Preparation of soy protein-based food gels and control of fibrous structure and rheological property by freezing, Food Struct., 2022, 32, 100258, DOI:10.1016/j.foostr.2022.100258.
- M. C. Onwezen, E. P. Bouwman, M. J. Reinders and H. Dagevos, A systematic review on consumer acceptance of alternative proteins: Pulses, algae, insects, plant-based meat alternatives, and cultured meat, Appetite, 2021, 159, 105058, DOI:10.1016/j.appet.2020.105058.
- R. Jiang, Z. Xiao, J. Huo, H. Wang, H. Li, S. Su, Y. Duan and Y. Gao, Effects of rice bran content on
plant-based simulated meat: From the aspects of apparent properties and structural characteristics, Food Chem., 2022, 380, 131842, DOI:10.1016/j.foodchem.2021.131842.
- Z. Xiao, R. Jiang, J. Huo, H. Wang, H. Li, S. Su, Y. Gao and Y. Duan, Rice bran meat analogs: Relationship between extrusion parameters, apparent properties and secondary structures, LWT--Food Sci. Technol., 2022, 163, 113535, DOI:10.1016/j.lwt.2022.113535.
- W. Jia, I. R. Sutanto, M. Ndiaye, J. K. Keppler and A. J. van der Goot, Effect of aqueous ethanol washing on functional properties of sunflower materials for meat analogue application, Food Struct., 2022, 33, 100274, DOI:10.1016/j.foostr.2022.100274.
- Y. Wang, S. M. Tibbetts and P. J. McGinn, Microalgae as Sources of High-Quality Protein for Human Food and Protein Supplements, Foods, 2021, 10, 3002, DOI:10.3390/foods10123002.
- M. Kent, H. M. Welladsen, A. Mangott and Y. Li, Nutritional Evaluation of Australian Microalgae as Potential Human Health Supplements, PLoS One, 2015, 10, e0118985, DOI:10.1371/journal.pone.0118985.
- R. Sathasivam and J.-S. Ki, A Review of the Biological Activities of Microalgal Carotenoids and Their Potential Use in Healthcare and Cosmetic Industries, Mar. Drugs, 2018, 16, 26, DOI:10.3390/md16010026.
- M. V. Vieira, I. P. Turkiewicz, K. Tkacz, C. Fuentes-Grünewald, L. M. Pastrana, P. Fuciños, A. Wojdylo and P. Nowicka, Microalgae as a Potential Functional Ingredient: Evaluation of the Phytochemical Profile, Antioxidant Activity and In-Vitro Enzymatic Inhibitory Effect of Different Species, Molecules, 2021, 26(24), 7593, DOI:10.3390/molecules26247593.
- P. J. l. B. Williams and L. M. L. Laurens, Microalgae as biodiesel & biomass feedstocks: Review & analysis of the biochemistry, energetics & economics, Energy Environ. Sci., 2010, 3, 554–590, 10.1039/B924978H.
- M. Isleten Hosoglu, Aroma characterization of five microalgae species using solid-phase microextraction and gas chromatography–mass spectrometry/olfactometry, Food Chem., 2018, 240, 1210–1218, DOI:10.1016/j.foodchem.2017.08.052.
- J. L. Pérez-Lloréns, Microalgae: From staple foodstuff to avant-garde cuisine, Int. J. Gastron. Food Sci., 2020, 21, 100221, DOI:10.1016/j.ijgfs.2020.100221.
- S. Xia, Y. Xue, C. Xue, X. Jiang and J. Li, Structural and rheological properties of meat analogues from Haematococcus pluvialis residue-pea protein by high moisture extrusion, LWT--Food Sci. Technol., 2022, 154, 112756, DOI:10.1016/j.lwt.2021.112756.
- M. P. Caporgno, L. Böcker, C. Müssner, E. Stirnemann, I. Haberkorn, H. Adelmann, S. Handschin, E. J. Windhab and A. Mathys, Extruded meat analogues based on yellow, heterotrophically cultivated Auxenochlorella protothecoides microalgae, Innovative Food Sci. Emerging Technol., 2020, 59, 102275, DOI:10.1016/j.ifset.2019.102275.
- Y. Jin, K. Y. Song and Y. Kim, A soy-based jerky made from transglutaminase-treated yuba film, J. Food Process. Preserv., 2022, 46(12) DOI:10.1111/jfpp.17180.
- S. M. Kim, H. W. Kim and H. J. Park, Preparation and characterization of surimi-based imitation crab meat using coaxial extrusion three-dimensional food printing, Innovative Food Sci. Emerging Technol., 2021, 71, 102711, DOI:10.1016/j.ifset.2021.102711.
- S. Y. Cho and G. H. Ryu, Effects of mealworm larva composition and selected process parameters on the physicochemical properties of extruded meat analog, Food Sci. Nutr., 2021, 9, 4408–4419, DOI:10.1002/fsn3.2414.
- M. Palanisamy, S. Töpfl, K. Aganovic and R. G. Berger, Influence of iota carrageenan addition on the properties of soya protein meat analogues, LWT--Food Sci. Technol., 2018, 87, 546–552, DOI:10.1016/j.lwt.2017.09.029.
- W. Dou, X. Zhang, Y. Zhao, Y. Zhang, L. Jiang and X. Sui, High moisture extrusion cooking on soy proteins: Importance influence of gums on promoting the fiber formation, Food Res. Int., 2022, 156, 111189, DOI:10.1016/j.foodres.2022.111189.
- H. Peng, J. Zhang, S. Wang, M. Qi, M. Yue, S. Zhang, J. Song, C. Wang, D. Zhang, X. Wang and C. Ma, High moisture extrusion of pea protein: Effect of l-cysteine on product properties and the process forming a fibrous structure, Food Hydrocolloids, 2022, 129, 107633, DOI:10.1016/j.foodhyd.2022.107633.
- S. Cho and G. Ryu, Effects of oyster mushroom addition on quality characteristics of full fat soy-based analog burger patty by extrusion process, J. Food Process Eng., 2022, 46(10) DOI:10.1111/jfpe.14128.
- P. Moorthi, C. A. A. Bakar, M. R. Ismail-Fitry and I. Ismail, Physicochemical and Sensory Characteristics of Meatless Nuggets of Boiled Chickpea and in Combination with Oyster Mushroom, Malays. Appl. Biol., 2022, 51, 17–25, DOI:10.55230/mabjournal.v51i6.2325.
- S. Y. Cho and G.-H. Ryu, Effects of mushroom composition on the quality characteristics of extruded meat analog, Korean J. Food Sci. Technol., 2020, 52, 357–362, DOI:10.9721/KJFST.2020.52.4.357.
- M. Mohamad Mazlan, R. A. Talib, N. L. Chin, R. Shukri, F. S. Taip, M. Z. Mohd Nor and N. Abdullah, Physical and Microstructure Properties of Oyster Mushroom-Soy Protein Meat Analog via Single-Screw Extrusion, Foods, 2020, 9(8), 1023, DOI:10.3390/foods9081023.
- M. Palanisamy, S. Töpfl, R. G. Berger and C. Hertel, Physico-chemical and nutritional properties of meat analogues based on Spirulina/lupin protein mixtures, Eur. Food Res. Technol., 2019, 245, 1889–1898, DOI:10.1007/s00217-019-03298-w.
- S. Xia, S. Shen, J. Song, K. Li, X. Qin, X. Jiang, C. Xue and Y. Xue, Physicochemical and structural properties of meat analogues from yeast and soy protein prepared via high-moisture extrusion, Food Chem., 2023, 402, 134265, DOI:10.1016/j.foodchem.2022.134265.
- S. Mandliya, A. Pratap-Singh, S. Vishwakarma, C. G. Dalbhagat and H. N. Mishra, Incorporation of Mycelium (Pleurotus eryngii) in Pea Protein Based Low Moisture Meat Analogue: Effect on Its Physicochemical, Rehydration and Structural Properties, Foods, 2022, 11(16), 2476, DOI:10.3390/foods11162476.
- A. Bakhsh, E.-Y. Lee, A. M. Bakry, D. Rathnayake, Y.-M. Son, S.-W. Kim, Y.-H. Hwang and S.-T. Joo, Synergistic effect of lactoferrin and red yeast rice on the quality characteristics of novel plant-based meat analog patties, LWT--Food Sci. Technol., 2022, 171, 114095, DOI:10.1016/j.lwt.2022.114095.
- P. Moll, H. Salminen, L. Stadtmueller, C. Schmitt and J. Weiss, Comparison of Binding Properties of a Laccase-Treated Pea Protein–Sugar Beet Pectin Mixture with Methylcellulose in a Bacon-Type Meat Analogue, Foods, 2023, 12, 85, DOI:10.3390/foods12010085.
- E. Röös, A. de Groote and A. Stephan, Meat tastes good, legumes are healthy and meat substitutes are still strange - The practice of protein consumption among Swedish consumers, Appetite, 2022, 174, 106002, DOI:10.1016/j.appet.2022.106002.
- A. Alcorta, A. Porta, A. Tárrega, M. D. Alvarez and M. Pilar Vaquero, Foods for plant-based diets: Challenges and innovations, Foods, 2021, 10(2), 293, DOI:10.3390/foods10020293.
- M. Estell, J. Hughes and S. Grafenauer, Plant protein and plant-based meat alternatives: Consumer and nutrition professional attitudes and perceptions, Sustainability, 2021, 13(3) DOI:10.3390/su13031478.
-
S. Kumar, V. Kumar, R. Sharma, A. A. Paul, P. Suthar and R. Saini, Plant Proteins as Healthy, Sustainable and Integrative Meat Alternates, IntechOpen, 2020, DOI:10.5772/intechopen.94094.
- M. Fiorentini, A. J. Kinchla and A. A. Nolden, Role of sensory evaluation in consumer acceptance of plant-based meat analogs and meat extenders: a scoping review, Foods, 2020, 9(9) DOI:10.3390/foods9091334.
- N. R. Rubio, N. Xiang and D. L. Kaplan, Plant-based and cell-based approaches to meat production, Nat. Commun., 2020, 11, 6276, DOI:10.1038/s41467-020-20061-y.
- A. Pakseresht, S. Ahmadi Kaliji and M. Canavari, Review of factors affecting consumer acceptance of cultured meat, Appetite, 2022, 170, 105829, DOI:10.1016/j.appet.2021.105829.
- C. Bryant, K. Szejda, N. Parekh, V. Desphande and B. Tse, A Survey of Consumer Perceptions of Plant-Based and Clean Meat in the USA, India, and China, Front. Sustain. Food Syst., 2019, 3 DOI:10.3389/fsufs.2019.00011.
- S. Emire, Y. Jha and F. Mekam, Role of Anti-nutritional Factors in Food Industry, Beverage and Food World, 2013, 23–28 Search PubMed.
- H. Hamid, N. Thakur and P. Kumar, Anti-nutritional factors, their adverse effects and need for adequate processing to reduce them in food, AgricINTERNATIONAL, 2017, 4, 56, DOI:10.5958/2454-8634.2017.00013.4.
- K. S. Poutanen, A. O. Kårlund, C. Gómez-Gallego, D. P. Johansson, N. M. Scheers, I. M. Marklinder, A.K. Eriksen, P.C. Silventoinen, E. Norlund, N. Sozer, K.J. Hanhineva, M. Kolehmainen and R. Landberg, Grains – a major source of sustainable protein for health, Nutr. Rev., 2022, 80(6), 1648–1663, DOI:10.1093/nutrit/nuab084.
- J. He, N. M. Evans, H. Liu and S. Shao, A review of research on plant-based meat alternatives: Driving forces, history, manufacturing, and consumer attitudes, Compr. Rev. Food Sci. Food Saf., 2020, 19(5), 2639–2656, DOI:10.1111/1541-4337.12610.
-
H. Akhondi and A. B. Ross, Gluten Associated Medical Problems, StatPearls Publishing, Treasure Island, FL, 2022 Search PubMed.
- M. Messina and C. Venter, Recent Surveys on Food Allergy Prevalence, Nutr. Today, 2020, 55, 22, DOI:10.1097/NT.0000000000000389.
- E. N. Mills, K. Adel-Patient, H. Bernard, M. Loose, N. Gillard, A.-C. Huet, C. Larré, C. Nitride, R. Pilolli, O. Tranquet, C. V. Pouke and L. Monaci, Detection and Quantification of Allergens in Foods and Minimum Eliciting Doses in Food-Allergic Individuals (ThRAll), J. AOAC Int., 2019, 102(5), 1346–1353, DOI:10.5740/jaoacint.19-0063.
- K. Kyriakopoulou, J. K. Keppler and A. J. van der Goot, Functionality of Ingredients and Additives in Plant-Based Meat Analogues, Foods, 2021, 10, 600, DOI:10.3390/foods10030600.
- A. Singh and N. Sit, Meat Analogues: Types, Methods of Production and Their Effect on Attributes of Developed Meat Analogues, Food Bioprocess Technol., 2022, 15, 1–19, DOI:10.1007/s11947-022-02859-4.
- F. Michel, C. Hartmann and M. Siegrist, Consumers' associations, perceptions and acceptance of meat and plant-based meat alternatives, Food Qual. Prefer., 2021, 87, 104063, DOI:10.1016/j.foodqual.2020.104063.
- C. Spence, X. Wan, A. Woods, C. Velasco, J. Deng, J. Youssef and O. Deroy, On tasty colours and colourful tastes? Assessing, explaining, and utilizing crossmodal correspondences between colours and basic tastes, Flavour, 2015, 4, 23, DOI:10.1186/s13411-015-0033-1.
- H. J. Lee, H. I. Yong, M. Kim, Y.-S. Choi and C. Jo, Status of meat alternatives and their potential role in the future meat market — A review, Asian-Australas. J. Anim. Sci., 2020, 33, 1533–1543, DOI:10.5713/ajas.20.0419.
- M. I. Khan, C. Jo and M. R. Tariq, Meat flavor precursors and factors influencing flavor precursors–A systematic review, Meat Sci., 2015, 110, 278–284, DOI:10.1016/j.meatsci.2015.08.002.
- A. Afzal, F. Saeed, M. Afzaal, A. A. Maan, A. Ikram, M. Hussain, I. Usman, Y. A. Shah and W. Anjum, The chemistry of flavor formation in meat and meat products in response to different thermal and non-thermal processing techniques: An overview, J. Food Process. Preserv., 2022, 46(10), e16847, DOI:10.1111/jfpp.16847.
-
J. S. Elmore and D. S. Mottram, Flavour development in meat, Improving the Sensory and Nutritional Quality of Fresh Meat, 2009, pp. 111–146, DOI:10.1533/9781845695439.1.111.
- P. Kale, A. Mishra and U. S. Annapure, Development of vegan meat flavour: A review on sources and techniques, Future Foods, 2022, 5, 100149, DOI:10.1016/j.fufo.2022.100149.
-
FCRN report Cooking up a Storm: Food, Greenhouse Gas Emissions and Our Changing Climate, International Journal of Climate Change Strategies and Management, 2009, 1, DOI:10.1108/ijccsm.2009.41401bae.006.
- L. H. Udara Willhelm Abeydeera, J. Wadu Mesthrige and T. I. Samarasinghalage, Global Research on Carbon Emissions: A Scientometric Review, Sustainability, 2019, 11, 3972, DOI:10.3390/su11143972.
- F. Görkem ÜÇTUĞ, D. Günaydin, B. Hünkar and C. Öngelen, Carbon footprints of omnivorous, vegetarian, and vegan diets based on traditional Turkish cuisine, Sustain. Prod. Consum., 2021, 26, 597–609, DOI:10.1016/j.spc.2020.12.027.
- I. Shabir, K. K. Dash, A. H. Dar, V. K. Pandey, U. Fayaz, S. Srivastava and R Nisha, Carbon footprints evaluation for sustainable food processing system development: A comprehensive review, Future Foods, 2023, 7, 100215, DOI:10.1016/j.fufo.2023.100215.
- A. Veeramani, G. M. Dias and S. I. Kirkpatrick, Carbon footprint of dietary patterns in Ontario, Canada: A case study based on actual food consumption, J. Cleaner Prod., 2017, 162, 1398–1406, DOI:10.1016/j.jclepro.2017.06.025.
- G. Thoma, S. W. Ellsworth and M. J. Yan, Chapter 1: Principles of Green Food Processing (Including Lifecycle Assessment and Carbon Footprint), RSC Green Chemistry, 2018, 2018, 1–52, 10.1039/9781782626596-00001.
-
Food product GHG emissions per kilogram worldwide, Statista, 2018, https://ourworldindata.org/grapher/ghg-per-kg-poore Search PubMed.
- M. Perignon, F. Vieux, L. G. Soler, G. Masset and N. Darmon, Improving diet sustainability through evolution of food choices: Review of epidemiological studies on the environmental impact of diets, Nutr. Rev., 2017, 75(1), 2–17, DOI:10.1093/nutrit/nuw043.
- E. Röös, H. Karlsson, C. Witthöft and C. Sundberg, Evaluating the sustainability of diets–combining environmental and nutritional aspects, Environ. Sci. Policy, 2015, 47, 157–166, DOI:10.1016/j.envsci.2014.12.001.
- I. Vågsholm, N. S. Arzoomand and S. Boqvist, Food Security, Safety, and Sustainability—Getting the Trade-Offs Right, Front. Sustain. Food Syst., 2020, 4(16) DOI:10.3389/fsufs.2020.00016.
-
A. Uçar, M. V. Yilmaz, F. P. Çakıroğlu, A. Uçar, M. V. Yilmaz and F. P. Çakıroğlu, Food Safety – Problems and Solutions, IntechOpen, 2016, DOI:10.5772/63176.
- J. I. Boye and Y. Arcand, Current Trends in Green Technologies in Food Production and Processing, Food Eng. Rev., 2013, 5, 1–17, DOI:10.1007/s12393-012-9062-z.
- F. Islam, F. Saeed, M. Afzaal, A. Ahmad, M. Hussain, M. A. Khalid, S. A. Saewan and A. O. Khashroum, Applications of green technologies-based approaches for food safety enhancement: A comprehensive review, Food Sci. Nutr., 2022, 10(9), 2855–2867, DOI:10.1002/fsn3.2915.
|
This journal is © The Royal Society of Chemistry 2024 |
Click here to see how this site uses Cookies. View our privacy policy here.